Abstract
While most pathogens infect via mucosal surfaces, most current vaccines are delivered by injection. This situation remains despite awareness of the potential benefits of mucosal delivery for inducing protection against mucosa-infecting pathogens. A major obstacle to the development of such vaccines is the paucity of safe and effective adjuvants that induce mucosal responses in non-rodents. Previously we demonstrated in sheep the potency of pulmonary-delivered influenza ISCOMATRIX™ vaccine, which induces both mucosal and systemic immunity, even with low antigen doses. In the current study, lung pre-exposure to influenza antigen alone significantly reduced the immune response to subsequent pulmonary-delivered influenza ISCOMATRIX™ vaccine. A single dose of influenza antigen, delivered to the lung without exogenous adjuvant, upregulated IL-10 expression in bronchoalveolar lavage cells and FOXP3 expression in lung tissue, suggestive of induction of a regulatory T cell (Treg) response. However, this effect was inhibited by addition of ISCOMATRIX™ adjuvant. Moreover, effective pulmonary immunization with influenza ISCOMATRIX™ vaccine was associated with a depletion of Treg markers within lung tissues. Lung exposure to influenza antigen induced a localized mucosal tolerance that reduced the efficacy of subsequent influenza ISCOMATRIX™ vaccination. An important role of ISCOMATRIX™ adjuvant in pulmonary vaccination appears to be the depletion of Treg in lung tissues. Pulmonary vaccination remains capable of inducing a strong immune response against mucosal pathogens, but likely requires an adjuvant to overcome mucosal tolerance. ISCOMATRIX™ appears to have considerable potential as a mucosal adjuvant for use in humans, a major unmet need in mucosal vaccine development.
Introduction
Despite the fact that most pathogens initially infect the host via mucosal surfaces, the vast majority of current vaccines are still delivered by injection and hence induce predominantly systemic immunity. While parenterally delivered vaccines can provide protection against mucosal pathogens, studies have shown that vaccinations at mucosal sites can generate more efficient local immune responses.Citation1,2 These observations have driven efforts to develop effective strategies for mucosally-delivered vaccines, with mixed success. Such approaches may be particularly important in efforts against most of the key pathogens for which we have so far failed to produce an effective vaccine, including human immunodeficiency virus or against which improved vaccines are greatly needed, such as for Mycobacterium tuberculosis.
It has long been recognized that there exist important differences between the induction and in particular the regulation of immune responses at mucosal surfaces, as compared to those relating to systemic immunity.Citation3 For example, continuous exposure of the lungs to inhaled environmental allergens means there is a need for a system to prevent inappropriate inflammation and hypersensitivity reactions.Citation4 Such tolerance mechanisms are potentially a key impediment to the development of effective mucosal vaccines, as this tolerance may need to be overcome for the induction of a protective immune response.Citation2,5,6
A number of studies by ourselves and others have demonstrated that vaccine delivery via the pulmonary route has the potential to induce both mucosal and systemic immune responses.Citation7-10 Aerosol vaccination of human papillomavirus type 16 virus like particles into the lower airways of humans induced both mucosal and serum antibody titers comparable to those induced by intramuscular vaccination.Citation8 Our group has previously demonstrated that deep lung delivery of an influenza ISCOMATRIX™ vaccine resulted in a strong combined mucosal and systemic immune response in sheep.Citation10 Sheep were used for these studies as they provide advantages from their size, plus their lungs are physiologically and immunologically more similar to those of humans than are rodent lungs.Citation11 The potency of this response was demonstrated by the provision for a substantial reduction in antigen dose. Specifically, lung vaccination with an influenza ISCOMATRIX™ vaccine induced a comparable response to a subcutaneously injected influenza vaccine containing 375-fold more antigen.Citation10
We later showed that pulmonary delivery of an influenza ISCOMATRIX™ vaccine induced a systemic memory recall response following influenza antigen challenge for up to one year.Citation12 This demonstrated that these vaccinations induced an effective systemic memory response that would last for at least the interval between annual influenza vaccinations.
Here, in an extension of that study, we subsequently re-immunized these same sheep with influenza ISCOMATRIX™ vaccine. Surprisingly, we noted that animals previously challenged in the lung with non-adjuvanted influenza antigen had a poor local mucosal response despite a normal systemic response, suggestive of mucosal tolerance. This observation prompted the studies presented in this paper, whereby we demonstrate that delivery of influenza vaccine antigen to the lung without adjuvant induces increased IL-10 secretion and recruitment of FOXP3+ cells to the lung, while the addition of ISCOMATRIX™ adjuvant reduces regulatory T cell (Treg) levels, a feature that correlates with the functional activity of this vaccine.
