Abstract
Brown adipose tissue (BAT) is a specialized organ responsible for thermogenesis, a process required for maintaining body temperature. Since the discovery that BAT and brite/beige cells are functional in adult humans, many studies have been focusing on the physiology and functionality of this organ. The brain is controlling the maintenance of body temperature through a complex neuronal network. One of the candidates to modulate thermogenesis at central level is glucagon-like peptide-1 (GLP-1), with GLP-1 receptors widely expressed throughout the brain. Our group has recently reported that stimulation of brain GLP-1 receptors in the ventromedial nucleus of the hypothalamus is essential for the activation not only of BAT thermogenesis, but also browning of white fat. Notably, both actions are mediated by specific inhibition of the energy sensor AMP-activated protein kinase (AMPK). In this commentary, we summarize the latest results on this topic, as well as the potential clinical relevance of the brain GLP-1 system to treat obesity.
The physiological relevance of the brown adipose tissue (BAT) in humans has been a matter of controversy during decades. Initially, it was thought that BAT was relevant only in rodents, hibernating mammals, and newborn humans.Citation1–4 However, novel evidence has clearly demonstrated that BAT is located in dispersed areas of the body in adult humans.Citation2,5–8 Recently, 2 reports indicated that both classical brown and recruitable brite/beige adipocytes may be contained in adipose tissue,Citation9,10 with the latter initially reported to appear in response to thermogenic stimuli in white adipose tissue (WAT) due to the so-called ‘browning’ process.Citation11-13
The brain plays an essential role in the maintenance of temperature.Citation14,15 Among the numerous brain areas involved in this function, the hypothalamus occupies a key position. The arcuate nucleus (ARC), dorsomedial nucleus (DMH), lateral hypothalamic area (LHA), paraventricular nucleus (PVH) and the ventromedial nucleus (VMH) have all been demonstrated to modulate BAT.Citation14,15 Indeed, these hypothalamic areas are not directly connected to the BAT, and a network of other brain sites, such as the preoptic area (POA) and rostral raphe pallidus (rRPa), is also involved in this regulation. The fact that the network involved in thermoregulation is large and complex indicates its physiological relevance and that a wide range of factors will be able to affect BAT function. Among those factors we can find neurotransmitters, glucose and different hormones.Citation14,15
One of those factors is the hormone glucagon-like peptide 1 (GLP-1), a posttranslational product of proglucagon, that is endogenously released mainly from the ileum after the ingestion of nutrients. When postprandial GLP-1 increases it augments glucose-induced insulin release.Citation16,17 In line with this, the secretion of GLP-1 is impaired in patients with type II diabetes mellitus and obesity.Citation18,19 In addition to its role in insulin secretion, GLP-1 has many other biological actions, and the GLP-1 receptor (GLP1-R) is expressed in organs like pancreatic islets, kidney, lung, heart, stomach, intestine, pituitary, or skin.Citation18 GLP-1 is also expressed in the brain, where GLP-1 receptors are also abundant.Citation20,21 Recent evidence has shown that brain GLP-1 modulates different metabolic actions, of which one is the regulation of BAT thermogenesis.Citation22 Intracerebroventricular (ICV) injection of GLP-1 reduced body weight and increased BAT thermogenesis independent of changes in feeding and insulin responsiveness. Consistently, the expression of genes involved in the activation of the thermogenic program, such as those coding for peroxisome proliferator-activated receptor-gamma co-activator-1alpha (PGC1α) and uncoupling protein-1 (UCP1), were up-regulated in the BAT of wild type mice centrally treated with GLP-1 receptor agonists.Citation22 In contrast, mice lacking the GLP-1 receptor did not show any alteration in BAT temperature or BAT gene expression after the stimulation of the brain GLP-1 system. The connection between the brain and the BAT seems to be the sympathetic nervous system, since acute central injection of GLP-1R and glucagon receptor agonists increases electrophysiological activity of the sympathetic fibers that innervate the iBAT.Citation22
