Abstract
The potentially oncogenic mevalonate pathway provides building blocks for protein prenylation and induces cell proliferation and as such is an important therapeutic target. Among mevalonate metabolites, only isopentenyl pyrophosphate (IPP) has been considered to be an immunologically relevant antigen for primate-specific, innate-like Vγ9Vδ2 T cells with antitumor potential. We show here that Vγ9Vδ2 T cells pretreated with the stress-related, inflammasome-dependent cytokine interleukin 18 (IL-18) were potently activated not only by IPP but also by all downstream isoprenoid pyrophosphates that exhibit combined features of antigens and cell-extrinsic metabolic cues. Vγ9Vδ2 T cells induced this way effectively proliferated even under severe lymphopenic conditions and the antioxidant N-acetylcysteine significantly improved reconstitution of γδ T cells predominantly with a central memory phenotype. The homeostatic cytokine IL-15 induced the differentiation of effector cells in an antigen-independent fashion, which rapidly produced abundant interferon γ (IFNγ) upon antigen re-encounter. IL-15 induced effector γδ T cells displayed increased levels of the cytotoxic lymphocyte-associated proteins CD56, CD96, CD161 and perforin. In response to stimulation with isoprenoid pyrophosphates, these effector cells upregulated surface expression of CD107a and exhibited strong cytotoxicity against tumor cells in vitro. Our data clarify understanding of innate immunosurveillance mechanisms and will facilitate the controlled generation of robust Vγ9Vδ2 T cell subsets for effective cancer immunotherapy.
Abbreviations:
- BTN, butyrophilin
- CFSE, carboxyfluorescein succinimidyl ester
- DMAPP, dimethylallyl pyrophosphate
- FPP, farnesyl pyrophosphate
- GGPP, geranylgeranyl pyrophosphate
- GPP, geranyl pyrophosphate
- IPP, isopentenyl pyrophosphate
- NAC, N-acetylcysteine
- N-BP, nitrogen-containing bisphosphonate
- NK, natural killer
- TCR, T cell receptor
Introduction
γδ T cells combine rapid, innate-like immunity with conventional adaptive features to participate in stress-induced immunosurveillance of pathogens and tumors.Citation1-4 Human γδ T cells are often subdivided into Vδ1 T cells and Vδ2 T cells according to their expression of T cell receptor (TCR) variable (V) region segments. Whereas Vγ9Vδ2 T cells, which are unique to primates, predominate in human peripheral blood, γδ T cells expressing Vδ1 preponderate in epithelial tissues such as skin and intestine.
Evidence for the antitumor potential of γδ T cells has already been obtained.Citation4-7 For instance, γδ T cell reconstitution was recently reported to represent an important long-term correlate of graft-versus-leukemia activity in leukemia patients receiving T cell-depleted allogeneic bone marrow. In an 8-year follow-up, patients with increased γδ T cells following allogeneic stem cell transplantation were shown to have a clear survival advantage.Citation8 The potential of γδ T cells in the treatment of advanced hematological malignancies has recently been emphasized. The successful adoptive transfer and in vivo expansion of haploidentical γδ T cells was shown to elicit substantial clinical responses in patients refractory to all prior therapies.Citation9 A particularly attractive aspect of γδ T cell-based immunotherapy for leukemia relates to the innate-like recognition of danger-associated molecular patterns without recognition of alloantigens that might result in graft-vs-host disease.
In comparison to that of αβ T cells, the TCR diversity of γδ T cells may be more limited, implying that γδ TCRs function more like pattern recognition receptors that detect either pathogen-associated molecular patterns or self-encoded danger-associated molecular patterns reflecting cell- or tissue-dysregulation.Citation1,3 The mevalonate pathway, which provides cholesterol and lipid donor substrates for posttranslational protein prenylation, is unique in regards to Vγ9Vδ2 T cells, as it not only provides metabolites but also the cognate antigen isopentenyl pyrophosphate (IPP).Citation3 In addition to being a self antigen, IPP is also produced by bacteria, protozoa and parasites via the non-mevalonate pathway of isoprenoid biosynthesis. Vγ9Vδ2 T cells can thus detect microbes through self recognitionCitation10 and expand from approximately 4% to 60% of peripheral blood T cells during a variety of infectious diseases.Citation2 Accumulation of IPP arising from mevalonate pathway dysregulation is well known to activate Vγ9Vδ2 T cells.
A standard therapeutic approach in the management of bone disease occurring in multiple myeloma involves nitrogen-containing bisphosphonates (N-BPs), such as zoledronate that,Citation11 inhibit farnesyl pyrophosphate (FPP) synthase, a key enzyme at an important branching point of the mevalonate pathway.Citation12 N-BP-induced depletion of FPP and downstream geranylgeranyl pyrophosphate (GGPP) prevents protein farnesylation and geranylgeranylation, respectively, including multiple members of the Ras superfamilyCitation13 Thus, mevalonate pathway inhibitor therapy has strong anti-bone resorptive activities, as well as direct and indirect antitumor effects.Citation7,14,15 However, as an on-target side effect, upstream IPP accumulates and activates Vγ9Vδ2 T cellsCitation3,Citation16-18 suggesting that γδ T cells contribute to innate immunosurveillance of mevalonate metabolism. This might indeed be relevant since permanently enhanced flux through the growth-promoting mevalonate pathway can lead to malignant transformation.Citation19 Specifically, increased protein geranylgeranylation has recently been shown to dictate the malignant phenotype.Citation20
We and others have recently shown that IL-18 is a potent costimulator of N-BP-induced γδ T-cell activation in vitro.Citation21-23 In vivo, lymph node macrophage-derived IL-18 has recently been shown to contribute to the rapid activation of strategically positioned innate lymphoid cells, including γδ T cells, responding to invading pathogens and limiting systemic pathogen spread.Citation24 In addition to microbial stress, IL-18 can also arise during metabolic stress. Disturbances in metabolic pathways can be sensed by innate immune receptors and lead to the expression of inactive proIL-18 and, via concomitant caspase-1 activation, the maturation and secretion of bioactive IL-18.Citation25
Although γδ T cells perform innate-like responses, the memory subset classification system of αβ T cells, distinguished based on the expression of certain CD45 isoforms and CD27, has also been applied to define γδ T cell subsets.Citation26 The majority of adult γδ T cells already express a memory phenotype. Central memory (TCM: CD45RA−CD27+) γδ T cells maintain proliferative capacity to ensure self renewal but lack immediate effector function. Conversely, effector memory (TEM: CD45RA−CD27−) γδ T cells and terminally differentiated γδ T cells (TEMRA: CD45RA+CD27−) progressively lose proliferative capacity concomitantly with the acquisition of effector function. The homeostatic cytokine IL-15, which is upregulated during tissue dysregulation and has been implicated in constitutive as well as lymphopenia-induced proliferation of αβ CD8+ T cells, may also affect phenotypic and functional differentiation of effector T cells.Citation27,28 With regard to Vγ9Vδ2 T cells, IL-15 has been shown to induce differentiation of γδ thymocytesCitation29 as well as to overcome the incomplete activation of Vγ9Vδ2 T cells in response to mycobacteria-infected dendritic cells.Citation30 Irrespective of this classification, CD56 expression has been reported to correlate with antitumor cytotoxicity and increased resistance of γδ T cells to Fas ligand and chemically induced apoptosis.Citation31
In the present work, we challenge the current view and demonstrate that the mevalonate pathway provides multiple danger signals for IL-18 primed human γδ T cells, which then effectively proliferate even under lymphopenic conditions. Mevalonate pathway intermediates can play a dual role as antigens on the one hand, and on the other, as growth-promoting metabolic cues. In the absence of antigen, IL-15 can convert central memory γδ T cells into potent CD56+ effector cells with the ability to rapidly produce large amounts of interferon γ (IFNγ) and kill tumor cells in response to antigen restimulation.