Results
Prior lung exposure to non-adjuvanted influenza antigen reduces the efficacy of lung-delivered influenza ISCOMATRIX™ vaccine
In a published study, the longevity of the immune response induced by pulmonary delivery of an influenza ISCOMATRIX™ vaccine was examined by vaccinating sheep 3 times, followed by influenza antigen challenge into the lung either 6 or 12 months later.Citation12 Sheep challenged 6 months after vaccination had a detectable memory recall response in both the lungs and sera, while sheep challenged 12 months post-immunization only had a detectable memory antibody response in their sera.Citation12
Here, in an extension of this study, 18 months after the initial immunization these same sheep were subsequently revaccinated in the lung with influenza ISCOMATRIX™ vaccine (Study 1; Table 1), then antigen-specific antibodies in sera and BAL fluid were quantified (). As no difference in antibody response was observed between vaccinated sheep challenged with antigen (without adjuvant) at 6 or 12 months (not shown), data from these animals were combined. Revaccination via the pulmonary route 18 months after the initial immunizations with influenza ISCOMATRIX™ vaccine successfully induced a systemic antibody response, as demonstrated by a significant increase in influenza-specific serum IgA and IgG (). Unexpectedly, and in contrast to numerous previous experiments,Citation10,12,13 there was no concordant increase in influenza-specific antibodies in BAL fluid (), despite these vaccinations being delivered to the lung.
Figure 1. Lack of a vaccine-induced lung antibody response in sheep immunized with influenza ISCOMATRIX™ vaccine and challenged intralung with influenza antigen. Study 1: A group of sheep (n = 11) previously vaccinated 3 times via the lung with influenza ISCOMATRIX™ vaccine then challenged with influenza antigen at either 6 or 12 months later,Citation12 were revaccinated via the lungs 18 months after the initial immunizations. Influenza specific antibodies in sera and lung washings collected one week prior to antigen challenge (pre) and one and 4 weeks after antigen challenge were analyzed by ELISA. Age and sex-matched control sheep were sham vaccinated with PBS (n = 12). Boxplots present the median (horizontal bar), interquartile range (box) and range (bars). *ANOVA with Dunnett's post-hoc analysis.
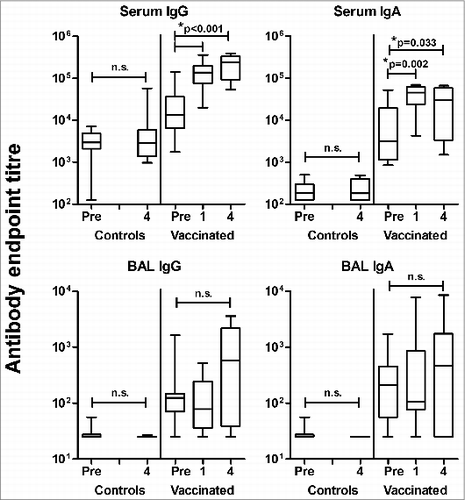
A key difference between this current observation and all our previous studies was exposure of the lung to non-adjuvanted antigen, suggesting that antigen challenge in the lung without adjuvant may result in a localized tolerogenic effect that inhibits the secretion of vaccine-induced antibodies in the lung mucosa. However, the complexity of this initial study meant further experimentation was necessary to support such an interpretation.
In a subsequent study with a different set of influenza naïve sheep, we therefore sought to determine whether pre-exposure of the lung to influenza antigen would impact upon the efficacy of a subsequent influenza ISCOMATRIX™ vaccine when delivered via the pulmonary route (Study 2; Table1). A single dose of non-adjuvanted influenza antigen delivered to the sheep lung induced a specific antibody response in BAL but not sera, as compared to untreated controls (). Commencing three weeks after antigen exposure, half of the animals received 3 influenza ISCOMATRIX™ vaccinations delivered via the deep lung. While these vaccinations induced influenza-specific IgA and IgG responses in both sera and BAL fluid of all animals, those pre-exposed to non-adjuvanted influenza antigen via the lung prior to vaccination showed significantly reduced local and systemic antibody responses (). Notably, this included a reduced functional activity of this antibody response as shown by lower serum haemagglutination inhibition (HAI) titers ().
