Abstract
Na-APR-1M74 is an aspartic protease that is rendered enzymatically inactive by site-directed mutagenesis and is a candidate antigen component in the Human Hookworm Vaccine. The mutant protease exerts vaccine efficacy by inducing antibodies that neutralize the enzymatic activity of wild type enzyme (Na-APR-1wt) in the gut of the hookworm, thereby depriving the worm of its ability to digest its blood meal. Previously, canines immunized with Na-APR-1M74 and challenged with Ancylostoma caninum were partially protected against hookworm challenge infection, especially from the loss in hemoglobin observed in control canines and canine immunoglobulin (Ig) G raised against Na-APR-1 was shown to inhibit the enzymatic activity of Na-APR-1wt in vitro, thereby providing proof of concept of Na-APR-1M74 as a vaccine antigen. The mutated version, Na-APR-1M74, was then expressed at the cGMP level using a Nicotiana benthamiana expression system (Fraunhofer, CMB, Delaware, MD), formulated with Alhydrogel®, and used to immunize mice in a dose-ranging study to explore the enzyme-neutralizing capacity of the resulting anti- Na-APR-1M74 IgG. As little as 0.99 μg of recombinant Na-APR-1M74 could induce anti Na-APR-1M74 IgG in mice that were capable of inhibiting Na-APR-1wt-mediated digestion of a peptide substrate by 89%. In the absence of enzymatic activity of Na-APR-1M74 as a surrogate marker of protein functionality, we developed an assay based on the binding of a quenched fluorescence-labeled inhibitor of aspartic proteases, BODIPY-FL pepstatin A (BDP). Binding of BDP in the active site of Na-APR-1wt was demonstrated by inhibition of enzymatic activity, and competitive binding with unlabelled pepstatin A. BDP also bound to Na-APR-1M74 which was assessed by fluorescence polarization, but with an ∼50-fold reduction in the dissociation constant. Taken together, these assays comprise a “toolbox” that could be useful for the analyses of Na-APR-1M74 as it proceeds through the clinical development as part of the Human Hookworm Vaccine pipeline.
Introduction
Hookworms infect over 400 million people worldwide,Citation1 predominantly in resource-poor rural and tropical regions, and is one of the most debilitating of the neglected tropical diseases (NTDs). In terms of disability-adjusted life years (DALYs) lost, hookworm ranks higher than schistosomiasis and leishmaniasis as the second most important parasitic disease after malaria.Citation2-4 Chronic infection with hookworm results in long-term pathological consequences primarily due to ongoing intestinal blood loss caused by the feeding activities of these hematophagous parasites. This can result in iron-deficiency anemia (IDA) and the subsequent impairment of the physical (e.g. stunting) and intellectual development of infected children, which can have significant consequences on future economic productivity.Citation5 In many low and middle income countries (LMIC), hookworm infection remains the leading cause of anemia.Citation6 Moreover, where there is extensive geographic overlap between hookworm and malaria infections, especially in in Sub-Saharan Africa, and co-infection with these 2 parasites can have an additive effect on the severity of IDA.Citation7 Hookworm infection can be treated effectively with chemotherapy; indeed, cure rates after single-dose treatments can reach 92%,Citation8 but rapid reinfection necessitates regular mass drug administration (MDA),Citation9 which is difficult to sustain in many hookworm endemic LMIC countries. High rates of drug failure with both albendazole and mebendazole have also been observed.Citation10 This potential lack of efficacy, coupled with the widespread distribution of the infection and the potential emergence of drug resistance Citation11 suggests that additional control measures are urgently needed.
To address this imperative, the Human Hookworm Vaccine is under development by the Product Development Partnership (PDP) of the Sabin Vaccine InstituteCitation5 to target Necator americanus, the hookworm species responsible for 3-quarters of all human infection.Citation12 The vaccine would have 2 recombinant antigen components N. americanus antigens (Na-GST-1—a glutathione-S-transferase, and Na-APR-1—an aspartic protease), both of which would be formulated on Alhydrogel®. Currently, each antigen component is undergoing independent phase I testing in healthy, adult volunteers in the USA and Brazil. In the future, the 2 antigen components will be co-formulated as a single monovalent vaccine and tested in hookworm-endemic populations of Brazil and sub-Saharan Africa to assess their efficacy in reducing moderate and heavy hookworm infections and the diminish the resultant blood loss and IDA.Citation13,14
Na-APR-1 is a major digestive aspartic protease of adult hookworms and is expressed in the parasite gut epithelium, where it is the first molecule to catabolize hemoglobin (Hb) that is released from lysed red blood cells after the worm takes a blood meal from the host intestinal wall.Citation15 Na-APR-1 is an efficacious vaccine antigen, as demonstrated by its ability to induce protection in laboratory animals (reviewed inCitation5). Furthermore, high levels of IgG (but not the potentially deleterious IgE) against Na-APR-1 in hookworm-infected people implies that vaccination of hookworm-exposed individuals could be both safe and effective, augmenting an existing IgG response to wild type Na-APR-1 expressed by hookworms, while avoiding potentially harmful allergic responses due to pre-existing anti-Na-APR-1 IgE.Citation16