The neuroanatomical organization of the core thermoregulatory network involved in the actions of GLP-1 remains largely unknown, but a recent study from our group intended to show the first clues in this aspect. By doing an acute central injection of a GLP-1 receptor agonist, namely liraglutide, we confirmed previous results indicating that the brain GLP-1 system increases the thermogenic activation of BAT independently of feeding behavior.Citation23 In addition, we also observed that the central injection of liraglutide increased browning of WAT. As it is well known that within the central nervous system (CNS), numerous neuronal populations express GLP-1R, including hypothalamic nuclei crucial for the regulation of energy balance,Citation21,24 we next investigated which hypothalamic area was mediating the central effects of liraglutide on BAT and WAT. Thus, we specifically injected liraglutide into the following hypothalamic nuclei in rats; arcuate nucleus (ARC), LHA, PVH, DMH, or VMH, all of which express GLP-1R in a greater or lesser extent. Interestingly, only the specific stimulation of the GLP-1 system within the VMH was able to recapitulate the effects found after ICV injection of liraglutide.Citation23
A role of the VMH is in thermoregulation is supported by anatomical data, demonstrating a link between the VMH and BAT.Citation25 VMH neurons relay to modulate the sympathetic nervous system in brainstem areas such as the raphe pallidus (RPa) and inferior olive (IO), 2 nuclei which have been functionally linked to the regulation of BAT thermogenesis.Citation26–28 Gene-modulation studies also support a role for the VMH in thermoregulation, with VMH-specific knockout of steroidogenic factor-1 (SF-1) displaying lower energy expenditure and expression of UCP1 in BAT.Citation29,30 In this sense, our group and others have previously reported that AMPK in the VMH is a key negative regulator of sympathetically activated BAT thermogenesis, integrating peripheral signals, such as thyroid hormone, estradiol, leptin, and bone morphogenetic protein 8b (BPM8b), as well as drugs such as nicotine.Citation31-34 Liraglutide is now a new member of this list, because mice treated centrally with liraglutide showed lower levels of hypothalamic pAMPK and its downstream target pACC in comparison to saline-treated mice.Citation23 Consistently, both the pharmacological AMPK activator AICAR and an adenoviral vector encoding constitutively active isoforms of the catalytic subunit AMPKα injected into the VMH, were able to completely blunt the actions of liraglutide on BAT and WAT. Altogether these results indicate that brain GLP-1 actions on BAT thermogenesis and WAT browning are mediated by a reduction in hypothalamic AMPK, specifically within the VMH.Citation23
It is important to highlight that these results indicate that GLP-1 activation in the VMH not only modulates BAT thermogenesis (an effect shown for several other factors, such as thyroid hormones, estrogens, leptin BMP8b, nicotine, etc.), but also controls the thermogenic program in WAT (not demonstrated for the other factors). So far, not many studies have assessed the central control of browning of white fat. In one study, the brain SIRT1 was demonstrated to play an important role. The lack of SIRT1 in POMC neurons caused a reduction in sympathetic nerve activity, brown-fat-like characteristics and UCP-1 expression in perigonadal WAT.Citation35 Another recent and elegant study also indicated that O-GlcNAc transferase (OGT) in agouti-related peptide (AgRP) neurons within the ARC suppress browning of white fat.Citation36
A key question is if the mechanistic aspects related to brain GLP-1 and thermogenesis will be of potential clinical relevance. Liraglutide improves glycemic control in type 2 diabetic patients with the additional benefits of weight loss and a low risk of hypoglycemia.Citation37,38 As GLP-1R agonists start to be included in treatment guidelines, they are generally being recommended as second- or third-line therapies after the failure of one or more oral antidiabetic drugs.Citation39 Besides its well-known anti-diabetic properties, liraglutide has been recommended for approval by the US Food and Drug Administration (FDA) committee also for the treatment of obesity. The specific proposed indication is “for chronic weight management in individuals with a body mass index of 30 kg/m2 or greater, or 27 kg/m2 or greater in the presence of at least 1 