Figure 2. Antigen pre-exposure in the lung is locally immunogenic but reduces the efficacy of subsequent pulmonary influenza ISCOMATRIX™ vaccination. Study 2: (A) Influenza antigen (15 μg) alone was delivered into the lungs of sheep (n = 16). Control sheep (n = 16) were left untreated. Sera and BAL samples collected one week before and 2 weeks (optimal timepoint for measuring priming responses) after antigen delivery were analyzed for influenza-specific antibodies by ELISA. Endpoint titers are shown from post-antigen exposure samples, with titers from pre-exposure samples subtracted. (B) Commencing 3 weeks after influenza antigen exposure in the lung, half of the treated and control groups (n = 8) received 3 influenza ISCOMATRIX™ vaccinations, delivered 3 weeks apart. Sera and BAL samples were collected from all animals one week before and after the final vaccination (optimal timepoint for measuring booster responses) and analyzed for influenza-specific antibodies by ELISA. Endpoint titers shown are from post-vaccination samples with titers from pre-exposure samples subtracted. (C) Neutralizing activity on post-vaccination samples was determined by HAI assay. **P < 0.01; ***P ≤ 0.001 compared with unvaccinated controls (ANOVA).
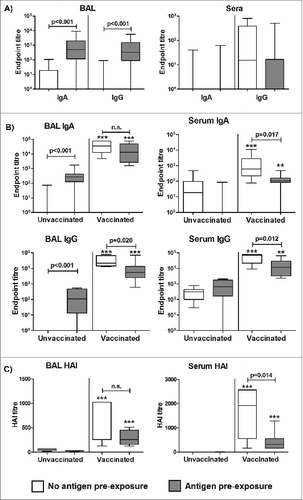
ISCOMATRIX™ adjuvant inhibits the pulmonary FOXP3+ response to lung-delivered antigen
FOXP3+ regulatory T cells are believed to play an important role in immune tolerance in the lung.Citation14 We therefore examined whether the reduced vaccine-induced antibody response resulting from prior exposure to influenza antigen in the lung was FOXP3 associated. First, we examined FOXP3 expression by BAL cells. There was no detectable effect of influenza antigen exposure in the lung on FOXP3 expression by BAL cells collected one week after delivery of this antigen () or after the third vaccination (). Interestingly however, pulmonary influenza ISCOMATRIX™ vaccinations produced a significant decrease in FOXP3 expression. This effect did not appear to be influenced by prior antigen-exposure; while the reduction in FOXP3 in the control group which did not receive antigen pre-exposure did not quite reach significance, it had the same pattern and almost identical median values as the antigen-treated group ().
Figure 3. Reduced FOXP3 expression in BAL cells following pulmonary influenza ISCOMATRIX™ vaccinations. Study 2: (A) BAL cells were collected from sheep 1 week after exposure to influenza antigen alone via the lung and a matching group that received no antigen (n = 16). (B) Half of each group received 3 influenza ISCOMATRIX™ vaccinations (Vax) while the other half were left unvaccinated (Con). BAL cells were collected one week after the third vaccination. FOXP3 levels in BAL cells were quantified by qPCR relative to GAPDH (*ANOVA).
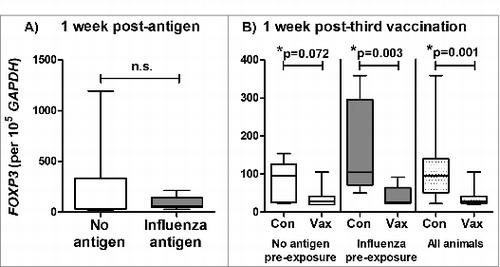
We speculated that one week post-exposure may be too late to observe the key events involved in the response to antigen and vaccine delivery to the lung and that we may be missing important events occurring in the lung tissues. To better characterize the above observation, we therefore performed a third study where BAL cells and lung tissue samples were collected from groups of sheep one day after receiving either influenza antigen alone or influenza ISCOMATRIX™ vaccine, delivered to the deep lung.
Delivery of influenza antigen into the deep lung induced a significant increase in the total number of cells present in BAL (). Morphological examination of these cells revealed the increase in cell numbers to be primarily the result of increased neutrophil infiltration (). This infiltration occurred irrespective of the addition of ISCOMATRIX™ adjuvant, indicating the neutrophils were recruited in response to the influenza antigen.
Figure 4. BAL cell composition following lung delivery of influenza antigen or influenza ISCOMATRIX™ vaccine. Study 3: Groups of sheep (n = 8) were left untreated or dosed via the lung with 3 doses of either influenza antigen alone or influenza ISCOMATRIX vaccine, spaced by 3 weeks. One day after the first and third dose, BAL cells were collected from the lungs by endoscopy, stained and counted. (A) Total counts of cells recovered. (B) Relative numbers of macrophages, neutrophils and lymphocytes (%). **P < 0.01; ***P < 0.001 compared to untreated animals.
