Abstract
In the present study, we describe forkhead box O3 (FOXO3)-specific, cytotoxic CD8+ T cells existent among peripheral-blood mononuclear cells (PBMCs) of cancer patients. FOXO3 immunogenicity appears specific, as we did not detect reactivity toward FOXO3 among T cells in healthy individuals. FOXO3 may naturally serve as a target antigen for tumor-reactive T cells as it is frequently over-expressed in cancer cells. In addition, expression of FOXO3 plays a critical role in immunosuppression mediated by tumor-associated dendritic cells (TADCs). Indeed, FOXO3-specific cytotoxic T lymphocytes (CTLs) were able to specifically recognize and kill both FOXO3-expressing cancer cells as well as dendritic cells. Thus, FOXO3 was processed and presented by HLA-A2 on the cell surface of both immune cells and cancer cells. As FOXO3 programs TADCs to become tolerogenic, FOXO3 signaling thereby comprises a significant immunosuppressive mechanism, such that FOXO3 targeting by means of specific T cells is an attractive clinical therapy to boost anticancer immunity. In addition, the natural occurrence of FOXO3-specific CTLs in the periphery suggests that these T cells hold a function in the complex network of immune regulation in cancer patients.
Abbreviations:
- APC, antigen presenting cell
- CTL, cytotoxic T lymphocyte
- CTLA4, cytotoxic T-lymphocyte associated protein 4
- DC, dendritic cell
- FOXO3, forkhead box O3
- IDO, indoleamine-2,3-dioxygenase
- PBMC, peripheral blood mononuclear cell
- TADC, tumor-associated DCs
- TGFβ, tumor growth factor β
- TNFα, tumor necrosis factor α
- Tregs, regulatory T cell
Introduction
The immune system responds effectively to infecting pathogens (non-self), while remaining unresponsive, and thus tolerant, to the body's own cells (self).Citation1 Several different cell types are involved in a complex network of central and peripheral tolerance mechanisms that ensure immune homeostasis, including regulatory T cells (Tregs), dendritic cells (DCs) and myeloid-derived suppressor cells.
DCs are essential to the initiation of a productive immune response by presenting antigen to T cells and by the secretion of pro-inflammatory cytokines. However, DCs are also responsible for controlling overactive immunity by regulating immune tolerance. While tolerance is an essential component of protecting the host from autoimmune disease, it is a major complication and obstacle in the pursuit of better immune-based therapies for cancer. Tumor-associated DCs (TADCs) are central to immunosuppressive mechanisms constraining tumor-specific immunity.Citation2,3 Many different molecules are responsible for the tolerogenic role of TADCs and it was recently shown that the enzyme indoleamine-2,3-dioxygenase (IDO) plays an important role in the occurrence of DC-mediated T-cell tolerance.Citation4 Tolerogenic DCs may also express inhibitory ligands on their cell surface (e.g., PD-L1 and PD-L2), produce immunosuppressive factors such as interleukin-10 (IL-10), tumor growth factor β (TGFβ), and Arginase (ARG1), all factors that suppress T-cell immunity.Citation5
Recently, pioneering work by Hurwitz and colleagues showed that increased expression of forkhead box O3 (FoxO3) was associated with or even responsible for TADC-induced T-cell tolerance in both mice and humans.Citation6,7 Additionally, they described elevated levels of FOXO3 expression in TADCs compared with non-tumor-associated DCs in prostate cancer patients. Elevated expression of FOXO3 in DC may be mediated by cytotoxic T-lymphocyte-associated protein 4 (CTLA4) interaction with B7 molecules.Citation8 FoxO3 induction may inhibit the production of immunostimulatory and inflammation-associated molecules, such as IL-6 and tumor necrosis factor (TNF). In contrast, attenuation of FoxO3 expression leads to reduced levels of the suppressive factors TGFβ, IDO and Arginase, as well as increased expression of the co-stimulatory molecule CD80 and the pro-inflammatory cytokine IL-6, molecular changes culminating in reduced DC tolerogenicity.Citation6 In support,, downregulation of FoxO3 expression resulted in more stimulatory DCs.Citation6,9
FoxO3 is a member of the Forkhead box transcription factor class-O family and was originally, described as a tumor suppressor gene.Citation10 In general, FoxO transcription factors play a crucial role in the regulation of a number of cellular functions, including cell death, protection from stress, and cell cycle arrest.Citation11 In this regard, FoxO3 inactivation leads to deregulated cell proliferation and has been associated with tumorigenesis. FoxO3 over-expression has been shown to inhibit tumor growth.Citation12 However, it was recently suggested that FoxO3a may in addition promote invasive tumor migration, allowing tumors to invade neighboring tissues and ultimately metastasize to distant organs.Citation13 Furthermore, importantly, nuclear exclusion of FoxO3a contributes to cell survival, and cytoplasmic location of FoxO3 seems to correlate with many forms of cancer and has been associated with poor survival in breast cancer.Citation14 Cytotoxic T lymphocytes (CTLs) recognize HLA-restricted, antigenic peptides, which are generated by proteasomal degradation in the cytoplasm.Citation15 We recently described specific CTL-responses in cancer patients toward another transcription factor, forkhead box P3 (FOXP3) that is generally expressed in Tregs.Citation16 Due to the important function of FoxO3 in cancer and immunity, in the current study we examined whether FOXO3 is a natural target for CTLs in human cancer patients and characterized the cytolytic capacity of these effector T cells.
Results
Spontaneous T-cell reactivity against FOXO3 in cancer patients
The amino acid sequence of the FOXO3 protein was screened using the database “SYFPEITHI”Citation17 to predict the best HLA-A2 nona-peptide epitopes. Two peptides were predicted to be top candidates with a predictive score of 28; FoxO392–100 (LLLEDSARV) and FoxO3118–126 (GLSGGTQAL). These 2 peptides were synthesized for further studies. We examined peripheral blood mononuclear cells (PBMCs) from different HLA-A2+ cancer patients as well as healthy donors for the presence of specific T-cell responses against the peptides by means of the IFNγ ELISPOT assay. Previously, we have made use of this assay for the identification of antigen-specific T-cell responses in cancer patients.Citation18–24 In total, we scrutinized PBMCs from 27 HLA-A2+ cancer patients (i.e., 5 patients with renal cell carcinoma, 16 patients with melanoma and 6 patients suffering from breast cancer) for reactivity against the 2 FOXO3-derived peptides (). Frequent and strong responses were detected against both peptides in several cancer patients and in all 3 forms of cancer. Notably we did not detect any responses against FoxO392–100 or FoxO3118–126 among PBMCs from healthy individuals. The difference between immune responses in healthy donors and cancer patients was illustrated by a Mann-Whitney test showing that the difference was significant for both peptides (P = 0.0001 and P = 0.0125, respectively). Examples of significant responses against each peptide are depicted in bar plots in , in which responses are compared to background for each patient.
Next, we examined 3 responding patients for the presence of FoxO3118–126 -specific cells directly ex vivo, without prior peptide stimulation in vitro (). Using the IFNγ ELISPOT assay, 2 renal cell carcinoma patients (RCC41 and RCC44) showed statistically significant T-cell responses to FoxO3118–126 ex vivo. The ELISPOT response of RCC44 is depicted in . Next we utilized an HLA-A2/FoxO3118–126 tetramer to detect and examine reactive T cells by flow cytometry. However, upon staining PBMCs from RCC44 with the HLA-A2/FoxO3118–126 tetramer, we failed to detect tetramer-positive cells directly ex vivo (). This discrepancy might be explained by the presence of non-HLA-A2 restricted CD4+ and CD8+ T cells contributing to the apparent ELISPOT response. However, it could also be caused by a low T-cell avidity. Next, we used PBMCs from RCC44 to generate T-cell bulk cultures against FoxO3118–126 in vitro. We stimulated PBMCs from the patient with FoxO3118–126 -pulsed autologous DCs in vitro and stained these cultures for HLA-A2/FoxO3118–126 –positive cells. After several rounds of peptidere-stimulation in vitro, a clear population of tetramer-positive T cells became detectable (). The culture was enriched for HLA-A2/ FoxO3118–126 -positive T-cells by fluorescence-activated cell sorting (FACS) isolation of tetramer-specific cells. Expansion of the isolated cells resulted in T-cell cultures of very high specificity (90–100% specific T cells) ().
