Abstract
Ticks are notorious vectors of disease for humans, and many species of ticks transmit multiple pathogens, sometimes in the same tick bite. Accordingly, a broad-spectrum vaccine that targets vector ticks and pathogen transmission at the tick/host interface, rather than multiple vaccines against every possible tickborne pathogen, could become an important tool for resolving an emerging public health crisis. The concept for such a tick protective vaccine comes from observations of an acquired tick resistance (ATR) that can develop in non-natural hosts of ticks following sensitization to tick salivary components. Mice are commonly used as models to study immune responses to human pathogens but normal mice are natural hosts for many species of ticks and fail to develop ATR. We evaluated HLA DR3 transgenic (tg) “humanized” mice as a potential model of ATR and assessed the possibility of using this animal model for tick protective vaccine discovery studies. Serial tick infestations with pathogen-free Ixodes scapularis ticks were used to tick-bite sensitize HLA DR3 tg mice. Sensitization resulted in a cytokine skew favoring a Th2 bias as well as partial (57%) protection to infection with Lyme disease spirochetes (Borrelia burgdorferi) following infected tick challenge when compared to tick naïve counterparts. I. scapularis salivary gland homogenate (SGH) and a group of immunoinformatic-predicted T cell epitopes identified from the I. scapularis salivary transcriptome were used separately to vaccinate HLA DR3 tg mice, and these mice also were assessed for both pathogen protection and epitope recognition. Reduced pathogen transmission along with a Th2 skew resulted from SGH vaccination, while no significant protection and a possible T regulatory bias was seen in epitope-vaccinated mice. This study provides the first proof-of-concept for using HLA DR tg “humanized” mice for studying the potential tick protective effects of immunoinformatic- or otherwise-derived tick salivary components as tickborne disease vaccines.
Abbreviations and Acronyms
TBD | = | Tickborne disease |
tg | = | Transgenic |
HLA DR3 | = | Human leukocyte antigen, D related 3 |
B6 | = | C57BL/6 |
ATR | = | Acquired tick resistance |
Bb | = | Borrelia burgdorferi |
Mn | = | Mus musculus |
SGH | = | Salivary gland homogenate |
IFN-γ | = | Interferon gamma |
IL-4 | = | Interleukin-4 |
ConA | = | Concanavalin A |
NPP | = | Naked peptide pool |
LPP | = | Liposomal peptide pool |
SFC | = | Spot forming cells |
NR | = | No response |
Introduction
Ticks are found in almost every region of the world and are second only to mosquitoes in their public health and veterinary importance.Citation1 However, ticks transmit the greatest variety of human and animal pathogens of any arthropod vector, including more than 20 emerging or Category A-C pathogens, all capable of causing significant disease in humans.Citation2 Few effective strategies exist for protecting humans and animals against infection caused by tickborne pathogens. Controlling ectoparasites of human and veterinary importance still relies heavily on chemical pesticides; however, effective and widespread chemical control of ticks suffers from development of resistance as well as human, animal, or environmental safety concerns.
A sound public health approach for preventing tickborne disease (TBD) would be to develop broad-spectrum vaccines or other effective means that target vector arthropods and the transmission process rather than every possible tickborne pathogen. Since most pathogens transmitted by ticks exhibit some form of transmission delay following attachment, a protective vaccine would need to stimulate immune responses during the early stages of feeding, prior to or soon after pathogen transmission commences.Citation1,3,4 The delay for effective transmission of pathogens, including the Lyme disease spirochete by nymphal Ixodes scapularis ticks is typically >24 hrs and, at least in tick-bite sensitized hosts, innate immune cells including basophils as well as CD4+ (helper) T cell activity can be activated before or soon after pathogen transmission begins.Citation5–7 T cells themselves are stimulated by a very limited number of highly specific antigenic determinants (epitopes) derived from the intruding organism's proteins. Algorithms that accurately model the MHC-peptide interface are central to the prediction of T cell epitopes and are available for mice and humans. Here, a “genes to vaccine” approach is applied to I. scapularis tick salivary proteins for predicting epitopes with immunogenic potential.
The concept of a tick protective vaccine has its foundation in the naturally occurring phenomenon of acquired tick resistance (ATR). Non-natural tick hosts, such as Guinea pigs and humans, demonstrate changes in their cell-mediated and humoral immune response upon repeat infestations with pathogen-free ticks.Citation8–10 Modification of cell-mediated immunity is characterized by migration of basophils, neutrophils and eosinophils to the tick bite site with migration facilitated by T cells and antibodies specific for tick salivary components.Citation8,11 Recent insights into the biology of basophils suggest their role as regulators of Th2 cell responses, through IL-4 expression or the differentiation of monocytes to macrophages.Citation12 Th2 polarization of the cytokine response to tick feeding has been observed both in vitro and in vivo.Citation13 Epidemiological and experimental evidence in animals and people shows that ATR can diminish or prevent pathogen transmission.Citation14,15 In fact, prior work has demonstrated that tick bite-derived ATR in the Guinea pig results in about 50–60% protection from Borrelia burgdorferi infection upon infected I. scapularis tick challenge.Citation15 Unfortunately, few reagents are available to fully characterize the Guinea pig immune response to tick-bites. Repeat tick infestations in a number of laboratory mouse strains, including BALB/c and C3H/HeN, result in significant polarization toward a Th2 cytokine profile but without protection from pathogen challenge or other evidence of ATR.Citation16 It may be possible that transgenic mice, specifically those genetically altered for human MHC II antigen presentation, could serve to fulfill the need for an alternative model in studying the tick protective response, as it would combine a ready availability of experimental reagents commonly exploited in mouse immunology studies with naturally occurring ATR that correlates with B. burgdorferi pathogen protection typically found in non-natural tick hosts.
Here, we report an evaluation of acquired tick resistance and epitope-driven immune recognition for tick protective vaccine development using tick-bite sensitized, whole tick salivary gland homogenate (SGH)-immunized, and tick SGH peptide-immunized HLA DR3 transgenic mice.
Results
Transgenic HLA DR3 mice as a model for acquired tick resistance
We used 3 serial infestations with pathogen-free I. scapularis nymphs to tick-bite sensitize HLA DR3 transgenic mice and C57BL/6 mice (B6); in this study, B6 mice serve as the genetic background control. While the HLA DR4 allele does have a greater worldwide frequency, the rationale for using the DR3 tg mouse model is founded upon the global distribution of HLA DR3 in Northern and Western Europeans and its coincidence with the worldwide distribution of B. burgdorferi (Bb), the causative agent of Lyme disease.Citation17,18 All tick sensitized mice, along with an equivalent number of tick naïve controls were subsequently pathogen challenged by infesting each animal with 3–4 nymphal I. scapularis derived from a cohort of Bb-infected ticks. Presence of Bb was confirmed by PCR in all challenge ticks recovered (data not shown). Tick-bite sensitized HLA DR3 tg mice exhibited a longer tick engorgement period () and a partial (57%) protection to Bb infection () when compared to tick naïve HLA DR3 tg mice. Moreover, Bb copy numbers from ear-punch biopsy samples were significantly lower (by 2.5-fold) in PCR positive, tick sensitized HLA DR3 tg mice compared to their tick naïve counterparts. Altered tick feeding, either shortened or prolonged, is indicative of host anti-tick responses. Grooming due to a heightened itch response contributes to abbreviated tick feeding.Citation10 Prolonged feeding usually is associated with increased pathogen transmission in tick-bite naïve hosts, while extended feeding on a tick sensitized host occurs due to an inability to obtain a proper bloodmeal. Primed host immune defenses decrease vascular size and recruit neutrophils and lymphocytes to the tick bite site creating a hostile environment for blood uptake and pathogen transmission.Citation10,19 However, unlike ATR typically seen in other non-natural tick hosts, there was no significant difference in the amount of blood ingested by tick-bite sensitized HLA DR3 tg mice compared to tick naïve HLA DR3 or tick naïve or sensitized B6 controls ().