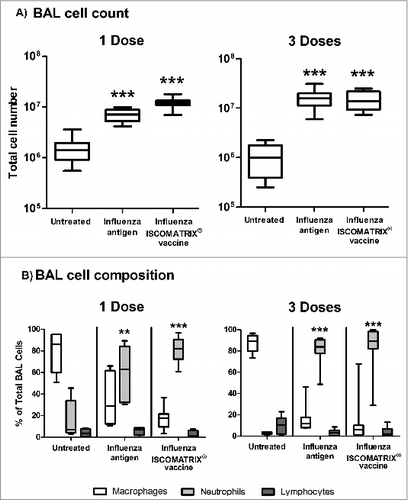
Gene expression analyses of these same animals revealed that a single dose of influenza antigen alone induced: i) a significant increase of FOXP3 in lung tissue with a trend toward increased CD4 expression, and ii) a significant increase in IL-10, but not FOXP3 or CD4 expression in BAL cells (). These effects were prevented when ISCOMATRIX™ adjuvant was added to the influenza antigen. There was no evidence of an increase in Treg recruitment into the lung tissue following 3 doses of influenza antigen, although there was a non-significant trend toward increased FOXP3, CD4 and IL-10 expression in BAL cells in this group ().
Figure 5. Expression of regulatory T cell markers 1 day after lung delivery of influenza antigen or influenza ISCOMATRIX™ vaccine. Study 3: Groups of sheep (n = 8) were left untreated or dosed via the lung with 3 doses of either influenza antigen alone or influenza ISCOMATRIX™ vaccine, spaced by 3 weeks. One day after the first and third dose, BAL cells and lung tissue samples were analyzed for FOXP3, CD4 and IL-10 by qPCR. *ANOVA.
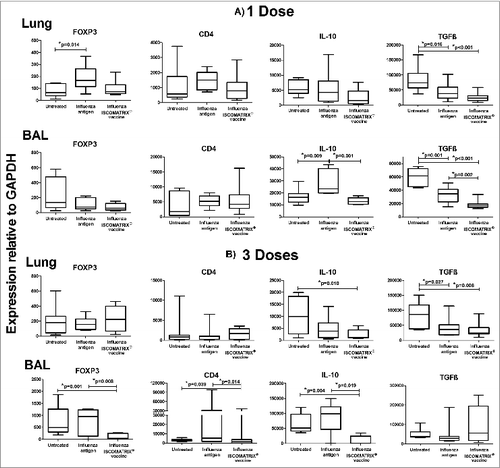
We also measured TGFß, a cytokine commonly involved in Treg development. This showed that influenza antigen typically caused a reduction in lung TGFß levels (). This occurred both with or without ISCOMATRIX™ adjuvant, though addition of ISCOMATRIX™ adjuvant did appear to further reduce lung TGFß levels. These reduced TGFß levels suggest the Treg response to influenza may not develop in the lungs themselves.
We have previously shown that 3 doses of pulmonary delivered influenza ISCOMATRIX™ vaccine are required for the full induction of immunity in sheep.Citation10 It was therefore interesting to observe that not one, but 3 doses of ISCOMATRIX™-adjuvanted influenza antigen significantly reduced levels of the Treg markers FOXP3, CD4 and IL-10 in BAL cells even below the basal levels present in unvaccinated controls ().
We believe the combined reduction in these markers is strongly suggestive of a reduction in Tregs. This reduction was not an artifact resulting from the diluent effect of increased infiltration by other cells, as reduced levels of Treg markers only occurred following 3 doses of vaccine, whereas increased cell infiltrate was observed for influenza antigen with and without adjuvant, as well as following both one and 3 doses of the influenza ISCOMATRIX™ vaccine ().
To further analyze the potential effect of 3 doses of influenza ISCOMATRIX™ vaccine on Treg levels in BAL cells, we plotted CD4 against FOXP3 expression (). While influenza antigen alone did not produce a significant change in the distribution of the CD4:FOXP3 plot, 5/8 sheep vaccinated with influenza plus IMX animals had no detectable FOXP3. Of the 5 sheep with no detectable FOXP3, 3/5 also had no detectable CD4 (). Interestingly, all 5 sheep that received influenza ISCOMATRIX™ vaccine and which had no detectable FOXP3, also had no detectable expression of IL-10, whereas all 3 animals from the same group which did express FOXP3, still had IL-10 expression (these data are included in ). This diverse response in the sheep is not unusual given the highly outbred nature of these animals. Hence the expression of IL-10, a key mediator of mucosal tolerance, was suppressed in influenza ISCOMATRIX™ vaccinated animals in which FOXP3 expression was suppressed, irrespective of their CD4 (and therefore T effector) status.