Figure 1. T-cell responses against FoxO3 in cancer patients compared to healthy individuals. (A–D) Peripheral blood mononuclear cells (PBMCs) derived from cancer patients or healthy donors were assayed for reactivity to FoxO3 peptides by interferon γ (IFNγ) ELISPOT assay. (A) T-cell responses against the FoxO392–100 (LLLEDSARV) peptide as measured by interferon γ (IFNγ) ELISPOT. PBMCs from 5 patients with renal cell carcinoma (RCC), 16 with malignant melanoma (MM), 6 breast cancer patients (CM) and 10 healthy donors (HD), were stimulated once with peptide and screened for responses against FoxO392–100 using IFNγ ELISPOT. The average number (in triplicates) of FoxO392–100-specific spots (after subtraction of spots with irrelevant HIVpol476–484 added peptide) was calculated per 5 × 105 PBMCs for each patient. A Mann-Whitney test was used to determine the significance of the response against the FoxO392–100 peptide in cancer patients compared to healthy donors; P-value = 0.0001. (B) In vitro IFNγ ELISPOT responses against the FoxO3118–126 (GLSGGTQAL) peptide measured in a similar manner as for the FoxO392–100 peptide. The average number (in triplicates) of FoxO3118–126 -specific spots (after subtraction of spots with irrelevant HIVpol476–484 peptide) were calculated per 5 × 105 PBMCs for each patient, with the exception of patient RCC44 (as indicated with *) who displayed a robust response per 2 × 105 PBMCs. A Mann-Whitney test was used to determine the significance of reactivity of FoxO3118–126 specific T cells in cancer patients compared to healthy donors; P-value = 0.0125. (C) Examples of significant ELISPOT responses to FoxO392–100 (black bars) as compared to irrelevant HIVpol476–484 peptide (gray bars) among PBMCs from different cancer patients, including 3 melanoma patients (MM03, MM25 and MM129), a breast cancer patient (CM.16) and 2 renal cell carcinoma patients (RCC41 and RCC44). (D) Examples of significant ELISPOT responses to FoxO3118–126 (black bars) as compared to irrelevant HIVpol476–484 peptide (gray bars) in PBMCs from different cancer patients, including a melanoma patient (MM129) and 2 renal cell carcinoma patients (RCC41and RCC44).
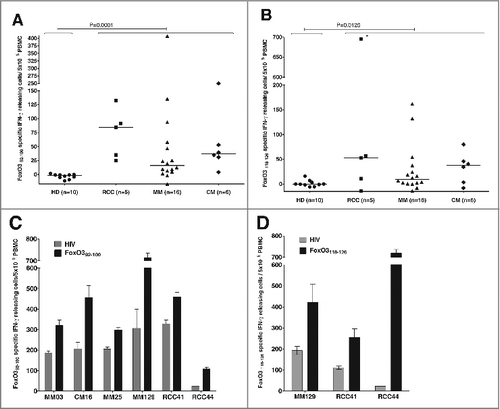
Figure 2. FoxO3118–126-specific T cells are present among PBMC ex vivo and are able to expand in vitro. (A–B) FoxO3118–126 responsive peripheral blood mononuclear cells (PBMCs) from cancer patients (refer to Figure 1) were assayed for reactivity to FoxO3 peptides without prior peptide stimulation by ELISPOT assay to detect interferon γ (IFNγ). Ninety-six-well plates coated with anti-human IFNγ antibody were incubated with PBMCs and the indicated peptide (in triplicates). (A) Ex vivo IFNγ ELISPOT as measured against either HIVpol476–484 (gray bars) or FoxO3118–126 (black bars) reactivity among PBMCs from 3 cancer patients. The renal cell carcinoma (RCC) patients RCC41 and RCC44 exhibited significant responses against FoxO3118–126 ex vivo; one-tailed Student's t-test. (B) Representative images (RCC44) from an IFNγ ELISPOT response against FoxO3118–126 compared to HIVpol476–484 ex vivo. (C) Immunofluorescence staining of PBMCs from RCC44 with fluorophore-conjugated tetramer-complexes HLA-A2/HIVpol476–484-PE and HLA-A2/HIVpol476–484-APC (left) or HLA-A2/FoxO3118–126-PE and HLA-A2/FoxO3118–126-APC (right). (D) Expansion of FoxO3118–126-specific T cells from PMBCs from RCC44 by culturing ex vivo, as measured by immunofluorescence staining with tetramer-complexes and cytofluorimetric analysis. The staining of the specific T-cell culture was performed after either several rounds of in vitro peptide stimulations using HLA-A2/HIVpol476–484-PE and HLA-A2/HIVpol476–484-APC (left panel) or HLA-A2/FoxO3118–126-PE and HLA-A2/FoxO3118–126-APC (second panel), or after further purification of FoxO3-specific T cells by tetramer-based cell sorting with HLA-A2/FoxO3118–126-PE and HLA-A2/FoxO3118–126-APC (third panel), or with HLA-A2/FoxO3118–126-PE or HLA-A2/FoxO3118–126-APC (right panel).
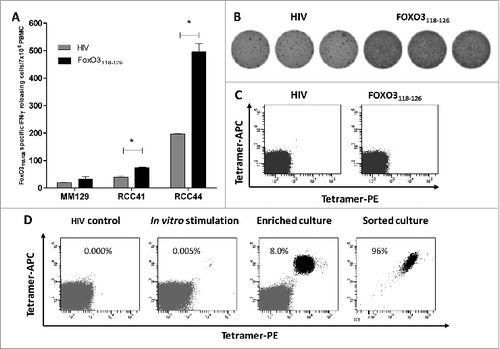
Cytotoxicity of FoxO3-specific CD8+ T cells
To analyze the functional capacity of ex vivo expanded FoxO3-specific T-cells we first analyzed the effect of FoxO3118–126 peptide stimulation of a tetramer-positive T-cell culture (). In this regard, upon FoxO3118–126-recognition the HLA-A2/ FoxO3118–126-positive T cells produced both IFNγ and TNFα as measured by intracellular staining and cytofluorimetric analysis (). In addition, the cells displayed the cytolytic marker CD107a on their surface ().Citation25 Next, the cytotoxic functional properties of different FoxO3-specific bulk cultures was tested in standard 51Cr release assays using transporter associated with antigen processing (TAP)-deficient T2 cells as target cells either loaded with FoxO3118–126 or an irrelevant control peptide from human immunodeficiency virus (HIV). T2 cells pulsed with FoxO3118–126 were lysed by the FoxO3118–126 specific T cells, whereas T2 cells pulsed with the irrelevant peptide from HIV were not lysed (). As expected, the specific lysis of FoxO3118–126 -pulsed T2-cells was more efficient after sorting of the HLA-A2/FoxO3118–126 specific cells ().
