Abstract
Conserved Streptococcus pneumoniae (Spn) proteins are currently under investigation as vaccine candidates. We recently identified a subset of children prone to frequent acute otitis media (AOM) that we refer to as stringently-defined otitis prone (sOP). We investigated the synchrony of serum antibody responses against 5 Spn protein vaccine antigens, PhtD, LytB, PcpA, PhtE, and PlyD1 resulting from nasopharyngeal colonization and AOM in sOP children (49 observations) and non-otitis prone (NOP) children (771 observations). Changes in serum IgG and IgM were quantitated with ELISA. IgG antibody concentrations against PhtD, PcpA, and PlyD1 rose in synchrony in sOP and NOP children; that is, the proteins appeared equally and highly immunogenic in children at age 6 to 15 months and then leveled off in their rise at 15 to 25 months. In contrast, rises in concentrations to PhtE and LytB were significantly slower and had not peaked in children even at 25 months of age, consistent with lower immunogenicity. Serum IgM responses against PhtD and PlyD1 were in synchrony in children at age 6–25 months old. PcpA did not induce a significant increase of serum IgM response in children, suggesting that primary responses to PcpA occurred prior to children attaining age 6 months old. PhtD, PcpA, and Ply elicit a synchronous natural acquisition of serum antibody in young children suggesting that a trivalent Spn protein vaccine combining PhtD, PcpA, and PlyD1 would be less likely to display antigen competition when administered as a combination vaccine in young children.
Abbreviations:
- Spn, Streptococcus pneumoniae
- AOM, acute otitis media
- OP, otitis prone
- sOP, stringently-defined otitis prone
- NOP, non-otitis prone
- PCV, pneumococcal conjugate vaccine
- NP, nasopharyngeal
- PhtD, pneumococcal histidine triad protein D
- PhtE, pneumococcal histidine triad protein E
- PcpA, pneumococcal choline binding protein A
- Ply, pneumolysin
- PlyD1, pneumolysin derivative 1
- ELISA, Enzyme-linked Immunosorbent Assay
- LC, log10 Concentration
- GAM, generalized additive model
- GAMM, generalized additive mixed model
- LME, linear mixed effects
Introduction
Streptococcus pneumoniae (Spn) is an important human pathogen, causing diseases such as acute otitis media (AOM), pneumonia, meningitis, and bacteremia.Citation1 As of March 2012, the World Health Organization (WHO) estimates that globally 476,000 child deaths under 5 years of age occurred during 2008 due to pneumococcal (Spn) infections. 5% of all cause-child mortality under 5 were due to pneumococcal infections.Citation2 To prevent pneumococcal infections in children during the first year of life and subsequently, a 7-valent protein-polysaccharide conjugate vaccine (PCV7) was introduced in 2000.Citation3 About 3 years after widespread use of PCV7 other serotypes emerged to replace the previously most common 7 serotypes and strains expressing those capsular serotypes caused disease, most prominently serotype 19A.Citation4 A strategy to address the newly emerging replacement serotypes was to add 6 more protein-polysaccharide ingredients to make a 13-valent pneumococcal conjugate vaccine (PCV13)Citation5 or 3 more types (PCV10).Citation6 The second-generation pneumococcal conjugate vaccines have proven highly efficacious in reducing nasopharyngeal (NP) colonization, pneumonia and invasive disease due to the strains expressing the targeted 10 to 13 polysaccharide capsule types. However, the other serotypes of >90 polysaccharide Spn capsular types may need to be targeted as well. Therefore, several groups are proceeding with study and development of pneumococcal vaccines based on conserved proteins expressed by Spn.
A number of Spn protein virulence factors have been identified as vaccine candidates, including PsaA, PspC, PspA, PcpA, Ply, and PhtD.Citation7 Age-dependent natural antibody induction has been detected for these antigens in children after Spn NP colonization and respiratory tract infections.Citation8,9 Moreover, naturally acquired antibody increasing with age has been correlated with reduced progression from NP colonization to AOM.Citation10,11 Our group has been investigating 5 pneumococcal proteins as possible ingredients to be included in a multi-component vaccine.Citation12-18 We sought conserved proteins of Spn with different functions during pathogenesis, including components that could elicit strong antibody responses to prevent adherence of Spn to NP and lung epithelium while also inducing rapid innate immune cell activation. We reasoned a multi-component vaccine could be more efficacious than a single-valent vaccine given the quick systemic dissemination of pneumococci during pathogenesis.Citation16,17,19,20 The 5 proteins studied have been PhtD and PhtE (pneumococcal histidine triad proteins), PcpA (a choline binding protein), LytB (a murein hydrolase) and PlyD1 (a non-toxic pneumolysin derivative).Citation21-25
The central focus of our research has been to examine serum and mucosal responses to the 5 protein vaccine candidates following natural NP colonization and AOM in young children. It may be true that immunogens could be prepared in pure form and adjuvanted to stimulate an immune response in young children when natural exposure to the protein would not stimulate a response. However, natural priming and boosting of the immune system is of recognized importance in successful vaccination and in sustaining immunogenicity and protection from disease.Citation19 Therefore, we hypothesized that among the antigens available, selection of those that were more immunogenic at the youngest ages enhances the chances of their success as vaccines.