Discussion
Delivery of vaccines via mucosal routes can confer several advantages over injected vaccines. However, the efficacy of such vaccine delivery routes is frequently compromised by tolerance mechanisms that exist to protect mucosal surfaces from inappropriate inflammatory responses upon exposure to ingested or inhaled antigens.Citation4 In this study we have made several observations relating to the suppression of functional antibody responses in the lung following vaccine antigen exposure and how this is overcome by the addition of an adjuvant.
First, we have shown that the initial exposure of the lungs of naïve animals to influenza antigen alone induces the upregulation of IL-10 by BAL cells and FOXP3 in lung tissues. Alveolar macrophages express the IL-10 receptor and increased IL-10 secretion is known to be a potent negative regulator of alveolar macrophage activity.Citation15 Increased FOXP3, as well as a trend toward increased CD4 levels (), suggest influenza antigen alone also induced either the development or migration of Treg into lung tissues. The reduction of TGFß in the lung tissue and BAL suggests the latter is perhaps the most likely. Treg have been shown to be important modulators of allergic responses in the lung,Citation16 with this function involving direct Treg activity in the lung tissues, rather than for example, the inhibition of immune cell activity in draining lymph nodes.Citation17 Further, it was recently shown that, under conditions of airway tolerance, resident macrophages induce the development of Treg in lung tissues.Citation14 Hence, the delivery of a single dose of influenza antigen appeared to result in activation of several key tolerizing events. Interestingly however, the same events did not occur after 3 doses. When antigen was delivered for the first time, the animals were influenza naïve and so the lungs did not contain any influenza-specific antibodies. However, the third dose was delivered into non-naïve animals and anti-influenza antibodies present in the lung fluids () would bind and potentially remove the antigen before it was able to exert its tolerizing effect.
A second observation is that the tolerizing events induced by influenza antigen alone were inhibited by the addition of ISCOMATRIX™ adjuvant. More importantly we demonstrated that influenza ISCOMATRIX™ vaccine inhibited Treg in the lung below baseline levels present in control sheep (shown by suppression of FOXP3 and IL-10 expression). Thus not only was the induction of an increased Treg response to co-delivered antigen prevented, but the intrinsic Treg levels already present in the lung were actively reduced. Intriguingly this effect only occurred after 3 vaccine doses, which correlates with the induction of a strong antibody response to intra-lung delivered vaccine,Citation10 and only occurred when ISCOMATRIX™ adjuvant was added to the antigen. Hence the reduction in intrinsic Treg levels by this ISCOMATRIX™ adjuvanted vaccine appears to be associated with its ability to induce a potent immune response. The role of an adjuvant is to stimulate an immune response, and therefore the addition of an adjuvant has the potential to induce the infiltration of other cell populations into the lung tissue that might complicate interpretation of these gene expression studies. However, as safety is of concern for any vaccines, we have very closely monitored the tissues of animals vaccinated via the pulmonary route. In numerous similar experiments performed over many years, we have never observed a significant cellular infiltration into the lung tissues of recipient animals (data not shown), suggesting the infiltration of cells such as granulocytes into the lung tissues is unlikely to impact on these results.
Third, we found that prior exposure to influenza antigen in the lung reduced the immune response generated after subsequent pulmonary immunization with influenza ISCOMATRIX™ vaccine. This indicates that the induction of mucosal tolerance by previous antigenic exposure in the lung can have a detrimental effect on the efficacy of pulmonary vaccinations. That said, the inhibitory effect was only partial as the vaccination still induced an antibody response including neutralizing activity, albeit to a lower level. Perhaps the most important point in this regard is that such a scenario is unlikely to occur in humans, at least for influenza. Indeed, the influenza antigen used in these studies was killed split (chemically-disrupted) virus and, as such, is incapable of infecting the host and while possessing some innate activating components, does not provide the same danger signals as a live pathogenic viral infection. Human lungs are not exposed to killed influenza but rather to live infections which, rather than being tolerizing, would boost immunity. Some live lung infections, such as Streptococcus pneumonia have been shown to modify the inflammatory response in the lung by induction of Tregs, increased numbers of which were identified in lymph nodes.Citation18 It is currently unknown what effect infections that induce lung Tregs would have on the efficacy of an influenza ISCOMATRIX™ vaccine.