Figure 3. Cytotoxic functional capacity of cancer patient-derived FoxO3118–126-specific T cells (A–D). Tetramer-staining and cytotoxicity-testing of a sorted T-cell culture with (E) low, (F) high and (G) pure FOXO3-specific T cells. Immunofluorescence staining and cytofluorimetric analysis of ex vivo-expanded, peptide-stimulated T cells. T cells were enriched from a renal cell carcinoma patient (RCC44) by several rounds of FoxO3-peptide stimulation and culturing in the presence of cytokines. (A) Tetramer analysis of FoxO3118–126-specific T cells from RCC44 T-cell culture stained with the following fluorophore-conjugated tetramers: HLA-A2/HIVpol476–484-PE and HLA-A2/HIVpol476–484-APC (top) or HLA-A2/FoxO3118–126-PE and HLA-A2/FoxO3118–126-APC (bottom). (B) Intracellular tumor necrosis factor α (TNFα) and interferon γ (IFNγ) staining analysis of the FoxO3118–126-specific culture stimulated with either HIVpol476–484 (top) or FoxO3118–126 (bottom) peptide for 5 h. (C and D) Cell surface expression of CD107a and production of IFNγ (C) and TNFα (D) by the FoxO3118–126–specific T-cell culture stimulated with either HIVpol476–484 (top) or FoxO3118–126 (bottom) peptide for 5 h. (E) Staining (left) of the specific T-cell culture by HLA-A2/HIVpol476–484-PE and HLA-A2/HIVpol476–484-APC (top) or HLA-A2/FoxO3118–126-PE and HLA-A2/FoxO3118–126-APC (bottom) and cytotoxic killing (right) by the same T-cell culture of T2 cells pulsed with irrelevant HIV-peptide (circles) or with FoxO3118–126 (squares). (F) FoxO3-specific T-cell culture tetramer-staining and cytotoxic killing of T2 cells loaded with either irrelevant HIV-peptide (circles) or with FoxO3118–126 (squares). (G) High specificity of FoxO3-specific T-cell culture enriched by tetramer-based cell sorting. tetramer-staining and cytotoxic killing of T2 cells loaded with either irrelevant HIV-peptide (circles) or with FoxO3118–126 (squares).
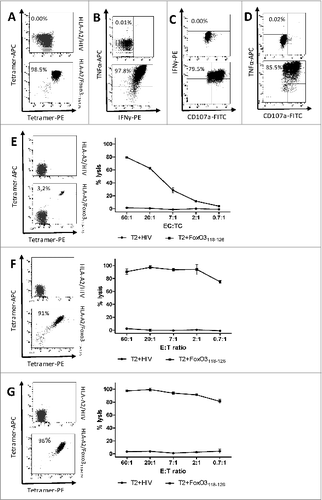
K562 cells are leukemic cells that express FoxO3Citation26 but do not express any HLA molecules. To establish the HLA-restriction as well as potential NK-cell mediated killing by the FoxO3118–126 specific T cells, we utilized either K562 cells transduced to express HLA-A1 or HLA-A2 or non-transduced K562 cells as target cells in 51chromium-release assays (). The HLA-A2 transduced FoxO3 expressing cells were effectively lysed by the FoxO3118–126-specific CTL, whereas the HLA-A1 transduced, as well as the non-HLA expressing cells, were not recognized. Hence, the killing by FoxO3118–126-specific CTL was, indeed, HLA-A2-restricted. Additionally, FoxO3 siRNA-mediated downregulation of FOXO3 protein expression in the HLA-A2-transduced K562 cells reduced the killing of the transduced K562 cells, implicating a link between the level of intracellular FOXO3 in target cells and FoxO3-specific T-cell cytotoxicity (). The expression levels of FOXO3 in the various K562 cells was confirmed by Western Blot and was not affected by the transduction with either HLA-A1 or HLA-A2 (). Furthermore, Western Blot confirmed that the use of FoxO3 siRNA reduced the level of FOXO3 protein expression in FoxO3 siRNA-treated K562 cells, although the levels were only partially knocked down ().
Figure 4. T-cell mediated killing is dependent on HLA-expression and intracellular levels of FOXO3. Cytotoxicity of FoxO3-specific T cells and dependence on target cell levels of HLA and FoxO3 was measured by chromium51-release assay with and without exogenous HLA or siRNA-mediated knockdown of FoxO3. (A) Lysis of the FoxO3-expressing, HLA-class I-deficient leukemic cell line K562 (circles) as well as K562 cells transduced with either HLA-A1 (K562/HLA-A1; squares) or HLA-A2 (K562/HLA-A2; stars) by FoxO3118–126-specific T cells. (B) Lysis of K562/HLA-A2 transfected with either control siRNA (circles) or FoxO3 siRNA (squares) by FoxO3118–126-specific T cells. Statistical analysis was performed by unpaired Student's t-test; * P<0 .05. (C) Expression level of FOXO3-protein examined by Western Blot analysis in the cell lines K562, K562/HLA-A1, K562/HLA-A2, K562/HLA-A2 transfected with either FoxO3 siRNA or control siRNA. Densitometric quantification and blotted membranes are shown.
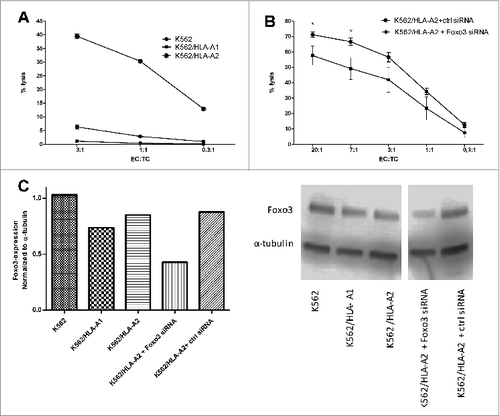
FoxO3-specific CD8 T cells recognize dendritic cells
We have previously described that self-reactive T cells, i.e., IDO- and PD-L1- specific CTLs, are able to specifically recognize standard prostaglandin E2 (PGE-2) matured autologous DCs generated in vitro.Citation24,27 First, the high expression of FoxO3 in our PGE2-matured DCs was confirmed by Western Blot analysis of DCs derived from 5 different patients. Thus, we next examined if FoxO3118–126-specific CTLs recognize autologous DCs acquired by this standard in vitro technique. We found that FoxO3118–126-specific CTLs released both IFNγ as well as TNFα in response to autologous, standard matured DCs in contrast to autologous monocytes, as determined by intracellular staining and fluorescence cytometry (). Similarly, FoxO3118–126-specific CTLs effectively lysed autologous DCs generated in vitro as determined by 51chromium-release assay (). Additionally, FoxO3 siRNA-mediated FoxO3 protein knockdown in autologous DCs attenuated the T-cell mediated killing of dendritic target cells (). This was confirmed in 3 independent assays. Western Blot analysis confirmed that the use of FoxO3 siRNA reduced the level of FOXO3 protein in the DCs, although the level was only partially downregulated ().
Figure 5. FoxO3118–126-specific T cells recognize and kill autologous dendritic cells. (A-D) Dendritic cells were derived from cancer patient peripheral blood monocytes enriched by adherence to plastic and cytokine stimulation ex vivo. FoxO3118–126-specific T cells response to autologous matured DCs and monocytes were determined by intracellular staining and fluorescence cytometry (A and B) or cytotoxicity assay (C). (A) Intracellular interferon γ (IFNγ) staining of FoxO3118–126-specific T cells stimulated with either directly isolated monocytes (left), or in vitro generated DC (right) for 5 h. (B) Intracellular tumor necrosis factor α (TNFα) staining of FoxO3118–126-specific T cells stimulated with either directly isolated monocytes (left), or in vitro generated DC (right) for 5 h. (C) Chromium51-release assay to determine the FoxO3118–126-specific T cell-mediated lysis of autologous, in vitro generated DCs transfected with control siRNA (circles) versus a FoxO3-specific siRNA (squares). Statistical analysis was performed by unpaired Student's t-test; *P < 0 .05. (D) Expression level of FOXO3-protein examined by Western Blot analysis in the autologous, in vitro generated DC transfected with a FoxO3-specific siRNA or autologous, in vitro generated DC (mock). Densitometric quantification and blotted membranes are shown.