We have been particularly interested to find a vaccine to prevent AOM caused by Spn and specifically in young children who experience repeated, closely spaced AOM infections, termed otitis prone (OP) children, since AOM is by far the most frequent form of Spn disease in children with an economic impact >$1 billion.Citation26 OP children are defined as children with at least 3 AOM episodes in 6 months or 4 episodes in a 12-month time span.Citation26-29 To meet the definition of stringently defined OP (sOP) children have every episode proven by a tympanocentesis-derived middle ear fluid positive culture of an otopathogenCitation30 while non-otitis prone (NOP) children are those with 0 to 2 episodes of AOM per year.Citation7,13-15 Our studies of relative immunogenicity in infants and toddlers following NP colonization and AOM in sOP children identified PhtD, PcpA and PlyD1 as the most immunogenic candidates among the 5 antigens tested to consider in a multi-component vaccine.Citation12
Combining vaccine ingredients into a multi-component product can lead to diminished immunogenicity compared to immunogenicity elicited when the components are administered as single ingredients.Citation31 mechanisms have been described to account for reduced immunogenicity in combination vaccines but common among the results has been a reduction in immunogenicity for the least immunogenic ingredient in a combination.Citation31 Nevertheless, when targeting a disease-causing organism, having a multi-component vaccine can increase the overall effectiveness of the vaccine compared to a single vaccine antigen.Citation31,32 Here we present a novel method of analysis of immunogenicity as a means to predict an increased likelihood of equivalent immunogenicity of a multi- component vaccine. The statistical models were applied to estimate nonlinear age-dependent antibody rises. Using PhtD, PcpA and PlyD1 as an example we show synchrony in the serum antibody responses to these 3 antigens but not with PhtE or LytB. We also show that among the 3 proteins PcpA is more immunogenic at the youngest age of children and has the best immunogenicity in sOP children.
Results
Serum IgG antibody response to Spn proteins in NOP children
The children studied here were selected from a large cohort based on the fact that they experienced culture-confirmed NP colonization with Spn repeatedly such that an immune response most likely would be stimulated. To confirm that antibody concentrations to these 5 proteins reflected a child's exposure to Spn and not a response to other natural NP organisms that cause AOM, such as Haemophilus influenzae, Moraxella catarrhalis and Staphylococcus aureus, we performed a protein homology search analysis using the basic local alignment search tool (BLASTp) algorithm. We found that the conserved 5 Spn proteins studied did not share significant homology with the other common organisms that cause AOM.
The antigen-specific serum antibody analysis reported in this study describes the change of antibody concentration over time among the children in the specified cohorts (left most panel) along with the corresponding rate of antibody concentration change against age change (ΔConcentration/ΔAge, right most panel) for each antigen tested as shown in the accompany figures. For the NOP children, all 5 Spn antigens stimulated an increase in serum IgG antibody concentrations over time (). Significant increases were measured in serum IgG against PhtD (P = 9.27 × 10−11, ), PcpA (P = 2.63 × 10−7, ), and PlyD1 (P = 2.50 × 10−7, ) in children at age 6-15 months old, and against PhtE (P =1.43 × 10−13, ) and LytB (P = 6.56 × 10−4, ) at age 6-25 months. Serum IgG responses to PhtD, PcpA and PlyD1 were in synchrony because the rate of rise (Δlog10 Concentration/ΔAge) in antibody concentration was not significantly different between age 6 and 15 months and then not significantly different between age 15 and 25 months for the 3 antigens (). In contrast the slope (rate of rise) in antibody concentration for LytB and PhtE were not similar to PhtD, PcpA and PlyD1 nor to each other ().
Figure 1. Serum IgG (green color) and IgM (blue color) antibody response to Spn proteins PhtD (A), LytB (B), PcpA (C), PhtE (D) and PlyD1 (E) in NOP children age 6–25 months. Serum IgG and IgM antibody concentrations were determined with a quantitative ELISA and then logarithm transformed. Mean (solid lines) and 95% confidence intervals (dashed lines) are shown and zero is shown as a red line. Functional estimates of log10 Concentration concentrations against age were obtained using a generalized additive model (left most panel). Antibody/age gradients (Δlog10 Concentration/ΔAge) with confidence bands are directly calculated from the fits, permitting the direct estimation and comparison of rates of (change in antibody rise)/(change in age) (right most panel). Response curves were estimated relative to a baseline defined at age 6 months, and therefore represent log transforms of proportional increase from that baseline. A total of 73 time points from 33 subjects were analyzed. Circles and crosses represent IgG data and IgM data, respectively.
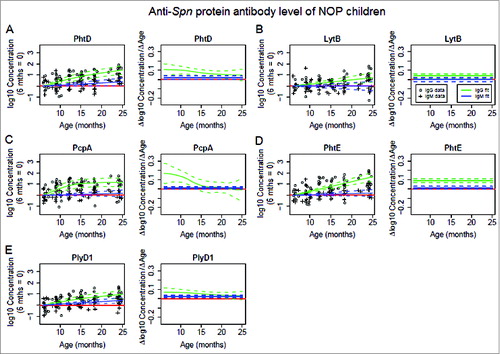
The mean rate of increase for serum IgG against PcpA was highest among 5 Spn proteins at age 6-10 months (). mean rate of increase of serum IgG antibody against PhtD () and PlyD1 () declined through 25 months. These results indicate that PcpA was more immunogenic than PhtD or PlyD1 at an earlier age, and much earlier than LytB or PhtE.
Serum IgG antibody response to Spn proteins in the sOP children
For sOP children PhtD, PcpA, PhtE and PlyD1 all except LytB stimulated an increase in serum IgG antibody concentrations over time (). Significant increases were measured in serum IgG against PhtD (P = 4.08 × 10−7, ), PcpA (P = 2.94 × 10−5, ) and PlyD1 (P = 9.68 × 10−11, ) in children age 6-15 months old, and against PhtE (P = 3.05 × 10−3, ) age 6-25 months old. Serum IgG concentrations to PhtD, PcpA and PlyD1 were in synchrony. is evident by examination of the slope for the 3 antigens (). In contrast the slope in antibody concentration for LytB and PhtE were not similar to PhtD, PcpA and PlyD1 ().