Table 1. Days to engorgement and engorgement weight of Ixodes scapularis nymphs fed on B6 and HLA DR3 tg mice during repeated infestations prior to pathogen challenge. Data are expressed as mean ± SD. Figures in parentheses are minimum and maximum values. Statistical significance (P < 0.05) when compared to HLA DR3 primary infestation data is denoted by *
Table 2. Infection status of B6 and HLA DR3 tg mice post Borrelia burgdorferi (Bb) challenge. qPCR results are expressed as mean ± SD copies of Bb recA genes normalized to 20,000 Mus musculus (Mn) nido genes. Figures in parentheses are minimum and maximum values. Statistical significance (P < 0.05) when compared to HLA DR3 naïve copy number denoted by *
Tick-bite sensitized HLA DR3 tg mice exhibited a cytokine profile characteristic of a Th2 bias when compared with tick naïve tg mice as demonstrated by IFNγ and IL-4 ELISpot analysis (). Splenocytes derived from tick sensitized HLA DR3 tg mice and co-cultured with SGH to stimulate a recall response expressed ≈3-fold average increase in IFNγ production over background (from −7 ± 12 to 13 ± 12 spot forming cells (SFC) over background/10Citation6 cells) when compared to naïve counterparts. However, this increase remained below our criteria for ELISpot positivity of 50 SFC per million cells above background and was overshadowed by a significant 40-fold greater average SFC in the IL-4 ELISpot. IL-4 production in sensitized HLA DR3 tg mice ranged between −73 ± 61 to 533 ± 300 SFC over background/10Citation6 cells, a significant 8-fold increase over naive ().
Figure 1. Ex vivo recall IFNγ and IL-4 responses stimulated by salivary gland homogenate (SGH) in tick naïve (•) and tick-bite sensitized (○) HLA DR3 tg mice. SGH was assayed for T cell reactivity by IFNγ and IL-4 ELISpot assay using splenocytes isolated from mice. Data are the mean spot-forming cells (SFC) over background per million splenocytes that secrete cytokines in response to SGH. Individual subject average responses are represented by dots and the mean cytokine responses across all subjects by white bars. The 50 SFC over background per million splenocytes cutoff is denoted by the dotted line. Statistical significance (P < 0.05) is noted by *.
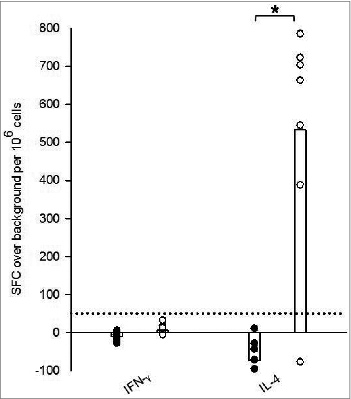
Immunization studies with whole tick SGH and selected SGH component epitopes
In a second experiment, instead of serial tick feeding to sensitize HLA DR3 tg mice, we immunized mice with either whole tick SGH or with selected T cell epitopes derived from immunoinformatic analysis of the I. scapularis salivary transcriptome. Tick salivary components in the form of either whole SGH prepared from 18 hr fed, pathogen-free nymphal I. scapularis or a pool of 11 immunoinformatic-predicted CD4+ T cell epitopes were administered intra-dermally as peptides formulated in liposomes (LLP) or without a carrier (NPP) and were followed by an assessment of pathogen protection and immune recognition.
Prediction and in vitro validation of selected tick SGH epitopes
Class II HLA epitopes were identified from the I. scapularis salivary transcriptome using the EpiMatrix T cell epitope mapping algorithm combined with ClustiMer analysis.Citation20,21 shows the EpiMatrix cluster score for a group of epitopes selected for synthesis because they were derived from salivary metalloproteases and other high abundance proteins in the transcriptome.Citation22 Ninety percent of the selected peptides demonstrated cluster scores >10, the threshold for predicted immunogenicity. Peptide 014 was the sole predicted epitope with a sub-threshold predictive score (8.88). Despite low predicted immunogenicity, peptide 014, was sourced from a salivary protein with fibrinolytic capabilities and a publication history suggesting its use as a vaccine candidate, and therefore was included in further testing.Citation22,23 All 11 peptides were assayed in vitro for their capacity to bind multiple HLA types including DRB1*0101, DRB1*0301, DRB1*0401, DRB1*0701 and DRB1*1501. Fifty-five percent of the peptides bound to at least 3 or 4 HLA alleles, and 36% bound to all 5. Of the 55 peptide-HLA binding interactions assayed, 53% were strong binders with high affinity, 11% moderate binders with mid-level affinity and 36% with low to non-detectable affinity (). The computational predictions and binding assay results were evaluated with classification of peptide-HLA binding pairs as either true positive, false positive, true negative or false negative. Positive predictions were defined as epitopes in the top 5th percentile (scoring > 1.64 on the EpiMatrix z-scale) and binding HLA at any affinity. Overall, the agreement with predictions, both positive and negative was 73%, which is consistent with prior studies.Citation24,25 With respect to each allele assayed, the values are 55% for DRB1*0101, 64% for DRB1*0301, 45% for DRB1*0401, 64% for DRB1*0701, and 91% for DRB1*1501. Discrepancies between positive predictions and actual binding include peptide folding, peptide aggregation under assay conditions, or the predictive accuracy of immunoinformatic algorithms. A large, retrospective comparison of the EpiMatrix with epitope mapping algorithms in the public domain showed that EpiMatrix was >75% accurate across all the HLA Class II alleles studied here, which is as accurate as or more accurate than other epitope prediction tools.Citation26 It is likely that a significant part of the discrepancy between predictions and HLA binding is due to peptide design and physical properties.
Table 3. HLA DR binding affinities for selected immunoinformatic-predicted Ixodes scapularis salivary epitopes. Coded peptide identifiers and predicted immunogenicity (EpiMatrix cluster score) are noted in the first 2 columns, respectively. Column 4, *0301, represents the allele found in the DR3 transgenic mouse model. IC50 values in μM units were calculated from curves fitted to dose-dependence competition binding data for each peptide-HLA DR allele pair. Peptide binding affinity is shown according to the following classification: IC50 < 10 μM (black), 10 < IC50 < 100 μM (dark gray), IC50 > 100 μM (light gray)
Borrelia pathogen challenge of SGH and epitope-immunized transgenic mice
Neither SGH- nor epitope-immunized HLA DR3 tg mice were completely protected from Bb infection when challenged by Bb-infected ticks and compared with control vaccinated animals. Although 100% infection rate is the “expected” outcome of the challenged control animals, observed results differed for the aberrant mouse due to uncharacteristic and excessive grooming resulting in early removal of ticks. The majority of mice in each vaccination arm did have detectable levels of Bb genes in ear punch biopsies (). However, similar to the tick-bite sensitized HLA DR3 tg mice, the level of Bb infection in the SGH-vaccinated mice as measured by qPCR Bb gene copy number was significantly lower (p = 0.038). Bb copy numbers within control vaccinated mice were 14-fold greater (55.9 ± 68.4) than the average 3.9 ± 4.7 copies detected in SGH-vaccinated HLA DR3 tg mice. NPP- and LPP-immunized mice exhibited mean Bb gene copy numbers of 22.1 ± 30.9 and 34.4 ± 23.1, respectively (). All SGH- and peptide-immunized mice had at least 2 infected challenge ticks that attached and fed for longer than 24 hrs, which is adequate to assure Bb transmission.Citation7
Table 4. Infection status of SGH- and peptide-vaccinated HLA DR3 tg mice post Borrelia burgdorferi (Bb) challenge. qPCR results are expressed as mean ± SD copies of Bb recA genes normalized to 20,000 Mn nido genes. Figures in parentheses are minimum and maximum values. Statistical significance (P < 0.05) when compared to control vaccinated HLA DR3 tg mice copy number denoted by *
Epitope-specific IFNγ and IL-4 responses in tick-bite sensitized transgenic mice
To begin dissecting the nature of the immune response to tick salivary components, splenocytes from both tick naïve and tick-bite sensitized HLA DR3 tg mice were evaluated for antigen-specific immune recall. Cells were stimulated with either whole SGH or individual peptides for measurement of IFNγ and IL-4 production by ELISpot assay. Only 2 peptides stimulated a differential response in tick sensitized mice when compared with their tick naïve counterparts (). Peptide 014 appeared to stimulate IFNγ production greater than 50 SFC per million splenocytes in sensitized HLA DR3 tg mice () with a 3-fold increase of 20 ± 14 mean SFC over background in naïve mice to 81 ± 13 in tick sensitized mice. However, this epitope was predicted to be a weak/non-binder for HLA DR3 () and may represent a spurious result. Differential IL-4 production among tick sensitized mice was only observed for peptide 017 (). Interestingly, tick sensitized mice demonstrated a significant decrease in production of IL-4, with a mean of −7 ± 30 SFC over background compared to a mean of 79 ± 42 SFC for tick naïve mice. While we did expect to see a greater number of individual peptide stimulations result in more robust IL-4 production among tick-bite sensitized mice, similar to what was observed following whole SGH stimulation (), from these results we can speculate that tick-bite sensitization stimulates T cells with different epitope specificities, and that the epitopes selected for this trial were not immunodominant.