We have previously shown that IgG from laboratory animals immunized with Na-APR-1 can inhibit the enzymatic activity of the recombinant enzyme.Citation16-18 Not only does this provide a serum correlate of vaccine efficacy, it implies that the protective effect of Na-APR-1 is likely due to the generation of anti-enzyme antibodies which are ingested by the worm during a blood meal and consequently inhibit the hemoglobinolytic activity of parasite-derived Na-APR-1 in the hookworm gut and block complete digestion of the blood meal. For reasons of safety, pertaining to the applicability of immunizing humans with a catalytically active enzyme, the active site aspartic acid residues of Na-APR-1 (Asp-97 and Asp-284) were mutated to alanines, rendering the enzyme proteolytically inactive (Na-APR-1M74), but still capable of adopting a fold comparable to that of the wild type enzyme (evidenced by its binding to pepstatin A—a specific inhibitor of aspartic proteases) and generating a neutralizing antibody response.Citation16 In order to monitor enzyme function through process development, we have developed a method to measure the binding of Na-APR-1M74 to BODIPY FL-pepstatin A (BDP) by fluorescence polarization, a technique that detects changes in optical polarization that occur when a fluorophore-labeled molecule becomes rotationally constrained by binding to a protein.Citation19,20 We also demonstrate the neutralizing properties of anti-Na-APR-1M74 antibodies (induced by vaccination with Na-APR-1M74) by showing inhibition of the enzymatic activity of Na-APR-1wt against a synthetic fluorescent substrate. Finally, we report the absence of serologic cross-reactivity between Na-APR-1M74 and its human homolog, cathepsin D. Taken together, these data provide evidence that Na-APR-1M74 could be used to safely and effectively vaccinate human subjects against hookworm disease.
Results
Na-APR-1M74 is immunogenic and does not cross-react with human cathepsin D
All dosages of Na-APR-1M74 were well-tolerated and immunogenic in all mouse groups tested, with average antibody titers for groups receiving adjuvanted formulations ranging from 9,057 GMU (lowest dose group—0.99 μg) to 266,202 GMU (highest dose group—50 μg). Groups G19 and G20, to which non-adjuvanted formulations were administered, displayed modest antibody titers. The serum pool showing the highest anti-Na-APR-1M74 IgG titer upon exsanguination at day 42 (G11—266,202 GMU) () was used to probe purified human cathepsin D. No detectable immunologic cross-reactivity between Na-APR-1M74 and human cathepsin D was evident by ELISA ().
Table 1. Antigen formulation administered to and titer and neutralizing capacity of IgG purified from pooled sera of each group
Anti-Na-APR-1M74 IgG inhibits Na-APR-1wt activity in vitro
In all 9 mouse IgG pools tested in the peptide substrate neutralization assay, 10 μg of polyclonal IgG inhibited enzyme activity by at least 90% after 60 min, and a 20-fold excess (5.0 μg) of antibody neutralized substrate cleavage by at least 75% in all but 2 groups, relative to equal amounts of negative control IgG. Group G18 was administered the lowest dose of Na-APR-1M74 (0.99 μg), and 10.0 μg of pooled IgG from this group neutralized Na-APR-1wt enzymatic activity by 89% after 60 min. Groups G19 and G20 received Na-APR-1M74 without adjuvant, and despite generating low anti-Na-APR-1M74 titers, 10.0 μg of pooled IgG from these groups inhibited enzymatic activity of Na-APR-1wt by 89% and 100%, respectively ( and ). Of the groups tested for anti-hemoglobinase activity (4 of the 9 groups were not tested due to insufficient antibody being available for the assay), all were capable of inhibiting Hb degradation at all time points assessed (up to 6 days), relative to equal amounts of negative control IgG. Products of Hb breakdown were observed up to 96 hours post-incubation with antibody and were most evident where Hb was incubated with normal mouse IgG (negative control) or in the absence of any IgG ().
Figure 2. Enzymatic activity of Na-APR-1 wt is inhibited by anti-Na-APR-1M74 IgG in vitro. (A) Na-APR-1 wt (37.5 nM) was incubated for 60 min at 37{degree sign}C with increasing amounts of different pools of IgG from mice vaccinated with Na-APR-1M74 in 50 mM sodium acetate (pH 5.5). Inhibition of activity was determined by a decrease in cleavage of the aspartic protease fluorogenic peptide substrate 7-methoxycoumarin-4-acetyl-GKPILFFRLK(DNP)-D-Arg-amide. G11 – G20 represent pooled, purified IgG from groups of 10 mice that were each vaccinated with one of a dilution series of Na-APR-1M74. "Negative" represents pooled, purified IgG from a group of 10 mice vaccinated with adjuvant only. (B) Na-APR-1 wt (37.5 nM) and 10 μg of human hemoglobin (Hb) were incubated for up to 144 hours at 37{degree sign}C with 5 μg of different pools of IgG from mice vaccinated with Na-APR-1M74 in 50 mM sodium acetate (pH 5.5). Inhibition of activity was determined by absence of Hb degradation as visualized by denaturing gel electrophoresis. Lane 1 = G11; lane 2 = G15; lane 3 = G18; lane 4 = G20; lane 5 = negative; lane 6 = no IgG; lane 7 = Hb only.