Figure 2. Serum IgG (green color) and IgM (blue color) antibody response to Spn proteins PhtD (A), LytB (B), PcpA (C), PhtE (D) and PlyD1 (E) in sOP children age 6–25 months. Serum IgG and IgM antibody concentrations were determined with a quantitative ELISA and then logarithm transformed. Mean (solid lines) and 95% confidence intervals (dashed lines) are shown and zero is shown as a red line. Functional estimates of log10 Concentration concentrations against age were obtained using a generalized additive model (left most panel). Antibody/age gradients (Δlog10 Concentration/ΔAge) with confidence bands are directly calculated from the fits, permitting the direct estimation and comparison of rates of (change in antibody rise)/(change in age) (right most panel). Response curves were estimated relative to a baseline defined at age 6 months, and therefore represent log transforms of proportional increase from that baseline. A total of 22 time points from 6 subjects were analyzed. Circles and crosses represent IgG data and IgM data, respectively.
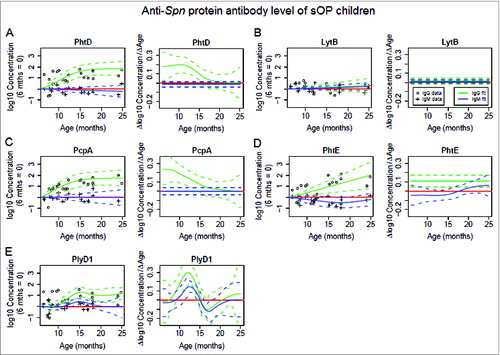
Figure 3. Range of age gradient for anti-Spn protein antibody concentrations. Barplots display the range (maximum - minimum) of the antibody/age gradients (Δlog10 Concentration/ΔAge) shown in (protiens PhtD, LytB, PcpA, PhtE, and PlyD1 for NOP and sOP subjects, respectively). Standard errors are included (not visible when approximately equal to zero).
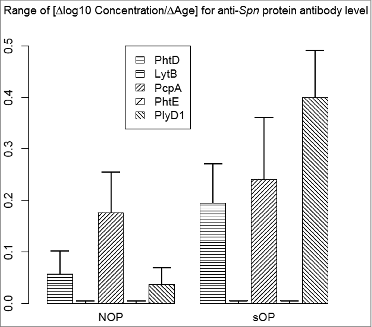
For sOP children the mean rate of increase for serum IgG against PcpA was the highest among 5 Spn proteins at age 6-9 months old, then declined through age 10 months and intersected 0 at age 24 months (). The mean rate of increase for serum IgG against PhtD declined significantly through age 17.5 months and descended sharply close to 0 at age 17.5 months (), much quicker than in NOP children (). Similarly, sOP children had an earlier and faster decline of serum IgG against PlyD1 through 12.5 months, intersecting 0 at age 16 months (), while NOP children showed a much slower declining slope of serum IgG increase without intersecting 0 over time (). Hence, the rate of increase of serum IgG against Spn proteins PhtD and PlyD1 declined earlier and quicker in sOP children () than in NOP children ().
Compared to NOP children (), sOP children kept a much lower constant and non-significant rate of increase for serum IgG against LytB over time (). In the PhtE plot for sOP children, the uncertainty of the behavior of the curve near 25 months, indicated by the larger width of the standard error bars at that point, was interpreted to indicate that we cannot rule out that the rate of increase falls near 25 months. Similarly, for PhtD and PcpA, we only observe that the curves fall within the standard error (SE) bars with high confidence. Overall, qualitative observations of response curves for IgG conform to a negative sOP main effect on the whole population, although statistical significance was not attained using the LME model methods.
Serum IgM antibody response to Spn proteins in NOP children
Serum IgM analysis allowed assessment of primary responses to the 5 Spn proteins in the studied children. For NOP children, PhtD (P = 0.026, ) and PlyD1 (P = 9.32 × 10−4, ) stimulated an increase of serum IgM antibody concentrations over the full time of observations. Thus, serum IgM responses against PhtD and PlyD1 were in synchrony in NOP children at age 6–25 months old. PcpA did not induce a significant increase of serum IgM response in children over time (P = 0.218, ). Taking into account the IgG response to PcpA the data suggest that primary responses to this antigen occurred prior to children attaining the age of 6 months old. LytB (P = 0.996, ) and PhtE (P = 0.166, ) did not induce a significant increase of serum IgM response in children over time. Taking into account the IgG response to LytB and PhtE, the data suggest that primary responses to these antigens continued to occur over time but at a rate that was not significant.
Serum IgM antibody response to Spn proteins in sOP children
For sOP children, PlyD1 stimulated a significant increase in serum IgM antibody concentrations over time (P = 1.05 × 10−4, ). PhtD (P = 0.446, ) and PcpA (P = 0.973, ) did not induce a significant increase of serum IgM response in children over time nor did LytB (P = 0.236, ) or PhtE (P = 0.117, ). Using the LME model, a significant negative sOP main effect on the whole population was observed for PhtD (P = 0.007), PhtE (P = 0.001) and PlyD1 (P = 0.013) in IgM responses. Taking into account the IgG response to PhtD, LytB, PcpA and PhtE, the IgM responses of sOP children had a similar explanation as applicable to the total and NOP population.