Results
The mevalonate pathway provides multiple danger signals for IL-18 primed Vγ9Vδ2 T cells
To systematically compare the established γδ T cell antigen IPP (C5) with the downstream metabolites dimethylallyl pyrophosphate (DMAPP;C5), GPP (C10), FPP (C15), and GGPP (C20) (Fig. S1), we first examined side-by-side the upregulation of IL-2Rα (CD25) on Vγ9Vδ2 T cells. At a saturating dose (10 μM), all mevalonate-derived isoprenoid pyrophosphates induced moderate CD25 expression on the majority of Vγ9Vδ2 T cells (Fig. S2). The stress-related and inflammasome-dependent cytokine IL-18 alone induced a pre-activated phenotype and strongly enhanced antigen-induced CD25 expression on Vγ9Vδ2 T cells (Fig. S2). The observed IL-18 effects were in accordance with the constitutive expression of IL-18Rα (CD218a) on Vγ9Vδ2 T cells (Fig. S2, top panel). Likewise, Vγ9Vδ2 T cells constitutively expressed butyrophilin 3A1 (BTN3A1, CD277) (Fig. S2, top panel), which binds phosphorylated nonpeptide antigens and mediates activation of Vγ9Vδ2 T cells.Citation32-35
To compare the potencies of mevalonate-derived isoprenoid pyrophosphates as agonists of Vγ9Vδ2 T cells, we also performed dose-response experiments (0.03 μM to 30 μM) in the absence and presence of IL-18. Mevalonate metabolites downstream of IPP turned out to be as potent as IPP (). At a concentration of 0.3 μM all pyrophosphate compounds already induced significant CD25 expression on Vγ9Vδ2 T cells (P < 0.05). At this concentration DMAPP was clearly the most potent, whereas all other compounds displayed similar, albeit reduced, potencies. At 3 μM, all compounds induced CD25 expression on ≥ 60% (P < 0.01) and at 30 μM on ≥ 80% of Vγ9Vδ2 T cells (P < 0.01) (). IL-18 alone induced CD25 expression on ≥ 70% of Vγ9Vδ2 T cells (P < 0.01) and 3 μM of isoprenoid pyrophosphate was sufficient to achieve CD25 expression on ≥ 96% of Vγ9Vδ2 T cells (P < 0.01), regardless of which compound was used.
Figure 1. IL-18 enhances mevalonate-derived isoprenoid pyrophosphate-induced upregulation of CD25 expression on Vγ9Vδ2 T cells. Peripheral blood mononuclear cells (PBMCs) at 1.5 × 106/mL were stimulated for 20 h in round-bottom 96-well plate with increasing concentrations of mevalonate-derived isoprenoid pyrophosphates in the absence or presence of 100 ng/mL IL-18 . Cells were stained with fluorophore-conjugated antibodies against CD3, Vδ2 and CD25 (or isotype control). The frequency of CD25+ Vδ2 T cells was assessed via cytofluorimetric analys isusing a FACSCanto II. Data are representative of 2 independent experiments.
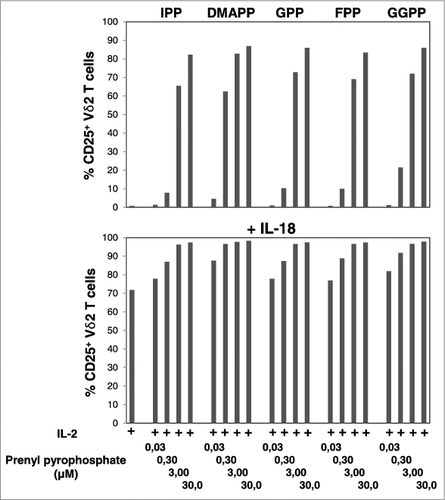
Although not essential, monocytes can serve as accessory cells during γδ T cell activation.Citation23,Citation36-38 In accordance with previous reports that innate lymphocytes can trigger dendritic cell maturation,Citation39 isoprenoid pyrophosphate-induced Vγ9Vδ2 T-cell activation also promoted the concomitant activation of monocytes (Fig. S3). Specifically, the downregulation of CD14, up to 3.5-fold decrease based on mean fluorescence index (MFI), as well as upregulation of both CD86 (up to 4.6-fold) and CD83 (up to 10-fold) was consistent with monocyte differentiation into functionally mature dendritic cells.Citation40
Next, we assessed Vγ9Vδ2 T-cell proliferation in response to all mevalonate-derived isoprenoid pyrophosphates. For this purpose, we performed carboxyfluorescein succinimidyl ester (CFSE) dye dilution assays of isolated T cells and counterstained Vδ2+ T cells. This approach was selected as it enriches γδ T cells and concomitantly eliminates the influence of accessory cells such as monocytes and dendritic cells. Data shown in demonstrate that all mevalonate-derived isoprenoid pyrophosphates induced Vγ9Vδ2 T cell proliferation with comparable magnitudes within 4 days. CFSE dye dilution patterns clearly indicated that the various isoprenoid pyrophosphates did not target individual clones but rather activated the entire population of circulating Vγ9Vδ2 T cells (). Within 14 days the various isoprenoid pyrophosphates induced >100-fold expansion of Vγ9Vδ2 T cells (Fig. S4). Isoprenoid pyrophosphate-induced proliferation of γδ T cells was further enhanced, when IL-18 was present, resulting in >200-fold expansion as compared to the cytokine control (P < 0.05).
Figure 2. Mevalonate-derived isoprenoid pyrophosphates induce proliferation of Vγ9Vδ2 T cells. T cells were isolated and labeled with 0.5 μM carboxyfluorescein diacetate succinimidyl ester (CFSE). CFSE-labeled T cells (1 × 106 cells/mL) were stimulated with 10 μM mevalonate-derived isoprenoid pyrophosphates and 100 U/mL IL-2 in round-bottom 96 wells for 5 days. After staining for Vδ2 using fluorophore-conjugated anti-TCR Vδ2 antibody, cells were analyzed via flow cytometry. Vδ2+ T cells were gated and selectively examined for CFSE dye dilution (stimulated: filled histogram; unstimulated control: open histogram). Data are representative of 3 independent experiments analyzing T cells from 3 different donors.
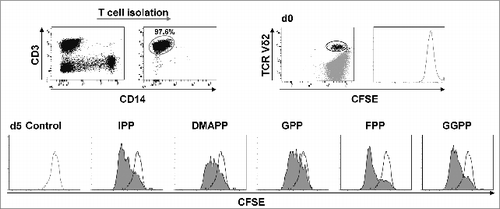
Mevalonate-derived isoprenoid pyrophosphates display antigenic features and act as cell-extrinsic metabolic cues
Previous studies have demonstrated that exogenous FPP and GGPP can be internalized and restore protein prenylation in breast cancer cells,Citation20 αβ T cells,Citation41 and natural killer (NK) cellsCitation42,43 during statin- or aminobisphosphonate-mediated inhibition of mevalonate metabolism. Thus, FPP and GGPP can also be considered cell-extrinsic metabolic cues regulating important cellular functions. This puts γδ T cells in a rather unique situation, as they may respond to these isoprenoid pyrophosphates both, as antigens and as metabolic cues. To examine this possibility in more detail, we first investigated the effects in purified αβ T cells (Fig. S5A), which are unable to recognize isoprenoid pyrophosphates with their TCR. Consistently, IPP, FPP and GGPP failed completely to induce αβ T-cell proliferation (Fig. S5B). The potent proliferative response of αβ T cells induced with anti-CD3/CD28 was strongly inhibited with simvastatin (Fig. S5C), a cholesterol-lowering drug, which inhibits mevalonate metabolism early on and thus prevents the generation of the downstream metabolites FPP and GGPP (Fig. S1).Citation7 Statins exhibit strong anti-proliferative effects on diverse cell types,Citation20,41 an activity that has been broadly attributed to the inhibition of prenylation and, in particular, to the suppression of Rho protein geranylgeranylation.Citation41 We found that addition of GGPP could actually restore αβ T-cell proliferation induced with anti-CD3/CD28 despite statin-mediated inhibition of mevalonate metabolism (Fig. S5C). This observation indicated that GGPP could act as cell-extrinsic metabolic cue that affects αβ T-cell function.
To examine the role of GGPP as an extrinsic metabolic cue in purified γδ T cells, we first induced Vγ9Vδ2 T-cell proliferation by γδ TCR triggering using an agonistic anti-Vγ9 antibody in the presence of IL-2. This antibody induced strong γδ T-cell aggregation, slight γδ TCR downregulation,Citation44 and substantial γδ T-cell expansion (). Statin treatment was then used to completely abrogate anti-Vγ9 induced proliferation. Under these conditions, add-back of GGPP at least partially restored γδ T-cell proliferation indicating that exogenous GGPP can enter the cell and exhibit effects within γδ T cells (). Interestingly, expression of CD56, which defines γδ T cells with increased antitumor activity,Citation31 was strongest in non-proliferating γδ T cells and gradually disappeared with the number of cell divisions ().
Figure 3. Cell-extrinsic geranylgeranyl pyrophosphate (GGPP) can restore Vγ9Vδ2 T cell proliferation during statin-mediated mevalonate pathway inhibition. (A) γδ T cells were isolated and stimulated with 10 μg/ml of an anti-Vγ9 antibody plus 100 U/mL IL-2 inducing cell aggregation (scale bar: 500 μm), γδ T cell receptor (TCR) downregulation and γδ T cell expansion. (B) Carboxyfluorescein diacetate succinimidyl ester (CFSE)-labeled γδ T cells were stimulated with an anti-Vγ9 antibody plus 100 U/mL IL-2 and proliferation was inhibited by 2 μg/ml simvastatin (statin). Add-back of 10 μg/ml GGPP partially restored proliferation. Cells were counterstained for CD56 and analyzed by flow cytometry. Data are representative of 3 independent experiments with 2 different donors.