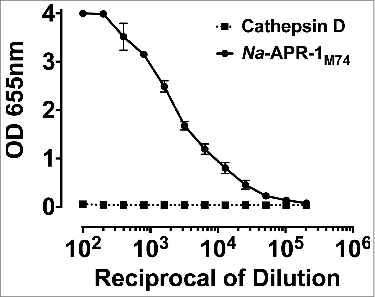
BODIPY FL-pepstatin A inhibits Na-APR-1wt enzymatic activity and competes with unlabelled pepstatin A for Na-APR-1wt binding
We have previously documented the inhibitory effect of pepstatin A on Na-APR-1wt enzymatic activity.Citation16 To assess whether BDP exhibited similar enzyme-inhibitory properties to unlabelled pepstatin A (and therefore would be suitable for use in the Na-APR-1M74 fluorescence polarization assay), a dilution series of BDP was incubated with Na-APR-1wt and the IC50 of BDP was determined to be 9.28 nM, which is comparable to the IC50 of BDP reported for human cathepsin D (10 nM)Citation25 (). The similar binding properties of BDP and pepstatin A were further investigated using a competitive binding assay where a dilution series of pepstatin A was incubated in presence of Na-APR-1wt and BDP. A decrease in mP (BDP binding) was observed with an increase in pepstatin A concentration (), indicating competitive and specific binding between the 2 inhibitor analogs.
Figure 3. BODIPY FL-pepstatin A inhibits Na-APR-1 wt activity and competes with unlabelled pepstatin A for Na-APR-1 wt binding. (A) Na-APR-1 wt (37.5 nM) was incubated for 120 min at 37{degree sign}C with increasing amounts of BDP in 50 mM sodium acetate (pH 3.5). Inhibition of Na-APR-1 wt activity was measured by a decrease in cleavage of the aspartic protease fluorogenic peptide substrate 7-methoxycoumarin-4-acetyl-GKPILFFRLK(DNP)-D-Arg-amide and the IC50 of BDP was determined as the concentration of BDP which inhibited Na-APR-1 wt activity by 50%. (B) Na-APR-1 wt (42 nM) was incubated at 37{degree sign}C with increasing amounts of pepstatin A in 50 mM sodium acetate (pH 3.5) and BDP was added to a final concentration of 4 nM. The fluorescence polarization change in BDP as a result of its binding to Na-APR-1 wt (expressed as mP units) is plotted as a function of pepstatin A concentration.
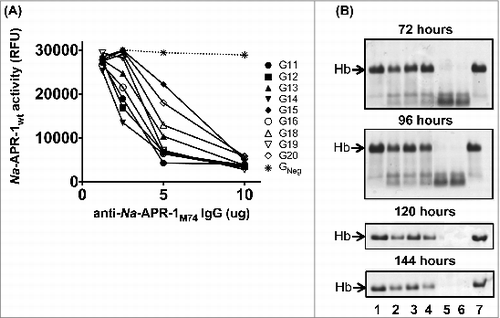
Na-APR-1wt-BDP binding can be measured by fluorescence polarization
The predicted structure of Na-APR-1M74 was modeled on the crystal structure of human cathepsin D docked with pepstatin A,Citation26 and was predicted to bind to the inhibitor. While pepstatin A made predicted contacts with the same amino acid side chains lining the active pocket of both Na-APR-1M74 and Na-APR-1wt, it was not predicted to form hydrogen bonds with the amino acid residues at positions 97 and 284 of Na-APR-1M74 due to their substitution from aspartic acids to alanines (). To assess the fluorescence polarization change of BDP induced by interaction with Na-APR-1, increasing amounts of Na-APR-1wt or Na-APR-1M74 were incubated with BDP. With both Na-APR-1wt and Na-APR-1M74, increases in mP value (BDP binding) were observed with increasing enzyme concentration, indicating binding of the inhibitor (). The dissociation constant (Kd) of BDP for Na-APR-1wt and Na-APR-1M74 was determined as 4 nM and 217 nM, respectively.
Figure 4. Assessing the binding interaction of Na-APR-1 and pepstatin A. Models of pepstatin A docked within the active site of (A) Na-APR-1 wt or (B) Na-APR-1M74 was modeled on human cathepsin D complexed with pepstatin A using MODELLER 23, 24. In each model, pepstatin A (blue) is shown interacting with amino acid side chains lining the binding pocket (magenta). Hydrogen bonds are represented as yellow dotted lines and, in (A), mediate the interaction of pepstatin A with the 2 active site aspartic acids (cyan). In (B), note the absence of hydrogen bond formation between pepstatin A and the cyan residues (red arrows), which have been mutated from aspartic acids to alanines. Figures were generated using the PyMOL Molecular Graphics System, Version 1.5.0.4 (Schrodinger, LLC). The interaction between (C) Na-APR-1 wt or (D) Na-APR-1M74 and BODIPY FL-pepstatin A (4 nM) was assessed by fluorescence polarization. Equal amounts of BSA were used as negative controls for each reaction and these "background" mP values have been subtracted. Each data point represents the average of 10 mp values calculated every minute for the first 10 min of each assay. Millipolarization values plateau in the presence of Na-APR-1wt amounts higher than 52 nM (A) and Na-APR-1M74 amounts higher than 2083 nM (B) and so were not included.