Figure 2. Ex vivo recall IFNγ (A) and IL-4 (B) cytokine responses in tick naïve (•) and tick-bite sensitized (○) HLA DR3 tg mice stimulated by SGH and individual tick salivary peptides predicted by immunoinformatics. Data are the mean spot-forming cells (SFC) over background per million splenocytes that secrete cytokines in response to SGH or individual epitopes in IFNγ and IL-4 ELISpot assays. Individual subject average responses are represented by dots and the mean cytokine responses across all subjects by white bars. The 50 SFC over background per million splenocytes cutoff is denoted by the dotted line. Statistical significance (P < 0.05) is noted by *.
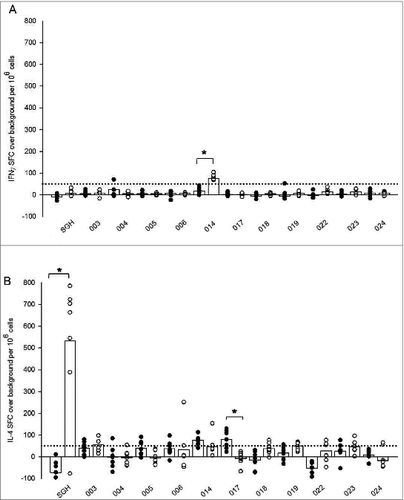
IL-4 production in SGH- and peptide-immunized transgenic mice
To better determine the antigenic potential of in silico-predicted tick SGH epitopes, individual peptide stimulations were screened by IL-4 ELISpot assay, as above, but using splenocytes derived from control-, whole SGH- and peptide-immunized HLA DR3 tg mice prior to pathogen challenge. Splenocytes from SGH-immunized mice restimulated with SGH demonstrated >6-fold increase in IL-4 production compared with control-vaccinated mice (P = 0.015) (). Specifically IL-4 production in control HLA DR3 tg mice exhibited a mean of −48 ± 78, while cytokine production in SGH-immunized mice peaked at 669 SFC with mean IL-4 production of 259 ± 263 SFC. Stimulation of splenocytes from SGH-immunized mice using either the peptide pool or individual peptides yielded minimal production of IL-4, never greater than 50 SFC per million typically considered to be a positive response.
Figure 3. Antigen-specific HLA DR3 tg mouse IL-4 responses stimulated by tick SGH and immunoinformatics-predicted tick salivary epitopes in control-, SGH-, and peptide-vaccinated mice. Whole SGH, peptide pool and individual epitopes were assayed for T cell reactivity by IL-4 ELISpot assay using splenocytes isolated from vaccinated mice. Data are the mean spot-forming cells (SFC) over background per million splenocytes that secreted cytokines in response to antigen-specific stimulation. Individual subject average responses from experimental vaccination arms are represented as: control immunizations (▪), SGH immunizations (○), naked peptide pool (NPP) immunizations (▴), liposomal peptide pool (LPP) (▿). The mean cytokine responses across all subjects are represented by white bars. The 50 SFC over background per million splenocytes cutoff is denoted by the dotted line. Statistical significance (P < 0.05) is noted by *.
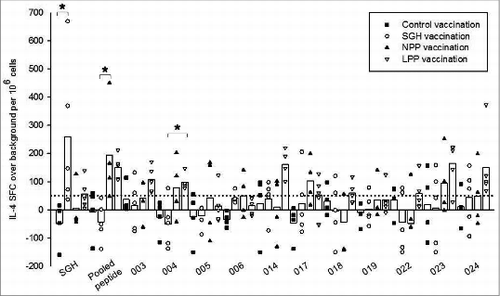
In mice immunized using the peptide pool (LPP and NPP), re-stimulation using either the peptide pool or individual peptides resulted in mean IL-4 production greater than 50 SFC per million for 8 out of 11 (73%) individual epitopes as well as the peptide pool, although results varied somewhat depending on whether the peptides were incorporated into liposomes (LPP) or were immunized as naked peptides (NPP)(). Recognition of the peptide pool yielded a significant ≈6-fold increase in production of IL-4 (mean = 194 ± 177 SFC) in NPP-immunized mice when compared to the SGH-immunized arm (P =0.005). In individual peptide stimulations, epitopes 003, 004, 014, 017, 018, 022, 023 and 024 all stimulated a mean greater than 50 SFC per million cells in LPP-vaccinated HLA DR3 tg mice, while only 3 of these 11 epitopes (004, 017 and 023) stimulated a mean more than 50 SFC per million cells in NPP-vaccinated mice, suggesting that peptide formulation in liposomes enhanced immunogenicity. One of these epitopes, 004, produced ≈3× increased SFC (mean = 98 ± 28 SFC) in LPP-vaccinated mice than in SGH-vaccinated mice (mean = −51 ± 85 SFC), which was a significant result (P =0.023). Peptide 004 was predicted to be a strong HLA DR3 binder. Five of the 8 epitopes that produced greater than 50 SFC per million in LPP-vaccinated mice were predicted to be either strong or moderate HLA DR3 binders, validating HLA binding as a pre-screening tool for epitope selection in future tick protective vaccine studies using HLA transgenic mice. Interestingly, mice immunized with the peptide pool either with and without liposomal carrier, did not respond to stimulation with whole SGH.
Three peptides (005, 006, and 019) failed to stimulate any significant IL-4 responses. The small cohort size of the study and restricted HLA background may explain this result. Alternatively, these sequences may stimulate helper T cell cytokines other than IL-4.
Cytokine profile of SGH and peptide-immunized transgenic mice
Concurrent characterization of TH1/TH2/TH17 cytokine profiles of immunized mice following prolonged (24, 48 and 72 hrs) co-culture with ConA, SGH and pooled peptides was assayed by cytokine bead array. displays 48 hr co-culture data which appeared to be the optimal incubation timepoint for cytokine expression. Co-culture with stimulants for 72 hrs resulted in little to no response possibly because of cell exhaustion due to prolonged stimulant exposure (data not shown). Co-culture with ConA served as positive control for this assay and although results varied for each cytokine in the panel (lowest IL-4 @ 16 pg/mL; highest IFNγ @ 260 pg/mL), for every cytokine assayed, concentrations (pg above background) were consistent between each of the experimental arms (data not shown).
Figure 4. Multiplex cytokine bead array (CBA) profile of splenocytes from vaccinated HLA DR3 tg mice co-cultured for 48 hrs either with whole SGH (A) or the tick salivary epitope peptide pool (B) used in 2 of the experimental vaccination arms. Data are concentrations of cytokines in picograms (pg/mL) above background elicited by splenocytes in response to antigen-specific stimulation. Values of zero are represented below the x-axis as no response (NR). Vaccination arms are represented as: Control vaccination arm- lightest gray, SGH immunization arm- white, NPP vaccination arm- dark gray, LP immunization arm- black. Control splenocytes were co-cultured with medium + adjuvant alone resulting in cytokine production below 12 pg/mL for all monitored cytokines (data not shown).
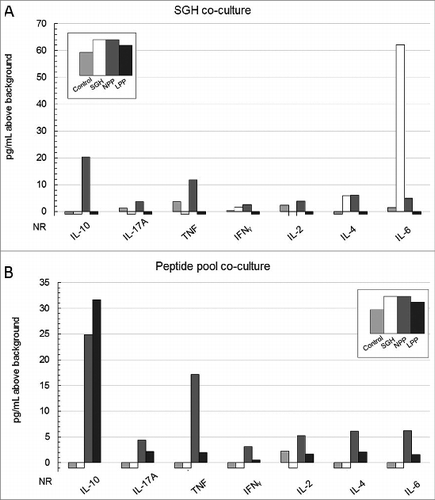
Not surprisingly, Th2-associated cytokines IL-4 (6 pg/mL) and TH17-predominant IL-6 (62 pg/mL) were the main cytokines stimulated by SGH in SGH-immunized HLA DR3 tg mice, comprising 98% of the overall response in this vaccination arm (). Mean IL-6 production stimulated by SGH in the SGH-immunized mice was 41-fold greater than the control immunization group while mean IL-4 production was 6-fold greater than in the control vaccine arm. IL-10 (20 pg/mL) and TNF (12 pg/mL) were the dominant cytokines expressed by NPP-vaccinated mice when re-stimulated with SGH representing 60% of the overall response (). The remainder of the overall cytokine response stimulated by SGH in NPP-immunized mice was generally distributed across the other cytokines assayed. SGH-stimulated cells from either control or LPP-vaccinated mice expressed little to no response in the CBA assay.