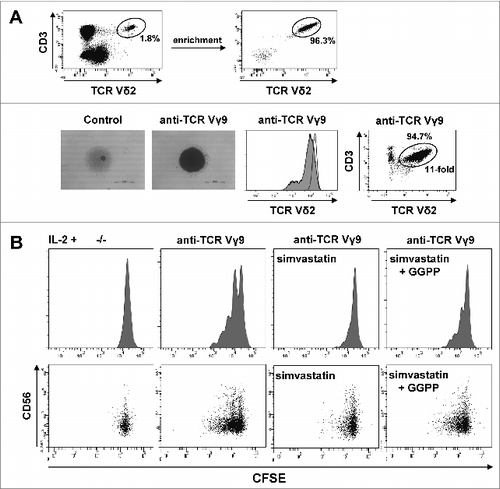
Oxidative stress that is induced during various inflammatory conditions but also during chemotherapy may have detrimental effects on T-cell function. Antioxidant effects of pyrophosphates from Plasmodium parasites, which promote cellular tolerance to hydrogen peroxide challenge, have previously been reported.Citation45 We therefore tested the sensitivity of γδ T cells to low levels of H2O2 (10 μM) and assessed oxidative stress using the CellROX Deep Red assay for the detection of cellular reactive oxygen species (ROS). All mevalonate-derived metabolites containing a pyrophosphate group exhibited a protective effect (). The pyrophosphate group appeared to be prerequisite for the antioxidant effect since isopentenyl monophosphate was ineffective. In contrast, the synthetic compound isopentenyl triphosphate could also protect γδ T cells against H2O2-mediated oxidative stress. Moreover, the stress-related cytokine IL-18, which has also been implicated in chemotherapy resistance,Citation46 had a similar antioxidant effect. The well-established antioxidant N-acetylcysteine (NAC), which increases intracellular glutathione levels,Citation47 was most potent in protecting γδ T cells against the oxidative insult ().
Figure 4. Mevalonate-derived isoprenoid pyrophosphates, IL-18 and N-acetylcysteine (NAC) increased cellular tolerance of Vγ9Vδ2 T cells to H2O2-induced oxidative stress. Peripheral blood mononuclear cells (PBMCs) at 1.5 × 106/mL were seeded in round-bottom 96 wells and treated with 10 μM of the various mevalonate-derived isoprenoid pyrophosphates, 100 ng/mL IL-18 or 100 μM N-acetylcysteine (NAC). Cells were challenged with 10 μM H2O2 for 1 h followed by the addition of 5 μM CellROX Deep Red Reagent for the detection of reactive oxygen species (ROS). After 30 min, cells were washed and cellular fluorescence was determined by flow cytometry. Data are representative of 4 independent experiments with 2 different donors.
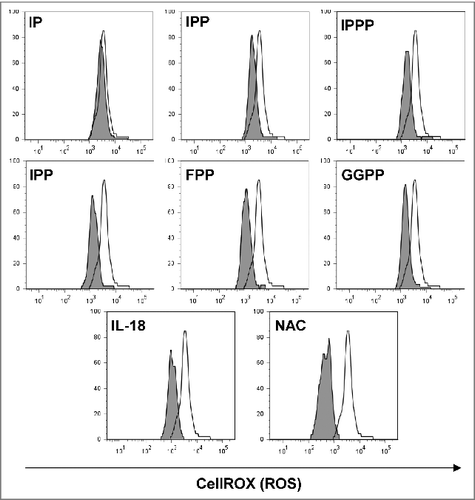
IL-18 plus NAC enhances proliferation of isoprenoid pyrophosphate-induced γδ T cells even under severe lymphopenic conditions
Immunologic reconstitution is a critical process following chemotherapy. γδ T-cell recovery has been reported to be an important long-term correlate of graft-versus-leukemia activity in leukemia patients receiving T-depleted allogeneic bone marrow.Citation8 To further challenge the γδ T-cell agonistic properties of the various mevalonate-derived isoprenoid pyrophosphates and the supportive role of IL-18, their ability to promote expansion of as few as 103 highly enriched γδ T cells was tested first. Such low γδ T-cell density and absence of accessory cells (), which simulates conditions of γδ T-cell reconstitution after immunosuppressive regimens, can cause considerable cell stressCitation48 and actually attenuated γδ T cell expansion (). However, when γδ T-cell cultures were supplemented with the antioxidant NAC, γδ T-cell expansion was clearly accelerated (). However, in the presence of IL-2 and NAC, all isoprenoid pyrophosphates including IPP still induced only moderate γδ T cell expansion (from 10-fold to 20-fold) (). In contrast, in the presence of IL-18, γδ T cells proliferated vigorously in response to all isoprenoid pyrophosphates, resulting in >1,000-fold expansion (P < 0.01 compared to cytokine control) (). Both in PBMCs and in purified γδ T cells, IL-2 plus IL-18 was sufficient to induce substantial γδ T-cell expansion (P < 0.05) relative to IL-2 alone, even in the absence of exogenous pyrophosphate antigens (Fig. S4 and ).
Figure 5. IL-18 and N-acetylcysteine enable Vγ9Vδ2 T cell proliferation under severe lymphopenic conditions. (A) Enriched γδ T cells devoid of CD14+ monocytes were obtained from peripheral blood mononuclear cells (PBMCs) using CD14 microbeads and magnetic depletion using LD columns. (B) Photo documentation of cell cultures: enriched γδ T cells were seeded at 103 cells per 96 well and stimulated with 10 μM geranylgeranyl pyrophosphate (GGPP) plus 100 U/mL IL-2 and 100 ng/mL IL-18 in the presence or absence of N-acetylcysteine (NAC). Scale bar: 500 μm. (C) Enriched γδ T cells were seeded in triplicates at 103 cells per 96 well and stimulated with 10 μM mevalonate-derived isoprenoid pyrophosphates plus 100 U/mL IL-2 and NAC in the absence or presence of 100 ng/mL IL-18 . After 14 days, cells were stained with fluorophore-conjugated antibodies CD3 and Vδ2 to confirm γδ T-cell identity. Absolute cell numbers and γδ T-cell expansion were determined by cytofluorimetric analysis (FACS). Data are representative of 4 independent experiments with T cells derived from 4 different donors.
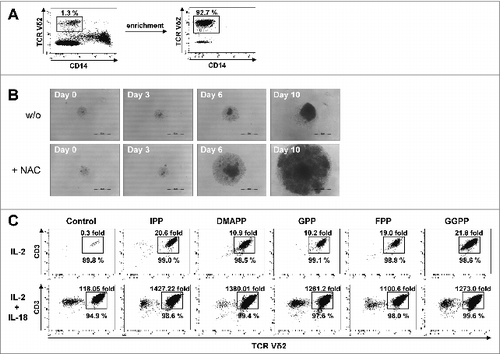
IL-15 induces the differentiation of CD56+ cytotoxic effector γδ T cells
Vγ9Vδ2 T cells expanded with mevalonate-derived isoprenoid pyrophosphates predominantly consisted of central memory cells and only of a minor subset of effector memory cells (). The various mevalonate-derived isoprenoid pyrophosphates induced very similar degrees of central memory predominance. However, when cultures were depleted of CD14+ monocytes and thus the major source of IL-18, treatment with the homeostatic cytokine IL-15 in the absence of antigen induced an upregulation of CD56 in a time-dependent fashion (). On day 4, the majority of Vγ9Vδ2 T cells were CD56+. Whereas the antigen-binding molecule BTN3A1 (CD277) remained unaffected by IL-15 treatment, IL-15Rα, the cytolytic protein perforin, the cytotoxic cell markers CD161 (KLRBI: killer cell lectin-like receptor subfamily B, member I) as well as the CD155 ligand CD96 were also upregulated during IL-15 induced differentiation (). Upon restimulation with various mevalonate-derived isoprenoid pyrophosphates, IL-15 induced CD56+ effector γδ T cells rapidly produced large amounts of IFNγ (). NK cells, which are also CD56+, served as internal control in these cultures, as they did not produce IFNγ, either spontaneously or in response to antigen stimulation.