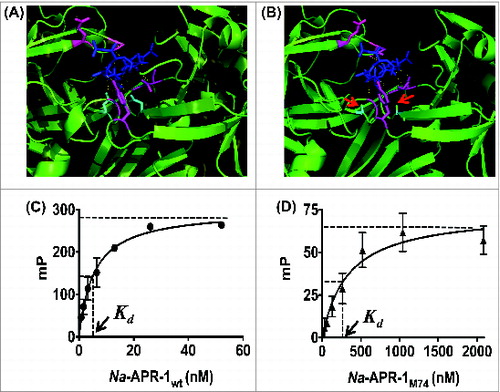
Discussion
Na-APR-1 is a major digestive aspartic protease of the human hookworm Necator americanus that initiates a semi-ordered catalytic cascade whereby host hemoglobin is degraded by a battery of mechanistically distinct enzymes absorbed by the gut epithelium in the process of nutrient acquisition.Citation15,27 Aspartic proteases are protective antigens against other parasites including Toxoplasma28 and blood-feeding schistosomes29 and ticks.30 Moreover, in the cases of Candida31 and poultry mite32 infections, passive transfer of anti-protease IgG significantly decreases the disease burden of challenged animals, highlighting the importance of anti-protease antibodies in protection. In a similar fashion, the recombinant Na-APR-1 enzyme confers protective immunity in animal challenge studies by likely inducing neutralizing IgG antibodies that inhibit the catalytic function of the parasite-derived molecule, compromising the worm's ability to adequately supply degraded hemoglobin during the blood meal.Citation16,17
This result led to the production of clinical grade Na-APR-1M74 and the current dose-ranging study in mice presented herein. In this study, there was no correlation between IgG pool titers and enzyme-inhibitory capacity, however, all IgG pools significantly neutralized Na-APR-1wt activity against a peptide substrate, regardless of titer. Moreover, inhibiting the degradation of Hb (the parasite's natural substrate and nutrient source) by anti-Na-APR-1M74 IgG simulates how this vaccine would exert its effects in vivo. Given that the enzyme-neutralizing capacity of anti-Na-APR-1M74 IgG is a likely correlate of vaccine efficacy, these results suggest that a significant protective effect could be achieved by administration of a low concentration of recombinant antigen, therefore contributing to cost-effective vaccine production and helping to minimize logistical issues associated with field delivery of an NTD vaccine in resource-poor nations where hookworm is endemic.
The human hookworm vaccine candidate Na-APR-1M74 is a Na-APR-1 vaccine that has been engineered for expression in the tobacco plant Nicotiana benthamiana.Citation13 The 2 active site aspartic acid residues have been substituted with alanines,Citation16 rendering the enzyme catalytically inactive due to the safety concerns associated with vaccination of humans with an active protease. An additional pre-requisite for safe vaccination is the absence of cross-reactivity between an vaccine antigen and a host homologs. There was no recognition by the human homolog to Na-APR-1 (cathepsin D) by IgG againstNa-APR-1M74, implying that anti-vaccine antibodies are unlikely to bind to the human protein.
However, mutating the active site aspartic acid residues of Na-APR-1 precluded the monitoring of the enzymatic activity Na-APR-1 as a quality control assay during product development of Na-APR-1M74. As such, we designed a binding assay using the aspartic protease inhibitor, pepstatin, A to assess protein functionality. It is known that aspartic proteases have to adopt correct structural folds in order to bind substrates or inhibitors such as pepstatin A in the active pocket due to the complex and intimate association between protease and inhibitor.Citation26,33 To detect and quantify the binding interactions between Na-APR-1M74 and pepstatin A, we employed the use of BDP (a fluorescent probe comprising pepstatin A conjugated to a BODIPY-FL fluorophoreCitation25) because of it's photostability and pH insensitivityCitation34 since assays were conducted in acidic buffers necessary for aspartic protease activity. BODIPY-FL pepstatin A exhibited selective, active site binding to Na-APR-1wt as evidenced by its ability to inhibit aspartic protease activity and compete with unlabelled pepstatin A for binding to the mutant protein, which is consistent with similar findings for human cathespin D.Citation25 We reasoned, therefore, that the BDP/Na-APR-1 interaction observed by fluorescence polarization was a specific binding event, indicating the docking of BDP within the active site of Na-APR-1wt and Na-APR-1M74. The ∼50-fold difference in Kd is likely explained by the ability of Na-APR-1M74 to bind BDP less efficiently due to the absence of contacts made by the catalytic aspartic acid residues as a result of active site mutations.