The predominant peptide pool-stimulated cytokine response in cells from peptide-vaccinated mice was IL-10 which comprised 37% of the total measured cytokine response in NPP-vaccinated (25 pg/mL) mice and 76% of the response in LPP-vaccinated mice (32 pg/mL). The remainder of the overall cytokine response stimulated by the peptide pool was fairly evenly distributed across the other cytokines assayed. Peptide pool-stimulated cells from either control or SGH-vaccinated mice expressed little to no cytokine response.
Discussion
Since its discovery by Trager in 1939, acquired resistance to tick feeding has been extensively studied in laboratory animals.Citation27 Repeated tick infestations of Guinea pigs and various murine strains act as separate puzzle pieces that when combined, provide an expanding picture of the features of tick-host interactions. Traditionally ATR was identified by the effect on the tick: altered feeding duration, decreased engorgement weight, reduced production and viability of ova, and inhibition of molting.Citation28 Observations of ATR in the Guinea pig model also has provided insight into the role of basophils at the host cutaneous bite site.Citation8 Even more, partial protection from Borrelia challenge seen in tick sensitized Guinea pigs provided evidence that potential vaccine candidates reside in the complex cocktail of tick saliva.Citation15 Though first identified in Guinea pigs, the Th2 bias of repeated tick infestations was best represented by gene expression and cytokine profile studies in BALB/c, C3H/HeN and C3H/HeJ murine strains. Citation29-32 However, in those mouse models, repeat tick exposure induced immune response changes but neither BALB/c nor C3H/HeN mice developed resistance.Citation16,33-35
Here, we have presented data that supports adding HLA DR3 transgenic mice to the list of model organisms for acquired tick resistance and tick protective vaccine discovery research. Of course, further characterization of these “humanized” transgenic mice exposed to repeat tick infestations is needed, including expanding the model to include other transgenic (e.g., DR4) murine strains. Future studies could examine the effects of ATR in HLA tg mice on molting success and egg production but most certainly should include a deeper characterization of the CD4+ T cell response to tick salivary components. Assessing the role of antibodies to tick salivary proteins, especially IgE, and characterizing cellular infiltrates at the tick bite site would likely yield additional immune correlates to the nearly 60% protection against Bb infection that we recorded. However, the strong Th2 bias present in the tick-bite sensitized IL-4 ELISpot studies linked with our observed 57% protection from Borrelia pathogen challenge, suggests that the HLA DR3 tg mouse model appears capable of bridging the previously useful Guinea pig ATR model and well-established murine models commonly used in immunology and early vaccine discovery studies. The off-the-shelf availability of resources and reagents for the murine model is one compelling reason for adopting “humanized” mice for tick protective vaccine development studies. Moreover, the presentation of human MHC II antigen in the context of the mouse model brings the HLA DR3 tg mouse model closer to the human perspective, which is an added benefit for vaccine discovery research.
As noted above, any vaccine-mediated tick protective response would likely need to be stimulated during the early stages of tick feeding, prior to or soon after pathogen transmission commences.Citation1,3,4 With this key concept in mind, splenocytes from tick-bite sensitized and SGH- or peptide-vaccinated HLA DR3 mice were re-stimulated with SGH that had been prepared from salivary glands harvested from nymphal I. scapularis early (18 hr) in the tick feeding process. Additionally, during immunization studies, tg mice were immunized with 18 hr SGH. Salivary gland dissection and collection parameters are noteworthy due to the dynamic nature of gene expression in the tick salivary transcriptome, and the desire to concentrate on vaccine candidates present during early feeding, prior to pathogen transmission. Previous studies have even suggested that tick salivary proteins expressed prior to 24 hrs are sufficient to induce, at least a partial tick protective immunity. Citation5,7,36
Empirical evidence of ATR in Guinea pigs subjected to repeat infestations with the tick Rhipicephalus sanguineus, demonstrated that experimental tick infestations were superior to vaccination with unfed, crude, whole tick extract.Citation37 Likewise, our own studies showed that the protective response to Bb pathogen challenge following immunizations with 18 hr SGH proved not as effective as when induced by repeat I. scapularis infestations. Tg mice immunized with SGH did have significantly fewer copies of Bb in ear punch biopsies than control animals, but the pathogen infection rates between the 2 groups of animals were similar. The IFNᵧ ELISpot assays documented ≈3x more spots over background in tick-bite sensitized mouse splenocytes re-stimulated with SGH when compared to their control counterparts. The IFNᵧ response of tick-bite sensitized DR3 mice, though significant, is eclipsed when compared to the 40-fold higher average IL-4 response to co-culture with SGH. Differential production of IL-4 between tick-bite sensitized and tick-bite naïve DR3 mice showed >8 -fold difference using similar assay conditions. The lower level of protection (reduced Bb gene copy numbers, ) afforded by SGH-vaccination also was correlated with the well documented Th2-polarized cytokine response to tick saliva. We noted by CBA, increased levels of IL-4, IL-10 and IL-6 in SGH-immunized co-cultures in agreement with Titus's review of the immune-modulatory factors of I. scapularis saliva.Citation38 We also demonstrated that, in the best case, 8/11 epitopes drawn from the I. scapularis salivary transcriptome stimulated an IL-4 recall response post peptide pool-vaccination (LPP). Albeit, the extent of Th2 polarization and pathogen protection by SGH or peptide vaccination was inferior to that seen in experimental tick-bite sensitizations, this study is proof of concept that in searching for a tick protective vaccine, the transgenic mouse model is worth exploring further with immunoinformatic-predicted tick salivary epitopes.
The cytokine response in peptide-vaccinated mice was not coordinated into a distinct Th1 or Th2 response as evident in the multiplex CBA data (). This non-polarized, IL-10 dominant immune response to peptide immunizations may explain the lack of protection upon pathogen challenge. Indeed, the CBA data from NPP-immunized mice co-cultured with pooled peptides () suggests conflicting immune responses driven by anti-inflammatory cytokine IL-10 and pro-inflammatory IL-4, IL-6 and TNF. It is highly likely that a different pool of tick-derived peptides would yield a cytokine profile that is Th2-focused. Since our findings point to a Th2 correlate of protection with a predominant IL-4 cytokine response, future studies will focus on identifying and immunizing the HLA DR3 tg mice with peptides that drive a more robust IL-4 response.
Increased production of IL-10 in response to tick saliva is a well characterized aspect of repeat tick infestations.Citation31,39 Our data in the HLA DR3 mouse supports and expands these findings showing high levels of IL-10 production in NPP-immunized mice in response to co-culture with SGH as well as the peptide pool (). IL-10 is a multifunctional anti-inflammatory cytokine that dampens the inflammatory response and helps prevent tissue damage. Its role in a T regulatory manner has been suggested only recently during transcriptional profiling of repeat tick infestations of BALB/c mice in which up-regulation of IL-10 and FoxP3 was monitored at the tick-bite site Citation29. It may be that immunizations with the selected immunoinformatic-derived peptides induced a T regulatory response, accountable for the considerable IL-10 accumulation and lack of protection from pathogen challenge. One particular peptide within the pool, peptide 017, with its significantly lower IL-4 production in tick-bite sensitized tg mice compared to naïve mice () suggests a potential regulatory function which may have influenced the immunization results. Post hoc in silico analysis of this epitope for possible TCR-face homology with human (self) sequences was performed using JanusMatrix, a novel algorithm developed after the tick salivary antigen epitopes were identified.Citation40 JanusMatrix parses the amino acid sequence of T cell epitopes into TCR-facing residues and HLA-facing residues, and then compares the TCR face to other putative T cell epitopes in the human genome. JanusMatrix defines cross-reactive T cell epitopes as those that have the same MHC allele restriction, the same or similar T cell-facing residues (epitope), and conserved binding of MHC-facing residues (agretope).Citation40 JanusMatrix analysis reveals that there are 9-mers in peptide 017 that share a TCR face with 10 human 9-mers. However, this analysis should be viewed conservatively, since JanusMatrix screens for homology against the human and not the murine genome. Regardless, the potential T regulatory role of tick saliva and salivary components is an interesting and likely necessary new frontier requiring consideration in going forward with tick protective vaccine research in both the novel HLA DR3 tg mouse model and humans. The JanusMatrix algorithm makes it possible to pre-screen candidate sequences for potential Treg activation to carefully select tick salivary protein epitopes that will support a Th2-focused immune response for better epitope-based vaccine design in the future.