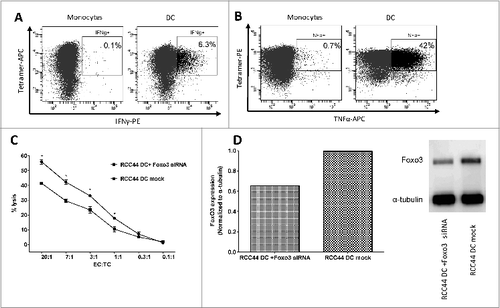
FoxO3118–126 -specific CTL skill cancer cells of different origin and B cells that cross present the epitope
FoxO3 is reportedly overexpressed in many cancers. We therefore examined if the FoxO3118–126-specific CTLs were able to recognize cancer cells of different origins. First, we examined the melanoma cell line FM55M1. We found that FoxO3118–126-specific CTLs release both IFNγ as well as TNFα in response to FM55M1, as determined by intracellular staining and fluorescence cytometry (). In order to examine the ability of the FoxO3118–126-specific CTL culture to kill cancer cells, FM55M1 cells were used as targetsin51chromium-release assays. We found that the CTL culture successfully recognized and effectively killed the HLA-A2+ FM55M1 cells (). Next, we examined 2 additional HLA-A2 positive melanoma cells lines, namelyFM6 and FM28. The FoxO3118–126-specific CTL efficiently lysed cells from both cell lines ()., We next sought to examine the universal character of the FoxO3118–126-specific CTL-mediated lysis by evaluating the ability of the FoxO3-specific T cells to recognize cancer cells of differing origins. Hence, the HLA-A2 positive breast cancer cell line MDA-MB-231 and the HLA-A2 positive colon cancer cell line SW480 were subsequently examined. The FoxO3118–126-specific CTLs recognized and killed both of these HLA-A2 positive cancer cell lines (). B cells are known to cross-present peptides. Hence, we utilized the FoxO3-negative Epstein Barr virus (EBV)-transformed B cell line KIG-Bcl to show that B cells are able to take up and cross-present a 23 amino acid long peptide (FoxO3116–138) that included the sequence of FoxO3118–126 (). To this end, the FoxO3118–126-specific CTLs were examined against KIG-Bcl cells pulsed with either the long FoxO3116–138 peptide, an irrelevant HIV peptide (as negative control) or the actual epitope FoxO3118–126 (as positive control). The long peptide FoxO3116–138 was efficiently presented by HLA-A2 on the surface of KIG-Bcl cells, since these cells were killed by FoxO3118–126-specific CTLs almost as effectively as the positive control cells. Of note, the KIG-Bcl cells did not appear to express FOXO3 protein as determined by Western Blot (). The expression of FOXO3 in the cancer cell lines used here have been previously describedCitation28–30 and was confirmed by Western Blot ().
Figure 6. FoxO3118–126-specific T cells recognize and kill a broad variety of cancer cells. (A-G) Cancer patient derived FoxO3118–126-specific T cells responses to melanoma, breast and colon cancer cells, as well as an antigen presenting B cell line were determined by intracellular staining and fluorescence cytometry (A) or cytotoxicity (chromium51 release) assay (B-G). (A) Intracellular tumor necrosis factor α (TNFα) and interferon γ (IFNγ) staining analysis of the FoxO3118–126-specific T-cell culture stimulated with either HIVpol476–484 (left) or FM55-M1 cells (right) at an effector to target cell (EC:TC) ratio of 10:1 for 5 h. (B) Lysis of the HLA-A2+ melanoma cell line FM55-M1 (squares) by a FoxO3118–126-specific T-cell culture at different EC:TC ratios. (C-F) FoxO3118–126-specific cultured T cell-mediated lysis of the HLA-A2+ melanoma cell line FM6 (C), the HLA-A2+ melanoma cell line FM28 (D), the HLA-A2+ breast cancer cell line MDA-MB-231 (E), and the HLA-A2+ colon cancer cell line SW480 (F). (G) FoxO3118–126-specific cultured T cell culture-mediated lysis of the HLA-A2+ EBV transfected B-cell line (KIG-Bcl) alone (circles) versus cells pulsed with long-peptide FoxO3116–138 (stars) or the FoxO3118–126-epitope (squares). (H) Densitometric quantification and blots of the expression of FOXO3 in a Western Blot of the cancer cell lines (FM55-M1, FM6, FM28, MDA-MB-231, and SW480) and the EBV-transfected B-cell line (KIG-Bcl).
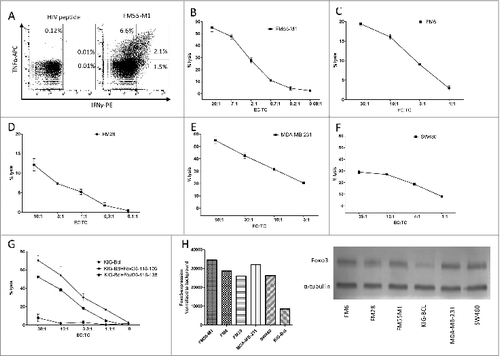
Discussion
In the present study we examined whether the transcription factor FOXO3 is a natural target for CTLs in cancer patients. In this respect, we found that peripheral blood from cancer patients harbors CD8+ T cell reactivity toward FOXO3 expressing cells by means of the enzyme-linked immunospot (ELISPOT) assay. We detected reactivity against 2 HLA-A2-restricted FoxO3-derived peptides in patients suffering from unrelated tumor types—i.e., melanoma, renal-cell carcinoma and breast cancer. Even more remarkably, we showed high numbers of specific T cells in a few cancer patients in whom we were able to measure specific T-cell responses directly ex vivo, which is highly unusual.Citation31 In contrast we were unable to detect immune responses against FoxO3 peptides in healthy controls. Hence, the immune responses toward FOXO3 were restricted to patients suffering from a malignant disease.
It has recently been described that FoxO3+ DCs play a critical role in the regulation of tumor immunity, i.e., modulating host responses to tumors.Citation2,3,7 Hence, FoxO3 has been described as a crucial player in the programming of the inflammatory versus the tolerogenic potential of dendritic cells. Further support for the regulatory role of FoxO3 in immune cells was recently published, showing that increased expression of FOXO3 in human monocytes leads to production of fewer pro-inflammatory cytokines and more IL-10 by these cells. Interestingly, the single nucleotide polymorphism that regulates this increased expression of FOXO3 was associated with a less severe course of the autoimmune diseases Crohn's disease and rheumatoid arthritis.Citation32
Here, we used FoxO3+, PGE2-matured DCs generated from blood monocytes to demonstrate the cytotoxicity exerted by FoxO3-reactive CTLs. Despite the fact that this strategy was primarily chosen in order to surmount the impracticality of isolating and labeling TADCs with 51Cr for cytotoxicity assays, it nevertheless illustrates that FoxO3-specific CTLs are capable of recognizing and killing FOXO3-expressing immune cells. In addition, we established that the FoxO3-specific CTLs recognized and killed FOXO3-expressing cancer cells. Thus, FoxO3-specific T cells effectively lysed FoxO3+ solid cancer cell lines of different origin. Hence, in both DCs and cancer cells the intracellular FOXO3 protein was degraded and the peptide epitope subsequently processed and presented on the cell surface in the context of HLA-A2 molecules. We further showed that a long peptide derived from FOXO3 was cross-presented on the surface of non-professional antigen-presenting cells (APCs), namelyEBV-transformed B cells). Non-professional APCs have previously been shown to cross-present HLA class I-restricted epitopes from exogenous NY-ESO or PD-L1 polypeptides.Citation27,33
The noticeable lack of tolerance against FOXO3 peptides in cancer patients could suggest a more general role for FoxO3-specific CTLs in the comprehensive regulation of the immune system. Hence, FoxO3-specific CTLs may represent a feedback mechanism to suppress the function of immunosuppressive APCs. In this respect, FOXO3 is not the only protein expressed in regulatory immune cells that have been described as potential targets for CTLs in recent years. Thus, spontaneous CD8+ T-cell responses toward epitopes derived from proteins such as FOXP3, IDO as well as PD-L1 have been described.Citation16,27,Citation34–37 These are all normal self proteins inducible in immune cells under many different physiological and/or pathological conditions, such as inflammation, infection and cancer. In normal ontogeny and tissue homeostasis in healthy organisms, the elimination of certain cells by cytolytic events is a fundamental regulatory mechanism.Citation38,39 Thus, self-reactive CTL responses may be important to maintain the homeostasis of the immune system. We have previously suggested the term “supporter T cells” for similar IDO-specific, CD8+ T cells.Citation35 Hence, FoxO3-specific CTLs could play an important role by eliminating regulatory APCs, thereby boosting effector immune responses. To understand this it will be important to consider the expression of FoxO3 in normal cells, especially since FoxO3 is expressed in the hippocampus, cortex and cerebellumCitation40 as well as in the placentaCitation41 and the intestinal epithelia.Citation42 FoxO3 is a critical transcription factor for normal cell cycle regulation. It is involved in numerous processes such as angiogenesis, cardiovascular development, vascular tone, oxidative stress, stem-cell proliferation, fertility, immunity and cancer. Thus many of the overall biological functions of self-reactive FoxO3-specific CTLs may vary depending on the microenvironment and the state of the immune response.