Figure 6. IL-15 converts central memory cells into interferon γ producing effector γδ T cells with a cytotoxic phenotype. (A) Peripheral blood mononuclear cells (PBMCs) at 1.5 × 106/mL were stimulated in round-bottom 96-well plate with 10 μM of the various mevalonate-derived isoprenoid pyrophosphates in the presence of 100 U/mL IL-2 and 100 ng/mL IL-18. After 9 days, cells were stained with fluorophore-conjugated antibodies against CD3, Vδ2, CD45RA and CD27 to determine memory phenotypes. (B) PBMCs depleted of CD14+ cells were cultured with 25 ng/mL IL-15 for the time indicated. Cells were stained with fluorophore-conjugated antibodies against CD3, Vδ2 and CD56 and analyzed by fluorescence cytometry. Vδ2+ T cells were gated on a flow cytometer and selectively analyzed for CD56 expression (filled histogram) vs. isotype control (open histogram). (C) CD14−PBMCs were either freshly analyzed (open histogram) or cultured with 25 ng/mL IL-15 for 4 days prior (filled histogram). Vδ2+ T cells were fixed, permeabilized (as needed) and stained with fluorophore-conjugated antibodies to determine the expression of CD277, IL-15Rα, perforin (intracellular), and CD161 and CD96 (filled histogram) vs. isotype control (dotted line). (D) CD14−PBMCs were cultured with 25 ng/mL IL-15 for 3 days and then stimulated with isopentenyl pyrophosphate (IPP), farnesyl-PP (FPP) or geranylgeranyl-PP (GGPP) for 5 hours. CD56+ cells, which included γδ T cells and natural killer (NK) cells, were gated and analyzed for intracellular interferon γ (IFNγ) and for surface Vδ2. Data are representative of five independent experiments with 3 different donors.
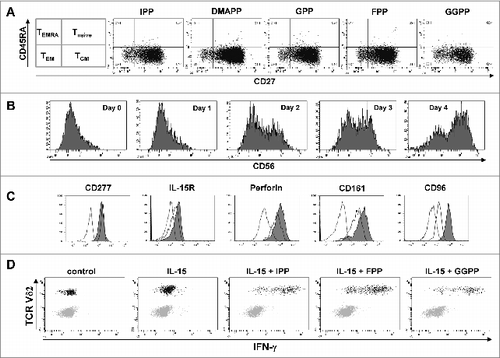
In addition to effector cytokine production, a rapid translocation of the lysosomal associated membrane protein CD107a, which is a component of cytotoxic granules, occurred in IL-15 differentiated γδ T cells upon stimulation with isoprenoid pyrophosphate antigens ().
Figure 7. Pyrophosphate antigen stimulated γδ T cells differentiated with IL-15 upregulate surface CD107a and display cytotoxic and anti-proliferative activity against MOLT-4 leukemic cells. (A) Purified γδ T cells were differentiated with 25 ng/mL IL-15 for 4 days, stimulated with isopentenyl pyrophosphate (IPP), farnesyl-PP (FPP) or geranylgeranyl-PP (GGPP) (each at 30 μM) for 4 hours and immunofluorescently stained for surface expression of CD107a in the absence of monensin (filled histogram) vs. isotype control (open histogram). (B) MOLT-4 cells were labeled with 0.5 μM carboxyfluorescein diacetate succinimidyl ester (CFSE) and mixed with IL-15 differentiated γδ T cells (IL-15 γδ T cells) at a ratio of 1:5. The cell mixture was stained with fluorophore-conjugated antibodies against CD3 and TCRVδ2 and analyzed by flow cytometry. In a 96-well round-bottom plate, CFSE-labeled MOLT-4 cells (3 × 104) were co-cultured with 1.5 × 105 IL-15 differentiated γδ T cells (ratio 1:5) that had been pre-activated with pyrophosphate antigens (30 μM) for 4 h. After overnight incubation (in the absence of pyrophosphate antigens), cells were stained for CD3 and TCRVδ2 (as described above) and analyzed by flow cytometry (bottom panel). Data are representative of 3 independent experiments with 2 different donors.
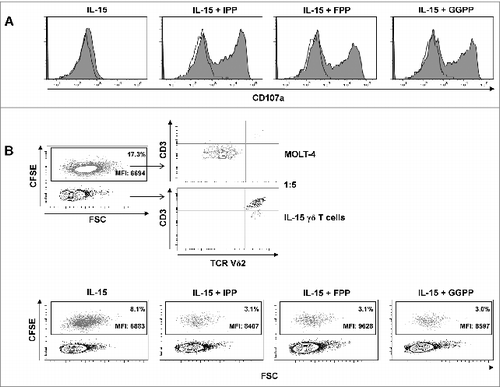
In line with surface CD107a exposure, IL-15 differentiated γδ T cells displayed strong cytotoxicity in overnight killing assays against CFSE-labeled MOLT-4 leukemic cells, which was further enhanced by pre-activation with isoprenoid pyrophosphate antigens (). Moreover, the higher CFSE fluorescence levels of surviving MOLT-4 target cells in the presence of antigen stimulation indicate that activated γδ T cells also inhibited leukemic cell proliferation ().
Discussion
In this study we first demonstrate that the potentially oncogenic mevalonate pathway provides multiple powerful agonists for human Vγ9Vδ2 T cells pre-activated with the stress-related, inflammasome-dependent cytokine IL-18. This observation was unexpected because so far only IPP has been recognized as an immunologically relevant γδ T-cell antigen, and other mevalonate metabolites have virtually been ignored. This oversight has been due to several reasons. First, early workCitation49 resulted in a ranking of prenyl pyrophosphate antigens, which suggested that the downstream metabolites are 30- to 300-fold less potent than IPP in stimulating γδ T cells.Citation50 This ranking relates to the ability of the various isoprenoid pyrophosphates to induce short-term proliferation of the γδ T cell clone 12G12. This γδ T cell clone has been derived from a tuberculoid leprosy patient using M. tuberculosis as a crude source of phosphorylated antigens.Citation51 Most bacteria including M. tuberculosis, however, synthesize isoprenoid pyrophosphates via the non-mevalonate pathway. (E)-4-hydroxy-3-methyl-but-2-enyl pyrophosphate (HMBPP), a metabolite of the non-mevalonate pathway, has been reported to be 10,000-fold more potent than IPP.Citation2 The use of M. tuberculosis as a source of antigens is therefore likely to select for γδ T cells with high avidity for HMBPP during clonal outgrowth.Citation52 It is thus conceivable that such HMBPP-induced γδ T cell clones display the reported preference for IPP and, concomitantly, declining reactivity for the downstream isoprenoid pyrophosphates such as FPP and GGPP, particularly because the absolute stereochemistry of double bonds may differ between mycobacterial and human FPP/GGPP. Second, IPP was already known as a mycobacterial antigen.Citation53 Third, treatment with nitrogen-containing bisphosphonates (N-BPs), such as zoledronate, activates γδ T cells by inducing accumulation of IPP despite the depletion of downstream metabolites. Together, these aspects may have enforced the general, though biased perspective that the downstream pyrophosphate compounds are less relevant, or even irrelevant, as γδ T cell agonists.
However, we found that γδ T cells responded with comparable magnitudes to the various isoprenoid pyrophosphates and did not select for individual γδ T cell clones or subsets but rather activated the bulk population of circulating Vγ9Vδ2 T cells also confirming that Vγ9Vδ2 TCRs reacted more like pattern recognition receptors.Citation1,3
We also show for the first time that the downstream metabolites, which include the protein prenylation donor substrates FPP and GGPP, can play a dual role and combine properties of antigens with features of cell-extrinsic metabolic cues in a unique fashion. Amongst purified γδ T cells, GGPP treatment could restore anti-Vγ9 antibody induced proliferation during treatment with statins, which are known to exhibit anti-proliferative effects by suppressing protein prenylation.Citation54
In addition to or instead of triggering the Vγ9Vδ2 TCR, isoprenoid pyrophosphates may obviously enter γδ T cells and exert intracellular effects. Along this line, Sandstrom et al. reported that binding of phosphorylated antigen to the intracellular B30.2 domain of butyrophilin subfamily 3, member A1 (BTN3A1) is required for Vγ9Vδ2 T cell activation.Citation35 Our observation that Vγ9Vδ2 T cells constitutively express BTN3A1 provides the explanation at the molecular level for our current finding that isoprenoid pyrophosphate-mediated Vγ9Vδ2 T cell activation can occur in the absence of any other cell type.
The similarity of the metabolic changes that occur in tumor cells and in activated T cells is increasingly appreciated.Citation55 As opposed to quiescent cells that prioritize efficient energy usage and ATP production, tumor cells and activated T cells must ensure high metabolic flux through growth-promoting pathways such as the mevalonate pathway for protein prenylation. With regard to γδ T cells this aspect is intriguing, since intermediate metabolites of the mevalonate pathway such as FPP and GGPP could then play a dual role as antigens on the one hand, and on the other, as growth-promoting metabolic cues with intrinsic antioxidant activity.
