Abstract
Clinical studies investigating the impact of natural killer (NK) cells in allogeneic hematopoietic stem cell transplantation settings have yielded promising results. However, NK cells are a functionally and phenotypically heterogeneous population. Therefore, we addressed the functional relevance of specific NK cell subsets distinguished by expression of CD117, CD27 and CD11b surface markers in graft-versus-leukemia (GVL)-reaction and graft-versus-host-disease (GVHD). Our results clearly demonstrate that the subset of c-Kit−CD27−CD11b+ NK cells expressed multiple cytotoxic pathway genes and provided optimal graft-versus-leukemia-effects, while significantly reducing T cell proliferation induced by allogeneic dendritic cells. Furthermore, these NK cells migrated to inflamed intestinal tissues where graft-versus-host-colitis was efficiently mitigated. For the first time, we identified the c-Kit−CD27−CD11b+ NK cell population as the specific effector NK cell subset capable of significantly diminishing GVHD in fully mismatched bone marrow transplantation settings. In conclusion, the subset of c-Kit−CD27−CD11b+ NK cells not only supports GVL, but also plays a unique role in the protection against GVHD by migrating to the peripheral GVHD target organs where they exert efficient immunoregulatory activities. These new insights demonstrate the importance of selecting the optimal NK cell subset for cellular immunotherapy following allogeneic hematopoietic stem cell transplantation.
Abbreviations:
- BMT, bone marrow transplantation
- c-Kit+ NK = c-Kit+CD27+CD11b− NK cells
- CD27+ NK = c-Kit−CD27+CD11b− NK cells
- CD11b+ NK = c-Kit−CD27−CD11b+ NK cells
- DP = c-Kit−CD27+CD11b+ NK cells
- GVHD, graft-versus-host disease
- GVL, graft-versus-leukemia
- HSCT, hematopoietic stem cell transplantation
- KIR, killer cell immunoglobulin-like receptor
- MLR, mixed lymphocyte reaction
- NK, natural killer
- TBI, total body irradiation
Introduction
Natural killer (NK) cells are known to play important roles in innate and adaptive immunity. Previous preclinical and clinical studies have shown that NK cells may efficiently reduce or prevent graft-versus-host-disease (GVHD) without any adverse impact on their important graft-versus-leukemia (GVL) effect.Citation1 Murine studies demonstrated that allogeneic, interleukin 2 (IL-2) stimulated NK cells protect from GVHD in models of allogeneic as well as haploidentical bone marrow transplantation (BMT).Citation1-3 In addition, adoptively transferred NK cells have been shown to provide an important antitumor effect.Citation2 In humans, killer cell immunoglobulin-like receptor (KIR) ligand mismatch in haploidentical BMT in GVH direction reduced the risk for GVHDCitation1, Citation4 and adoptive cell therapies using expanded NK cells have been established for the treatment of various malignancies and for leukemia relapse.Citation5
NK cells are a heterogeneous population that can be classified into phenotypically and functionally distinct NK cell subsets, but the pathophysiological relevance of these NK cell subsets in the context of GVL and GVHD is so far unknown. It is well established that murine NK cells can be subdivided according to the expression of the cell surface molecules CD27 and CD11b.Citation6,7 In addition, the expression of CD117, also called c-Kit, a transmembrane receptor tyrosine kinase, provides a critical signal for survival, expansion, and maturation of NK cells.Citation8-10 We previously reported an immunoregulatory role for the subpopulation of IL-18 induced c-Kit expressing NK cells during tumor progression and autoimmune disease.Citation9, 10
In this study, we aimed to investigate which of the above subsets exerts the immunoregulatory effects described in the context of GVL and GVHD. Our data in murine models revealed that the subset of c-Kit−CD27−CD11b+ NK cells specifically mediates the highest anti-leukemic activities and the most potent GVHD amelioration via its migratory ability and efficient suppression of T-cell proliferation.
Results
Adoptive transfer of c-Kit−CD27−CD11b+ NK cells impairs GVHD progression and severity of GVHD colitis
To address the impact of different NK cell subsets on acute GVHD, we used a murine model for complete MHC-mismatched bone marrow transplantation (BMT). Balb/c (H-2d) mice were lethally irradiated followed by BMT from MHC-mismatched allogeneic donors (C57Bl/6, H-2b). Additional, allogeneic T cells were given to induce acute GVHD (). Based on our previous findings that c-Kit+ NK cells are capable of mediating diverse immunoregulatory functions in cancer and autoimmune disease,Citation9,10 we first addressed the possible impact of c-Kit expressing NK cells on GVHD. To this end, we administered either naïve cell-sorted or IL-18 induced c-Kit-expressing donor NK cells 1 d prior to the adoptive transfer of alloreactive T cells into recipient mice. Following treatment, various clinicopathological features were assessed every 2-3 days, including survival, body weight, and clinical GVHD score criteria, such as diarrhea, hunched posture, loss of activity, fur texture, and skin inflammation.Citation11 Unexpectedly, mice receiving freshly sorted (data not shown) or IL-18 induced c-Kit+CD27+CD11b− NK cells (called Kit+ NK) showed a slight worsening of GVHD ().
Figure 1. CD11b+ NK cells as main effectors reducing GVHD symptoms. (A) Murine allogeneic BMT model. One day after lethal total body irradiation (TBI), Balb/c mice were co-transplanted with 5×106 freshly isolated T-cell depleted bone marrow (BM) cells plus 1×106 MoFlo-sorted NK subsets from C57Bl/6 donors. Two days after TBI, 7×105 T cells from C57Bl/6 origin were injected i.v. to induce acute graft-versus host disease (GVHD). The control bone marrow transplant (BMT) group did not receive any additional T cells. (B) Course of GVHD score is depicted that has been assessed by clinical monitoring every 2-3 days following transfer of BM (black, n = 4) with additional transfer of allogeneic T cells to induce GVHD (red, n = 4) plus IL-18-induced Kit+CD27+CD11b− natural killer (NK) cells (Kit+, violet curve, n = 4). (C) Course of GVHD-score comparing a BMT control group (BM, black, n = 6) with mice developing GVHD induced by allogeneic T cells (BM+T, red, n = 5) and mice that additionally received either Kit−CD27+CD11b− (CD27+, blue, n = 4) or Kit−CD27−CD11b+ NK cells (CD11b+, green curve, n = 5). All NK cell subsets were expanded by 1,500 U/mL IL-2 for 5 days as described in Methods. (D) Survival of the same cohorts as described above. In the above panels, (B-D) one representative experiment out of 3 is depicted showing, the mean (4-6 mice/group) ± SD. Statistical analysis was performed by Student's t-test; p<0.05 CD11b+NK with GVHD group at day 28; ns for all other groups. (E) Colonoscopy on day 14 of mice from the BMT control, GVHD group, and mice that additionally received IL-2 expanded CD27+ or CD11b+ NK cell subsets. Two representative photographs are shown for each group with n = 2-5 mice/group.