In terms of using FOXO3 as a target in clinical cancer therapy, attenuating FOXO3 protein expression by the silencing of the FOXO3 gene is complicated by the fact that FoxO3 is not only important for modulating DC function but may also impact cancer cell survival via targeting by FoxO3-specific CTLs. Thus, FOXO3 knockdown should somehow specifically target FOXO3 gene expression in dendritic cells, thus preventing downregulation of FOXO3 in tumor cells. Alternatively, FoxO3-specific CTLs may be immensely useful for immune therapy. The induction of FoxO3-specific immune responses could function highly synergistically with additional anticancer immunotherapy, not only by eliminating cancer cells but especially TADCs, which suppress anticancer immunity. Obviously, one should be especially careful of autoimmune reactions when aiming at a self protein such as FOXO3. However, the circulation of a detectable number of FoxO3-specific T cells measurable directly ex vivo did not seem to have caused autoimmunity in the patients. The questions remain under what conditions FoxO3-specific T cells become activated and what the potential role of such auto-reactive T cells is in regards to immune regulation and cancer. Thus, the data presented here justify further examination of the role for FoxO3-specific CTLs in immunity and the potential anticancer therapeutic use of FoxO3-specific CTLs in the clinic.
Materials and Methods
Patients
Peripheral blood mononuclear cells (PBMCs) were collected from healthy individuals (average age of 41 years) and cancer patients (melanoma, renal cell carcinoma and breast cancer patients with an average age of 64 years). Blood samples were drawn a minimum of 4 weeks after termination of any kind of anticancer therapy. PBMCs were isolated using Lymphoprep (Fresenius Kabi, Oslo, Norway) separation, HLA-typed and frozen in fetal calf serum (FCS) with 10% dimethyl sulfoxide (DMSO). The protocol was approved by the Scientific Ethics Committee for The Capital Region of Denmark and conducted in accordance with the provisions of the Declaration of Helsinki. Written informed consent from the patients was obtained before study entry.
Peptides
To identify HLA-A2 restricted CTL epitopes for FoxO3, the amino acid sequence of FoxO3 was analyzed using the database SYFPEITHI (http://www.syfpeithi.de) and the epitope prediction search option.Citation17 Two nonamer peptides, FoxO392–100 (LLLEDSARV) and FoxO3118–126 (GLSGGTQAL), scored highest according to the SYFPEITHI algorithm, and thus came out as the top candidate epitopes. The HLA-A2 high affinity binding epitope HIV-1 pol476–484 (ILKEPVHGV) was used as a control. In addition a 23 amino acid long peptide FoxO3116–138 (AGGLSGGTQALLQPQQPLPPPQP) that included the sequence of FoxO3118–126 was synthesized for cross presentation assays.
ELISPOT assay
The ELISPOT assay was used to quantify cytokine (IFNγ) release by peptide specific T cells, as previously described.Citation18 PBMCs from healthy donors or patients were stimulated once in vitro with peptide prior to analysis to increase the sensitivity of the assay, as previously describedCitation43. Briefly, nitrocellulose bottomed 96-well plates (MultiScreen MSIPN4W; Millipore) were coated overnight with the capture anti-human IFNγ antibody (Clone 1D1K, Mabtech). The wells were washed and subsequentlyblocked by X-VIVO medium (Lonza). The PBMCs were added in triplicates or duplicates at different cell concentrations either with relevant or irrelevant peptide and incubated overnight. The wells were washed prior to addition of the biotinylated secondary monoclonal antibody (mAb 7-B6–1-Biotin, Mabtech), followed by the avidin-alkaline phosphatase conjugate (AP-avidin; Mabtech). Finally, the enzyme substrate nitro-blue tetrazolium/ 5-bromo-4-chloro-3’indolyphosphate (NBT/BCIP;Invitrogen Life Technologies) was added to develop spots. The spots were counted using the ImmunoSpot Series 2.0 Analyzer (CTL Analyzers). The definition of an ELISPOT response was based on the guidelines provided by the CIMT Immunoguiding Program (CIP) panel (http://www.cimt.eu/workgroups/cip) as well as Moodie et al.Citation44 using either an empirical or statistical approach. The empirical approach is based on the “signal-to-noise” ratio and suggests that the threshold for response definition should be defined as >6 specific spots per 105 PBMCs. The non-parametric distribution free resampling (DFR) method can be used when experiments are performed in triplicates and allows statistical comparison of antigen-stimulated wells with negative control wells. This method was utilized in . The non-parametric unpaired Mann-Whitney test was used to compare FoxO3-specific responses between cancer patients and healthy donors. P-values <0 .05 were considered significant.
Generation of FoxO3-specific T-cell cultures
Peripheral blood lymphocytes (PBLs) from a renal cell carcinoma patient were stimulated with irradiated (30 Gy) autologous DCs pulsed with FoxO3118–126 peptide (PBL:DC ratio 10:1) and 40 U/mL IL-7 (PeproTech, London, UK). 20 U/mL IL-12 was added the next day (PeproTech, London, UK). Three subsequent stimulations with FoxO3118–126-loaded irradiated autologous DC followed, at weekly intervals with addition of 20 U/mL IL-12 (PeproTech, London, UK) the next day. The culture was then stimulated 3 times with FoxO3118–126-loaded irradiated autologous PBLs (culture:PBL ratio 1:1) at weekly intervals with addition of 120 U/mL IL-2 (Proleukin, Novartis) the next day. The culture was tested for specificity in an IFNγ ELISpot assay and stained with tetramers for cytofluorimetric analysis.
The culture was enriched for specific cells by staining with the fluorophore-conjugated tetramers HLA-A2/FoxO3118–126-PE and HLA-A2/FoxO3118–126-APC followed by flourescence activated cell sorting (FACS) on a FACS Aria flow cytometer (BD Biosciences). The sorted cells were expanded by incubation with 0.6 μg anti-CD3 antibody (eBioscience, clone OKT3), 3000 U/mL IL-2 (Proleukin, Novartis) and allogeneic PBMCs as feeder cells. Sorting was performed twice, starting with a larger gate during the initial sort and, after culturing for 28 d to expand the enriched cells, with a more restrictive gate in a subsequent sort.
Flow cytometry
Staining with tetramers was done in PBS + 2% FCS for 15 min at 37°C, followed by staining with CD4-FITC and CD8-PerCP (BD Biosciences) fluorophore-conjugated antibodies and the dead cell marker LIVE/DEAD Fixable Near-IR Dead Cell Stain Kit (Invitrogen) for 30 min on ice. The tetramers were prepared using the MHC-peptide exchange technology as described.Citation45 The fluorophore-conjugated MHC tetramer complexes used were: HLA-A2/FoxO3118–126-PE, HLA-A2/FoxO3118–126-APC and as a control HLA-A2/HIVpol476–484 -PE and HLA-A2/HIVpol476–484 -APC. Samples were analyzed on a BD FACS Canto using FACS DIVA software (BD Biosciences) and FlowJo software.