The commercial production of an active enzyme is monitored using a catalytic assay, whereby a batch would progress through development depending on its performance within acceptable limits of enzymatic activity. As with any conformationally-dependent interaction between enzyme and substrate, pepstatin A binding to Na-APR-1M74 requires that the protein adopts a similar folding as the wild type molecule and so an assay which evaluates this function of Na-APR-1M74, like the fluorescence polarization assay proposed here, could be used as quality control assay in product development of Na-APR-1M74 (lot to lot monitoring) and to monitor the stability of the vaccine over during clinical development.
A cornerstone of vaccine product development is the availability of quality control and functional assays that monitor the status of the vaccine during manufacture and then during clinical development. Often, these assays become important components in the licensure of the vaccine.Citation35 In this study, we have presented (1) a fluorescence polarization assay to monitor the lot to lot production of the human hookworm vaccine candidate Na-APR-1M74 and (2) an antibody inhibition assay to assess the functional capacity of anti-Na-APR-1M74 IgG induced by vaccination. Together, these assays comprise a “toolbox” for monitoring the Na-APR-1M74 vaccine antigen, which will undoubtedly aid in the development of a human vaccine to fight this debilitating NTD.
Methods
Ethics statement
All work involving experimental procedures with laboratory animals was approved by the Animal Research Ethics Committee of George Washington University Medical Center.
Antigen production
Na-APR-1M74 (lot# 1709) was recombinantly produced as a catalytic inactive enzyme using the tobacco plant Nicotiana benthamiana expression system in partnership with Fraunhofer CMB.Citation13 Na-APR-1wt was expressed in Pichia pastoris as previously described for Ac-APR-1, the APR-1 ortholog from the dog hookworm, Ancylostoma caninum.Citation21
Mouse immunizations, IgG purification and ELISA
Ten groups of 10 female BALB/c mice (designated G11—G20) were vaccinated in a intraperitoneally in prime-boost regimen on days 0 and 28, with serially diluted doses of Na-APR-1M74/Alhydrogel® (Brenntag Biosector 2%) (3% Al(OH)3) (). A group of 10 mice was similarly immunized with 400 μg of Alhydrogel® alone (Gneg) to serve as an adjuvant control. All mice were exsanguinated at 42 days prime immunization and the IgGs were purified from 1.0 ml of pooled sera from each group of mice using protein G sepharose (VWR). Bound IgGs were eluted with 1.0 ml of 0.1 M glycine, pH 2.7, equilibrated by the addition of 200 μl of 1 M Tris-HCl, pH 9, and concentrated to 100 μl with a Nanosep centrifugation devices (Pall). Levels of IgG against Na-APR-1M74 were determined by a qualified indirect ELISA and expressed as Arbitrary Units as described in detail elsewhere.Citation16,22 Antigen was coated onto microtiter plates at 1.0 μg/ml and serum pools were serially diluted (1:1,000 to 1:204,800). After addition of goat anti-mouse IgG-HRP (Jackson) (1:5000), peroxidase activity was detected with tetramethyl benzidine chromogenic substrate (Life Technologies) measured at 655 nm.
Cross-reactivity analysis
To assess for serologic cross-reactivity between Na-APR-1M74 and human cathepsin D (Sigma), each protein was coated onto microtiter plates at 1.0 μg/ml and probed with serial dilutions of anti-Na-APR-1M74 serum pool G11 (1:1,000 to 1:204,800) and titers were measured as described above.
Antibody inhibition assays
Na-APR-1wt (0.25 μg) was incubated with 1.0 μM of the fluorogenic aspartic protease peptide substrate 7-methoxycoumarin-4-acetyl-GKPILFFRLK(DNP)-D-Arg-amide (MoCAc-GKPILFFRLK) (Sigma) and pools of mouse anti-Na-APR-1M74 IgG (G11—G20) or pooled canine anti-Na-APR-1mut IgG in 50 mM sodium acetate, pH 5.5. Final reaction volume was 200 μl and assays were performed at 37°C for 60 min in a Polarstar Omega Microplate Reader (BMG Labtech) using excitation and emission wavelengths of 330 nm and 390 nm, respectively. Inhibition of Na-APR-1wt enzymatic activity was measured as a decrease in relative fluorescence compared to the signal generated from similar reactions containing IgG from mice vaccinated with adjuvant only. To measure the inhibition of hemoglobinase activity, 0.25 μg of Na-APR-1wt and 10 μg of human Hb were incubated in the presence of 5.0 μg of representative canine anti-Na-APR-1mut IgG or mouse anti-Na-APR-1M74 pools in 50 mM sodium acetate, pH 5.5 (final reaction volume = 200 μl). Reactions were carried out at 37°C and aliquots were taken every 24 h for a total of 144 h to visualize the extent of Hb degradation by SDS-PAGE. A negative control reaction containing an equal amount of Gneg IgG was also performed.