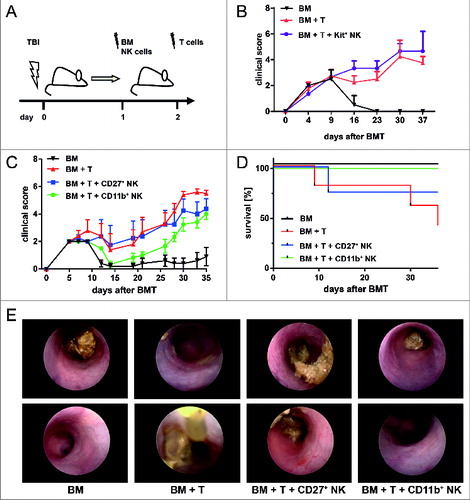
We next investigated the specific influence of distinct IL-2 expanded NK cell subsets on GVHD. We compared the effect of IL-2 expanded c-Kit−CD27+CD11b− (hereinafter referred to as CD27+) and c-Kit−CD27−CD11b+ (hereinafter referred to as CD11b+) NK cells on GVHD development (). The sorting strategy and purity of these NK cell subsets is shown in Figure S1. Our data reveal that transfer of IL-2 expanded CD11b+ NK cells delayed onset and reduced severity of acute GVHD in comparison to untreated GVHD controls or mice receiving IL-2 expanded CD27+ NK cells, displaying a significantly reduced GVHD score as compared to the control group receiving BM and alloreactive T cells (BM+T; *p<0.05 on day 28) (). Remarkably, animals in the group receiving IL-2 expanded CD11b+ NK cells also showed a significantly improved survival compared to the untreated GVHD control group (**p = 0.0068) and survived during the whole experimental period ().
Since one of the primary symptoms of GVHD is the occurrence of persistent and vast diarrhea, we performed colonoscopy by use of a mini-endoscope and observed the development of a severe GVHD colitis with macroscopic changes including thickening of the colon, granularity of the mucosal surface, visible fibrin, and change of the vascular pattern (). Of note, mice treated with IL-2 expanded CD11b+ NK cells, but not with IL-2 expanded CD27+ NK cells, showed a milder form of colitis () in accordance with the reduced clinical GVHD symptoms ().
CD11b+ NK cell infusion preserves GVL
Following our observation that IL-2 expanded CD11b+ NK cells were the only NK cell subset that reduced acute GVHD, we aimed to exclude a possible negative impact on GVL effects. Therefore, we monitored tumor load of Balb/c mice that received Balb/c-derived luciferase-expressing (luc+) BCL1 leukemia cells prior to allogeneic BMT and GVHD induction. Mice in the BMT control group that received T cell-depleted bone marrow (BM) succumbed to leukemia following day 17 (top of ) as shown by bioluminescence imaging (BLI) of the luc+ BCL1 leukemia cells. In contrast, all mice additionally receiving alloreactive T cells (BM + T), a portion of which further received IL-2 expanded CD27+ or CD11b+ NK cells (as defined above), were protected from leukemia by a strong GVL effect ().
Figure 2. CD11b+ NK cells have no negative impact on GVL. (A-C) Bioluminescence imaging (BLI) of Balb/c bearing luc+ BCL1 leukemia. Animals received T cell-depleted bone marrow (BM) +/- allogeneic T cells +/- defined natural killer (NK) cell subsets. (A) Impact of graft-versus-host disease (GVHD)-inducing T cells on GVL (BM + T). (B) Influence of additional treatment with IL-2 expanded CD27+ or CD11b+ NK cells on leukemia growth. In the above panels (A and B) days after bone marrow transplant (BMT) and BCL1 injection are indicated along the top of the panels and 3 representative animals per group are depicted over time. (C) Average photons emitted from luc+ BCL1 cells observed from ventral or lateral positioned imaging; n = 3 animals per group; Error bars represent SD.
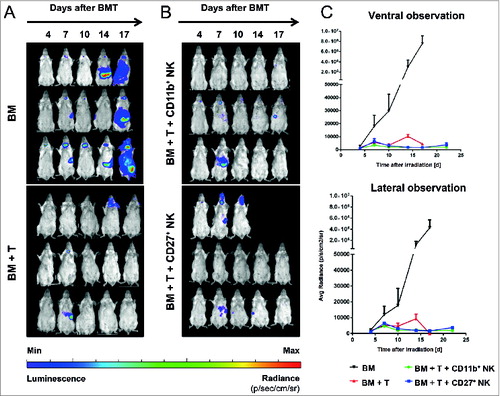
In mice that received allogeneic BMT and were treated with alloreactive T cells +/- the subset of IL-2 expanded CD27+ NK cells, we also observed severe GVHD in addition to the GVL effects. In contrast and of particular importance, mice treated with IL-2 expanded CD11b+ NK cells showed efficient GVL response () accompanied by a significantly reduced GVHD and improved survival.