It has been shown previously that lung exposure to an antigen in the absence of adjuvant induces T cell tolerance, thereby reducing the immune response generated.Citation19 It is therefore likely that an adjuvant is required to circumvent such a tolerance event for effective pulmonary vaccination. However such vaccine studies typically use adjuvants such as cholera toxin, which are unlikely to be of utility in humans due to toxicity concerns. ISCOMATRIX™ adjuvant is currently in clinical development and has thus far proven to be safe and effective in numerous clinical trials.Citation20 The suggestion that addition of ISCOMATRIX™ adjuvant inhibits antigen-induced tolerance mechanism in the lung supports its potential as a mucosal adjuvant. It also suggests at least one possible role of ISCOMATRIX™ adjuvant in pulmonary vaccination is to overcome mucosal tolerance.
Taken together, we have shown that exposure of the lung to killed influenza viral antigen reduces the efficacy of an influenza ISCOMATRIX™ vaccine, most likely by the induction of a localized mucosal tolerance. Pulmonary vaccination remains a delivery method that is capable of inducing both a strong mucosal and systemic immune response against mucosal pathogens. However, in order for this delivery method to generate an immune response, especially at the site of mucosal delivery, an adjuvant is essential to overcome mucosal tolerance. ISCOMATRIX™ appears to have considerable potential as a mucosal adjuvant for use in humans, a major unmet need in mucosal vaccine development. The evidence presented in this study suggests the mechanism of action of a pulmonary-delivered influenza ISCOMATRIX™ vaccine may include or require the suppression of Treg levels in the lung, and that vaccine-induced immunity via the lung is still possible even in the presence of mucosal tolerance.
Materials and Methods
Animals
Female Merino sheep were housed in pens within the School of Veterinary Science animal facility, the University of Melbourne, Parkville. Sheep were fed lucerne chaff mixed with commercial pellets and allowed access to water ad libidum. All experimental procedures were approved by the Veterinary Science Animal Experimentation Ethics Committee of the University of Melbourne.
Pulmonary vaccinations
Influenza antigen used was sucrose gradient purified A/Solomon Island/03/2006 H1N1 (studies 1 and 2) or B/Brisbane/60/08 H1N1 (study 3) virus, which had been inactivated and detergent disrupted. Antigen concentration was based on hemagglutinin content and determined by single radial immunodiffusion. Good manufacturing practice (GMP)-grade ISCOMATRIX™ adjuvant was prepared by CSL Limited as previously described.Citation21 The influenza ISCOMATRIX™ vaccine used in this study consisted of 15 μg influenza antigen and 75 ISCO units of ISCOMATRIX™ adjuvant.
For pulmonary immunizations, sheep were restrained in a harness and a bronchoscope (Pentax 16FG), lubricated with lignocaine gel (Orion, LIG01829F), inserted via the nostril to the lower left caudal lobe of the lung. Solutions were infused in a total volume of 5 mL of phosphate buffered saline (PBS), followed by 10 mL of air to ensure complete delivery.
Blood (10 mL) was collected from the jugular vein using an 18G needle with syringe, then left to coagulate for collection of sera. For collection of BAL, 10 mL PBS was delivered into the left caudal lobe of the lung via a bronchoscope and then immediately withdrawn. Lung tissue samples were collected from the lower left caudal lobe, post mortem. Samples were stored at −20°C until analyzed.
Antibody ELISA
Antigen-specific antibodies in BAL and serum samples were measured by enzyme-linked immunosorbent assay (ELISA). Briefly, 96 well Maxisorp flat bottom plates (NUNC, 44-2404-21) were coated overnight with 50 μL of 10 μg/mL antigen in carbonate buffer, pH 9.6. Plates were then blocked with 1% sodium casein before adding 100 μL of 1 in 5 serial dilutions of samples in duplicates. Binding of antigen-specific antibodies was detected using rabbit anti-sheep IgG conjugated with horse radish peroxidase (Southern Biotech, 6150-05) or anti-bovine/ovine IgA (AbD Serotech, MCA2438) followed by rabbit anti-mouse horse radish peroxidase (Dako, P0260). Color was developed by addition of tetramethylbenzidine (TMB) substrate (Invitrogen, 00-2023), stopped by the addition of 2M H2SO4, optical density at 450 nm was determined on a Bio-Tek ELx800 plate reader and endpoint titers calculated.