weight-related comorbidity.” In an analysis involving 3731 patients, the liraglutide group lost an average 8% of body weight vs 2.6% with placebo at 56 weeks, thereby meeting the FDA benchmark for weight-loss drugs of a 5% difference between active treatment and placebo. Whether this decrease in body weight is linked to decreased food intake or increase energy expenditure is not yet clear in human subjects.Citation40 As a pilot study, we provided data from a cohort of obese patients with T2D who had been treated for 1 y with the antidiabetic drugs metformin and metformin in combination with the GLP-1R agonists exenatide and liraglutide. As expected, after 1 y of antidiabetic treatment, all study groups showed a decrease in fasting plasma glucose and insulin concentrations. In addition, the groups treated with metformin combined with exenatide or liraglutide showed a significant decrease in BMI and in total body fat percentage and a significant increase in fat free mass.Citation23 Accordingly, resting energy expenditure adjusted for fat free mass was significantly increased in patients treated with metformin in combination with exenatide or liraglutide; but not in patients treated only with metformin. Although it is still too early to state that the hypothalamic stimulation of the GLP-1 receptors is, at least partially, responsible of the anti-obesity effects of this drug, we cannot rule out the possibility that an increase in resting energy expenditure might be mediated by peripheral stimulation of GLP-1 receptors. Anyhow, taking into account all our findings, rats and humans, it is tempting to suggest that in addition to its anorexigenic effect, liraglutide works through specific central pathway to increase energy expenditure that ultimately lead to a further reduction in body weight. Rodent studies assessing the same drug combinations used in patients using both wild type mice and brain specific GLP-1 receptor knockout mice will be needed to solve this question. The relevance of the central effects of GLP1-agonists is highlighted by the findings that liraglutide treatment of mice lacking GLP-1 receptors in the brain improved glucose metabolism, which occurred without any major changes in food intake or body weight in animals fed a chow diet or high fat diet.Citation41 However, the actions of liraglutide on the browning of white fat were not tested in this experiment.
Whether the same mechanisms are shared between liraglutide and new peptides that are agonists to both GLP-1- and other related hormone receptors remains to be fully established. Initial reports using this strategy showed very promising results. A peptide with agonism at the glucagon and GLP-1 receptors reduced body weight gain, improved glucose metabolism and ameliorated hepatic steatosis in diet-induced obese mice.Citation42 Another peptide with potent co-agonism at both GLP-1 and glucose-dependent insulinotropic polypeptide (GIP) was efficient in several models of obesity and diabetes, including rodents, monkeys and humans.Citation43 Although these peptides were not tested at central level, it seems plausible to hypothesize that part of their metabolic actions may be mediated by the CNS, but again, further studies are needed to confirm at what extent the stimulation of brain GLP-1 receptors is necessary for the efficacy of these new promising therapeutic strategies. In addition, these findings raise the possibility that other well documented effects of GLP-1 receptor activation such as gastrointestinal motility, gastrointestinal secretion, blood pressure, modulation of innate immune-mediated inflammation or bone metabolism could also be exerted to some extent at central level (). In summary, based on their incretin effects, potent GLP-1 agonists were developed and are at present at the forefront in the management of type 2 diabetic patients. Clinical experience indicated that these compounds could also suitable for the treatment of obesity, which has now been well documented. In parallel, studies carried out in experimental animals demonstrate the likelihood that many of the beneficial actions of GLP-1 agonists on energy homeostasis are exerted at central level. Noteworthy, the findings that hypothalamic GLP-1 receptors, leading to AMPK inhibition and subsequent activation of BAT thermogenesis and WAT browning open up a new paradigm regarding the mechanisms by which GLP-1 exert a beneficial effect in obesity.