Gradient ranges for antibody response to Spn proteins
shows the antibody/age gradient (Δlog10 Concentration/ΔAge) ranges (maximum minus minimum gradient) for each gradient curve shown in , with standard error bars. The purpose of the analysis is to consider specifically whether or not the rate of increase changes with age. A range of 0 is equivalent to constant gradient. For LytB and PhtE the estimates and standard errors of the range were negligible. In comparison, the ranges for PhtD, PcpA and PlyD1 all differed from zero with a high degree of confidence.
Discussion
Since most pediatric vaccines in the US and many other countries are given at 2, 4, and 6 months of age and a booster at 15 months of age, immunogens that elicit responses at an earlier age are generally preferred. The results of this study suggest that infants naturally exposed to PhtD, PcpA and Ply antigens in the first months of life are more likely to respond to active immunization with these antigens due to concurrent and/or prior priming from natural exposure.
PhtD, PcpA and PlyD1 have been described by our group as better immunogens following natural exposure to Spn in both NOPCitation12 and sOPCitation13 children. Here we describe a new mathematical approach that further strengthens this observation. Moreover, the model predicts non-interference among the 3 proteins by demonstrating that on common exposure to the proteins expressed by the organism, antibody rises synchronously at the same rate at the same child age. Concurrent vaccination of similarly immunogenic components would be less likely to result in diminished antibody responses caused by antigenic competition if the antigens are given as a multi-component product.Citation31 Thus a second key aspect of the analysis is confirmed by prior clinical data.Citation33,34 Also, during pre-clinical testing the trivalent vaccine containing PhtD, PcpA and PlyD1 showed similar immunogenicity as occurred following immunization with individual components of the vaccine (Sanofi Pasteur, personal communication). Third, the modeling of IgM responses in children informs on child age when immune priming occurs. Taken together the results suggest that the model could be applied prospectively in future analysis of other vaccine ingredients intended to be combined in a single vaccine product. Further studies comparing the human immune efficacy among the different combinations of these antigens and each individual component with or without adjuvants are warranted to confirm the observations in the current study. However, the current work supports that PhtD, PcpA and PlyD1 are compatible to be formulated in a single trivalent vaccine that is now in Phase I studies by Sanofi Pasteur (NCT01764126).
In this study, the observations were from children with NP Spn culture positive samples. A robust natural antibody response to the 5 Spn proteins and other Spn protein antigens has been observed in young children in our prior findings and those of others.Citation8,12,Citation13,35-37 As previously observed, natural exposure to Spn can elicit antibody production to various Spn proteins but multiple exposures to Spn may only reflect a booster-type response to certain antigens.Citation8 In the current study only antibody responses to PhtD, PcpA and PlyD1 and not PhtE and LytB showed booster responses.
Our study suggests that detecting the synchrony of natural antibody responses in young children may provide valuable preclinical screening data for identifying efficacious Spn protein vaccine antigens. Although antigens that elicit a robust natural immune response would logically be good vaccine candidates since natural exposures to the potential pathogen would likely prime and/or boost vaccine-induced immunity, it is important to know when the vaccine antigens are expressed during infection, that they are accessible to antibodies, and that the antibodies provide protection. example, some cell wall proteins such as PsaA are more accessible to antibodies upon adhering to and invading host cells as a result of reduced production of capsular polysaccharide.Citation38,39 Furthermore, if a rare protein on the surface has a crucial function, it might not be very immunogenic but antibody to it could be very protective. Our lab has recently demonstrated that vaccination with PhtD, PcpA and PlyD1 as a trivalent vaccine protects mice from lethal challenge,Citation40 supporting their importance during pathogenesis and accessibility to antibody for clearance.
Our study of antibody response synchrony and the analysis we performed is novel. We sought a method to understand relative, age-dependent immunogenicity of multiple vaccine candidates. A plot of antibody concentrations over time allowed visualization of the rate of rise of antibody (Δlog10 Concentration) over the age range when samples were collected from the child study population (ΔAge). plot created a slope that could be compared statistically among the vaccine candidates by analysis of differences in the rate of rise (Δlog10 Concentration/ΔAge). Taking the age of the child at 6 months (when we had our first serum samples) as a baseline, plotting Δlog10 Concentration/ΔAge also allowed identification of when, in time, the rate of rise, Δlog10 Concentration/ΔAge crossed the zero time point thereby showing when a maximum IgG or IgM response was achieved. The serum antibodies against PhtD, PcpA, and PlyD1 reached a plateau at age 21 months with a baseline gradient of increase for both NOP and sOP children. We reason this as a sign of potentially peaked humoral immune response to the Spn antigens in young children. Such analysis corroborated the synchrony of responses. In this study, the values for the Δlog10 Concentration/ΔAge represent the gradient increase of naturally stimulated antibody against Spn within an age time span of 18 months in young children. From the data, we gain insight regarding the process of natural acquisition of serum antibody against Spn in young children. We were interested primarily in estimating rates of antibody increase, and their dependence on age. Therefore, the rates of change in antibody concentrations over time do not depend on the initial values (i.e., at 6 months).