Methods
Predicting immunogenic peptides from the I. scapularis salivary transcriptome with EpiMatrix and ClustiMer analysis
EpiMatrix is a T cell epitope-mapping algorithm used to identify putative HLA ligands/T cell epitopes contained within protein sequences (e.g., epitopes identified from pathogen genomes).Citation41,42 Unlike previous EpiMatrix analyses of genomes, the tick salivary transcriptome was used as the input sequence and subsequent source of epitope peptides.Citation21 Computation was performed by using a set of HLA allele-specific coefficient matrices. To complete an analysis using the EpiMatrix algorithm, target protein sequences were parsed into overlapping 9-mer frames in which each frame overlaps the last by 8 amino acids. Each amino acid in the 9-mer was then assigned a positive or negative coefficient based on its previously determined propensity to positively or negatively impact peptide binding when located at that amino-acid position within the HLA-binding groove.Citation43 The coefficients were then summed to produce a raw score for each 9-mer. Raw scores were normalized with respect to a distribution derived from a large set of randomly generated peptide sequences. The resulting Z-scores from this distribution were directly comparable across predictions for different alleles.
ClustiMer identified “clustered” or promiscuous epitopes.Citation44,45 Potential T cell epitopes are not randomly distributed throughout protein sequences but instead tended to “cluster” in specific regions. T cell epitope “clusters” range from 9 to roughly 25 amino acids in length and, considering their affinity to multiple alleles and across multiple frames, could contain anywhere from 4 to 40 binding motifs. Class II epitope selections for vaccine design were usually limited to selected “promiscuous epitopes” – epitopes that had the potential to be recognized in the context of more than one HLA. EpiMatrix ClustiMer scores above 10 were considered to be immunogenic.
Peptide synthesis and HLA binding assay
All peptides used in this study were synthesized by 9-fluoronylmethoxy-carbonyl (Fmoc) synthesis to a purity of >80% as determined by HPLC by 21st Century Biochemicals (Marlborough, MA, USA). The peptide masses were confirmed by MALDI-TOF mass spectrometry.
Peptides were assayed for HLA affinity in a competition binding assay based on Steere et al.Citation46 In 96-well plates, a test peptide and a reference peptide compete for binding to a purified class II HLA molecule (Benaroya Research Institute) for 24 h at 37°C. Non-biotinylated test peptides were evaluated over a wide range of concentrations (0.01–200 μM) while biotinylated standard peptide was held at a fixed concentration (0.1 μM). Peptide-class II HLA complexes were then captured on ELISA plates using pan anti-HLA-DR antibody (L243, BioXCell). Plates were washed and incubated with Europium-labeled streptavidin (Perkin-Elmer) for one hour at room temperature. Europium activation buffer was added to develop the plates for 15–20 min at room temperature before they were read on a Time Resolved Fluorescence (TRF) plate reader. All assays were performed in triplicate. Dose dependence curves were generated by fitting data using the 4-parameter logistic equation, and IC50 values were calculated in SigmaPlot 11.0 (Systat). Based on the IC50 values, peptide binding to a given HLA allele was classified as high affinity (1–10 μM), moderate affinity (10–100 μM), low affinity (>100 μM). Peptides that did not inhibit the binding of the biotinylated reference peptide at any concentration were considered non-binders. Binding assays were performed for 5 alleles: DRB1*0101, DRB1*0301, DRB1*0401, DRB1*0701, and DRB1*1501, providing a broad representation of class II HLA allele binding pockets.Citation47
Experimental animals
Six to 14 week-old female C57BL/6 mice (B6) were purchased from Harlan. HLA DR3 transgenic mice on a C57BL/6 background were obtained from Dr. Chella David (Mayo Medical School) under MTA. The mice express the HLA DR3α and β genes on a B.10-Ab0 mouse background and were back-crossed over 6 generations with class II-negative on a C57BL/6 background.Citation48 Specifically, DRBI*0301 (DR3) transgenic mice were generated by co-injection of an HLADRα genomic fragment and a DRB1*0301β gene fragment into (C57BL/6 X DBA/2)F1 X C57BL/6 embryos and backcrossed to B10 mice as detailed previously.Citation49 The DR3 transgene was first introduced into B10.M mice at the Mayo Clinic by repeated backcrossing. Subsequently, the DR3 gene was introduced into the class II-negative H2A0 strain by mating the B10.M-DRBI*0301 line with the B10.Ab0 line, similar to the strategy detailed previously for HLADQ transgenic mice.Citation50,51 All studies were performed in full compliance with the standards of the University of Rhode Island Institutional Animal Care and Use Committee and in accordance with NIH publications entitled “Principles for Use of Animals” and “Guide for the Care and Use of Laboratory Animals.”
Tick rearing and salivary gland homogenate
Ixodes scapularis ticks were reared using standard methods.Citation4 Adult ticks were collected from nature to create immature tick colonies. One hundred larvae from each egg batch were screened by PCR using pathogen-specific primers for Borrelia burgdorferi and Borrelia miyamotoi. Certified pathogen-free larval stage ticks were blood fed on hamsters or white-footed mice. To generate Bb-infected nymphs, pathogen-free larvae were allowed to feed on white-footed mice previously infected with the B31 strain of B. burgdorferi, and were then held until molting. Such methods generally yield >90% Bb infectivity. All unfed ticks were maintained at 23°C and >90% relative humidity under 14 h light/10 h dark photoperiod before infesting hosts. Methods for generating and maintaining Bb-infected and uninfected tick colonies, including animal care, followed approved URI IACUC protocols.
Pathogen-free nymphs were allowed to feed for 18–20 hours prior to dissection for salivary glands. Partially fed ticks were dissected in ice-cold phosphate buffered saline (PBS) within 4 hrs of being removed from the host animal. After removal, glands were washed in the clean buffer and tissues were stored at −70°C in PBS until cell lysis by sonication. Protein concentration was detected by UV nanodrop quantification (ThermoScientific, ND-1000).
Tick-bite sensitizations
Tick-bite sensitizations
Prior to each infestation, animals were anesthetized by IP injection of ketamine (20mg/mL) (Vedco, NDC50989-161-06) and xylazine (2mg/mL) (Lloyd Laboratories, 4821) and placed in stainless steel, quarter-inch wire mesh tubes for up to 3 hours. Tick attachment was assessed by counting attached ticks 2 hrs after placement. At this time, all but 3 attached ticks were removed in an effort to minimize “sharing” of tick salivary immunosuppressive proteins. By selective removal, the 3 ticks that remained attached were distributed as far apart as possible. A total of 14 HLA DR3 and 11 B6 mice were sensitized to tick bites 3 times using pathogen-free nymphs attached for 72 hrs. Tick feedings occurred at 2 week intervals. Additionally, groups of 16 HLA DR3 and 11 B6 mice acted as non-tick sensitized (tick naïve) controls. Biologic data related to tick feeding (engorgement weight and days to engorgement) were analyzed by Kruskal-Wallis ANOVA of Variance on Ranks and the means compared by Dunn's test (P < 0.05) (SigmaPlot).
Pathogen challenge
Two weeks following the 3rd infestation, mice, including naïve controls, were challenged with 3 Bb-infected nymphs. All animals were monitored daily, and tick feeding parameters (duration of attachment, total recovered engorged, and engorgement weight) were recorded. Animals subjected to Bb challenge were allowed to rest for 4 weeks to allow for dissemination of bacteria.
Detection of Borrelia infection
The Bb infection status of each animal was assessed 4 weeks following infected tick challenge by direct PCR assay of ear punch biopsy. Positive infection status of an animal was determined if Borrelia specific genes were amplified from ear punch samples by quantitative PCR.