Unique gene profile of specific NK cell subsets predestines their antitumor and migratory capacity
To determine whether the favorable effect of the CD11b+ NK cells in GVL and GVHD is predicted by specific genomic properties, we performed microarray analysis of the four major NK cell subsets that can be phenotypically distinguished by expression of the surface markers c-Kit, CD27 and CD11b (). We applied a flow cytometric gating strategy and cell sorting to isolate the different NK cell subpopulations based on previous work by ourselves and others.Citation6, Citation9, Citation12 Microarray analysis revealed that these selected NK cell subsets can be characterized by significantly distinct gene expression patterns (). In line with our functional results in GVL and GVHD, the murine NK population can be mainly classified into 2 major subsets expressing either CD27 or CD11b as shown by hierarchical clustering (). Expression of the genes related to the surface molecules c-Kit, CD27 and CD11b (Itgam) was used as quality control of the NK cell subset isolation (). Further gene ontology (GO) analysis indicated important functional differences between NK cell subsets regarding their cytotoxic potential and the expression of chemokine receptors (). Expression of a broad panel of these genes has been confirmed at the protein level ( and data not shown). Mean fluorescence intensity (MFI) of chemokine receptors CCR7, CXCR3, and CCR2 on the surface of the different NK cell subsets correspond to their gene expression data, and CCR9 showed an even higher MFI on the CD11b+ NK cell subset (). Interestingly, chemokine (C-C) motif receptor 9 (CCR9) is known to play an important role in lymphocyte trafficking to the intestine where its ligand chemokine (C-C) motif ligand 25 (CCL25) is strongly expressed by the intestinal epithelium.Citation13,14
Figure 3. Gene profiling of murine NK subsets. Transcriptome analysis of phenotypically distinct subsets of natural killer (NK) cells in mice. (A) Gating strategy of C57Bl/6 splenocytes for MoFlo sorting of NK cell subsets. Viable single NK1.1+ and CD3−/CD19− cells were distinguished based on expression of c-Kit, CD27 and CD11b. (B) Gene profiling of the four pre-dominant NK cell subsets. Unsupervised hierarchical clustering (i.e., Pearson centered, average linkage) of significantly regulated genes (at least 2-fold different from the median of all samples) of MoFlo sorted NK cell subpopulations from 2-3 independent experiments. Relative microarray expression levels are represented by a pseudocolor scale, as indicated. (C) Gene clustering of mean values of genes for the characteristic expression of NK surface molecules c-Kit, CD27 and CD11b (Itgam). (D-E) Genes were grouped according to their gene ontology. Gene clustering of mean values from 2-3 independent experiments of genes involved in cell-mediated cytotoxicity (D) and chemokine receptor expression (E). (F) Cytofluorimetric analysis of expression of different chemokine receptors performed on enriched NK cell subsets isolated from C57Bl/6 splenocytes. Mean ± SD of CCR7, CXCR3, CCR9 and CCR2 on Kit+CD27+ (black), CD27+ (red), Kit−CD27+CD11b+ (DP; blue) and CD11b+ (green) NK cells are indicated. Histograms depict the mean fluorescence index (MFI) of expression levels on NK cell subsets from 3 experiments. Error bars represent SD. Statistical significance was determined by Kruskal-Wallis test; *p<0.05; **p<0.01; ****p<0.0001.
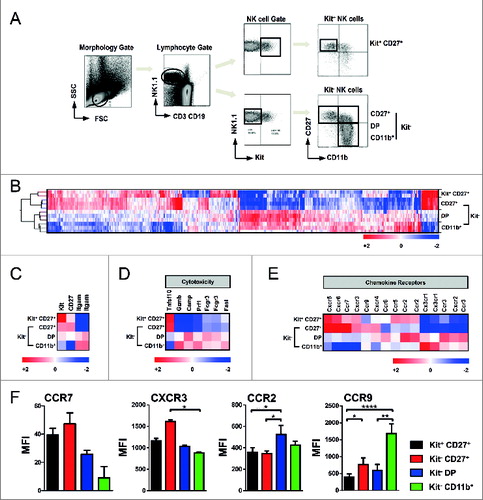
In sum, microarray analysis revealed important differences in gene expression patterns between the CD27 or CD11b expressing NK cell subsets pointing to specific functional properties concerning their cytotoxic potential and migratory behavior.
Adoptive transfer of congenic NK cells shows tissue specific migration of each NK subset
Due to the intrinsic differences in the chemokine receptor repertoire () we next sought to investigate whether the four NK cell subsets might vary in their migratory profile. In order to address this question, we first analyzed the homing of highly purified NK cells from congenic mice transferred in the setting of BMT. As illustrated in , when CD45.1+ bulk NK cells isolated from splenocytes were transferred together with CD45.2+ bone marrow cells into sublethally irradiated CD45.2+ recipient mice, the subset of Kit+ NK cells migrated to the bone marrow (BM), whereas CD11b+ NK cells preferentially settled in peripheral organs such as spleen, blood, lung and intestinal tract (, Fig. S2) comparable to the natural distribution of NK cell subsets in naïve mice (Fig. S3).
Figure 4. Migration capacity of specific NK cell subsets. (A) Scheme of murine transplantation model to study migration capacity. One day after sublethal total body irradiation (TBI), mice received 2×106 freshly isolated bone marrow (BM) and congenic CD45.1+ NK subsets. Purified bulk NK cells (B), MoFlo-sorted Kit+CD27+ (C), Kit−CD27+ (D), Kit−CD27+CD11b+ (DP) (E) or CD11b+ (F) NK cell subsets show different behaviors in their migration. Bone marrow, spleen, blood and lung of C57Bl/6 mice were analyzed in week 1 and 2 after transplantation by fluorescence cytometry. Kit− CD45.1+ NK are indicated by red, Kit+ cells by black dots. One representative experiment out of 3 is depicted with percentages of either Kit+ (black) and Kit− (red) indicated in the plots.