Figure 1. Schematic representation of the biological action of the brain GLP-1 system. Brain GLP-1 reduces food intake through both homeostatic and hedonic mechanisms; increases thermogenesis in brown adipose tissue; and stimulates lipid mobilization in white adipose tissue. Under hyperglycemic and hyperinsulinemic conditions, brain GLP-1 increases insulin secretion in pancreatic islets; reduces glucose utilization in muscle and suppresses hepatic glucose production. Whether other important actions of GLP-1 such as cardiovascular or bone metabolism are also regulated by brain GLP-1 remain to be elucidated.
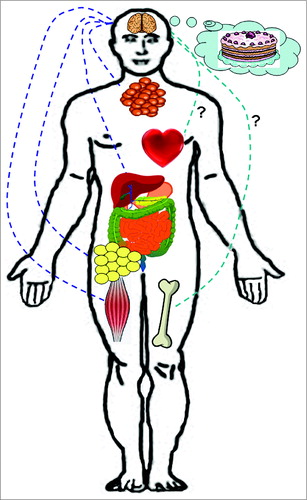
Disclosure of Potential Conflicts of Interest
No potential conflicts of interest were disclosed.
Funding
This work has been supported by grants from Ministerio de Economia y Competitividad (CD: BFU2011-29102; RN: BFU2012-35255), Xunta de Galicia (ML: 2012-CP070; RN: EM 2012/039 and 2012-CP069), Fondo de Investigaciones Sanitarias (ML: PI12/01814), Centro de Investigación Biomédica en Red (CIBER) de Fisiopatología de la Obesidad y Nutrición (CIBERobn). CIBERobn is an initiative of the Instituto de Salud Carlos III (ISCIII) of Spain which is supported by FEDER funds. The research leading to these results has also received funding from the European Community's Seventh Framework Programme under the following grant: CD, ML and RN: FP7/2007-2013: n° 245009: NeuroFAST and ERC StG 2011-OBESITY53-281408 to RN.
References
- Lowell BB, Spiegelman BM. Towards a molecular understanding of adaptive thermogenesis. Nature 2000; 404:652-60; PMID:10766252
- van Marken Lichtenbelt WD, Vanhommerig JW, Smulders NM, Drossaerts JM, Kemerink GJ, Bouvy ND, Schrauwen P, Teule GJ. Cold-activated brown adipose tissue in healthy men. N Engl J Med 2009; 360:1500-8; PMID:19357405; http://dx.doi.org/10.1056/NEJMoa0808718
- Tran TT, Kahn CR. Transplantation of adipose tissue and stem cells: role in metabolism and disease. Nat Rev Endocrinol 2010; 6:195-213; PMID:20195269; http://dx.doi.org/10.1038/nrendo.2010.20
- Whittle AJ, Lopez M, Vidal-Puig A. Using brown adipose tissue to treat obesity–the central issue. Trends Mol Med 2011; 17:405-11; PMID:21602104
- Zingaretti MC, Crosta F, Vitali A, Guerrieri M, Frontini A, Cannon B, Nedergaard J, Cinti S. The presence of UCP1 demonstrates that metabolically active adipose tissue in the neck of adult humans truly represents brown adipose tissue. FASEB J 2009; 23:3113-20; PMID:19417078; http://dx.doi.org/10.1096/fj.09-133546
- Nedergaard J, Bengtsson T, Cannon B. Unexpected evidence for active brown adipose tissue in adult humans. Am J Physiol Endocrinol Metab 2007; 293:E444-52; PMID:17473055; http://dx.doi.org/10.1152/ajpendo.00691.2006
- Cypess AM, Lehman S, Williams G, Tal I, Rodman D, Goldfine AB, Kuo FC, Palmer EL, Tseng YH, Doria A, et al. Identification and importance of brown adipose tissue in adult humans. N Engl J Med 2009; 360:1509-17; PMID:19357406; http://dx.doi.org/10.1056/NEJMoa0810780