Total genomic DNA was extracted from ear punches using the DNeasy Blood and Tissue kit (Qiagen, 69504) according to manufacturer's protocol. Additionally, DNA was extracted from a non-infected mouse ear punch and a culture of Bb strain B31 for qPCR standard generation. Primers amplifying mouse nido were used as a reference gene during simultaneous detection of Bb recA gene. The oligonucleotide primers used to detect mouse nidogen were nido.F 5′-CCA GCC ACA GAATAC CAT CC-3′, and nido.R 5′-GGA CT ACT CTG CTG CCATC-3′.Citation52 The oligonucleotide primers used to detect Bb recA were nTM17.F 5′-GTG GAT CTATTG TAT TAG ATG AGG CTC TCG-3′ and nTM17.R 5′-GCC AAA GTT CTG CAA CAT TAA CAC CTA AAG-3′.Citation53 Real-time quantitative PCR (qPCR) was performed using an Mx4000 Multiplex Quantitative PCR System (Stratagene, La Jolla CA). Quantitation of DNA copy number was performed using Brilliant SYBR Green qPCR Master Mix (Agilent, 600828) with 10 ng of total DNA in 50 μl reactions. Thermal profile was 95°C for 15 min then 40 cycles of 95°C for 30 s and 55°C for 1 min and 72°C for 1 min. Fluorescence was measured at the end of the 55°C step every cycle. Samples were run in experimental duplicate with inter-plate and no template controls. SYBR threshold was locked at 0.010 with ranges of efficiency between 95–100%. Copies of Bb recA genes were normalized against 20,000 copies of mouse nido genes. Samples were determined to be unifected if no Bb recA genes were detected in either duplicate, as indicated by “No CT value." Positive infection status was recorded if Bb recA genes were identified in either one or both duplicates. Copy number data were analyzed by Kruskal-Wallis ANOVA of Variance on Ranks and the means compared by Dunn's test (P < 0.05) using SigmaPlot software.
ELISpot detection of cytokine production in HLA DR3 tg mice
The frequency of epitope-specific splenocytes was determined by IFNγand IL-4 ELISpot assay using the Mabtech IFN-gamma (Mabtech, 3321–4HPT-4) or IL-4 (Mabtech, 3311–2HW-Plus) ELISpot Kits according to the manufacturer's protocol (Mariemont, OH). Briefly, spleens were harvested from groups of naive and sensitized HLA DR3 transgenic mice and mascerated to produce single cell splenocyte suspensions in RPMI–10% (Fisher, SH30027 FS) fetal bovine serum–1% (Thermo Scientific, SH3007003HI) penicillin/streptomycin–1% (Lonza, 17602E) l-glutamine–0.1% (MP Biomedicals, 091680149) and BME (Sigma Aldrich Aldrich, M6250–10ML) at a concentration of 1.5 × 10Citation6 cells/mL. Cells were transferred at 1.5 × 105/well to ELISpot plates pre-coated with anti-murine IFN-gamma by the manufacturer. IL-4 plates were not pre-coated and were prepared 24 hrs in advance according to manufacturer's specification. Cells were stimulated with SGH at 20 μg/mL in triplicate wells and individual peptides at (20 mg/mL also in triplicate). Cells co-cultured with ConA (2 μg/ml) (Sigma Aldrich, C5275–5MG) acted as positive controls while cells stimulated with no peptide (PBS only) served as a negative control. ELISpot plates were incubated at 37°C, 5% CO2 for 18 hours, washed, incubated with a secondary HRP labeled anti-IFN-gamma antibody or anti-IL-4 antibody and developed by addition of TMB substrate. Raw spot counts were recorded by a CTL S5 UV ELISPOT reader. Results were recorded as the mean number of SFC over background and adjusted to spots per one million cells seeded. Responses to stimulations are considered positive if the number of spots are: 1) at least twice greater than background, 2) greater than 50 spot forming cells per one million splenocytes over background (i.e. one response over background per 20,000 splenocytes), and 3) statistically significant by Wilcoxon Signed Rank Test in comparison with the corresponding spot forming cell data set for other groups (p < 0.05) using SigmaPlot software.
Immunization of HLA DR3 transgenic mice
Prior to each immunization, animals were anesthetized by IP injection of ketamine (20mg/mL) and xylazine as previously stated.
A control group of 8 HLA DR3 tg mice were immunized intra-dermally with saline and Imject Alum® adjuvant (ThermoScientific, 77161) in 1:1 ratio for a total inoculation of 100 μL on 3 occasions over 4 weeks (days 0, 14, 25).
A group of 9 HLA DR3 transgenic mice received intra-dermal immunizations of 50 μg/100 μL of SGH with 50 μg/100 μL of adjuvant on 3 occasions over 4 weeks (days 0, 14, 25).
A group of 8 HLA DR3 transgenic mice were immunized intra-dermally with a pool of tick salivome predicted peptides, in equal representation, and a 1:1 ratio of adjuvant for a total of 50 μg peptide/100 μL dose on 3 occasions over 4 weeks (days 0, 14, 25). This immunization arm was referred to as naked peptide pool (NPP).
A group of 9 HLA DR3 transgenic mice received intradermal immunizations of liposomal formulated peptides and an equal volume of adjuvant in 100 μL on 3 occasions over 4 weeks (days 0, 14, 25). This immunization arm was referred to as peptide pool in liposomes (LPP). Sterically stable cationic liposomes were prepared from 3 lipid components: dioleylphosphatidylethanolamine (DOPE), dimethylaminoethane-carbamoyl-cholesterol (DC-cholesterol), and polyethylene glycol 2000-phosphatidyl-ethanolamine (PEG). The lipids were mixed in chloroform, dried in a rotary evaporator, and re-suspended in phosphate-buffered saline (PBS) to make empty multi-lamellar vesicles. These vesicles were sonicated 5 times for 30 seconds each at 4°C to convert them into multi-lamellar liposomes. Multi-lamellar liposomes (10 nmol) were mixed with peptides, flash frozen, and freeze-dried overnight. To encapsulate peptides in liposomes, the resulting powder was re-suspended with sterile distilled water and vortexed for 15 seconds every 5 minutes for 30 minutes at room temperature. PBS was added to yield a final liposome concentration of 10 mM lipid/mg peptides. Vesicles <150 nm in diameter were produced by 20–30 cycles of extrusion through polycarbonate filters using a Lipofast extruder (Avestin, Canada). Liposome formulations were stored at 4°C until use.
Cytokine profile detection of immunized HLA DR3 mice
At day 42, 4-5 mice from each immunization arm were euthanized to determine the effect of immunizations on cytokine production. The frequency of epitope-specific splenocytes was determined by IL-4 ELISpot assay using methods as previously stated with the following differences. Post immunization, spleens were harvested from control immunization groups and groups of HLA DR3 tg mice immunized to SGH, NPP and LPP. Cells were enumerated and plated as previously stated. Cells were co-cultured with SGH at 20 μg/mL in triplicate wells, pooled peptides, PP (20 μg/mL) and individual peptides (20 μg/mL). Cells stimulated with ConA (2 μg/ml) acted as positive controls while cells stimulated with PBS (no peptide) served as negative controls. ELISpot plates were incubated at 37°C, 5% CO2 for 18 hours. Washing, detection and spot detection was completed as previously stated. Positive response criteria for ELISpot analysis remains as previously stated, however statistical significance is determined by Kruskal-Wallis ANOVA of Variance on Ranks with post-hoc Dunn's test in comparison with the corresponding spot forming cell data set for other groups (P < 0.05).
Additionally, cytokines were simultaneously monitored by cytokine bead array (CBA) according to manufacturer's specifications using the BD Bioscience Th1/Th2/Th17 kit and appropriate standards (BD Bioscience, 560485, 561665) for detection of IL-10, IL-17A, TNF, IFN-gamma, IL-2, IL-4 and IL-6. Briefly, splenocytes were harvested from immunized mice and transferred in triplicate to non-tissue culture, v-bottom 96 well plates for stimulation with ConA (2 μg/mL), PBS, SGH (20 μg/mL) and pooled peptides, PP (20 μg/mL). Cells were incubated at 37°C, 5% CO2 for 24, 48 and 72 hrs. Post incubation, plates were pelleted and supernatant was collected and stored at −80°C until the CBA assay was performed. Cell supernatants from individual mice within immunization arms and timepoints were combined for testing. Supernatants were monitored for cytokine production using an LSRII Flow Cytometer and analyzed using the FloJo software. Cytokine production is presented as picogram per mL values above background.
Borrelia challenge of immunized HLA DR3 Mice
Additionally on day 42, the remaining 4 mice from each immunization arm were challenged with 3 Bb-infected nymphs as previously stated. Infection status of each animal was determined 3 weeks post challenge, as previously stated to asses any levels of protection afforded by immunization. qPCR-generated Bb RecA gene copy number data were analyzed by Kruskal-Wallis ANOVA of Variance on Ranks and the means compared by Student-Newman-Keul's test (P < 0.05) (SigmaPlot).