We studied 5 candidate antigens in young children in this study. Synchrony of antibody response across the age range of children studied was identified for PhtD, PcpA and PlyD1 for IgG responses. Immunogenicity was present across the age span 6-15 months for all 3 proteins and all 3 proteins peaked at around 15 months of age and then titered off consistent with maximized response. The IgM concentrations, showing primary response showed a similar pattern of early increases in the 6–15 month time span. The synchronously increased natural antibodies against PhtD, PcpA and PlyD1 at an early age (by 15 months) support the notion that these particular Spn antigens are priming the host immune system. A primed immune system to particular antigens would be more likely to respond to a vaccine containing those antigens. The vulnerability of children to Spn infections at earlier ages may be due to insufficient natural immune responses to Spn antigen exposure. It should be recognized that insufficient natural immune responses to Spn antigen exposure may be overcome by vaccination with pure, concentrated antigen and adjuvant. There also is the opposite hypothesis: if children respond vigorously to vaccine candidates such as PhtD, PcpA and PlyD1 proteins by 15 months, perhaps they should have been protected against further Spn colonization events prior to 24 months. It is well established that children are less frequently colonized with Spn after 24 months compared to before 24 months of age. Thus it might be argued that better vaccine candidates might be proteins that the children are not observed to respond to at an early age but upon immune system maturation respond at an older age. Vaccines (purified and mixed with adjuvant) given at an early age that will stimulate the immune response might be superior to those that are strong immunogens at earlier ages, but do not protect the children from infection. We have recently evaluated the alternative hypothesis and rejected it because we found that elevated serum antibody concentrations to PhtD, PcpA and PlyD1 are correlated with protection from infections (acute otitis media) caused by Spn in children from 6 months to 24 months of age (Almudevar and Pichichero, unpublished results).
The child who experiences recurrent AOM is the most vulnerable to the morbidity of AOM, and any vaccination strategy for AOM must especially target that population. By eliciting synchronous antibody rises at younger ages, a trivalent vaccine containing these 3 antigens might be expected to confer more efficacious protection than any individual antigen in sOP children. Our group has previously shown that sOP children mount lower serum antibody responses against PhtD, PhtE, PcpA, Ply and LytBCitation13 and against NTHi vaccine candidates P6, protein D and OMP26 during NP colonization and AOM.Citation7 Here, for the first time, we showed that PhtD, PcpA and PlyD1 were all sufficiently immunogenic to produce significant rise of serum IgG in sOP children even at the younger age of 6–10 months. Also, the current study for the first time examined IgM responses. From the IgM data we found support for the conclusion that primary responses to PhtD, PcpA and Ply occur at younger ages than PhtE and LytB, supporting the selection of PhtD, PcpA and PlyD1 as the vaccine components to move forward with in human clinical trials. The antibody concentrations in the current study were log transformed, weight-based antibody concentrations (μg/ml for IgG and ng/ml for IgM) determined by using a quantitative ELISA assay whereas the antibody concentrations reported in our prior studies were endpoint ELISA titers.Citation12,13 Moreover, the log transformed concentrations in this work were calculated by subtracting the mean log transformed concentration of the 6 month serum samples for the analysis of the rate of rise by using the GAM model.
Our study has limitations. Due to our stringent selection criteria, a limited number of subjects were included in this study. Nevertheless, as the statistical analysis shows it was possible to compare the difference of age-dependent antibody changes with these limited samples by using the GAM method. To use the 6-month age point as baseline for synchrony analysis, it was essential to compare the absolute concentrations of antibody in the 6-month serum samples. It is probable that natural exposure to Spn could result in a high response to one or more of the proteins by 6 months and the well-regulated adaptive immune system could have limited the antibody response level to that protein. If that was the case the present analysis would consider the antigen to be poor at eliciting an antibody response. To address this potential limitation, we assessed the 6-month baseline antibody concentrations for the proteins studied and confirmed they were not significantly different from what was previously reported in a larger study cohort.Citation12,13
Conclusions
Serum IgG antibody responses against PhtD, PcpA, and PlyD1 were in synchrony, which suggests that they are similarly immunogenic and therefore compatible to be combined in a trivalent Spn protein vaccine.
Materials and Methods
Study cohort
Children were selected for this study from a population previously describedCitation12,26 (). In total, 39 infants born between February 2006 and August 2010 were included (). Six sOP children provided 49 observations from 22 time points and 33 NOP children provided 771 observations from 79 time points. These children were selected based on detected Spn colonization of the NP or AOM caused by Spn at 4 or more of 6 prospective visits (at age 6, 9, 12, 15, 18, and 24–25 months), and availability of sera collected at those visits from a longitudinal study cohort of >500 total population. Because of the selection criteria, the subject numbers are low albeit sufficient to reach statistically valid conclusions. All children received pneumococcal conjugate vaccine containing 7 serotypes at age 2, 4, 6 and 15 months of age. The study was approved by the Rochester General Hospital Research Subjects Review Board.
Table 1. Demographics of the subjects
Quantitative enzyme-linked immunosorbent assay (ELISA)
Serum protein-specific antibody concentrations were quantitated using an ELISA. Recombinant proteins, PhtD, PhtE, PcpA, LytB and PlyD1 were provided by Sanofi Pasteur (Canada). 96-well Nunc-Immulon 4 plates were coated with 1 μg/mL of individual Spn antigens except 1.74 μg/mL of PhtE (100 μL/well) in coating buffer (bicarbonate, [pH 9.6]) and incubated overnight at 4°C. After washing the plates were blocked with 4% skim milk at 37°C for 1 h (200 μL per well). After five washes, 100 μL of serum at a starting dilution of 1:100 (in PBS buffered 4% skim milk) was added to the wells and diluted serially 2 fold. The mixture was incubated at room temperature for 1 h followed by the addition of affinity purified goat anti-human IgG or IgM antibody conjugated to horseradish-peroxidase (Bethyl Laboratories, Inc., Montgomery, TX) as a secondary antibody. The reaction products were developed with TMB Microwell Peroxidase Substrate System (KPL, Gaithersburg, MD), stopped by the addition of 1.0 M phosphoric acid and read by an automated ELISA reader using a 450-nm filter. To provide quantitative results on antibody concentrations, the concentration of the specific antibody present in the unknown sample was determined by comparing to an internal reference serum (pool of human serum with high anti-Spn antigen titers). The concentrations of IgG and IgM in the reference serum were quantitatively measured by using a human IgG/IgM ELISA quantitation kit (Bethyl laboratories).