Inhibitory effect of BODIPY FL-pepstatin A on Na-APR-1wt enzymatic activity
Na-APR-1wt (37.5 nM) was incubated with a dilution series (400—0.391 nM) of BDP (Life Technologies) (200 nM in DMSO) in 50 mM sodium acetate, pH 3.5 for 15 minutes at room temperature before the addition of MoCAc-GKPILFFRLK (1.0 μM). Final reaction volume was 100 μl and assays were performed at 37°C for 120 min in a Polarstar Omega Microplate Reader using excitation and emission wavelengths of 330 nm and 390 nm, respectively. Fluorescence (Na-APR-1wt activity) was plotted as a function of inhibitor concentration and curve-fitted using the exponential function in Graphpad Prism. Results presented are the average of 3 experiments. The IC50 of BDP was determined as the concentration of BDP that inhibited Na-APR-1wt activity by 50%.
Competitive binding of pepstatin A and BODIPY FL-pepstatin A to Na-APR-1wt
A doubling dilution series of pepstatin A (8000—15 nM) was added to 50 mM sodium acetate, pH 3.5, in the wells of a black microtiter plate in the presence of Na-APR-1wt (42 nM). BDP was added to a final concentration of 4 nM. A well containing no enzyme served as a negative control. The final reaction volume was 300 μl and reactions were incubated for 50 min and then carried out at 37°C in a Polarstar Omega Microplate Reader. The polarized light in each sample was emitted at right angles to the exciting light (485 nm) and then passed through an emission filter (520 nm) and a vertical fixed polarizer. Vertical and horizontal light emissions were measured every minute for 10 min and polarization values were calculated from these using the Polarstar Omega Microplate Reader software. Values were averaged and expressed in millipolarization (mP) units and plotted logarithmically as a function of pepstatin A concentration using Graphpad Prism.
Binding of BODIPY FL-pepstatin A to Na-APR-1
The three-dimensional structures of Na-APR-1wt and Na-APR-1M74 were predicted from the structure of human cathepsin D [Protein Data Bank (PDB) code 1LYB] in complex with pepstatin A docked in the active site using the MODELLER application.Citation23,24 Alignments for the models were performed within MODELLER using the align function. Due to the relatively high sequence identity between the human cathespin D template and the query sequences (38% over 373 residues), no additional loop refinement was performed. The isovaleryl (IVA) and statine (STA) residues of pepstatin were incorporated into the model using block residues corresponding to the CHARMM type ‘undf’. Twenty models were calculated, and the model with the lowest Discrete Optimized Protein Energy (DOPE) score was selected. Figures were generated using the PyMOL Molecular Graphics System, Version 1.5.0.4 (Schrodinger, LLC). To assess the fluorescence polarization change of BDP induced by interaction with Na-APR-1wt, a doubling dilution series of Na-APR-1wt (208—0.8 nM) was added to 50 mM sodium acetate, pH 3.5, in the wells of a black microtiter plate, with the last well in the series containing no enzyme. BDP was added to a final concentration of 4 nM. The final reaction volume was 300 μl and reactions were carried out at 37°C in a Polarstar Omega Microplate Reader and mP values calculated as above. The same assay was used to assess the interaction between BDP and Na-APR-1M74 except that the dilution series for the recombinant protein was 4166—32 nM. Negative control assays containing equal amounts of BSA instead of Na-APR-1 were run in parallel. Values from negative control reactions were subtracted from corresponding enzyme-containing reactions and these “background-corrected” mP data sets were plotted and curve-fitted using the hyperbola function in Graphpad Prism.
Disclosure of Potential Conflicts of Interest
No potential conflicts of interest were disclosed.
Funding
This research was funded by the Sabin Vaccine Institute with a grant awarded by the Bill and Melinda Gates Foundation. MSP was supported by an NHMRC Early Career Fellowship. AL is an NHMRC Senior Principal Research Fellow.