Generation of dendritic cells
Dendritic cells (DCs) were generated from monocytes isolated from cancer patient-derived PBMCs by plastic adherence. The monocytes were cultured for 6 d in RPMI-1640 with 10% FCS with 250 U/mL IL-4 and 1000 U/mL GM-CSF (PeproTech). DCs were matured for 2 d by addition of 1000 U/mL IL-1β, 1000 U/mL IL-6, 1000 U/mL TNFα (PeproTech) and 1 μg/mL PGE2 (Cayman Chemical).
Cytotoxicity assay
CTL-mediated cytotoxicity was measured by 51Chromium-release assays, as previously described.Citation46 Target cells included: T2 cells with HIV-1 pol476–484 (ILKEPVHGV) or T2 cells with FoxO3118–126, the melanoma cell lines FM55M1, FM6, FM28,Citation47, the breast cancer cell line MDA-MB-231, or the colon cancer cell line SW480, all available at the American Type Culture Collection (ATCC), the EBV-transformed B-cell line KIG-Bcl,Citation48, the natural killer target cell line K652 or transduced K562 cells expressing HLA-A1 or HLA-A2 (a kind gift from Mariam Heemskerk, University Hospital Leiden, The Netherlands), and autologous DCs generated in vitro. FoxO3 expression has previously been described for the cell lines: MDA-MB-231,Citation29 SW-480,Citation30 K562.Citation26 For the cross-presentation assay KIG-Bcl cells were pulsed for 1 h, with or without the long FoxO3116–138 peptide before being examined in a 4 h cytotoxicity assay.
All cell lines included in the study were tested and authenticated by HLA genotyping. The cell lines were routinely confirmed with their HLA typing and antigen expression by flow cytometry and coculture assays, respectively.
Intracellular cytokine staining
For detection of cell subpopulations producing cytokines, FoxO3-specific T-cell cultures were stimulated with 5 μg/mL of relevant, or irrelevant, peptide for 5 h at 37°C with 5% CO2. Cells were stained with CD107a-FITC (eBioscience) and GolgiPlug (BD Bioscience) at a dilution of 1:200 was added after the first hour of incubation. After 4 additional hours cells were washed twice with PBS, stained with fluorochrome conjugated antibodies for surface markers (CD3-Amcyan, CD4-PerCP and CD8-Pacific Blue, all from BD Bioscience). Cells were washed an additional time and thereafter fixed and permeabilized with Fixation/Permeabilization and Permeabilization Buffer (eBioscience), according to manufacturer's instructions. Cells were subsequently stained with the following fluorochrome-conjugated antibodies for detection of intracellular cytokines: IFNγ-PE (eBioscience), TNFα−APC (eBioscience). Relevant isotype controls were used to enable correct compensation and confirm antibody specificity. At least 105 events were acquired. Stained cells were analyzed using a BD FACSCanto II flow cytometer. Analysis was performed with BD FACSDiva Software. Finally, cytokine production by FoxO3-specific T-cell cultures stimulated with monocytes, matured DC or melanoma cells FM55-M1 (effector: target =10:1) was analyzed.
Downregulation of FoxO3 using siRNA
SignalSilence FoxO3a siRNA I (#6302S) was obtained from Cell Signaling. Medium GC Duplex control stealth RNAi was obtained from Invitrogen. For downregulation of FoxO3, mDC or K562 cells transfected with HLA-A2 (3 × 106) were transfected with 100 pmol FoxO3 siRNA using electroporation parameters, as previously described.Citation49 Mock-transfected DCs used as controls underwent electroporation using the same conditions.
BCA protein ELISA
Cell lines were collected and analyzed for expression of siRNA knock down and mRNA transfection with FoxO3 by protein gel blot. Cells were lysed by addition of 100 uL RIPA buffer + protease and phosphatase inhibitors per 106 cells, and mixed by inverting 30 min at 4˚C. RIPA buffer, protease and phosphatase inhibitors were prepared according to Abcam “Western blotting–a beginner's guide.” To remove DNA and cell debris, cell lysate was sonicated (Branson, Digital sonifier) for 20 sec on ice followed by centrifugation at 18000 x g for 10 min at 4˚C. Protein concentration in cell lysate was measured by Pierce BCA Protein Assay kit (Thermo Scientific) according to manufacturer's recommendations. In short, 200 uL of reagent mix (50:1, reagent A: reagent B) and either 25 uL standard or sample were added per well to a 96F microwell plate (Nunc, Thermo Fisher Scientific). The plate was incubated 30 minat 37˚C and read by ELISA plate reader (Synergy HT, Biotek) at a wavelength of 450 nm.
Western Blot
Western Blot was set up with either 3 or 5 ug total protein of each sample, a Magic Mark XP Western Standard Ladder (Invitrogen) and a Benchmark Prestained Protein Ladder (Invitrogen). Laemmli sample buffer (BioRad) + 5% 2-mercaptoethanol (BioRad) was diluted 1:1 in cell lysate and samples denatured at 100˚C for 5 min. Denatured samples and ladders were loaded onto a 10% Mini protean TGX Stain-free gel (BioRad) and run at 200 V in 10x Tris/Glycine/SDS buffer (BioRad) (diluted 1:10 in dH2O). Protein was transferred to 0.2 μm nitrocellulose membrane (Transblot turbo transfer pack, BioRad) by semidried transfer at 150 A. The membranes were blocked for 1 h in blocking buffer containing PBS + 0.05% Tween20 (Sigma-Aldrich) + 5% skimmed milk powder (BioRad). The membranes were stained with the following primary antibodies: rabbit anti-human FoxO3 (clone 75D8, Cell Signaling Technologies) and mouse anti-human α-tubulin (clone DM1A, Abcam) over night at 4˚C.The next day, membranes were washed and incubated with appropriate horseradish peroxidase conjugated secondary antibodies, includinganti-rabbit IgG HRP-linked antibody (Cell Signaling Technologies) and peroxidase labeled goat anti-mouse IgG (KPL) for 1h at room temperature. Between each staining the membrane was washed 6× for 10 min each in blocking buffer. Following the last wash, the membrane was washed twice in PBS + 0.05% Tween20. For signal development Super Signal West Femto Max Sensitivity Substrate (Thermo Scientific) was used according to manufacturer's recommendations, and the membranes were photographed by a C-DiGit® Chemiluminescent Blot Scanner (Li-Cor).
Statistical analysis
Statistical analysis was performed using GraphPad Prism 5. Comparison of Elispot responses between healthy donors and cancer patients was performed using a two-sided Mann-Whitney test. For individual patients, the difference between elispot responses against control HIV peptide and FOXO3 peptides was tested using the non-parametric distribution free resampling method (DFR), proposed by Moodie et al.Citation44 Comparison between cytotoxicity responses against cells transfected with FOXO3-specific or control siRNA was tested using a one-sided unpaired Student's t-test. In all analyses, a P < 0.05 was considered to be significant.
Disclosure of Potential Conflicts of Interest
No potential conflicts of interest were disclosed.
Acknowledgment
We thank Andy Hurwitz, Stephanie Watkins and Matt Thompson for advice on FoxO3 and protein expression in DC. We thank Merete Jonassen, Tina Seremet and Kirsten Nikolajsen for excellent technical assistance. We thank Tobias Wirenfeldt Klausen for help with statistical analysis.
Funding
The study was supported by Herlev Hospital, Danish Cancer Society Danish Medical Research Council and Toyotafonden. The funders had no role in study design, data collection and analysis, decision to publish, or preparation of the manuscript.