A four-parameter logistic-log function was used to form the reference and sample curves. The lower detection limits of the IgG were 1.88 μg/ml for PhtD, 0.99 μg/ml for LytB, 0.42 μg/ml for PcpA, 0.025 μg/ml for PhtE, 0.17 μg/ml for PlyD1. The lower detection limits of the IgM were 26.78 ng/ml for PhtD, 6.5 ng/ml for LytB, 7.35 ng/ml for PcpA, 24 ng/ml for PhtE, 7.19 ng/ml for PlyD1. The intra-assay coefficient of variation was ≤30% for all antigens.
Data processing and statistical analysis
All antibody concentrations were logarithm transformed. Estimates of functional relationship between mean log10 Concentration (LC) response and age (months) were obtained using a generalized additive model (GAM).Citation37 GAM combines inference methods of linear models with nonlinear predictor terms. The estimated curve is mathematically tractable, permitting estimates of both mean LC response as a function of age, and the LC/age gradient, which is the mathematical derivative of LC response function. In addition, 95% confidence bands were constructed for response and gradient functions. Response curves were estimated relative to a baseline defined at age 6 months, and therefore represent log transforms of proportional increase from baseline. For statistical analysis of the rate of rise using the GAM model, it was necessary to establish a baseline concentration of antibody and assign that value (antibody concentration) as zero. Our analysis showed that the 6-month time point was the best as the baseline. Hence, the 6 month log transformed antibody concentrations were subtracted from all the log transformed antibody concentrations at subsequent time points. Mathematically, the motivation for using GAM is in its ability to model antibody concentrations with nonlinear dependence on age. Rates of increase (mathematical derivatives, or slopes) can be calculated directly from the fit, and standard errors can then be calculated for these rates. This permits investigation and comparison not only of absolute antibody concentrations, but also of rates of increase, as well as their dependence on age, in a statistically rigorous manner.
Models were fit using the generalized additive mixed model (GAMM) function, part of the R statistical computing environment.Citation41 This function allows inclusion of random effects in the model. This permits modeling of appropriate correlation structure induced by use of subject concentration repeated measures.
A separate analysis was used to estimate sOP main effects. Because of positive LC/age gradients generally observed, an sOP main effect was most significant at higher ages. A linear mixed effects model (LME) was constructed for measures collected at ages ≥15 months, and an sOP main effect was incorporated directly into the model. P< 0.05 was considered significant.
Disclosure of Potential Conflicts of Interest
Michael E. Pichichero has received research grants from Pfizer and Sanofi Pasteur. Dabin Ren and Anthony L. Almudevar have declared no conflicts of interest.
Acknowledgments
We thank Jill Mangiafesto and her team for their work on the ELISA assays. We thank Dr. Robert Zagursky for his assistance in the data interpretation. We thank Dr. Janet Casey and the nurses and staff of Legacy Pediatrics and the collaborating pediatricians from Sunrise Pediatrics, Westfall Pediatrics, Lewis Pediatrics and Long Pond Pediatrics and the parents who consented and the children who participated in this challenging study.
Funding
This study was supported by NIH NIDCD R01 08671 and Sanofi Pasteur. The funders had no role in study design, data collection and analysis, decision to publish, or preparation of the manuscript.
References
- McCullers JA, Tuomanen EI. Molecular pathogenesis of pneumococcal pneumonia. Front Biosci: J Virtual Libr 2001; 6:D877-89; PMID:11502489; http://dx.doi.org/10.2741/McCullers
- Huber VC, Thomas PG, McCullers JA. A multi-valent vaccine approach that elicits broad immunity within an influenza subtype. Vaccine 2009; 27:1192-200; PMID:19135117; http://dx.doi.org/10.1016/j.vaccine.2008.12.023
- Perera PY, Derrick SC, Kolibab K, Momoi F, Yamamoto M, Morris SL, Waldmann TA, Perera LP. A multi-valent vaccinia virus-based tuberculosis vaccine molecularly adjuvanted with interleukin-15 induces robust immune responses in mice. Vaccine 2009; 27:2121-7; PMID:19356615; http://dx.doi.org/10.1016/j.vaccine.2009.01.132
- Pichichero ME, Casey JR. Emergence of a multiresistant serotype 19A pneumococcal strain not included in the 7-valent conjugate vaccine as an otopathogen in children. JAMA 2007; 298:1772-8; PMID:17940232; http://dx.doi.org/10.1001/jama.298.15.1772