Inflammasomes, which are multiprotein complexes that operate as platforms for the activation of caspase-1,Citation56 have critical roles in tumor immune surveillance.Citation57 Active caspase-1 catalyzes the proteolytic maturation of pro-IL-1β or pro-IL-18 resulting in the release of mature IL-1β or IL-18. There is growing evidence that inflammasome-generated IL-18 is crucial for the activation of innate lymphocytes including γδ T cells in vitroCitation21-23,Citation42,43,58,59 and in vivo.Citation24 In the present work, IL-18 by itself induced a pre-activated phenotype with increased CD25 expression and potently costimulated γδ T-cell expansion in response to the various mevalonate-derived isoprenoid pyrophosphates. IL-18, which has previously been implicated in chemoresistance,Citation46 also increased tolerance of γδ T cells against oxidative stress. In this manner, isoprenoid pyrophosphates, which themselves acted as antioxidants, IL-18, and the antioxidant NAC collaborated to promote γδ T-cell expansion, even under severe lymphopenic conditions including lack of any accessory cells. The clinical term “lymphopenia” refers to a count of less than 103 lymphocytes per μL (106/mL) of blood in adults. In vitro, T cells are usually maintained between 0.5 – 2 × 106/mL. T-cell activation at low cell density (104/mL) has been shown to cause considerable oxidative stress and leads to T-cell apoptosis.Citation48,60 In our work we have challenged purified γδ T cells at a cell density of 5 × 103/mL. Under such conditions, which resemble γδ T-cell reconstitution after allogeneic stem cell transplantation, isoprenoid pyrophosphates, IL-18 and NAC enabled γδ T-cell reconstitution and resulted in more than 1,000-fold expansion. These findings may have clinical implications since γδ T-cell reconstitution after allogeneic stem cell transplantation is considered an important long-term correlate of graft-versus-leukemia activity.Citation8
Effective T-cell immunity against pathogens or cancer requires an immediate effector response as well as memory development to resist pathogen or tumor recurrence. Therefore, a balanced distribution in γδ T cell phenotype between central and effector memory versus terminally differentiated effector γδ T cells may ensure both immediate effector functions, (e.g.,cytokine production and cytotoxicity), coincident with maintained proliferative potential to improve long-term protection.Citation26 Preliminary evidence has suggested that effector memory γδ T cells are indeed required to achieve objective clinical responses.Citation61 This is a concern as the central memory phenotype may prevail in expanded γδ T cells and becomes a serious issue in male cancer patients because men beyond 30 years of age generally have a strong bias toward the central memory phenotype.Citation62 Therefore, our current observation that IL-15 converts central memory cells into effector γδ T cells with a cytotoxic phenotype including the ability to produce large amounts of IFNγ with innate-like kinetics is clinically relevant. The cytotoxic phenotype of IL-15 induced effector γδ T cells included CD56 expression, suggesting that CD56 may be a marker of true effector γδ T cells,Citation31 but also encompassed expression of CD161 and of CD96. Whereas CD161, previously referred to as killer cell lectin-like receptor subfamily B member I (KLRBI), has been considered a marker of CD4+ effector T cells,Citation63,64 CD96 is the ligand of the poliovirus receptor CD155 and functions as a killer cell regulatory molecule.Citation65
CD107a, which is a component of cytotoxic granules, transiently accumulates on the surface of cytotoxic lymphocytes upon degranulation. For the purpose of flow cytometric detection, surface exposure of CD107a can be prolonged by treatment with monensin, which inhibits clathrin-dependent endocytosis.Citation66 In our work, we surprisingly detected strong surface expression of CD107a on antigen-stimulated effector γδ T cells in the absence of monensin. This finding indicates that antigen-induced activation of IL-15 differentiated γδ T cells, which triggers effector responses, is accompanied by upregulation and prolonged surface expression of CD107a. This would be consistent with a recently proposed novel role of CD107a, since engineered surface expression of CD107a has recently been shown to protect NK cells from degranulation-associated damage.Citation67
Our current work emphasizes the potential of the mevalonate pathway as an important therapeutic target as it provides multiple potent danger signals for human Vγ9Vδ2 T cells (). These danger signals combine features of antigens and metabolic cues. The stress-related, inflammasome-dependent cytokine IL-18 induces a pre-activated phenotype, increases responses to antigens, tolerance to oxidative stress, and further, enables γδ T-cell proliferation even under severe lymphopenic conditions. The homeostatic cytokine IL-15 converts central memory Vγ9Vδ2 T cells into cytotoxic, IFNγ producing CD56+ effector cells. Our findings will pave the way for the controlled generation of robust γδ T cell subsets for the effective immunotherapy of cancer.
Figure 8. IL-18 and IL-15 regulate γδ T cell surveillance of mevalonate metabolism. IL-18 induces a pre-activated phenotype in γδ T cells, increases immunological responses to antigens as well as tolerance to oxidative stress, and enables proliferation under severe lymphopenic conditions; IL-15 converts central memory Vγ9Vδ2 T cells into effector cells, which produce with innate-like kinetics large amounts of interferon γ (IFNγ) upon antigen stimulation and display potent cytotoxicity against tumor cells.
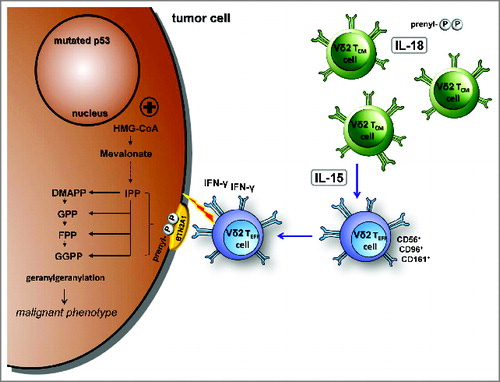
Materials and Methods
Reagents, antibodies and cell lines
Isoprenoids from Echelon Biosciences or from Isoprenoids LC were analyzed by nuclear magnetic resonance (NMR) imaging and were at least 95% pure. Simvastatin and N-acetylcysteine (NAC) were obtained from Sigma-Aldrich. Human recombinant IL-2 (rIL-2) was from Novartis Pharmaceuticals. Human rIL-15 was from PeproTech. Human rIL-18 was from Medical & Biological Laboratories. The fixable-cell-permeant, fluorescein-based tracer CFSE (carboxyfluorescein diacetate succinimidyl ester) was obtained from Life Technologies. H2O2 was from Sigma-Aldrich. Brefeldin A was from BD Biosciences.
Fluorophore conjugated monoclonal antibodies used were as follows: anti-CD3 (SK7-PerCP-Cy5.5), anti-CD3 (UCHT-1-V450), anti-CD14 (MϕP9-FITC, MϕP9-APC), anti-CD25 (2A3-PE), anti-CD27 (M-T271-APC), anti-CD45RA (HI101-PE-Cy7), anti-CD56 (NKH-1-PE), anti-CD83 (HB15-PE), anti-CD86 (2331/FUN-1-PE), anti-CD107a (H4a3-APC), and anti-IFNγ (B27-FITC) were all from BD Biosciences; anti-TCR Vδ2 (IMMU 389-FITC) and the unconjugated, preservative-free anti-Vγ9 (IMMU 360) were from Beckman Coulter; anti-CD96 (NK92.39-PE), anti-CD161 (HP-3610-PerCP-Cy5.5), and anti-CD218a (H44-PE) were from eBioscience; anti-TCR Vδ2 (B6-PerCP), anti-CD277 (BT3.1-PE), and anti-human perforin (B-D48-PE) were from BioLegend; anti-C-C chemokine receptor 2 (CCR2) (48607-APC) was from R&D.
Cell isolation, cell staining and flow cytometry
All donors (n = 10) gave written informed consent in accordance with the Declaration of Helsinki to the use of their residual peripheral blood buffy coats for research purposes, with approval from the University Hospital of Innsbruck Review Board. CD3+ T cells were isolated using CD3 microbeads and LS columns. Untouched γδ T cells were isolated from peripheral blood mononuclear cells (PBMCs) using the TCRγδ T Cell Isolation Kit. Monocytes were depleted from PBMCs using CD14 microbeads and LD columns. All procedures were performed according to the manufacturer's instructions (Miltenyi Biotec). Fix&Perm (AN DER GRUB Bio Research) was used for intracellular perforin detection. Cell suspensions were stained on ice for 30 min in the dark with various combinations of directly fluorochrome-conjugated antibodies in PBS containing 0.5% fetal calf serum (FCS) and 50 μg/mL human IgG (Octapharma) to block Fc γ receptors. Fixable viability dye eFluor 780 was used to label dead cells. For all samples, acquisition and analysis was performed on a FACSCanto II flow cytometer and FACS Diva 6.1.2 as well as FlowJo V7.2.5 software (BD Biosciences) by first applying dead cell and doublet discrimination followed by the indicated cell gating.