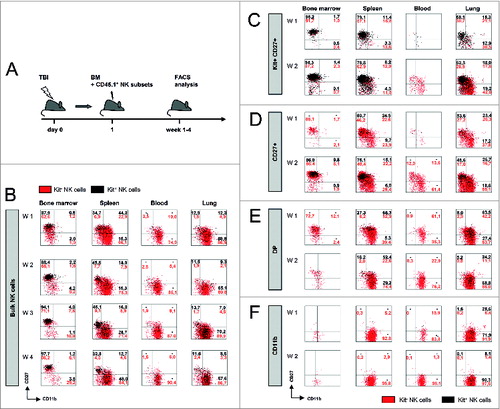
Furthermore, upon adoptive transfer of the four cell-sorted NK subsets from congenic mice based on their phenotypes as described above, we were able to monitor their distinct migratory behavior following BMT. Remarkably, transferred Kit+ NK cells, which are generally defined as immature NK cells, preferentially homed to the BM and only scarcely to spleen and lung (). Further analysis of the absolute numbers of NK cell subsets (in addition to the percentage) in the different tissues revealed that Kit+ NK cells showed proliferation, but almost no differentiation into mature NK cells specifically in the BM ( and Fig. S2A). These observations indicate that BM stroma did not support NK cell maturation. In line with this observation, BM of naïve mice (before irradiation) also harbored very few CD11b+ NK cells (Fig. S4A). Additionally, cytofluorimetric analysis following total body irradiation (TBI) and transfer of congenic BM allowed monitoring of the relative and absolute numbers of all the different NK cell subsets that repopulated the BM (Fig. S4B). On day 18 after BMT, recipient mice displayed similar NK cell numbers and subset distribution as naïve animals.
In peripheral organs, Kit−CD27+ as well as DP NK cells acquired CD11b expression during the first 2 weeks following BMT (). In contrast, adoptively transferred mature CD11b+ NK cell subsets rarely re-entered the BM, irreparably lost Kit-expression, and were ubiquitously detected in the various peripheral organs (). In additional BLI experiments specifically performed with the CD11b+ NK subset isolated from Luciferase-expressing transgenic mice (B6-L2G85.CD45.1), we confirmed the migration of the CD11b+ NK cells to the GVHD target organs (Fig. S2D and E).
In sum, CD11b+ NK cells possess the ability to traffic to peripheral organs that might be targets of GVHD. Remarkably, in mice developing GVHD following allogeneic BMT plus T cell transfer, a failure in immune reconstitution with significantly reduced numbers of NK cells, specifically mature CD11b+ NK cells, could be observed (Fig. S5). This observation highlights that adoptive transfer of this specific NK subset might diminish clinically manifested GVHD symptoms.
CD11b+ NK cells are highly cytotoxic effector cells that mediate GVL
As described above, the adoptive transfer of IL-2 expanded CD11b+ NK cells delayed the onset and reduced the severity of acute GVHD, but preserved GVL ( and ). As the GVL reaction is known to be mainly mediated by alloreactive T cells, we further aimed to investigate the direct cytotoxic capacity of the CD11b+ NK cell subset on malignant cells. To this end, we first investigated the cytotoxic capacity against syngeneic B16F10 melanoma cells as a classical murine NK cell target (). In vitro killing assays clearly demonstrated that CD11b+ NK cells possessed significant cytotoxicity and were able to eliminate 60% of B16F10 cells at an effector-to-target (E:T) cell ratio of 5:1. In contrast, CD27+ NK cells were only capable of killing 25% and c-Kit+ less than 20% of the tumor cells at the same E:T cell ratio (). Additionally, we performed time lapse video-microscopy to directly observe the killing of B16 tumor cells by the IL-2 expanded NK subsets over 12 h and were impressed by the velocity with which CD11b+ NK cells could induce tumor cell death ( and unpublished data). These observations were in line with the expression of multiple genes involved in cytotoxic pathways that has been shown for the CD11b+ NK cells (). We furthermore aimed to address the antitumor capacity of specifically the IL-2 expanded CD11b+ NK cells against the allogeneic luc+ BCL1 leukemia cell line. As BCL1 leukemia cells do not grow in vitro, we performed the cytotoxicity assays directly in vivo in the setting of allogeneic BMT, but transferred only 1×106 CD11b+ NK cells without alloreactive T cells. As shown in , the BLI signal was reduced in mice treated with IL-2 expanded CD11b+ NK cells demonstrating their anti-leukemic capacity in vivo ().
Figure 5. High cytotoxicity and GVL effect mediated by CD11b+ NK cells. (A) Tumor lysis capacity of freshly MoFlo sorted natural killer (NK) cell subsets from C57Bl/6 splenocytes determined against B16F10 melanoma (from C57Bl/6) at different effector-to-target (E:T) cell ratios with addition of IL-2, as described in Methods. After 24 h, specific tumor cell lysis was assessed by crystal violet assay. The graph shows 1 representative out of at least 3 independent experiments measured in triplicates. Error bars represent SD; Statistical analysis was performed by Student's t-test compared lysis induced by CD11b+ vs Kit+ NK at all E:T ratios; p<0.005. (B) Photographs from microscopy illustrating the tumor lytic effect induced by either IL-2 expanded CD27+ or CD11b+ NK cells on B16F10 tumor cells following 24 h of co-incubation. One representative photograph per condition was selected out of 3 independent experiments with similar results. (C) Luminescence imaging to assess the anti-leukemic capacity of CD11b+ NK cells in vivo. Balb/c mice were lethally irradiated at day 0 and received luc+ BCL1 cells, allogeneic T cell-depleted bone marrow (BM) +/- IL-2 expanded CD11b+ NK cells (BM + CD11b+ NK), as described for the graft-versus-host disease (GVHD) and graft versus leukemia (GVL) setting. Days after BMT and BCL1 injection are indicated along the top of the panels and 3 representative animals per group are depicted over time. (D) Average photons emitted from luc+ BCL1 cells observed from ventral or lateral positioned imaging; n = 3 animals per group; error bars represent SD.