- Virtanen KA, Lidell ME, Orava J, Heglind M, Westergren R, Niemi T, Taittonen M, Laine J, Savisto NJ, Enerbäck S, et al. Functional brown adipose tissue in healthy adults. N Engl J Med 2009; 360:1518-25; PMID:19357407; http://dx.doi.org/10.1056/NEJMoa0808949
- Wu J, Bostrom P, Sparks LM, Ye L, Choi JH, Giang AH, Khandekar M, Virtanen KA, Nuutila P, Schaart G, et al. Beige adipocytes are a distinct type of thermogenic fat cell in mouse and human. Cell 2012; 150:366-76; PMID:22796012; http://dx.doi.org/10.1016/j.cell.2012.05.016
- Jespersen NZ, Larsen TJ, Peijs L, Daugaard S, Homoe P, Loft A, de Jong J, Mathur N, Cannon B, Nedergaard J, et al. A classical brown adipose tissue mRNA signature partly overlaps with brite in the supraclavicular region of adult humans. Cell Metab 2013; 17:798-805; PMID:23663743; http://dx.doi.org/10.1016/j.cmet.2013.04.011
- Walden TB, Hansen IR, Timmons JA, Cannon B, Nedergaard J. Recruited vs. nonrecruited molecular signatures of brown, "brite," and white adipose tissues. Am J Physiol Endocrinol Metab 2012; 302:E19-31; PMID:21828341; http://dx.doi.org/10.1152/ajpendo.00249.2011
- Wu J, Cohen P, Spiegelman BM. Adaptive thermogenesis in adipocytes: is beige the new brown? Genes Dev 2013; 27:234-50; PMID:23388824; http://dx.doi.org/10.1101/gad.211649.112
- Giralt M, Villarroya F. White, brown, beige/brite: different adipose cells for different functions? Endocrinology 2013; 154:2992-3000; PMID:23782940; http://dx.doi.org/10.1210/en.2013-1403
- Contreras C, Gonzalez F, Fernø J, Dieguez C, Rahmouni K, Nogueiras R, Lopez M. The brain and brown fat. Ann Med 2014:1-19; PMID:24915455; http://dx.doi.org/10.3109/07853890.2014.919727
- Morrison SF, Madden CJ, Tupone D. Central neural regulation of brown adipose tissue thermogenesis and energy expenditure. Cell Metab 2014; 19:741-56; PMID:24630813; http://dx.doi.org/10.1016/j.cmet.2014.02.007
- Mojsov S, Weir GC, Habener JF. Insulinotropin: glucagon-like peptide I (7-37) co-encoded in the glucagon gene is a potent stimulator of insulin release in the perfused rat pancreas. J Clin Invest 1987; 79:616-9; PMID:3543057; http://dx.doi.org/10.1172/JCI112855
- Calanna S, Christensen M, Holst JJ, Laferrere B, Gluud LL, Vilsboll T, Knop FK. Secretion of glucagon-like peptide-1 in patients with type 2 diabetes mellitus: systematic review and meta-analyses of clinical studies. Diabetologia 2013; 56:965-72; PMID:23377698; http://dx.doi.org/10.1007/s00125-013-2841-0
- Campbell JE, Drucker DJ. Pharmacology, physiology, and mechanisms of incretin hormone action. Cell Metab 2013; 17:819-37; PMID:23684623; http://dx.doi.org/10.1016/j.cmet.2013.04.008
- Goke R, Fehmann HC, Goke B. Glucagon-like peptide-1(7-36) amide is a new incretin/enterogastrone candidate. Eur J Clin Invest 1991; 21:135-44; PMID:1647951; http://dx.doi.org/10.1111/j.1365-2362.1991.tb01802.x
- Gu G, Roland B, Tomaselli K, Dolman CS, Lowe C, Heilig JS. Glucagon-like peptide-1 in the rat brain: distribution of expression and functional implication. J Compar Neurol 2013; 521:2235-61; PMID:23238833; http://dx.doi.org/10.1002/cne.23282