References
- Pullan RL, Smith JL, Jasrasaria R, Brooker SJ. Global numbers of infection and disease burden of soil transmitted helminth infections in 2010. Parasit Vectors 2014; 7:37; PMID:24447578; http://dx.doi.org/10.1186/1756-3305-7-37
- Hotez P. A handful of 'antipoverty' vaccines exist for neglected diseases, but the world's poorest billion people need more. Health Aff (Millwood) 2011; 30:1080-7; PMID:21653960; http://dx.doi.org/10.1377/hlthaff.2011.0317
- Hotez PJ, Brooker S, Bethony JM, Bottazzi ME, Loukas A, Xiao S. Hookworm infection. N Engl J Med 2004; 351:799-807; PMID:15317893; http://dx.doi.org/10.1056/NEJMra032492
- Murray CJ, Vos T, Lozano R, Naghavi M, Flaxman AD, Michaud C, Ezzati M, Shibuya K, Salomon JA, Abdalla S, et al. Disability-adjusted life years (DALYs) for 291 diseases and injuries in 21 regions, 1990-2010: a systematic analysis for the Global Burden of Disease Study 2010. Lancet 2012; 380:2197-223; PMID:23245608; http://dx.doi.org/10.1016/S0140-6736(12)61689-4
- Hotez PJ, Bethony JM, Diemert DJ, Pearson M, Loukas A. Developing vaccines to combat hookworm infection and intestinal schistosomiasis. Nat Rev Microbiol 2010; 8:814-26; PMID:20948553; http://dx.doi.org/10.1038/nrmicro2438
- Kassebaum NJ, Jasrasaria R, Naghavi M, Wulf SK, Johns N, Lozano R, Regan M, Weatherall D, Chou DP, Eisele TP, et al. A systematic analysis of global anemia burden from 1990 to 2010. Blood 2014; 123:615-24; PMID:24297872; http://dx.doi.org/10.1182/blood-2013-06-508325
- Brooker S, Akhwale W, Pullan R, Estambale B, Clarke SE, Snow RW, Hotez PJ. Epidemiology of plasmodium-helminth co-infection in Africa: populations at risk, potential impact on anemia, and prospects for combining control. Am J Trop Med Hyg 2007; 77:88-98; PMID:18165479
- Vercruysse J, Behnke JM, Albonico M, Ame SM, Angebault C, Bethony JM, Engels D, Guillard B, Nguyen TV, Kang G, et al. Assessment of the anthelmintic efficacy of albendazole in school children in seven countries where soil-transmitted helminths are endemic. PLoS Negl Trop Dis 2011; 5:e948; PMID:21468309; http://dx.doi.org/10.1371/journal.pntd.0000948
- Albonico M, Smith PG, Ercole E, Hall A, Chwaya HM, Alawi KS, Savioli L. Rate of reinfection with intestinal nematodes after treatment of children with mebendazole or albendazole in a highly endemic area. Trans R Soc Trop Med Hyg 1995; 89:538-41; PMID:8560535; http://dx.doi.org/10.1016/0035-9203(95)90101-9
- Soukhathammavong PA, Sayasone S, Phongluxa K, Xayaseng V, Utzinger J, Vounatsou P, Hatz C, Akkhavong K, Keiser J, Odermatt P. Low efficacy of single-dose albendazole and mebendazole against hookworm and effect on concomitant helminth infection in Lao PDR. PLoS Negl Trop Dis 2012; 6:e1417; PMID:22235353; http://dx.doi.org/10.1371/journal.pntd.0001417
- Albonico M, Engels D, Savioli L. Monitoring drug efficacy and early detection of drug resistance in human soil-transmitted nematodes: a pressing public health agenda for helminth control. Int J Parasitol 2004; 34:1205-10; PMID:15491582; http://dx.doi.org/10.1016/j.ijpara.2004.08.001
- Stoll NR. This wormy world. J Parasitol 1947; 33:1-18; PMID:20284977; http://dx.doi.org/10.2307/3273544
- Beaumier CM, Gillespie PM, Hotez PJ, Bottazzi ME. New vaccines for neglected parasitic diseases and dengue. Trans Res 2013; 162:144-55; PMID:23578479; http://dx.doi.org/10.1016/j.trsl.2013.03.006
- Hotez PJ, Diemert D, Bacon KM, Beaumier C, Bethony JM, Bottazzi ME, Brooker S, Couto AR, Freire Mda S, Homma A, et al. The human hookworm vaccine. Vaccine 2013; 31 Suppl 2:B227-32; PMID:23598487; http://dx.doi.org/10.1016/j.vaccine.2012.11.034
- Williamson AL, Brindley PJ, Abbenante G, Prociv P, Berry C, Girdwood K, Pritchard DI, Fairlie DP, Hotez PJ, Dalton JP, et al. Cleavage of hemoglobin by hookworm cathepsin D aspartic proteases and its potential contribution to host specificity. FASEB J 2002; 16:1458-60; PMID:12205047
- Pearson MS, Bethony JM, Pickering DA, de Oliveira LM, Jariwala A, Santiago H, Miles AP, Zhan B, Jiang D, Ranjit N, et al. An enzymatically inactivated hemoglobinase from Necator americanus induces neutralizing antibodies against multiple hookworm species and protects dogs against heterologous hookworm infection. FASEB J 2009; 23:3007-19; PMID:19380510; http://dx.doi.org/10.1096/fj.09-131433
- Pearson MS, Pickering DA, Tribolet L, Cooper L, Mulvenna J, Oliveira LM, Bethony JM, Hotez PJ, Loukas A. Neutralizing antibodies to the hookworm hemoglobinase Na-APR-1: implications for a multivalent vaccine against hookworm infection and schistosomiasis. J Infect Dis 2010; 201:1561-9; PMID:20367477; http://dx.doi.org/10.1086/651953