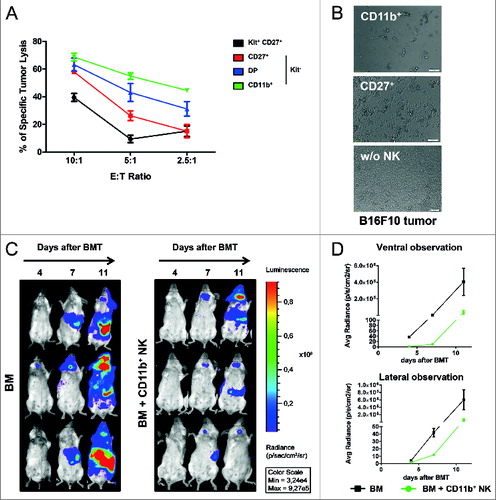
CD11b+ NK cells induce lysis of allogeneic DCs thereby suppressing GVHD induction
Next, we asked if the mechanism by which NK cells inhibit GVHD,Citation15 could also be attributed to the cytotoxic capacity of the subset of CD11b+ NK cells. To solve this question, we performed cytotoxicity and mixed lymphocyte reaction (MLR) experiments ().
Figure 6. CD11b+ NK cells efficiently suppress T-cell proliferation by elimination of allogeneic DCs. (A and B) Mixed lymphocyte reaction (MLR) addressing the impact of CD27+ or CD11b+ NK subsets on carboxyfluorescein succinimidyl ester (CFSE) labeled T cells from C57Bl/6 stimulated by dendritic cells (DCs) from Balb/c donors. CFSE dilution was measured by fluorescence cytometry. (B) Bone marrow derived DCs were additionally stimulated over night by lipopolysaccharide (LPS) before the MLR was initiated. (C) Same MLR setting as in (A) but with separation of NK subsets from direct T cell and DC contact using transwell-plates. (D and E) Killing capacity by either CD27+ or CD11b+ NK cells determined by cytofluorimetric analysis of analysis of Annexin V and the % DC viability calculated by the ratio of Annexin/7AAD. (E) Same setting as (D) but additional 24 h LPS stimulation of DCs. (A-E) NK:DC ratio was 1:1. Statistical significance was determined by Kruskal-Wallis test; *p<0.05; **p<0.01.
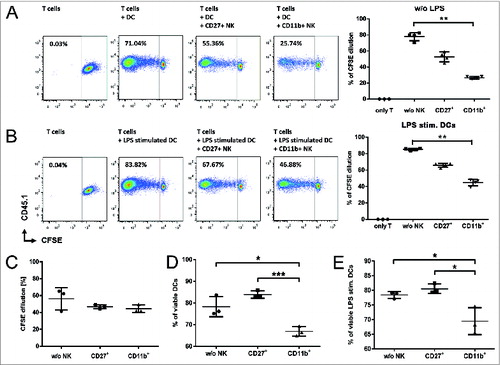
Indeed, donor CD11b+ NK cells showed a significantly higher reduction of T-cell proliferation compared to CD27+ NK cells in MLR performed with MoFlo sorted, IL-2 expanded donor NK cell subsets and CFSE labelled donor T cells stimulated by allogeneic DCs (). Importantly, T-cell suppression was induced even when the allogeneic DCs have been lipopolysaccharide (LPS) activated to mimic the inflammatory conditions at the onset of GVHD (). In our experiments, T-cell inhibition depended on direct cell-cell interaction as it could be completely abolished in MLR settings using transwells to separate NK cells from both T cells and DCs (). While we observed only a residual cytotoxicity against alloreactive T cells mediated by both CD27+ and CD11b+ NK cells (data not shown), we found that the IL-2 expanded CD11b+ NK subset, but not CD27+ NK cells, efficiently induced apoptosis in allogeneic DCs () even following additional LPS stimulation ().
In summary, our functional testing revealed that the CD11b+ NK cell subset represents not only the NK fraction with the highest antitumor and GVL effect, but also mediates an important suppression of alloreactive T cells. The impact on T-cell proliferation seemed partially dependent on the NK cytotoxicity against activated T cells, but was mainly attributable to the elimination of allogeneic DCs.
With this work, we succeeded to identify CD11b+ NK cells as the main effector NK subset mediating the best improvement of GVHD by specific migration capacity, high cytotoxic potential and inhibition of DC induced alloreactive T-cell proliferation under inflammatory conditions. Importantly, our data also demonstrate that the CD11b+ NK cells support GVL effects by their high antitumor efficiency.
Discussion
It has recently been published in a murine model of allogeneic BMT that IL-2 expanded NK cells can diminish GVHD symptoms without any negative impact on GVL in a murine model of allogeneic GVHD.Citation3, Citation15 Our study demonstrates for the first time that it is specifically the subset of CD11b+ NK cells that mediates these important effects on GVL and GVHD.
NK cells are a heterogeneous population that can be divided into functionally distinct subsets based on the expression of CD27, CD11b and also CD117/c-Kit.Citation6-8 It has been suggested that the different NK subsets correspond to distinct differentiation steps from initially CD27+ NK cells acquiring CD11b as a marker of mature NK cells.Citation6, Citation12 Recently, we and other groups reported immunosuppressive functions of naïve as well as IL-18 induced c-Kit+CD27+CD11b− NK cells that were mediated by down-modulation of the innate and adaptive immune system in tumor models and autoimmune disease.Citation8-10, Citation16
With this background, we sought to determine the pathophysiological relevance of the above NK subsets in GVHD and GVL ( and ). We transferred highly purified c-Kit+ (), or IL-2 expanded CD27+ versus CD11b+ NK cells prior to GVHD induction (). Interestingly, we observed a worsening of GVHD in mice receiving c-Kit-expressing NK cells. This result can be explained by the ability of c-Kit+ NK cells to suppress the innate arm of immunity by lysis of conventional c-Kit− NK cells.Citation9 In line with that, our work highlights that the c-Kit− cytotoxic CD11b+ NK cell subset is one of the driving factors specifically maintaining the right balance between efficient GVL effects without adverse GVHD. Of note, while freshly sorted NK cell subsets lack GVHD-reducing effects in our experiments (unpublished data), IL-2 expanded CD11b+ NK cells led to a significant reduction of GVHD (). Specifically, the morphological signs of GVHD colitis were impressively reduced as shown by colonoscopy (). The reduction of the clinical GVHD symptoms was limited to the early phase of GVHD (until d 30 post BMT), nevertheless all animals treated with CD11b+ NK survived during the whole period of the experiment (). Of note, while the adoptive transfer of CD11b+ NK cells delayed the onset and reduced the severity of acute GVHD, we observed support of the GVL reaction as assessed by in vivo BLI experiments addressing the expansion of luc+ BCL1 leukemic cells in the setting of allogeneic BMT with or without induction of GVHD ( and ).