References
- Nurieva RI, Liu X, Dong C. Molecular mechanisms of T-cell tolerance. Immunol Rev 2011; 241:133-44; PMID:21488895; http://dx.doi.org/10.1111/j.1600-065X.2011.01012.x
- Hurwitz AA, Watkins SK. Immune suppression in the tumor microenvironment: a role for dendritic cell-mediated tolerization of T cells. Cancer Immunol Immunother 2012; 61:289-93; PMID:22237887; http://dx.doi.org/10.1007/s00262-011-1181-5
- Zhu Z, Singh V, Watkins SK, Bronte V, Shoe JL, Feigenbaum L, Hurwitz AA. High-avidity T cells are preferentially tolerized in the tumor microenvironment. Cancer Res 2013; 73:595-604; PMID:23204239; http://dx.doi.org/10.1158/0008-5472.CAN-12-1123
- Muller AJ, DuHadaway JB, Chang MY, Ramalingam A, Sutanto-Ward E, Boulden J, Soler AP, Mandik-Nayak L, Gilmour SK, Prendergast GC. Non-hematopoietic expression of IDO is integrally required for inflammatory tumor promotion. Cancer Immunol Immunother 2010; 59:1655-63; PMID:20640572; http://dx.doi.org/10.1007/s00262-010-0891-4
- Pletinckx K, Dohler A, Pavlovic V, Lutz MB. Role of dendritic cell maturity/costimulation for generation, homeostasis, and suppressive activity of regulatory T cells. Front Immunol 2011; 2:1-16; PMID:22566792; http://dx.doi.org/10.3389/fimmu.2011.00039
- Watkins SK, Zhu Z, Riboldi E, Shafer-Weaver KA, Stagliano KE, Sklavos MM, Ambs S, Yagita H, Hurwitz AA. FOXO3 programs tumor-associated DCs to become tolerogenic in human and murine prostate cancer. J Clin Invest 2011; 121:1361-72; PMID:21436588; http://dx.doi.org/10.1172/JCI44325
- Watkins SK, Hurwitz AA. FOXO3: A master switch for regulating tolerance and immunity in dendritic cells. Oncoimmunology 2012; 1:252-4; PMID:22720261; http://dx.doi.org/10.4161/onci.1.2.18241
- Dejean AS, Beisner DR, Ch'en IL, Kerdiles YM, Babour A, Arden KC, Castrillon DH, DePinho RA, Hedrick SM. Transcription factor Foxo3 controls the magnitude of T cell immune responses by modulating the function of dendritic cells. Nat Immunol 2009; 10:504-13; PMID:19363483; http://dx.doi.org/10.1038/ni.1729
- Shafer-Weaver KA, Watkins SK, Anderson MJ, Draper LJ, Malyguine A, Alvord WG, Greenberg NM, Hurwitz AA. Immunity to murine prostatic tumors: continuous provision of T-cell help prevents CD8 T-cell tolerance and activates tumor-infiltrating dendritic cells. Cancer Res 2009; 69:6256-64; PMID:19622771; http://dx.doi.org/10.1158/0008-5472.CAN-08-4516
- Paik JH, Kollipara R, Chu G, Ji H, Xiao Y, Ding Z, Horner JW, Carrasco DR, Jiang S, Gilliland DG, et al. FoxOs are lineage-restricted redundant tumor suppressors and regulate endothelial cell homeostasis. Cell 2007; 128:309-23; PMID:17254969; http://dx.doi.org/10.1016/j.cell.2006.12.029
- Kops GJ, Dansen TB, Polderman PE, Saarloos I, Wirtz KW, Coffer PJ, Huang TT, Bos JL, Medema RH, Burgering BM. Forkhead transcription factor FOXO3a protects quiescent cells from oxidative stress. Nature 2002; 419:316-21; PMID:12239572; http://dx.doi.org/10.1038/nature01036
- Yu C, Zhang Z, Liao W, Zhao X, Liu L, Wu Y, Liu Z, Li Y, Zhong Y, Chen K, et al. The tumor-suppressor gene Nkx2.8 suppresses bladder cancer proliferation through upregulation of FOXO3a and inhibition of the MEK/ERK signaling pathway. Carcinogenesis 2012; 33:678-86; PMID:22223847; http://dx.doi.org/10.1093/carcin/bgr321
- Storz P, Doppler H, Copland JA, Simpson KJ, Toker A. FOXO3a promotes tumor cell invasion through the induction of matrix metalloproteinases. Mol Cell Biol 2009; 29:4906-17; PMID:19564415; http://dx.doi.org/10.1128/MCB.00077-09
- Hu MC, Lee DF, Xia W, Golfman LS, Ou-Yang F, Yang JY, Zou Y, Bao S, Hanada N, Saso H, et al. IkappaB kinase promotes tumorigenesis through inhibition of forkhead FOXO3a. Cell 2004; 117:225-37; PMID:15084260; http://dx.doi.org/10.1016/S0092-8674(04)00302-2
- Pamer E, Cresswell P. Mechanisms of MHC class I–restricted antigen processing. Annu Rev Immunol 1998; 16:323-58; PMID:9597133; http://dx.doi.org/10.1146/annurev.immunol.16.1.323
- Larsen SK, Munir S, Woetmann A, Froesig TM, Odum N, Svane IM, Becker JC, Andersen MH. Functional characterization of Foxp3-specific spontaneous immune responses. Leukemia 2013; 27:2332-40; PMID:23812418; http://dx.doi.org/10.1038/leu.2013.196
- Rammensee HG, Falk K, Roetzschke O. MHC molecules as peptide receptors. Curr Biol 1995; 5:35-44; PMID:7697344; http://dx.doi.org/10.1016/S0960-9822(95)00011-X
- Andersen MH, Pedersen LO, Becker JC, thor Straten P. Identification of a Cytotoxic T Lymphocyte Response to the Apoptose Inhibitor Protein Survivin in Cancer Patients. Cancer Res 2001; 61:869-72; PMID:11221872
- Scheibenbogen C, Sun Y, Keilholz U, Song M, Stevanovic S, Asemissen AM, Nagorsen D, Thiel E, Rammensee HG, Schadendorf D. Identification of known and novel immunogenic T-cell epitopes from tumor antigens recognized by peripheral blood T cells from patients responding to IL-2-based treatment. Int J Cancer 2002; 20; 98:409-14; PMID:11920592; http://dx.doi.org/10.1002/ijc.10205
- Herr W, Ranieri E, Gambotto A, Kierstead LS, Amoscato AA, Gesualdo L, Storkus WJ. Identification of naturally processed and HLA-presented Epstein-Barr virus peptides recognized by CD4(+) or CD8(+) T lymphocytes from human blood. Proc Natl Acad Sci U S A 1999; 96:12033-38; PMID:10518571; http://dx.doi.org/10.1073/pnas.96.21.12033
- Andersen MH, Fensterle J, Ugurel S, Reker S, Houben R, Guldberg P, Berger TG, Schadendorf D, Trefzer U, Bröcker EB, et al. Immunogenicity of constitutively active V599EBRaf. Cancer Res 2004; 64:5456-60; PMID:15289355; http://dx.doi.org/10.1158/0008-5472.CAN-04-0937
- Andersen MH, Svane IM, Kvistborg P, Nielsen OJ, Balslev E, Reker S, Becker JC, Straten PT. Immunogenicity of Bcl-2 in cancer patients. Blood 2005; 15:728-34; PMID:15367432; http://dx.doi.org/10.1182/blood-2004-07-2548
- Wenandy L, Sorensen RB, Sengelov L, Svane IM, Thor SP, Andersen MH. The immunogenicity of the hTERT540–548 peptide in cancer. Clin Cancer Res 2008; 14:4-7; PMID:18172245; http://dx.doi.org/10.1158/1078-0432.CCR-07-4590
- Sorensen RB, Berge-Hansen L, Junker N, Hansen CA, Hadrup SR, Schumacher TN, Svane IM, Becker JC, thor Straten P, Andersen MH. The immune system strikes back: cellular immune responses against indoleamine 2,3-dioxygenase. PLoS One 2009; 4:e6910; PMID:19738905; http://dx.doi.org/10.1371/journal.pone.0006910