- Francis JP, Richmond PC, Pomat WS, Michael A, Keno H, Phuanukoonnon S, Nelson JB, Whinnen M, Heinrich T, Smith WA, et al. Maternal antibodies to pneumolysin but not to pneumococcal surface protein A delay early pneumococcal carriage in high-risk Papua New Guinean infants. Clin Vaccine Immunol 2009; 16:1633-8; PMID:19776196; http://dx.doi.org/10.1128/CVI.00247-09
- Schachern PA, Tsuprun V, Goetz S, Cureoglu S, Juhn SK, Briles DE, Paparella MM, Ferrieri P. Viability and virulence of pneumolysin, pneumococcal surface protein A, and pneumolysin/pneumococcal surface protein A mutants in the ear. JAMA Otolaryngol Head Neck Surg 2013; 139:937-43; PMID:24051749; http://dx.doi.org/10.1001/jamaoto.2013.4104
- Feldman C, Anderson R. Review: current and new generation pneumococcal vaccines. J Infect 2014; 69:309-25; PMID:24968238; http://dx.doi.org/10.1016/j.jinf.2014.06.006
- Rapola S, Jantti V, Haikala R, Syrjanen R, Carlone GM, Sampson JS, Briles DE, Paton JC, Takala AK, Kilpi TM, et al. Natural development of antibodies to pneumococcal surface protein A, pneumococcal surface adhesin A, and pneumolysin in relation to pneumococcal carriage and acute otitis media. J Infect Dis 2000; 182:1146-52; PMID:10979911; http://dx.doi.org/10.1086/315822
- Lebon A, Verkaik NJ, Labout JA, de Vogel CP, Hooijkaas H, Verbrugh HA, van Wamel WJ, Jaddoe VW, Hofman A, Hermans PW, et al. Natural antibodies against several pneumococcal virulence proteins in children during the pre-pneumococcal-vaccine era: the generation R study. Infect Immun 2011; 79:1680-7; PMID:21282409; http://dx.doi.org/10.1128/IAI.01379-10
- Rapola S, Kilpi T, Lahdenkari M, Takala AK, Makela PH, Kayhty H. Do antibodies to pneumococcal surface adhesin a prevent pneumococcal involvement in acute otitis media? J Infect Dis 2001; 184:577-81; PMID:11494164; http://dx.doi.org/10.1086/322763
- Simell B, Ahokas P, Lahdenkari M, Poolman J, Henckaerts I, Kilpi TM, Käyhty H. Pneumococcal carriage and acute otitis media induce serum antibodies to pneumococcal surface proteins CbpA and PhtD in children. Vaccine 2009; 27:4615-21; PMID:19524618; http://dx.doi.org/10.1016/j.vaccine.2009.05.071
- Pichichero ME, Kaur R, Casey JR, Xu Q, Almudevar A, Ochs M. Antibody response to Streptococcus pneumoniae proteins PhtD, LytB, PcpA, PhtE and Ply after nasopharyngeal colonization and acute otitis media in children. Hum Vaccines Immunother 2012; 8:799-805; PMID:22495112; http://dx.doi.org/10.4161/hv.19820
- Kaur R, Casey JR, Pichichero ME. Serum antibody response to five Streptococcus pneumoniae proteins during acute otitis media in otitis-prone and non-otitis-prone children. Pediat Infect Dis J 2011; 30:645-50; PMID:21487325; http://dx.doi.org/10.1097/INF.0b013e31821c2d8b
- Sharma SK, Casey JR, Pichichero ME. Reduced memory CD4+ T-cell generation in the circulation of young children may contribute to the otitis-prone condition. J Infect Dis 2011; 204:645-53; PMID:21791667; http://dx.doi.org/10.1093/infdis/jir340
- Sharma SK, Casey JR, Pichichero ME. Reduced serum IgG responses to pneumococcal antigens in otitis-prone children may be due to poor memory B-cell generation. J Infect Dis 2012; 205:1225-9; PMID:22383675; http://dx.doi.org/10.1093/infdis/jis179
- Khan MN, Sharma SK, Filkins LM, Pichichero ME. PcpA of Streptococcus pneumoniae mediates adherence to nasopharyngeal and lung epithelial cells and elicits functional antibodies in humans. Microbes Infect/Institut Pasteur 2012; 14:1102-10; PMID:22796387; http://dx.doi.org/10.1016/j.micinf.2012.06.007
- Khan MN, Pichichero ME. Vaccine candidates PhtD and PhtE of Streptococcus pneumoniae are adhesins that elicit functional antibodies in humans. Vaccine 2012; 30:2900-7; PMID:22349524; http://dx.doi.org/10.1016/j.vaccine.2012.02.023
- Sharma SK, Roumanes D, Almudevar A, Mosmann TR, Pichichero ME. CD4+ T-cell responses among adults and young children in response to Streptococcus pneumoniae and Haemophilus influenzae vaccine candidate protein antigens. Vaccine 2013; 31:3090-7; PMID:23632305; http://dx.doi.org/10.1016/j.vaccine.2013.03.060
- Pichichero ME. Booster vaccinations: can immunologic memory outpace disease pathogenesis? Pediatrics 2009; 124:1633-41; PMID:19933727; http://dx.doi.org/10.1542/peds.2008-3645
- Casal J, Tarrago D. Immunity to Streptococcus pneumoniae: factors affecting production and efficacy. Curr Opin Infect Dis 2003; 16:219-24; PMID:12821811; http://dx.doi.org/10.1097/00001432-200306000-00006
- Adamou JE, Heinrichs JH, Erwin AL, Walsh W, Gayle T, Dormitzer M, Dagan R, Brewah YA, Barren P, Lathigra R, et al. Identification and characterization of a novel family of pneumococcal proteins that are protective against sepsis. Infect Immun 2001; 69:949-58; PMID:11159990; http://dx.doi.org/10.1128/IAI.69.2.949-958.2001