- Richards P, Parker HE, Adriaenssens AE, Hodgson JM, Cork SC, Trapp S, Gribble FM, Reimann F. Identification and characterization of GLP-1 receptor-expressing cells using a new transgenic mouse model. Diabetes 2014; 63:1224-33; PMID:24296712; http://dx.doi.org/10.2337/db13-1440
- Lockie SH, Heppner KM, Chaudhary N, Chabenne JR, Morgan DA, Veyrat-Durebex C, Ananthakrishnan G, Rohner-Jeanrenaud F, Drucker DJ, DiMarchi R, et al. Direct control of brown adipose tissue thermogenesis by central nervous system glucagon-like peptide-1 receptor signaling. Diabetes 2012; 61:2753-62; PMID:22933116; http://dx.doi.org/10.2337/db11-1556
- Beiroa D, Imbernon M, Gallego R, Senra A, Herranz D, Villarroya F, Serrano M, Fernø J, Salvador J, Escalada J, et al. GLP-1 agonism stimulates brown adipose tissue thermogenesis and browning through hypothalamic AMPK. Diabetes 2014; 63:3346-58; PMID:24917578; http://dx.doi.org/10.2337/db14-0302
- Shimizu I, Hirota M, Ohboshi C, Shima K. Identification and localization of glucagon-like peptide-1 and its receptor in rat brain. Endocrinology 1987; 121:1076-82
- Bamshad M, Song CK, Bartness TJ. CNS origins of the sympathetic nervous system outflow to brown adipose tissue. Am J Physiol 1999; 276:R1569-78; PMID:10362733
- Cano G, Passerin AM, Schiltz JC, Card JP, Morrison SF, Sved AF. Anatomical substrates for the central control of sympathetic outflow to interscapular adipose tissue during cold exposure. J Compar Neurol 2003; 460:303-26; PMID:12692852; http://dx.doi.org/10.1002/cne.10643
- Morrison SF. RVLM and raphe differentially regulate sympathetic outflows to splanchnic and brown adipose tissue. Am J Physiol 1999; 276:R962-73; PMID:10198373
- Uno T, Shibata M. Role of inferior olive and thoracic IML neurons in nonshivering thermogenesis in rats. Am J Physiol Regul Integr Comp Physiol 2001; 280:R536-46; PMID:11208585
- Kim KW, Zhao L, Donato J Jr., Kohno D, Xu Y, Elias CF, Lee C, Parker KL, Elmquist JK. Steroidogenic factor 1 directs programs regulating diet-induced thermogenesis and leptin action in the ventral medial hypothalamic nucleus. Proc Natl Acad Sci U S A 2011; 108:10673-8; PMID:21636788; http://dx.doi.org/10.1073/pnas.1102364108
- Jo YH. Endogenous BDNF regulates inhibitory synaptic transmission in the ventromedial nucleus of the hypothalamus. J Neurophysiol 2012; 107:42-9; PMID:21994261; http://dx.doi.org/10.1152/jn.00353.2011
- Lopez M, Varela L, Vazquez MJ, Rodriguez-Cuenca S, Gonzalez CR, Velagapudi VR, Morgan DA, Schoenmakers E, Agassandian K, Lage R, et al. Hypothalamic AMPK and fatty acid metabolism mediate thyroid regulation of energy balance. Nat Med 2010; 16:1001-8; PMID:20802499; http://dx.doi.org/10.1038/nm.2207
- Whittle AJ, Carobbio S, Martins L, Slawik M, Hondares E, Vazquez MJ, Morgan D, Csikasz RI, Gallego R, Rodriguez-Cuenca S, et al. BMP8B increases brown adipose tissue thermogenesis through both central and peripheral actions. Cell 2012; 149:871-85; PMID:22579288; http://dx.doi.org/10.1016/j.cell.2012.02.066
- Martinez de Morentin PB, Whittle AJ, Fernø J, Nogueiras R, Dieguez C, Vidal-Puig A, López M. Nicotine induces negative energy balance through hypothalamic AMP-activated protein kinase. Diabetes 2012; 61:807-17; PMID:22315316; http://dx.doi.org/10.2337/db11-1079