- Skwarczynski M, Dougall AM, Khoshnejad M, Chandrudu S, Pearson MS, Loukas A, Toth I. Peptide-based subunit vaccine against hookworm infection. PLoS One 2012; 7:e46870
- Dandliker WB, Feigen GA. Quantification of the antigen-antibody reaction by the polarization of fluorescence. Biochem Biophys Res Commun 1961; 5:299-304; PMID:13719565; http://dx.doi.org/10.1016/0006-291X(61)90167-X
- Jolley ME. Fluorescence polarization assays for the detection of proteases and their inhibitors. J Biomolecular Screening 1996; 1:33-8; http://dx.doi.org/10.1177/108705719600100112
- Loukas A, Bethony JM, Mendez S, Fujiwara RT, Goud GN, Ranjit N, Zhan B, Jones K, Bottazzi ME, Hotez PJ. Vaccination with recombinant aspartic hemoglobinase reduces parasite load and blood loss after hookworm infection in dogs. PLoS Med 2005; 2:e295; PMID:16231975; http://dx.doi.org/10.1371/journal.pmed.0020295
- Jariwala AR, Oliveira LM, Diemert DJ, Keegan B, Plieskatt JL, Periago MV, Bottazzi ME, Hotez PJ, Bethony JM. Potency testing for the experimental Na-GST-1 hookworm vaccine. Expert Rev Vaccines 2010; 9:1219-30; PMID:20923271; http://dx.doi.org/10.1586/erv.10.107
- Fiser A, Sali A. MODELLER: generation and refinement of homology-based protein structure models. In: Carter CW, Sweet RM, eds. Methods in Enzymology. San Diego: Taylor & Francis, 2003:363-93
- Sali A, Blundell TL. Comparative protein modelling by satisfaction of spatial restraints. J Mol Biol 1993; 234:779-815; PMID:8254673; http://dx.doi.org/10.1006/jmbi.1993.1626
- Chen CS, Chen WN, Zhou M, Arttamangkul S, Haugland RP. Probing the cathepsin D using a BODIPY FL-pepstatin A: applications in fluorescence polarization and microscopy. J Bioch Biophys Methods 2000; 42:137-51; PMID:10737220; http://dx.doi.org/10.1016/S0165-022X(00)00048-8
- Metcalf P, Fusek M. Two crystal structures for cathepsin D: the lysosomal targeting signal and active site. EMBO J 1993; 12:1293-302; PMID:8467789
- Williamson AL, Lecchi P, Turk BE, Choe Y, Hotez PJ, McKerrow JH, Cantley LC, Sajid M, Craik CS, Loukas A. A multi-enzyme cascade of hemoglobin proteolysis in the intestine of blood-feeding hookworms. J Biol Chem 2004; 279:35950-7; PMID:15199048; http://dx.doi.org/10.1074/jbc.M405842200
- Zhao G, Zhou A, Lu G, Meng M, Sun M, Bai Y, Han Y, Wang L, Zhou H, Cong H, et al. Identification and characterization of Toxoplasma gondii aspartic protease 1 as a novel vaccine candidate against toxoplasmosis. Parasit Vectors 2013; 6:175; PMID:23768047; http://dx.doi.org/10.1186/1756-3305-6-175
- Verity CK, McManus DP, Brindley PJ. Vaccine efficacy of recombinant cathepsin D aspartic protease from Schistosoma japonicum. Parasite Immunol 2001; 23:153-62; PMID:11240906; http://dx.doi.org/10.1046/j.1365-3024.2001.00369.x
- Leal AT, Seixas A, Pohl PC, Ferreira CA, Logullo C, Oliveira PL, Farias SE, Termignoni C, da Silva Vaz I, Jr., Masuda A. Vaccination of bovines with recombinant Boophilus Yolk pro-Cathepsin. Vet Immunol Immunopathol 2006; 114:341-5; PMID:16997384; http://dx.doi.org/10.1016/j.vetimm.2006.08.011
- Sandini S, La Valle R, Deaglio S, Malavasi F, Cassone A, De Bernardis F. A highly immunogenic recombinant and truncated protein of the secreted aspartic proteases family (rSap2t) of Candida albicans as a mucosal anticandidal vaccine. FEMS Immunol Med Microbiol 2011; 62:215-24; PMID:21535228; http://dx.doi.org/10.1111/j.1574-695X.2011.00802.x
- Bartley K, Huntley JF, Wright HW, Nath M, Nisbet AJ. Assessment of cathepsin D and L-like proteinases of poultry red mite, Dermanyssus gallinae (De Geer), as potential vaccine antigens. Parasitology 2012; 139:755-65; PMID:22310226; http://dx.doi.org/10.1017/S0031182011002356
- Baldwin ET, Bhat TN, Gulnik S, Hosur MV, Sowder RC, 2nd, Cachau RE, Collins J, Silva AM, Erickson JW. Crystal structures of native and inhibited forms of human cathepsin D: implications for lysosomal targeting and drug design. Proc Natl Acad Sci U S A 1993; 90:6796-800; PMID:8393577; http://dx.doi.org/10.1073/pnas.90.14.6796
- Karolin J, Johansson L, Strandberg L, Ny T. Fluorescence and adsorption spectroscopic properties of dipyrrometheneboron difluordie (BODIPY) derivatives in liquids, lipid membranes, and proteins. J Am Chem Soc 1994; 116:7801-6; http://dx.doi.org/10.1021/ja00096a042
- Metz B, van den Dobbelsteen G, van Els C, van der Gun J, Levels L, van der Pol L, Rots N, Kersten G. Quality-control issues and approaches in vaccine development. Expert Rev Vaccines 2009; 8:227-38; PMID:19196202; http://dx.doi.org/10.1586/14760584.8.2.227