HAI titer
The titer of functional antibodies was determined by measuring the inhibition of red blood cell agglutination by influenza virus through a HAI assay. Serum and BAL fluid samples were tested for HAI activity against egg-grown A/Solomon Island/03/2006 virus (H1N1) using turkey red blood cells. The HAI titer was determined as the dilution of sample that inhibited influenza virus agglutination of red blood cells.
Quantitative PCR
RNA was extracted from BAL cells using a Qiagen RNeasy Plus Mini Kit (74134), and from tissue samples using TRI Reagent® (Ambion, AM9738) according to manufacturer's instructions. RNA was stored at −80°C. Extracted RNA was converted to cDNA using a Qiagen QuantiTect Reverse Transcription Kit (205319, as per manufacturer's protocol). cDNA was diluted 2:15 with TE and stored at −20°C until analyzed.
Table 1. Experimental design
Gene expression was measured using the Mx3005P QPCR System (Agilent Technologies). All reactions were carried out in 96-well plates. 2 μL of cDNA was mixed with 12.5 μL GoTaq® qPCR Master Mix (Promega, A6001), 0.2 μM primers in 2 μL and 8.5 μL H2O for a final reaction volume of 25 μL. Primers were designed based on the listed NCBI Reference Sequence using Primer-Blast (NCBI) and are shown in .
Table 2. Primers
Cycling conditions: initial denaturation of 95°C for 10 minutes; 45 cycles of 95°C for 30 seconds, 60°C for 30 seconds and 72°C for 30 seconds, followed by 95°C for 1 minute, 60°C for 30 seconds, and 95°C for 30 seconds. Results were analyzed using the MxPro Software (Stratagene) and LinRegPCR Software (J. M. Ruitger).
Differential BAL cell counts
BAL cells were attached to a microscope slide using a cytospin (Shandon, Thermo Fisher Scientific), then allowed to air dry overnight. Slides were fixed in methanol for 2 minutes, allowed to air-dry then stained with Haem-Quick (Grale HDS, CP-L-HAEMKWIK-00). Neutrophils, macrophages and lymphocytes were counted on slides and evaluated as a percentage of total BAL cell counts.
Statistical analyses
Data were normalized by log-transformation than analyzed by one way analysis of variance (ANOVA).
Disclosure of Potential Conflicts of Interest
MJP is an employee of CSL Limited.
Funding
This work was supported by the Victorian Government's Operational Infrastructure Support Program and by Linkage Project grant LP100200728 from the Australian Research Council of Australia. PS is supported by a Senior Research Fellowship from the National Health and Medical Research Council of Australia.
References
- Brandtzaeg P. Induction of secretory immunity and memory at mucosal surfaces. Vaccine 2007; 25:5467-84; PMID:17227687; http://dx.doi.org/10.1016/j.vaccine.2006.12.001
- Ogra PL, Faden H, Welliver RC. Vaccination strategies for mucosal immune responses. Clin Microbiol Rev 2001; 14:430-+; PMID:11292646; http://dx.doi.org/10.1128/CMR.14.2.430-445.2001
- Hu W, Pasare C. Location, location, location: tissue-specific regulation of immune responses. J Leukocyte Biol 2013; 94:409-21; PMID:23825388; http://dx.doi.org/10.1189/jlb.0413207
- Curotto de Lafaille MA, Lafaille JJ, Graca L. Mechanisms of tolerance and allergic sensitization in the airways and the lungs. Curr Opin Immunol 2010; 22:616-22; PMID:20884192; http://dx.doi.org/10.1016/j.coi.2010.08.014
- Chen K, Cerutti A. Vaccination strategies to promote mucosal antibody responses. Immunity 2010; 33:479-91; PMID:21029959; http://dx.doi.org/10.1016/j.immuni.2010.09.013
- Mestecky J, Russell MW, Elson CO. Perspectives on mucosal vaccines: is mucosal tolerance a barrier? J Immunol 2007; 179:5633-8; PMID:17947632; http://dx.doi.org/10.4049/jimmunol.179.9.5633
- Vujanic A, Wee JLK, Snibson KJ, Edwards S, Pearse M, Quinn C, Moloney M, Taylor S, Scheerlinck JP, Sutton P. Combined mucosal and systemic immunity following pulmonary delivery of ISCOMATRIXTM adjuvanted recombinant antigens. Vaccine 2010; 28:2593-7; PMID:20096391; http://dx.doi.org/10.1016/j.vaccine.2010.01.018