The overall modulation of GVHD by the subset of CD11b+ NK cells corresponds well to the effects obtained by the whole NK cell pool following IL-2 stimulation as reported by Olson et al.Citation3 However, there remained a strong need to develop a deeper understanding of the effectiveness of the various NK cell subpopulations in treating GVHD.
Multiple reasons might explain the exclusive impact of the CD11b+ subset in GVHD prevention as compared to the other NK subsets. To gain further insights, we performed microarray and functional characterization of the distinct NK cell subsets. As it has been suggested in previous studies,Citation6 our functional data confirmed that CD11b+ NK cells are excellent tumor killers in vitro and in vivo (), a result consistent with the expression of multiple genes involved in cytotoxic pathways ().
Another important aspect might be the ability of the CD11b+ NK cells to settle to peripheral organs and to inflamed tissues such as the intestine. In contrast, CD27+ NK, especially the c-Kit-expressing subset, homed preferentially to the bone marrow ( and S2). This observation might explain one possible reason why c-Kit+ NK cells were not able to mediate protection against GVHD (). In accord, gene array and flow cytometry analysis revealed a higher expression of CCR9 on CD11b+ NK cells, a chemokine receptor known to mediate lymphocyte trafficking to the intestinal tract.Citation13,14, Citation17 In addition, we aimed to further investigate the superiority of the CD11b+ NK subset in the pathophysiological mechanism of GVHD induction. Our in vitro results indicate that CD11b+ NK cells mediate suppression of DC induced T-cell proliferation (). In line with previous studies reporting the NK induced killing of alloreactive T cells in Fas/FasL and NKG2D dependent manner,Citation18 our experiments also revealed the lysis of activated T cells, but only at lower levels and without significant differences between the NK subsets (not shown). In our experimental setting, the main mechanism distinguishing the impact of the different NK subpopulations was the cytotoxic capacity of the CD11b+ subset directed against allogeneic bone marrow derived DCs, even when inflammatory conditions were mimicked by additional LPS stimulation inducing DC maturation (). In summary, the specific subset of CD11b+ NK cells provides the best migratory capability to peripheral GVHD target organs, most efficient inhibition of DC mediated T-cell proliferation and protection against acute GVHD concurrently with GVL support. Further studies should address whether CD11b+ NK cell might even dampen ongoing GVHD reaction rather than to protect from GVHD onset.
In humans, patients with acute myeloid leukemia (AML) that underwent haploidentical hematopoietic stem cell transplantation (HSCT) showed improved event-free survival and reduced GVHD in case of killer cell immunoglobulin-like receptor (KIR) ligand mismatch in GVH direction.Citation1, Citation4 Since then, however, some subsequent studies also reported contrasting results.Citation19,20 Furthermore, clinical trials have been performed with adoptive transfer of expanded donor NK cells for different malignancies and for relapse either after immunosuppression or in the setting of haploidentical HSCT.Citation5,21-27 All studies have evinced the overall safety of NK cell therapy, typically eliciting partial or short-term remission; however, only a few studies have demonstrated significant clinical benefit.Citation21,27 Difficulties in obtaining uniformly successful control of GVL and GVHD by NK cells can be accounted for by major differences in clinical settings and study outlines, but possibly also by intrinsic heterogeneity in NK cell preparations and the individual composition of functionally different NK cell subsets in the administered cell products.
Regarding the development of NK cell therapeutics, our work highlights the importance of selecting the optimal NK cell subset able to reach the organ of interest and improve the cytotoxic function of NK cells in donor lymphocyte infusions. Therefore, our results are of high relevance for further optimization of NK cell therapy in HSCT. Interestingly, immunomonitoring analysis of mice developing GVHD showed significantly reduced numbers of mature CD11b+ NK cells, thereby underlining a possible impact of GVHD on the immune reconstitution and development of NK cells (Fig. S5). It is already known that mature, KIR-expressing CD56dimCD16high NK cells, as the putative counterpart of the murine CD11b+ NK subset, require longer time intervals relative to CD56highCD16bright NK cells to recover after HSCT.Citation28,29 Additionally, in regards to the possible clinical benefit of cytotoxic effector NK cells on GVHD prevention demonstrated herein, future studies are needed to address possible correlations between the lack of NK cell maturation and GVHD development, as well as the impact of NK cells on immune reconstitution after transplantation of hematopoietic stem cells.
Materials and Methods
Animals
Balb/c, Ly5.2 (CD45.2+) and Ly5.1 (CD45.1+) C57Bl/6 mice were obtained from Elevage Janvier (Le Genest St. Isle, France) and used at 7–9 weeks of age. Luciferase-expressing (luc+) B6-L2G85.CD45.1 (H-2b) mice were kindly provided by A. Beilhack.Citation30 Animal experiments were approved by the government of Mittelfranken and Hessen, Germany.
Bone marrow transplantation and adoptive transfer of NK cell subsets
For autologous BMT, C57Bl/6 WT mice were sublethally irradiated with 5 Gy and received 2×106 BM cells from WT +/− 5×105 CD45.1+ NK cells. Isolation of CD45.1+ NK cell subsets from splenocytes has been performed by use of the NK Cell Isolation Kit II (Miltenyi, Bergisch Gladbach, Germany) followed by cell sorting on a MoFlo Legacy (Beckman Coulter, Brea, CA, USA) according to expression of c-Kit (CD117), CD27 and CD11b.Citation31 NK cell subsets showed purity >95%. C-Kit+ NK cells were either MoFlo sorted from splenocytes of C57Bl/6 or in vitro induced by IL-18, as recently described.Citation9 All other sorted NK cell subsets were ex vivo expanded with 1,500 U/mL IL-2 for 5 days.Citation18 Throughout the whole manuscript the specific NK cell subsets were defined as follows: c-Kit+ NK = c-Kit+CD27+CD11b− NK cells; CD27+ NK = c-Kit−CD27+CD11b− NK cells; CD11b+ NK = c-Kit−CD27−CD11b+ NK cells; DP = c-Kit−CD27+CD11b+ NK cells.