- Betts MR, Brenchley JM, Price DA, De Rosa SC, Douek DC, Roederer M, Koup RA. Sensitive and viable identification of antigen-specific CD8+ T cells by a flow cytometric assay for degranulation. J Immunol Methods 2003; 281:65-78; PMID:14580882; http://dx.doi.org/10.1016/S0022-1759(03)00265-5
- Birkenkamp KU, Essafi A, van der Vos KE, da CM, Hui RC, Holstege F, Koenderman L, Lam EW, Coffer PJ. FOXO3a induces differentiation of Bcr-Abl-transformed cells through transcriptional down-regulation of Id1. J Biol Chem 2007; 282:2211-20; PMID:17132628; http://dx.doi.org/10.1074/jbc.M606669200
- Munir S, Andersen GH, Met O, Donia M, Frosig TM, Larsen SK, Klausen TW, Svane IM, Andersen MH. HLA-restricted cytotoxic T cells that are specific for the immune checkpoint ligand PD-L1 occur with high frequency in cancer patients. Cancer Research 2013; 73:1674-776; PMID:23328583; http://dx.doi.org/10.1158/0008-5472.CAN-12-3507
- Yin L, Huang L, Kufe D. MUC1 oncoprotein activates the FOXO3a transcription factor in a survival response to oxidative stress. J Biol Chem 2004; 279:45721-7; PMID:15322085; http://dx.doi.org/10.1074/jbc.M408027200
- Chen J, Gomes AR, Monteiro LJ, Wong SY, Wu LH, Ng TT, Karadedou CT, Millour J, Ip YC, Cheung YN, et al. Constitutively nuclear FOXO3a localization predicts poor survival and promotes Akt phosphorylation in breast cancer. PLoS One 2010; 20:e12293; PMID:20808831; http://dx.doi.org/10.1371/journal.pone.0012293
- Qi W, Weber CR, Wasland K, Roy H, Wali R, Joshi S, Savkovic SD. Tumor suppressor FOXO3 mediates signals from the EGF receptor to regulate proliferation of colonic cells. Am J Physiol Gastrointest Liver Physiol 2011; 300:G264-72; PMID:21109589; http://dx.doi.org/10.1152/ajpgi.00416.2010
- Keilholz U, Weber J, Finke JH, Gabrilovich DI, Kast WM, Disis ML, Kirkwood JM, Scheibenbogen C, Schlom J, Maino VC, et al. Immunologic monitoring of cancer vaccine therapy: results of a workshop sponsored by the Society for Biological Therapy. J Immunother 2002; 25:97-138; PMID:12074049; http://dx.doi.org/10.1097/00002371-200203000-00001
- Lee JC, Espeli M, Anderson CA, Linterman MA, Pocock JM, Williams NJ, Roberts R, Viatte S, Fu B, Peshu N, et al. Human SNP links differential outcomes in inflammatory and infectious disease to a FOXO3-regulated pathway. Cell 2013; 155:57-69; PMID:24035192; http://dx.doi.org/10.1016/j.cell.2013.08.034
- Gnjatic S, Atanackovic D, Matsuo M, Jager E, Lee SY, Valmori D, Chen YT, Ritter G, Knuth A, Old LJ. Cross-presentation of HLA class I epitopes from exogenous NY-ESO-1 polypeptides by nonprofessional APCs. J Immunol 2003; 170:1191-96; PMID:12538675; http://dx.doi.org/10.4049/jimmunol.170.3.1191
- Nair S, Boczkowski D, Fassnacht M, Pisetsky D, Gilboa E. Vaccination against the forkhead family transcription factor Foxp3 enhances tumor immunity. Cancer Res 2007; 67:371-80; PMID:17210720; http://dx.doi.org/10.1158/0008-5472.CAN-06-2903
- Sorensen RB, Hadrup SR, Svane IM, Hjortso MC, thor Straten P, Andersen MH. Indoleamine 2,3-dioxygenase specific, cytotoxic T cells as immune regulators. Blood 2011; 117:2200-10; PMID:21079151; http://dx.doi.org/10.1182/blood-2010-06-288498
- Sorensen RB, Kollgaard T, Andersen RS, van den Berg JH, Svane IM, thor Straten P, Andersen MH. Spontaneous cytotoxic T-Cell reactivity against indoleamine 2,3-dioxygenase-2. Cancer Res 2011; 71:2038-44; PMID:21406395; http://dx.doi.org/10.1158/0008-5472.CAN-10-3403
- Munir S, Andersen GH, Woetmann A, Odum N, Becker JC, Andersen MH. Cutaneous T cell lymphoma cells are targets for immune checkpoint ligand PD-L1-specific, cytotoxic T cells. Leukemia 2013; 27:2251-53; PMID:23660624; http://dx.doi.org/10.1038/leu.2013.118
- Denning DP, Hatch V, Horvitz HR. Programmed elimination of cells by caspase-independent cell extrusion in C. elegans. Nature 2012; 488:226-30; PMID:22801495; http://dx.doi.org/10.1038/nature11240
- Feig C, Peter ME. How apoptosis got the immune system in shape. Eur J Immunol 2007; 37:61-70; PMID:17972347; http://dx.doi.org/10.1002/eji.200737462
- Maiese K, Hou J, Chong ZZ, Shang YC. A fork in the path: developing therapeutic inroads with FoxO proteins. Oxid Med Cell Longev 2009; 2:119-29; PMID:20592766; http://dx.doi.org/10.4161/oxim.2.3.8916
- Lappas M, Lim R, Riley C, Menon R, Permezel M. Expression and localisation of FoxO3 and FoxO4 in human placenta and fetal membranes. Placenta 2010; 31:1043-50; PMID:20934750; http://dx.doi.org/10.1016/j.placenta.2010.09.009
- Snoeks L, Weber CR, Wasland K, Turner JR, Vainder C, Qi W, Savkovic SD. Tumor suppressor FOXO3 participates in the regulation of intestinal inflammation. Lab Invest 2009; 89:1053-62; PMID:19636295; http://dx.doi.org/10.1038/labinvest.2009.66
- McCutcheon M, Wehner N, Wensky A, Kushner M, Doan S, Hsiao L, Calabresi P, Ha T, Tran TV, Tate KM, et al. A sensitive ELISPOT assay to detect low-frequency human T lymphocytes. J Immunol Methods 1997; 210:149-66; PMID:9520298; http://dx.doi.org/10.1016/S0022-1759(97)00182-8
- Moodie Z, Price L, Janetzki S, Britten CM. Response determination criteria for ELISPOT: toward a standard that can be applied across laboratories. Methods Mol Biol 2012; 792:185-96; PMID:21956511; http://dx.doi.org/10.1007/978-1-61779-325-7_15
- Toebes M, Coccoris M, Bins A, Rodenko B, Gomez R, Nieuwkoop NJ, van de Kasteele W, Rimmelzwaan GF, Haanen JB, Ovaa H, et al. Design and use of conditional MHC class I ligands. Nat Med 2006; 12:246-51; PMID:16462803; http://dx.doi.org/10.1038/nm1360
- Andersen MH, Bonfill JE, Neisig A, Arsequell G, Sondergaard I, Valencia G, Neefjes J, Zeuthen J, Elliott T, Haurum JS. Phosphorylated peptides can be transported by TAP molecules, presented by class I MHC molecules, and recognized by phosphopeptide-specific CTL. J Immunol 1999; 163:3812-8; PMID:10490979
- Pawelec G, Marsh SG. ESTDAB: a collection of immunologically characterised melanoma cell lines and searchable databank. Cancer Immunol Immunother 2006; 55:623-7; PMID:16421722; http://dx.doi.org/10.1007/s00262-005-0117-3
- Berke Z, Andersen MH, Pedersen M, Fugger L, Zeuthen J, Haurum JS. Peptides spanning the junctional region of both the abl/bcr and the bcr/abl fusion proteins bind common HLA class I molecules. Leukemia 2000; 14:419-26; PMID:10720136; http://dx.doi.org/10.1038/sj.leu.2401703
- Met O, Balslev E, Flyger H, Svane IM. High immunogenic potential of p53 mRNA-transfected dendritic cells in patients with primary breast cancer. Breast Cancer Res Treat 2011; 125:395-406; PMID:20336365; http://dx.doi.org/10.1007/s10549-010-0844-9