- Garcia P, Gonzalez MP, Garcia E, Lopez R, Garcia JL. LytB, a novel pneumococcal murein hydrolase essential for cell separation. Mol Microbiol 1999; 31:1275-81; PMID:10096093; http://dx.doi.org/10.1046/j.1365-2958.1999.01238.x
- Glover DT, Hollingshead SK, Briles DE. Streptococcus pneumoniae surface protein PcpA elicits protection against lung infection and fatal sepsis. Infect Immun 2008; 76:2767-76; PMID:18391008; http://dx.doi.org/10.1128/IAI.01126-07
- Hava DL, Camilli A. Large-scale identification of serotype 4 Streptococcus pneumoniae virulence factors. Mol Microbiol 2002; 45:1389-406; PMID:12207705
- Marriott HM, Mitchell TJ, Dockrell DH. Pneumolysin: a double-edged sword during the host-pathogen interaction. Curr Mol Med 2008; 8:497-509; PMID:18781957; http://dx.doi.org/10.2174/156652408785747924
- Pichichero ME, Casey JR, Almudevar A. Reducing the frequency of acute otitis media by individualized care. Pediat Infect Dis J 2013; 32:473-8; PMID:23340550; http://dx.doi.org/10.1097/INF.0b013e3182862b57
- Aprile MA, Wardlaw AC. Aluminium compounds as adjuvants for vaccines and toxoids in man: a review. Can J Public Health 1966; 57:343-60; PMID:5329166
- Alho OP, Laara E, Oja H. What is the natural history of recurrent acute otitis media in infancy? J Family Practice 1996; 43:258-64; PMID:8797753
- Poehling KA, Szilagyi PG, Grijalva CG, Martin SW, LaFleur B, Mitchel E, Barth RD, Nuorti JP, Griffin MR. Reduction of frequent otitis media and pressure-equalizing tube insertions in children after introduction of pneumococcal conjugate vaccine. Pediatrics 2007; 119:707-15; PMID:17403841; http://dx.doi.org/10.1542/peds.2006-2138
- Sharma SK, Pichichero ME. Cellular immune response in young children accounts for recurrent acute otitis media. Curr Allergy Asthma Rep 2013; 13:495-500; PMID:24022464
- Pichichero ME. New combination vaccines. Pediat Clin N Am 2000; 47:407-26; PMID:10761511; http://dx.doi.org/10.1016/S0031-3955(05)70214-5
- Darrieux M, Goulart C, Briles D, Leite LC. Current status and perspectives on protein-based pneumococcal vaccines. Crit Rev Microbiol 2013; PMID:23895377
- Leroux-Roels G, Maes C, De Boever F, Traskine M, Ruggeberg JU, Borys D. Safety, reactogenicity and immunogenicity of a novel pneumococcal protein-based vaccine in adults: a phase I/II randomized clinical study. Vaccine 2014; 32:6838-46; PMID:24607003; http://dx.doi.org/10.1016/j.vaccine.2014.02.052
- Bologa M, Kamtchoua T, Hopfer R, Sheng X, Hicks B, Bixler G, Hou V, Pehlic V, Yuan T, Gurunathan S. Safety and immunogenicity of pneumococcal protein vaccine candidates: monovalent choline-binding protein A (PcpA) vaccine and bivalent PcpA-pneumococcal histidine triad protein D vaccine. Vaccine 2012; 30:7461-8; PMID:23123106; http://dx.doi.org/10.1016/j.vaccine.2012.10.076
- Simell B, Korkeila M, Pursiainen H, Kilpi TM, Kayhty H. Pneumococcal carriage and otitis media induce salivary antibodies to pneumococcal surface adhesin a, pneumolysin, and pneumococcal surface protein a in children. J Infect Dis 2001; 183:887-96; PMID:11237805; http://dx.doi.org/10.1086/319246
- Briles DE, Hollingshead SK, Paton JC, Ades EW, Novak L, van Ginkel FW, Benjamin WH Jr. Immunizations with pneumococcal surface protein A and pneumolysin are protective against pneumonia in a murine model of pulmonary infection with Streptococcus pneumoniae. J Infect Dis 2003; 188:339-48; PMID:12870114; http://dx.doi.org/10.1086/376571
- Baril L, Briles DE, Crozier P, King JD, Hollingshead SK, Murphy TF, McCormick JB. Natural materno-fetal transfer of antibodies to PspA and to PsaA. Clin Exp Immunol 2004; 135:474-7; PMID:15008981; http://dx.doi.org/10.1111/j.1365-2249.2003.02357.x
- Hammerschmidt S, Wolff S, Hocke A, Rosseau S, Muller E, Rohde M. Illustration of pneumococcal polysaccharide capsule during adherence and invasion of epithelial cells. Infect Immun 2005; 73:4653-67; PMID:16040978; http://dx.doi.org/10.1128/IAI.73.8.4653-4667.2005
- Schulz C, Hammerschmidt S. Exploitation of physiology and metabolomics to identify pneumococcal vaccine candidates. Expert Rev Vaccines 2013; 12:1061-75; PMID:24053399; http://dx.doi.org/10.1586/14760584.2013.824708
- Verhoeven D, Xu Q, Pichichero ME. Vaccination with a Streptococcus pneumoniae trivalent recombinant PcpA, PhtD and PlyD1 protein vaccine candidate protects against lethal pneumonia in an infant murine model. Vaccine 2014; 32:3205-10; PMID:24731814; http://dx.doi.org/10.1016/j.vaccine.2014.04.004
- Del Giudice G, Rappuoli R. Genetically derived toxoids for use as vaccines and adjuvants. Vaccine 1999; 17 Suppl 2:S44-52; PMID:10506408; http://dx.doi.org/10.1016/S0264-410X(99)00234-0