Disclosure of Potential Conflicts of Interest
Two of the contributing authors, Anne S. De Groot and William D. Martin, are senior officers and majority shareholders at EpiVax, Inc., a privately owned biotechnology company located in Providence, RI. Leonard Moise is employed by and holds stock options in EpiVax. These authors acknowledge that there is a potential conflict of interest related to their relationship with EpiVax and attest that the work contained in this research report is free of any bias that might be associated with the commercial goals of the company.
Acknowledgments
The authors are grateful to Emily Troiano for dissection of tick salivary glands, Jason LaPorte for assisting with tick infestations and Tori Deschenes for assistance with CBA. We also thank Dr. José Ribeiro for his invaluable informatics assistance with the tick salivome.
Funding
This work was supported by NIH U19 grant (AI082642) (to A.D.G.). It is Contribution No. 5404 of the Rhode Island Agriculture Experiment Station. The content is solely the responsibility of the authors and does not necessarily represent the official views of the National Institute of Allergy and Infectious Diseases or the National Institutes of Health.
References
- Sonenshine D. Biology of Ticks. New York: Oxford University Press, 1991.
- Balashov IS. A translation of Bloodsucking ticks (Ixodoidea)–vectors of diseases of man and animals. College Park, MD: Entomological Society of America, 1972.
- Barbour AG, Fish D. The biological and social phenomenon of Lyme disease. Science (New York, NY) 1993; 260:1610–6; http://dx.doi.org/10.1126/science.8503006
- Mather TN, Mather ME. Intrinsic competence of three ixodid ticks (Acari) as vectors of the Lyme disease spirochete. J Med Entomol 1990; 27:646-50; PMID:2388239
- Gern L. Life cycle of Borrelia burgdorferi sensu lato and transmission to humans. Curr Prob Dermatol 2009; 37:18-30; PMID:19367095; http://dx.doi.org/10.1159/000213068
- van der Burg SH, Bijker MS, Welters MJP, Offringa R, Melief CJM. Improved peptide vaccine strategies, creating synthetic artificial infections to maximize immune efficacy. Adv Drug Deliv Rev 2006; 58:916-30; PMID:16979788; http://dx.doi.org/10.1016/j.addr.2005.11.003
- Piesman J, Mather TN, Sinsky RJ, Spielman A. Duration of tick attachment and Borrelia burgdorferi transmission. J Clin Microbiol 1987; 25:557-8; PMID:3571459
- Allen JR. Tick resistance: basophils in skin reactions of resistant guinea pigs. Int J Parasitol 1973; 3:195-200; PMID:4706571; http://dx.doi.org/10.1016/0020-7519(73)90024-6
- Askenase PW, Bagnall BG, Worms MJ. Cutaneous basophil-associated resistance to ectoparasites (ticks). I. Transfer with immune serum or immune cells. Immunology 1982; 45:501-11; PMID:7061108
- Krause PJ, Grant-Kels JM, Tahan SR, Dardick KR, Alarcon-Chaidez F, Bouchard K, Visini C, Deriso C, Foppa IM, Wikel S. Dermatologic changes induced by repeated Ixodes scapularis bites and implications for prevention of tick-borne infection. Vector Borne Zoonotic Dis 2009; 9:603-10; PMID:19196014; http://dx.doi.org/10.1089/vbz.2008.0091
- Brown SJ, Askenase PW. Immune rejection of ectoparasites (ticks) by T cell and IgG1 antibody recruitment of basophils and eosinophils. Fed Proc 1983; 42:1744-9; PMID:6601034
- Nadif R, Zerimech F, Bouzigon E, Matran R. The role of eosinophils and basophils in allergic diseases considering genetic findings. Curr Opin Allergy Clin Immunol 2013; 13:507-13; PMID:23974679; http://dx.doi.org/10.1097/ACI.0b013e328364e9c0
- Bowman A, Nuttall P, eds. Ticks: Biology, Disease and Control: Cambridge University Press, 2008; 186-204.
- Burke G, Wikel SK, Spielman A, Telford SR, McKay K, Krause PJ. Hypersensitivity to ticks and Lyme disease risk. Emer Infect Dis 2005; 11:36-41; PMID:15705320
- Nazario S, Das S, de Silva AM, Deponte K, Marcantonio N, Anderson JF, Fish D, Fikrig E, Kantor FS. Prevention of Borrelia burgdorferi transmission in guinea pigs by tick immunity. Am J Trop Med Hyg 1998; 58:780-5; PMID:9660463
- Schoeler GB, Manweiler SA, Wikel SK. Ixodes scapularis: effects of repeated infestations with pathogen-free nymphs on macrophage and T lymphocyte cytokine responses of BALB/c and C3H/HeN mice. Exp Parasitol 1999; 92:239-48; PMID:10425152; http://dx.doi.org/10.1006/expr.1999.4426
- Xu Q, Seemanapalli SV, Reif KE, Brown CR, Liang FT. Increasing the recruitment of neutrophils to the site of infection dramatically attenuates Borrelia burgdorferi infectivity. J Immunol (Baltimore, Md: 1950) 2007; 178:5109-15; PMID:17404293; http://dx.doi.org/10.4049/jimmunol.178.8.5109
- Gonzalez-Galarza FF CS, Middleton D, Jones AR. Allele frequency net: a database and online repository for immune gene frequencies in worldwide populations. Nucleic Acid Res 2011; 39: D913-9; PMID:21062830; http://dx.doi.org/10.1093/nar/gkq1128
- Kurtenbach K, Hanincová K, Tsao JI, Margos G, Fish D, Ogden NH. Fundamental processes in the evolutionary ecology of Lyme borreliosis. Nat Rev Micro 2006; 4:660-9; PMID:16894341; http://dx.doi.org/10.1038/nrmicro1475
- De Groot AS, Bosma A, Chinai N, Frost J, Jesdale BM, Gonzalez MA, Martin W, Saint-Aubin C. From genome to vaccine: in silico predictions, ex vivo verification. Vaccine 2001; 19:4385-95; PMID:11483263; http://dx.doi.org/10.1016/S0264-410X(01)00145-1
- Ribeiro JM, Alarcon-Chaidez F, Francischetti IM, Mans BJ, Mather TN, Valenzuela JG, Wikel SK. An annotated catalog of salivary gland transcripts from Ixodes scapularis ticks. Insect Biochem Mol Biol 2006; 36:111-29; PMID:16431279; http://dx.doi.org/10.1016/j.ibmb.2005.11.005
- Francischetti IM, Mather TN, Ribeiro JM. Cloning of a salivary gland metalloprotease and characterization of gelatinase and fibrin(ogen)lytic activities in the saliva of the Lyme disease tick vector Ixodes scapularis. Biochem Biophys Res Commun 2003; 305:869-75; PMID:12767911; http://dx.doi.org/10.1016/S0006-291X(03)00857-X
- Maritz-Olivier C, Stutzer C, Jongejan F, Neitz AWH, Gaspar ARM. Tick anti-hemostatics: targets for future vaccines and therapeutics. Trends Parasitol 2007; 23:397-407; PMID:17656153; http://dx.doi.org/10.1016/j.pt.2007.07.005
- Moise L, Terry F, Ardito M, Tassone R, Latimer H, Boyle C, Martin WD, De Groot AS. Universal H1N1 influenza vaccine development: identification of consensus class II hemagglutinin and neuraminidase epitopes derived from strains circulating between 1980 and 2011. Hum Vaccines Immunother 2013; 9:1598-607; PMID:23846304; http://dx.doi.org/10.4161/hv.25598
- Moise L, Tassone R, Latimer H, Terry F, Levitz L, Haran JP, Ross TM, Boyle CM, Martin WD, De Groot AS. Immunization with cross-conserved H1N1 influenza CD4+ T-cell epitopes lowers viral burden in HLA DR3 transgenic mice. Hum Vaccines Immunother 2013; 9:2060-8; PMID:24045788; http://dx.doi.org/10.4161/hv.26511
- De Groot AS, Martin W. Reducing risk, improving outcomes: bioengineering less immunogenic protein therapeutics. Clin Immunol (Orlando, Fla) 2009; 131:189-201; PMID:19269256; http://dx.doi.org/10.1016/j.clim.2009.01.009