GVHD and GVL mouse model
Balb/c mice were lethally irradiated with 8 Gy. On day 1, Balb/c received 5×106 BM cells +/− 1×106 NK cells derived from allogeneic C57Bl/6. On day 2, 7×105 MACS purified T cells from spleen of C57Bl/6 (Pan T Cell Isolation Kit II, Miltenyi, Bergisch Gladbach, Germany) were adoptively transferred. For GVL experiments, 5×105 luciferase+ BCL1 leukemia cells derived from Balb/c mice were injected i.v. on day 0.
Bioluminescence imaging (BLI)
In brief, 10 min after i.p. injection of 1.5 mg luciferin in 100μl PBS, mice were imaged with an IVIS Lumina II and analyzed using Living Image 2.5 (Caliper Life Science, PerkinElmer, USA).Citation32
Murine colonoscopy
Endoscopy was performed with use of a mini-endoscope as previously described.Citation33
Flow cytometry
Flow cytometry was performed on a FACS Canto II (BD Biosciences, Heidelberg, Germany) and analysed using FlowJo Software (Treestar, Ashland, Oregon, USA). Detailed information on the methods and antibodies used is given in the online supplementary information.
Microarray analysis
Microarray analysis was performed using whole mouse genome expression arrays (4×44 K) on an Agilent scanner as described in the online supplementary information. Enrichment of GO terms was calculated using DAVID tools.Citation34
Cytotoxicity in vitro analysis
Crystal violet assay has been previously describedCitation31 and is briefly described in online supplementary information.
Mixed lymphocyte reaction (MLR)
Bone marrow derived dendritic cells (DCs) from Balb/c (H-2d) were generated in vitro as previously described.Citation35 Information on the MLR is given in the supplementary information.
Statistical analysis
Statistical analysis was performed as indicated in the figure legends by use of Graphpad Prism Software (CA, USA).
Disclosure of Potential Conflicts of Interest
No potential conflicts of interest were disclosed.
Supplemental Material
Supplemental data for this article can be accessed on the publisher's website.
981483_Supplementary_Materials.zip
Download Zip (13.1 MB)Acknowledgments
The authors would like to thank A. Abendroth, J. Schneider, K. Schmitt for technical support, M. Klug and L. Schwarzfischer-Pfeilschifter for assistance in performance of microarray experiments, and U. Appelt for MoFlo cell sorting. We are grateful to A. Cerwenka, P. Hoffmann, and L. Zitvogel for helpful discussions.
Funding
Authors were supported by the ELAN program (EU, DD, IA) and the IZKF program (EU) of the University Erlangen, by the German Cancer Aid (Max Eder Nachwuchsgruppe, Deutsche Krebshilfe, EU) and by the LOEWE Center for Cell and Gene Therapy (EU, FG, MAR), Frankfurt, funded by the Hessian Ministry of Higher Education, Research and the Arts, Germany (III L 4- 518/17.004). DD is a fellow of the ‘Förderkolleg’ of the Bavarian Academy of Sciences and was funded by BayGene and the German Research Foundation (DU548/2-1).
References
- Ruggeri L, Capanni M, Urbani E, Perruccio K, Shlomchik WD, Tosti A, et al. Effectiveness of donor natural killer cell alloreactivity in mismatched hematopoietic transplants. Science 2002; 295:2097-100.
- Asai O, Longo DL, Tian ZG, Hornung RL, Taub DD, Ruscetti FW, et al. Suppression of graft-versus-host disease and amplification of graft-versus-tumor effects by activated natural killer cells after allogeneic bone marrow transplantation. J Clin Invest 1998; 101:1835-42.
- Olson JA, Leveson-Gower DB, Gill S, Baker J, Beilhack A, Negrin RS. NK cells mediate reduction of GVHD by inhibiting activated, alloreactive T cells while retaining GVT effects. Blood 115:4293-301.
- Giebel S, Locatelli F, Lamparelli T, Velardi A, Davies S, Frumento G, et al. Survival advantage with KIR ligand incompatibility in hematopoietic stem cell transplantation from unrelated donors. Blood 2003; 102:814-9.
- Miller JS, Soignier Y, Panoskaltsis-Mortari A, McNearney SA, Yun GH, Fautsch SK, et al. Successful adoptive transfer and in vivo expansion of human haploidentical NK cells in patients with cancer. Blood 2005; 105:3051-7.
- Chiossone L, Chaix J, Fuseri N, Roth C, Vivier E, Walzer T. Maturation of mouse NK cells is a 4-stage developmental program. Blood 2009; 113:5488-96.
- Hayakawa Y, Smyth MJ. CD27 dissects mature NK cells into two subsets with distinct responsiveness and migratory capacity. J Immunol 2006; 176:1517-24.
- Colucci F, Di Santo JP. The receptor tyrosine kinase c-kit provides a critical signal for survival, expansion, and maturation of mouse natural killer cells. Blood 2000; 95:984-91.
- Terme M, Ullrich E, Aymeric L, Meinhardt K, Coudert JD, Desbois M, et al. Cancer-induced immunosuppression: IL-18-elicited immunoablative NK cells. Cancer Res 2012; 72:2757-67.
- Ehlers M, Papewalis C, Stenzel W, Jacobs B, Meyer KL, Deenen R, et al. Immunoregulatory natural killer cells suppress autoimmunity by down-regulating antigen-specific CD8+ T cells in mice. Endocrinology 2012; 153:4367-79.
- Cooke KR, Kobzik L, Martin TR, Brewer J, Delmonte J, Jr., Crawford JM, et al. An experimental model of idiopathic pneumonia syndrome after bone marrow transplantation: I. The roles of minor H antigens and endotoxin. Blood 1996; 88:3230-9.
- Hayakawa Y, Huntington ND, Nutt SL, Smyth MJ. Functional subsets of mouse natural killer cells. Immunol Rev 2006; 214:47-55.