Cell culture: γδ T cell proliferation, differentiation and cytokine measurements
All cell cultures were performed in round-bottom 96 wells using complete RPMI 1640 supplemented with 10% FBS (HyClone).Citation23,43,68 PBMCs or enriched γδ T cells were stimulated in triplicates with isoprenoid pyrophosphates (10–50 μM) in the presence or absence of 100 U/mL IL-2, 100 ng/mL IL-18 or 25 ng/mL IL-15 . To assess γδ T cell proliferation, cells were labeled with 0.5 μM CFSE, stimulated and cultured for 5 days. Progressive dye dilution as a consequence of cell divisions was measured amongst Vδ2+ T cells by counterstaining (as described above) and cytofluorimetric analysis.
For intracellular staining of IFNγ, 2 × 106/mL cells were stimulated with 30 μM isoprenoid pyrophosphates for 5 h in the presence of brefeldin A and were then harvested, washed, and stained with fluorochrome-conjugated anti-CD3 and anti-TCRVδ2 for 30 min at 4°C. Cells were washed twice and treated with Fix&Perm. Fixed, permeabilized cells were stained with fluorochrome-conjugated anti-IFNγ antibody. After 2 more washes, the cells were analyzed by fluorescence cytometry.
Oxidative stress analysis
To monitor changes in cellular tolerance to oxidative stress, 1.5 × 106/mL PBMCs () were pretreated with the various isoprenoid pyrophosphates, IL-18 or NAC. The cells were exposed to 10 μM H2O2 in culture medium. After 1 h, 5 μM CellROX Deep Red reagent (Life Technologies) was added for 30 min. Cells were then washed 3 times and cellular fluorescence was measured by flow cytometry.
In vitro tumor-killing assays
MOLT-4 cells (DSMZ: ACC 362) were used as target cells. MOLT-4 cells are leukemic T cells that lack CD3 surface expression. MOLT-4 cells were labeled with 0.5 μM CFSE and 3 × 104 cancer cells were incubated with 1.5 × 105 γδ T cells (ratio 1:5) in a 96-well round-bottom plate. After overnight incubation (20 h), cells were stained with anti-CD3-V450 and anti-TCRVδ2-PerCP and analyzed by fluorescence cytometry. Whereas loss of CFSE-labeled target cells is indicative of γδ T cell cytotoxicity, CFSE fluorescence intensity of surviving target cells can additionally indicate anti-proliferative activity of γδ T cells.
Statistical analyses
Experiments were always set up at least as duplicates or as triplicates, and repeated at least 2 times. Group comparisons were performed using Student's t test for paired samples. A P value equal to or less than 0.05 was considered statistically significant. Microsoft Excel and SPSS software (SPSS Inc.) were used for calculations.
Disclosure of Potential Conflicts of Interest
No potential conflicts of interest were disclosed.
953410_Supplementary_Materials.zip
Download Zip (866.4 KB)Acknowledgments
We appreciate the participation of the TILAK hospital holding company, which serves as a partner in the Oncotyrol research program. We thank also Walter Nussbaumer for providing us with buffy coats, Juliana Komuczki and Andrea Rahm for technical assistance, and Wolfgang Horninger for continuous support.
Funding
This work was supported by Oncotyrol, a center for personalized cancer medicine. The Competence Center Oncotyrol is funded within the scope of the Competence Centers for Excellent Technologies (COMET) through BMVIT, BMWFJ, through the province of Salzburg and the Tiroler Zukunftsstiftung/Standortagentur Tirol. The COMET Program is conducted by the Austrian Research Promotion Agency (FFG). This work was also supported by the Austrian Cancer Society/Tirol.
References
- Bonneville M, O'Brien RL, Born WK. Gammadelta T cell effector functions: a blend of innate programming and acquired plasticity. Nat Rev Immunol 2010; 10:467-78; PMID:20539306
- Chien YH, Meyer C, Bonneville M. gammadelta T Cells: First Line of Defense and Beyond. Annu Rev Immunol 2014; 32:121-55; PMID:24387714; http://dx.doi.org/10.1146/annurev-immunol-032713-120216
- Vantourout P, Hayday A. Six-of-the-best: unique contributions of gammadelta T cells to immunology. Nat Rev Immunol 2013; 13:88-100; PMID:23348415
- Hannani D, Ma Y, Yamazaki T, Dechanet-Merville J, Kroemer G, Zitvogel L. Harnessing gammadelta T cells in anticancer immunotherapy. Trends Immunol 2012; 33:199-206; PMID:22364810; http://dx.doi.org/10.1016/j.it.2012.01.006
- Gomes AQ, Martins DS, Silva-Santos B. Targeting gammadelta T lymphocytes for cancer immunotherapy: from novel mechanistic insight to clinical application. Cancer Res 2010; 70:10024-7; PMID:21159627; http://dx.doi.org/10.1158/0008-5472.CAN-10-3236
- Kabelitz D, Wesch D, He W. Perspectives of gammadelta T cells in tumor immunology. Cancer Res 2007; 67:5-8; PMID:17210676; http://dx.doi.org/10.1158/0008-5472.CAN-06-3069
- Thurnher M, Nussbaumer O, Gruenbacher G. Novel aspects of mevalonate pathway inhibitors as antitumor agents. Clin Cancer Res 2012; 18:3524-31; PMID:22529099; http://dx.doi.org/10.1158/1078-0432.CCR-12-0489
- Godder KT, Henslee-Downey PJ, Mehta J, Park BS, Chiang KY, Abhyankar S, Lamb LS. Long term disease-free survival in acute leukemia patients recovering with increased gammadelta T cells after partially mismatched related donor bone marrow transplantation. Bone Marrow Transp 2007; 39:751-7; PMID:17450185; http://dx.doi.org/10.1038/sj.bmt.1705650
- Wilhelm M, Smetak M, Schaefer-Eckart K, Kimmel B, Birkmann J, Einsele H, Kunzmann V. Successful adoptive transfer and in vivo expansion of haploidentical gammadelta T cells. J Transl Med 2014; 12:45; PMID:24528541; http://dx.doi.org/10.1186/1479-5876-12-45
- Kronenberg M, Kinjo Y. Infection, autoimmunity, and glycolipids: T cells detect microbes through self-recognition. Immunity 2005; 22:657-9; PMID:15963780; http://dx.doi.org/10.1016/j.immuni.2005.06.001
- Larocca A, Child JA, Cook G, Jackson GH, Russell N, Szubert A, Gregory WM, Brioli A, Owen RG, Drayson MT, et al. The impact of response on bone-directed therapy in patients with multiple myeloma. Blood 2013; 122:2974-7; PMID:23974194; http://dx.doi.org/10.1182/blood-2013-04-498139
- Goldstein JL, Brown MS. Regulation of the mevalonate pathway. Nature 1990; 343:425-30; PMID:1967820; http://dx.doi.org/10.1038/343425a0
- Konstantinopoulos PA, Karamouzis MV, Papavassiliou AG. Post-translational modifications and regulation of the RAS superfamily of GTPases as anticancer targets. Nat Rev Drug Discov 2007; 6:541-55; PMID:17585331; http://dx.doi.org/10.1038/nrd2221
- Thurnher M, Gruenbacher G, Nussbaumer O. Regulation of mevalonate metabolism in cancer and immune cells. Biochim Biophys Acta 2013; 1831:1009-15; PMID:23524243; http://dx.doi.org/10.1016/j.bbalip.2013.03.003
- Riganti C, Massaia M. Inhibition of the mevalonate pathway to override chemoresistance and promote the immunogenic demise of cancer cells: Killing two birds with one stone. Oncoimmunology 2013; 2:e25770; PMID:24327936; http://dx.doi.org/10.4161/onci.25770