- Trager W. Acquired immunity to ticks. J Parasitol 1939; 25:57-81; http://dx.doi.org/10.2307/3272160
- Wikel SK. Host immunity to ticks. Annu Rev Entomol 1996; 41:1-22; PMID:8546443; http://dx.doi.org/10.1146/annurev.en.41.010196.000245
- Heinze DM, Wikel SK, Thangamani S, Alarcon-Chaidez FJ. Transcriptional profiling of the murine cutaneous response during initial and subsequent infestations with Ixodes scapularis nymphs. Parasit Vectors 2012; 5:26; PMID:22309607; http://dx.doi.org/10.1186/1756-3305-5-26
- Mejri N, Franscini N, Rutti B, Brossard M. Th2 polarization of the immune response of BALB/c mice to Ixodes ricinus instars, importance of several antigens in activation of specific Th2 subpopulations. Parasite Immunol 2001; 23:61-9; PMID:11240897; http://dx.doi.org/10.1046/j.1365-3024.2001.00356.x
- Ferreira BR, Silva JS. Successive tick infestations selectively promote a T-helper 2 cytokine profile in mice. Immunology 1999; 96:434-9; PMID:10233725; http://dx.doi.org/10.1046/j.1365-2567.1999.00683.x
- Schoeler GB, Manweiler SA, Wikel SK. Cytokine responses of C3H/HeN mice infested with Ixodes scapularis or Ixodes pacificus nymphs. Parasite Immunol 2000; 22:31-40; PMID:10607289; http://dx.doi.org/10.1046/j.1365-3024.2000.00272.x
- Borský I, Hermánek Jí, Uhlír J, Dusbábek Fe. Humoral and cellular immune response of BALB/c mice to repeated infestations with Ixodes ricinus nymphs. Int J Parasitol 1994; 24:127-32; PMID:8021100; http://dx.doi.org/10.1016/0020-7519(94)90066-3
- Mbow ML, Christe M, Rutti B, Brossard M. Absence of acquired resistance to nymphal Ixodes ricinus ticks in BALB/c mice developing cutaneous reactions. J Parasitol 1994; 80:81-7; PMID:8308662; http://dx.doi.org/10.2307/3283349
- Christe M, Rutti B, Brossard M. Influence of the genetic background and parasite load of mice on the immune response developed against nymphs of Ixodes ricinus. Parasitol Res 1999; 85:557-61; PMID:10382605; http://dx.doi.org/10.1007/s004360050595
- Narasimhan S, Deponte K, Marcantonio N, Liang X, Royce TE, Nelson KF, Booth CJ, Koski B, Anderson JF, Kantor F, et al. Immunity against Ixodes scapularis salivary proteins expressed within 24 hours of attachment thwarts tick feeding and impairs Borrelia transmission. PloS One 2007; 2:e451; PMID:17505544; http://dx.doi.org/10.1371/journal.pone.0000451
- Bechara GH, Szabo MP, Mukai LS, Rosa PC. Immunisation of dogs, hamsters and guinea pigs against Rhipicephalus sanguineus using crude unfed adult tick extracts. Vet Parasitol 1994; 52:79-90; PMID:8030191; http://dx.doi.org/10.1016/0304-4017(94)90038-8
- Titus RG, Bishop JV, Mejia JS. The immunomodulatory factors of arthropod saliva and the potential for these factors to serve as vaccine targets to prevent pathogen transmission. Parasite Immunol 2006; 28:131-41; PMID:16542315
- Ferreira BR, Silva JS. Saliva of Rhipicephalus sanguineus tick impairs T cell proliferation and IFN-γ-induced macrophage microbicidal activity. Vet Immunol Immunopathol 1998; 64:279-93; PMID:9730222; http://dx.doi.org/10.1016/S0165-2427(98)00135-4
- Moise L, Gutierrez AH, Bailey-Kellogg C, Terry F, Leng Q, Abdel Hady KM, Verberkmoes NC, Sztein MB, Losikoff PT, Martin WD, et al. The two-faced T cell epitope: examining the host-microbe interface with JanusMatrix. Hum Vaccines Immunother 2013; 9:1577-86; PMID:23584251; http://dx.doi.org/10.4161/hv.24615
- Moise L, Buller RM, Schriewer J, Lee J, Frey SE, Weiner DB, Martin W, De Groot AS. VennVax, a DNA-prime, peptide-boost multi-T-cell epitope poxvirus vaccine, induces protective immunity against vaccinia infection by T cell response alone. Vaccine 2011; 29:501-11; PMID:21055490; http://dx.doi.org/10.1016/j.vaccine.2010.10.064
- Moss SF, Moise L, Lee DS, Kim W, Zhang S, Lee J, Rogers AB, Martin W, De Groot AS. HelicoVax: epitope-based therapeutic Helicobacter pylori vaccination in a mouse model. Vaccine 2011; 29:2085-91; PMID:21236233; http://dx.doi.org/10.1016/j.vaccine.2010.12.130
- Sturniolo T, Bono E, Ding J, Raddrizzani L, Tuereci O, Sahin U, Braxenthaler M, Gallazzi F, Protti MP, Sinigaglia F, et al. Generation of tissue-specific and promiscuous HLA ligand databases using DNA microarrays and virtual HLA class II matrices. Nat Biotechnol 1999; 17:555-61; PMID:10385319; http://dx.doi.org/10.1038/9858
- Bond KB, Sriwanthana B, Hodge TW, De Groot AS, Mastro TD, Young NL, Promadej N, Altman JD, Limpakarnjanarat K, McNicholl JM. An HLA-directed molecular and bioinformatics approach identifies new HLA-A11 HIV-1 subtype E cytotoxic T lymphocyte epitopes in HIV-1-infected Thais. AIDS Res Hum Retrov 2001; 17:703-17; PMID:11429111; http://dx.doi.org/10.1089/088922201750236988
- Dong Y, Demaria S, Sun X, Santori FR, Jesdale BM, De Groot AS, Rom WN, Bushkin Y. HLA-A2-restricted CD8+-cytotoxic-T-cell responses to novel epitopes in Mycobacterium tuberculosis superoxide dismutase, alanine dehydrogenase, and glutamine synthetase. Infect Immun 2004; 72:2412-5; PMID:15039371; http://dx.doi.org/10.1128/IAI.72.4.2412-2415.2004
- Steere AC, Klitz W, Drouin EE, Falk BA, Kwok WW, Nepom GT, Baxter-Lowe LA. Antibiotic-refractory Lyme arthritis is associated with HLA-DR molecules that bind a Borrelia burgdorferi peptide. J Exp Med 2006; 203:961-71; PMID:16585267; http://dx.doi.org/10.1084/jem.20052471
- Southwood S, Sidney J, Kondo A, del Guercio MF, Appella E, Hoffman S, Kubo RT, Chesnut RW, Grey HM, Sette A. Several common HLA-DR types share largely overlapping peptide binding repertoires. J Immunol (Baltimore, Md: 1950) 1998; 160:3363-73; PMID:9531296
- Kong YC, Lomo LC, Motte RW, Giraldo AA, Baisch J, Strauss G, Hämmerling GJ, David CS. HLA-DRB1 polymorphism determines susceptibility to autoimmune thyroiditis in transgenic mice: definitive association with HLA-DRB1*0301 (DR3) gene. J Exp Med 1996; 184:1167-72; PMID:9064334; http://dx.doi.org/10.1084/jem.184.3.1167
- Strauss G, Vignali DA, Schonrich G, Hammerling GJ. Negative and positive selection by HLA-DR3(DRw17) molecules in transgenic mice. Immunogenetics 1994; 40:104-8; PMID:8026858
- Cosgrove D, Gray D, Dierich A, Kaufman J, Lemeur M, Benoist C, Mathis D. Mice lacking MHC class II molecules. Cell 1991; 66:1051-66; PMID:1909605; http://dx.doi.org/10.1016/0092-8674(91)90448-8
- Nabozny GH, Baisch JM, Cheng S, Cosgrove D, Griffiths MM, Luthra HS, David CS. HLA-DQ8 transgenic mice are highly susceptible to collagen-induced arthritis: a novel model for human polyarthritis. J Exp Med 1996; 183:27-37; PMID:8551230; http://dx.doi.org/10.1084/jem.183.1.27
- Yang L, Weis JH, Eichwald E, Kolbert CP, Persing DH, Weis JJ. Heritable susceptibility to severe Borrelia burgdorferi-induced arthritis is dominant and is associated with persistence of large numbers of spirochetes in tissues. Infect Immun 1994; 62:492-500; PMID:8300208
- Morrison TB, Ma Y, Weis JH, Weis JJ. Rapid and sensitive quantification of borrelia burgdorferi-infected mouse tissues by continuous fluorescent monitoring of PCR. J Clin Microbiol 1999; 37:987-92; PMID:10074514