- Wurbel MA, Malissen M, Guy-Grand D, Malissen B, Campbell JJ. Impaired accumulation of antigen-specific CD8 lymphocytes in chemokine CCL25-deficient intestinal epithelium and lamina propria. Journal of immunology 2007; 178:7598-606.
- Papadakis KA, Prehn J, Nelson V, Cheng L, Binder SW, Ponath PD, et al. The role of thymus-expressed chemokine and its receptor CCR9 on lymphocytes in the regional specialization of the mucosal immune system. Journal of immunology 2000; 165:5069-76.
- Olson JA, Zeiser R, Beilhack A, Goldman JJ, Negrin RS. Tissue-specific homing and expansion of donor NK cells in allogeneic bone marrow transplantation. Journal of immunology 2009; 183:3219-28.
- Ebata K, Shimizu Y, Nakayama Y, Minemura M, Murakami J, Kato T, et al. Immature NK cells suppress dendritic cell functions during the development of leukemia in a mouse model. Journal of immunology 2006; 176:4113-24.
- Hart AL, Ng SC, Mann E, Al-Hassi HO, Bernardo D, Knight SC. Homing of immune cells: role in homeostasis and intestinal inflammation. Inflamm Bowel Dis 2010; 16:1969-77.
- Olson JA, Leveson-Gower DB, Gill S, Baker J, Beilhack A, Negrin RS. NK cells mediate reduction of GVHD by inhibiting activated, alloreactive T cells while retaining GVT effects. Blood 2010; 115:4293-301.
- Davies SM, Ruggieri L, DeFor T, Wagner JE, Weisdorf DJ, Miller JS, et al. Evaluation of KIR ligand incompatibility in mismatched unrelated donor hematopoietic transplants. Killer immunoglobulin-like receptor. Blood 2002; 100:3825-7.
- Farag SS, Bacigalupo A, Eapen M, Hurley C, Dupont B, Caligiuri MA, et al. The effect of KIR ligand incompatibility on the outcome of unrelated donor transplantation: a report from the center for international blood and marrow transplant research, the European blood and marrow transplant registry, and the Dutch registry. Biology of blood and marrow transplantation : journal of the American Society for Blood and Marrow Transplantation 2006; 12:876-84.
- Rubnitz JE, Inaba H, Ribeiro RC, Pounds S, Rooney B, Bell T, et al. NKAML: a pilot study to determine the safety and feasibility of haploidentical natural killer cell transplantation in childhood acute myeloid leukemia. Journal of clinical oncology : official journal of the American Society of Clinical Oncology 2010; 28:955-9.
- Curti A, Ruggeri L, D’Addio A, Bontadini A, Dan E, Motta MR, et al. Successful transfer of alloreactive haploidentical KIR ligand-mismatched natural killer cells after infusion in elderly high risk acute myeloid leukemia patients. Blood 2011; 118:3273-9.
- Geller MA, Cooley S, Judson PL, Ghebre R, Carson LF, Argenta PA, et al. A phase II study of allogeneic natural killer cell therapy to treat patients with recurrent ovarian and breast cancer. Cytotherapy 2011; 13:98-107.
- Koehl U, Sorensen J, Esser R, Zimmermann S, Gruttner HP, Tonn T, et al. IL-2 activated NK cell immunotherapy of three children after haploidentical stem cell transplantation. Blood cells, molecules & diseases 2004; 33:261-6.
- Passweg JR, Tichelli A, Meyer-Monard S, Heim D, Stern M, Kuhne T, et al. Purified donor NK-lymphocyte infusion to consolidate engraftment after haploidentical stem cell transplantation. Leukemia 2004; 18:1835-8.
- Nguyen S, Beziat V, Norol F, Uzunov M, Trebeden-Negre H, Azar N, et al. Infusion of allogeneic natural killer cells in a patient with acute myeloid leukemia in relapse after haploidentical hematopoietic stem cell transplantation. Transfusion 2011; 51:1769-78.
- Stern M, Passweg JR, Meyer-Monard S, Esser R, Tonn T, Soerensen J, et al. Pre-emptive immunotherapy with purified natural killer cells after haploidentical SCT: a prospective phase II study in two centers. Bone marrow transplantation 2013; 48:433-8.
- Bjorkstrom NK, Riese P, Heuts F, Andersson S, Fauriat C, Ivarsson MA, et al. Expression patterns of NKG2A, KIR, and CD57 define a process of CD56dim NK-cell differentiation uncoupled from NK-cell education. Blood 2010; 116:3853-64.
- Bosch M, Dhadda M, Hoegh-Petersen M, Liu Y, Hagel LM, Podgorny P, et al. Immune reconstitution after anti-thymocyte globulin-conditioned hematopoietic cell transplantation. Cytotherapy 2012; 14:1258-75.
- Beilhack A, Schulz S, Baker J, Beilhack GF, Wieland CB, Herman EI, et al. In vivo analyses of early events in acute graft-versus-host disease reveal sequential infiltration of T-cell subsets. Blood 2005; 106:1113-22.
- Meinhardt K, Kroeger I, Abendroth A, Muller S, Mackensen A, Ullrich E. Influence of NK cell magnetic bead isolation methods on phenotype and function of murine NK cells. Journal of immunological methods 2012; 378:1-10.
- Waldner MJ, Wirtz S, Jefremow A, Warntjen M, Neufert C, Atreya R, et al. VEGF receptor signaling links inflammation and tumorigenesis in colitis-associated cancer. The Journal of experimental medicine 2010; 207:2855-68.
- Becker C, Fantini MC, Neurath MF. High resolution colonoscopy in live mice. Nature protocols 2006; 1:2900-4.
- Huang da W, Sherman BT, Lempicki RA. Systematic and integrative analysis of large gene lists using DAVID bioinformatics resources. Nature protocols 2009; 4:44-57.
- Lutz MB, Kukutsch N, Ogilvie AL, Rossner S, Koch F, Romani N, et al. An advanced culture method for generating large quantities of highly pure dendritic cells from mouse bone marrow. Journal of immunological methods 1999; 223:77-92.