- Hayday AC. Gammadelta T cells and the lymphoid stress-surveillance response. Immunity 2009; 31:184-96; PMID:19699170; http://dx.doi.org/10.1016/j.immuni.2009.08.006
- Gober HJ, Kistowska M, Angman L, Jeno P, Mori L, De Libero G. Human T cell receptor gammadelta cells recognize endogenous mevalonate metabolites in tumor cells. J Exp Med 2003; 197:163-8; PMID:12538656; http://dx.doi.org/10.1084/jem.20021500
- Kunzmann V, Bauer E, Wilhelm M. Gamma/delta T-cell stimulation by pamidronate. N Engl J Med 1999; 340:737-8; PMID:10068336; http://dx.doi.org/10.1056/NEJM199903043400914
- Clendening JW, Pandyra A, Boutros PC, El Ghamrasni S, Khosravi F, Trentin GA, Martirosyan A, Hakem A, Hakem R, Jurisica I, et al. Dysregulation of the mevalonate pathway promotes transformation. Proc Natl Acad Sci U S A 2010; 107:15051-6; PMID:20696928; http://dx.doi.org/10.1073/pnas.0910258107
- Freed-Pastor WA, Mizuno H, Zhao X, Langerod A, Moon SH, Rodriguez-Barrueco R, Barsotti A, Chicas A, Li W, Polotskaia A, et al. Mutant p53 Disrupts Mammary Tissue Architecture via the Mevalonate Pathway. Cell 2012; 148:244-58; PMID:22265415; http://dx.doi.org/10.1016/j.cell.2011.12.017
- Li W, Kubo S, Okuda A, Yamamoto H, Ueda H, Tanaka T, Nakamura H, Yamanishi H, Terada N, Okamura H. Effect of IL-18 on expansion of gammadelta T cells stimulated by zoledronate and IL-2. J Immunother 2010; 33:287-96; PMID:20445349; http://dx.doi.org/10.1097/CJI.0b013e3181c80ffa
- Tsuda J, Li W, Yamanishi H, Yamamoto H, Okuda A, Kubo S, Ma Z, Terada N, Tanaka Y, Okamura H. Involvement of CD56brightCD11c+ cells in IL-18-mediated expansion of human gammadelta T cells. J Immunol 2011; 186:2003-12; PMID:21239711; http://dx.doi.org/10.4049/jimmunol.1001919
- Nussbaumer O, Gruenbacher G, Gander H, Komuczki J, Rahm A, Thurnher M. Essential requirements of zoledronate-induced cytokine and gammadelta T cell proliferative responses. J Immunol 2013; 191:1346-55; PMID:23794630; http://dx.doi.org/10.4049/jimmunol.1300603
- Kastenmuller W, Torabi-Parizi P, Subramanian N, Lammermann T, Germain RN. A spatially-organized multicellular innate immune response in lymph nodes limits systemic pathogen spread. Cell 2012; 150:1235-48; PMID:22980983; http://dx.doi.org/10.1016/j.cell.2012.07.021
- Jin C, Henao-Mejia J, Flavell RA. Innate immune receptors: key regulators of metabolic disease progression. Cell Metab 2013; 17:873-82; PMID:23747246; http://dx.doi.org/10.1016/j.cmet.2013.05.011
- Dieli F, Poccia F, Lipp M, Sireci G, Caccamo N, Di Sano C, Salerno A. Differentiation of effector/memory Vdelta2 T cells and migratory routes in lymph nodes or inflammatory sites. J Exp Med 2003; 198:391-7; PMID:12900516; http://dx.doi.org/10.1084/jem.20030235
- Geginat J, Lanzavecchia A, Sallusto F. Proliferation and differentiation potential of human CD8+ memory T-cell subsets in response to antigen or homeostatic cytokines. Blood 2003; 101:4260-6; PMID:12576317; http://dx.doi.org/10.1182/blood-2002-11-3577
- Boyman O, Krieg C, Homann D, Sprent J. Homeostatic maintenance of T cells and natural killer cells. Cell Mol Life Sci 2012; 69:1597-608; PMID:22460580; http://dx.doi.org/10.1007/s00018-012-0968-7
- Ribot JC, Ribeiro ST, Correia DV, Sousa AE, Silva-Santos B. Human gammadelta Thymocytes Are Functionally Immature and Differentiate into Cytotoxic Type 1 Effector T Cells upon IL-2/IL-15 Signaling. J Immunol 2014; 192:2237-43; PMID:24489097; http://dx.doi.org/10.4049/jimmunol.1303119
- Meraviglia S, Caccamo N, Salerno A, Sireci G, Dieli F. Partial and ineffective activation of V gamma 9V delta 2 T cells by Mycobacterium tuberculosis-infected dendritic cells. J Immunol 2010; 185:1770-6; PMID:20592281; http://dx.doi.org/10.4049/jimmunol.1000966
- Alexander AA, Maniar A, Cummings JS, Hebbeler AM, Schulze DH, Gastman BR, Pauza CD, Strome SE, Chapoval AI. Isopentenyl pyrophosphate-activated CD56+ {gamma}{delta} T lymphocytes display potent antitumor activity toward human squamous cell carcinoma. Clin Cancer Res 2008; 14:4232-40; PMID:18594005; http://dx.doi.org/10.1158/1078-0432.CCR-07-4912
- Harly C, Guillaume Y, Nedellec S, Peigne CM, Monkkonen H, Monkkonen J, Li J, Kuball J, Adams EJ, Netzer S, et al. Key implication of CD277/butyrophilin-3 (BTN3A) in cellular stress sensing by a major human gammadelta T-cell subset. Blood 2012; 120:2269-79; PMID:22767497; http://dx.doi.org/10.1182/blood-2012-05-430470
- Vavassori S, Kumar A, Wan GS, Ramanjaneyulu GS, Cavallari M, El Daker S, Beddoe T, Theodossis A, Williams NK, Gostick E, et al. Butyrophilin 3A1 binds phosphorylated antigens and stimulates human gammadelta T cells. Nat Immunol 2013; 14:908-16; PMID:23872678
- Wang H, Henry O, Distefano MD, Wang YC, Raikkonen J, Monkkonen J, Tanaka Y, Morita CT. Butyrophilin 3A1 Plays an Essential Role in Prenyl Pyrophosphate Stimulation of Human Vgamma2Vdelta2 T Cells. J Immunol 2013; 191:1029-42; PMID:23833237; http://dx.doi.org/10.4049/jimmunol.1300658
- Sandstrom A, Peigne CM, Leger A, Crooks JE, Konczak F, Gesnel MC, Breathnach R, Bonneville M, Scotet E, Adams EJ. The Intracellular B30.2 Domain of Butyrophilin 3A1 Binds Phosphoantigens to Mediate Activation of Human Vgamma9Vdelta2 T Cells. Immunity 2014; 40(4):490-500; PMID:24703779
- Fiore F, Castella B, Nuschak B, Bertieri R, Mariani S, Bruno B, Pantaleoni F, Foglietta M, Boccadoro M, Massaia M. Enhanced ability of dendritic cells to stimulate innate and adaptive immunity on short-term incubation with zoledronic acid. Blood 2007; 110:921-7; PMID:17403919; http://dx.doi.org/10.1182/blood-2006-09-044321
- Roelofs AJ, Jauhiainen M, Monkkonen H, Rogers MJ, Monkkonen J, Thompson K. Peripheral blood monocytes are responsible for gammadelta T cell activation induced by zoledronic acid through accumulation of IPP/DMAPP. Br J Haematol 2009; 144:245-50; PMID:19016713; http://dx.doi.org/10.1111/j.1365-2141.2008.07435.x
- Castella B, Riganti C, Fiore F, Pantaleoni F, Canepari ME, Peola S, Foglietta M, Palumbo A, Bosia A, Coscia M, et al. Immune modulation by zoledronic acid in human myeloma: an advantageous cross-talk between Vgamma9Vdelta2 T cells, alphabeta CD8+ T cells, regulatory T cells, and dendritic cells. J Immunol 2011; 187:1578-90; PMID:21753152; http://dx.doi.org/10.4049/jimmunol.1002514
- Munz C, Steinman RM, Fujii S. Dendritic cell maturation by innate lymphocytes: coordinated stimulation of innate and adaptive immunity. J Exp Med 2005; 202:203-7; PMID:16027234; http://dx.doi.org/10.1084/jem.20050810
- Zhou LJ, Tedder TF. CD14+ blood monocytes can differentiate into functionally mature CD83+ dendritic cells. Proc Natl Acad Sci U S A 1996; 93:2588-92; PMID:8637918; http://dx.doi.org/10.1073/pnas.93.6.2588
- Dunn SE, Youssef S, Goldstein MJ, Prod'homme T, Weber MS, Zamvil SS, Steinman L. Isoprenoids determine Th1/Th2 fate in pathogenic T cells, providing a mechanism of modulation of autoimmunity by atorvastatin. J Exp Med 2006; 203:401-12; PMID:16476765; http://dx.doi.org/10.1084/jem.20051129