- Nardelli-Haefliger D, Lurati F, Wirthner D, Spertini F, Schiller JT, Lowy DR, Ponci F, De Grandi P. Immune responses induced by lower airway mucosal immunisation with a human papillomavirus type 16 virus-like particle vaccine. Vaccine 2005; 23:3634-41; PMID:15882523; http://dx.doi.org/10.1016/j.vaccine.2005.02.019
- Amorij JP, Saluja V, Petersen AH, Hinrichs WLJ, Huckriede A, Frijlink HW. Pulmonary delivery of an inulin-stabilized influenza subunit vaccine prepared by spray-freeze drying induces systemic, mucosal humoral as well as cell-mediated immune responses in BALB/c mice. Vaccine 2007; 25:8707-17; PMID:17996993; http://dx.doi.org/10.1016/j.vaccine.2007.10.035
- Wee JL, Scheerlinck JP, Snibson KJ, Edwards S, Pearse M, Quinn C, Sutton P. Pulmonary delivery of ISCOMATRIX influenza vaccine induces both systemic and mucosal immunity with antigen dose sparing. Mucosal Immunol 2008; 1:489-96; PMID:19079216; http://dx.doi.org/10.1038/mi.2008.59
- Scheerlinck JP, Snibson KJ, Bowles VM, Sutton P. Biomedical applications of sheep models: from asthma to vaccines. Trends Biotechnol 2008; 26:259-66; PMID:18353472; http://dx.doi.org/10.1016/j.tibtech.2008.02.002
- Vujanic A, Snibson KJ, Wee JL, Edwards SJ, Pearse MJ, Scheerlinck JP, Sutton P. Long-term antibody and immune memory response induced by pulmonary delivery of the influenza Iscomatrix vaccine. Clin Vaccine Immunol: CVI 2012; 19:79-83; PMID:22072721; http://dx.doi.org/10.1128/CVI.05265-11
- Vujanic A, Wee JL, Snibson KJ, Edwards S, Pearse M, Quinn C, Moloney M, Taylor S, Scheerlinck JP, Sutton P. Combined mucosal and systemic immunity following pulmonary delivery of ISCOMATRIX adjuvanted recombinant antigens. Vaccine 2010; 28:2593-7; PMID:20096391; http://dx.doi.org/10.1016/j.vaccine.2010.01.018
- Soroosh P, Doherty TA, Duan W, Mehta AK, Choi H, Adams YF, Mikulski Z, Khorram N, Rosenthal P, Broide DH, et al. Lung-resident tissue macrophages generate Foxp3+ regulatory T cells and promote airway tolerance. J Exp Med 2013; 210:775-88; PMID:23547101; http://dx.doi.org/10.1084/jem.20121849
- Hussell T, Bell TJ. Alveolar macrophages: plasticity in a tissue-specific context. Nat Rev Immunol 2014; 14:81-93; PMID:24445666; http://dx.doi.org/10.1038/nri3600
- Thorburn AN, Foster PS, Gibson PG, Hansbro PM. Components of Streptococcus pneumoniae suppress allergic airways disease and NKT cells by inducing regulatory T cells. J Immunol 2012; 188:4611-20; PMID:22461699; http://dx.doi.org/10.4049/jimmunol.1101299
- Lloyd CM, Murdoch JR. Tolerizing allergic responses in the lung. Mucosal Immunol 2010; 3:334-44; PMID:20505664; http://dx.doi.org/10.1038/mi.2010.19
- Preston JA, Thorburn AN, Starkey MR, Beckett EL, Horvat JC, Wade MA, O'Sullivan BJ, Thomas R, Beagley KW, Gibson PG, et al. Streptococcus pneumoniae infection suppresses allergic airways disease by inducing regulatory T-cells. Eur Respiratory J 2011; 37:53-64; PMID:20525707; http://dx.doi.org/10.1183/09031936.00049510
- Wikstrom ME, Batanero E, Smith M, Thomas JA, von Garnier C, Holt PG, Stumbles PA. Influence of mucosal adjuvants on antigen passage and CD4(+) T cell activation during the primary response to airborne allergen. J Immunol 2006; 177:913-24; PMID:16818746; http://dx.doi.org/10.4049/jimmunol.177.2.913
- Morelli AB, Becher D, Koernig S, Silva A, Drane D, Maraskovsky E. ISCOMATRIX: a novel adjuvant for use in prophylactic and therapeutic vaccines against infectious diseases. J Med Microbiol 2012; 61:935-43; PMID:22442293; http://dx.doi.org/10.1099/jmm.0.040857-0
- Drane D, Pearse M. The ISCOMATRIX adjuvant In Schijns VE, O‘Hagan DT, eds. Immunopotentiators in Modern Vaccines. Amsterdam: Boston Elsevier Academic Press, 2006.