- Tanida M, Yamamoto N, Shibamoto T, Rahmouni K. Involvement of hypothalamic AMP-activated protein kinase in leptin-induced sympathetic nerve activation. PloS one 2013; 8:e56660; PMID:23418591; http://dx.doi.org/10.1371/journal.pone.0056660
- Ramadori G, Fujikawa T, Fukuda M, Anderson J, Morgan DA, Mostoslavsky R, Stuart RC, Perello M, Vianna CR, Nillni EA, et al. SIRT1 deacetylase in POMC neurons is required for homeostatic defenses against diet-induced obesity. Cell Metab 2010; 12:78-87; PMID:20620997; http://dx.doi.org/10.1016/j.cmet.2010.05.010
- Ruan HB, Dietrich MO, Liu ZW, Zimmer MR, Li MD, Singh JP, et al. O-GlcNAc Transferase Enables AgRP Neurons to Suppress Browning of White Fat. Cell 2014; 159:306-17; PMID:25303527; http://dx.doi.org/10.1016/j.cell.2014.09.010
- Marre M, Shaw J, Brandle M, Bebakar WM, Kamaruddin NA, Strand J, Zhang K, Yin R, Wu J, Horvath TL, et al. Liraglutide, a once-daily human GLP-1 analogue, added to a sulphonylurea over 26 weeks produces greater improvements in glycaemic and weight control compared with adding rosiglitazone or placebo in subjects with Type 2 diabetes (LEAD-1 SU). Diabet Med 2009; 26:268-78; PMID:19317822; http://dx.doi.org/10.1111/j.1464-5491.2009.02666.x
- Astrup A, Rossner S, Van Gaal L, Rissanen A, Niskanen L, Al Hakim M, Madsen J, Rasmussen MF, Lean ME; NN8022-1807 Study Group. Effects of liraglutide in the treatment of obesity: a randomised, double-blind, placebo-controlled study. Lancet 2009; 374:1606-16; PMID:19853906; http://dx.doi.org/10.1016/S0140-6736(09)61375-1
- Nathan DM, Buse JB, Davidson MB, Ferrannini E, Holman RR, Sherwin R, Zinman B; American Diabetes Association; European Association for the Study of Diabetes. Medical management of hyperglycaemia in type 2 diabetes mellitus: a consensus algorithm for the initiation and adjustment of therapy: a consensus statement from the American Diabetes Association and the European Association for the Study of Diabetes. Diabetologia 2009; 52:17-30; PMID:18941734; http://dx.doi.org/10.1007/s00125-008-1157-y
- Torekov SS, Madsbad S, Holst JJ. Obesity–an indication for GLP-1 treatment? Obesity pathophysiology and GLP-1 treatment potential. Obesity Rev 2011; 12:593-601; PMID:21401851; http://dx.doi.org/10.1111/j.1467-789X.2011.00860.x
- Sisley S, Gutierrez-Aguilar R, Scott M, D'Alessio DA, Sandoval DA, Seeley RJ. Neuronal GLP1R mediates liraglutide's anorectic but not glucose-lowering effect. J Clin Invest 2014; 124:2456-63; PMID:24762441; http://dx.doi.org/10.1172/JCI72434
- Day JW, Ottaway N, Patterson JT, Gelfanov V, Smiley D, Gidda J, Findeisen H, Bruemmer D, Drucker DJ, Chaudhary N, et al. A new glucagon and GLP-1 co-agonist eliminates obesity in rodents. Nat Chem Biol 2009; 5:749-57; PMID:19597507; http://dx.doi.org/10.1038/nchembio.209
- Finan B, Ma T, Ottaway N, Muller TD, Habegger KM, Heppner KM, Kirchner H, Holland J, Hembree J, Raver C, et al. Unimolecular dual incretins maximize metabolic benefits in rodents, monkeys, and humans. Sci Transl Med 2013; 5:209ra151; PMID:24174327; http://dx.doi.org/10.1126/scitranslmed.3007218