- Gruenbacher G, Gander H, Nussbaumer O, Nussbaumer W, Rahm A, Thurnher M. IL-2 costimulation enables statin-mediated activation of human NK cells, preferentially through a mechanism involving CD56+ dendritic cells. Cancer Res 2010; 70:9611-20; PMID:20947520; http://dx.doi.org/10.1158/0008-5472.CAN-10-1968
- Nussbaumer O, Gruenbacher G, Gander H, Thurnher M. DC-like cell-dependent activation of human natural killer cells by the bisphosphonate zoledronic acid is regulated by gammadelta T lymphocytes. Blood 2011; 118:2743-51; PMID:21673342; http://dx.doi.org/10.1182/blood-2011-01-328526
- Correia DV, d'Orey F, Cardoso BA, Lanca T, Grosso AR, de Barros A, Martins LR, Barata JT, Silva-Santos B. Highly active microbial phosphoantigen induces rapid yet sustained MEK/Erk- and PI-3K/Akt-mediated signal transduction in anti-tumor human gammadelta T-cells. PLoS One 2009; 4:e5657; PMID:19479075
- Lindberg BG, Merritt EA, Rayl M, Liu C, Parmryd I, Olofsson B, Faye I. Immunogenic and antioxidant effects of a pathogen-associated prenyl pyrophosphate in Anopheles gambiae. PLoS One 2013; 8:e73868; PMID:23967351; http://dx.doi.org/10.1371/journal.pone.0073868
- Yao L, Zhang Y, Chen K, Hu X, Xu LX. Discovery of IL-18 as a novel secreted protein contributing to doxorubicin resistance by comparative secretome analysis of MCF-7 and MCF-7/Dox. PLoS One 2011; 6:e24684; PMID:21931812; http://dx.doi.org/10.1371/journal.pone.0024684
- Mitchell JR, Jollow DJ, Potter WZ, Gillette JR, Brodie BB. Acetaminophen-induced hepatic necrosis. IV. Protective role of glutathione. J Pharmacol Exp Ther 1973; 187:211-7; PMID:4746329
- Ma Q, Wang Y, Lo AS, Gomes EM, Junghans RP. Cell density plays a critical role in ex vivo expansion of T cells for adoptive immunotherapy. J Biomed Biotechnol 2010; 2010:386545; PMID:20625484; http://dx.doi.org/10.1155/2010/386545
- Morita CT, Lee HK, Wang H, Li H, Mariuzza RA, Tanaka Y. Structural features of nonpeptide prenyl pyrophosphates that determine their antigenicity for human gamma delta T cells. J Immunol 2001; 167:36-41; PMID:11418629; http://dx.doi.org/10.4049/jimmunol.167.1.36
- Morita CT, Jin C, Sarikonda G, Wang H. Nonpeptide antigens, presentation mechanisms, and immunological memory of human Vgamma2Vdelta2 T cells: discriminating friend from foe through the recognition of prenyl pyrophosphate antigens. Immunol Rev 2007; 215:59-76; PMID:17291279; http://dx.doi.org/10.1111/j.1600-065X.2006.00479.x
- Tanaka Y, Sano S, Nieves E, De Libero G, Rosa D, Modlin RL, Brenner MB, Bloom BR, Morita CT. Nonpeptide ligands for human gamma delta T cells. Proc Natl Acad Sci U S A 1994; 91:8175-9; PMID:8058775; http://dx.doi.org/10.1073/pnas.91.17.8175
- Grunder C, van Dorp S, Hol S, Drent E, Straetemans T, Heijhuurs S, Scholten K, Scheper W, Sebestyen Z, Martens A, et al. gamma9 and delta2CDR3 domains regulate functional avidity of T cells harboring gamma9delta2TCRs. Blood 2012; 120:5153-62; PMID:23018643; http://dx.doi.org/10.1182/blood-2012-05-432427
- Porcelli SA, Morita CT, Modlin RL. T-cell recognition of non-peptide antigens. Curr Opin Immunol 1996; 8:510-6; PMID:8794014; http://dx.doi.org/10.1016/S0952-7915(96)80039-2
- Mo H, Elson CE. Studies of the isoprenoid-mediated inhibition of mevalonate synthesis applied to cancer chemotherapy and chemoprevention. Exp Biol Med (Maywood) 2004; 229:567-85; PMID:15229351
- MacIver NJ, Michalek RD, Rathmell JC. Metabolic regulation of T lymphocytes. Annu Rev Immunol 2013; 31:259-83; PMID:23298210; http://dx.doi.org/10.1146/annurev-immunol-032712-095956
- Schroder K, Tschopp J. The inflammasomes. Cell 2010; 140:821-32; PMID:20303873; http://dx.doi.org/10.1016/j.cell.2010.01.040
- Zitvogel L, Kepp O, Galluzzi L, Kroemer G. Inflammasomes in carcinogenesis and anticancer immune responses. Nat Immunol 2012; 13:343-51; PMID:22430787; http://dx.doi.org/10.1038/ni.2224
- Li W, Okuda A, Yamamoto H, Yamanishi K, Terada N, Yamanishi H, Tanaka Y, Okamura H. Regulation of Development of CD56(bright)CD11c(+) NK-like Cells with Helper Function by IL-18. PLoS One 2013; 8:e82586; PMID:24376549
- Sugie T, Murata-Hirai K, Iwasaki M, Morita CT, Li W, Okamura H, Minato N, Toi M, Tanaka Y. Zoledronic acid-induced expansion of gammadelta T cells from early-stage breast cancer patients: effect of IL-18 on helper NK cells. Cancer Immunol Immunother 2013; 62:677-87; PMID:23151944; http://dx.doi.org/10.1007/s00262-012-1368-4
- Amrani YM, Gill J, Matevossian A, Alonzo ES, Yang C, Shieh JH, Moore MA, Park CY, Sant'Angelo DB, Denzin LK. The Paf oncogene is essential for hematopoietic stem cell function and development. J Exp Med 2011; 208:1757-65; PMID:21844206; http://dx.doi.org/10.1084/jem.20102170
- Dieli F, Vermijlen D, Fulfaro F, Caccamo N, Meraviglia S, Cicero G, Roberts A, Buccheri S, D'Asaro M, Gebbia N, et al. Targeting human {gamma}delta} T cells with zoledronate and interleukin-2 for immunotherapy of hormone-refractory prostate cancer. Cancer Res 2007; 67:7450-7; PMID:17671215; http://dx.doi.org/10.1158/0008-5472.CAN-07-0199
- Caccamo N, Dieli F, Wesch D, Jomaa H, Eberl M. Sex-specific phenotypical and functional differences in peripheral human Vgamma9/Vdelta2 T cells. J Leukoc Biol 2006; 79:663-6; PMID:16461739; http://dx.doi.org/10.1189/jlb.1105640
- Rodriguez MW, Paquet AC, Yang YH, Erle DJ. Differential gene expression by integrin beta 7+ and beta 7- memory T helper cells. BMC Immunol 2004; 5:13; PMID: 15236665; http://dx.doi.org/10.1186/1471-2172-5-13
- Annunziato F, Cosmi L, Liotta F, Maggi E, Romagnani S. Defining the human T helper 17 cell phenotype. Trends Immunol 2012; 33:505-12; PMID:22682163; http://dx.doi.org/10.1016/j.it.2012.05.004
- Chan CJ, Martinet L, Gilfillan S, Souza-Fonseca-Guimaraes F, Chow MT, Town L, Ritchie DS, Colonna M, Andrews DM, Smyth MJ. The receptors CD96 and CD226 oppose each other in the regulation of natural killer cell functions. Nat Immunol 2014; 15(5):431-8; http://dx.doi.org/10.1038/ni.2850. [Epub ahead of print].
- Dickson RB, Willingham MC, Pastan IH. Receptor-mediated endocytosis of alpha 2-macroglobulin: inhibition by ionophores and stimulation by Na+ and HCO3(-). Ann N Y Acad Sci 1982; 401:38-49; PMID:6188403; http://dx.doi.org/10.1111/j.1749-6632.1982.tb25705.x
- Cohnen A, Chiang SC, Stojanovic A, Schmidt H, Claus M, Saftig P, Janßen O, Cerwenka A, Bryceson YT, Watzl C. Surface CD107a/LAMP-1 protects natural killer cells from degranulation-associated damage. Blood 2013; 122:1411-8; PMID:23847195; http://dx.doi.org/10.1182/blood-2012-07-441832
- Gruenbacher G, Gander H, Rahm A, Nussbaumer W, Romani N, Thurnher M. CD56+ human blood dendritic cells effectively promote TH1-type gammadelta T-cell responses. Blood 2009; 114:4422-31; PMID:19762486; http://dx.doi.org/10.1182/blood-2009-06-227256