Abstract
Bioconversion of hemicellulosic hydrolysate into ethanol plays a pivotal role in the overall success of biorefineries. For the efficient fermentative conversion of hemicellulosic hydrolysates into ethanol, the use of immobilized cells system could provide the enhanced ethanol productivities with significant time savings. Here, we investigated the effect of 2 important factors (e.g., cell concentration and stirring) on ethanol production from sugarcane bagasse hydrolysate using the yeast Scheffersomyces stipitis immobilized in calcium alginate matrix. A 22 full factorial design of experiment was performed considering the process variables- immobilized cell concentration (3.0, 6.5 and 10.0 g/L) and stirring (100, 200 and 300 rpm). Statistical analysis showed that stirring has the major influence on ethanol production. Maximum ethanol production (8.90 g/l) with ethanol yield (Yp/s) of 0.33 g/g and ethanol productivity (Qp) of 0.185 g/l/h was obtained under the optimized process conditions (10.0 g/L of cells and 100 rpm).
Abbreviations
Yp/s | = | ethanol yield |
Qp | = | ethanol productivity |
2G | = | second generation |
CO2 | = | carbon di-oxide |
SB | = | sugarcane bagasse |
Min | = | minutes |
ANOVA | = | analysis of variance |
df | = | degree of freedom |
Ca-alginate | = | calcium alginate |
HPLC | = | high performance liquid chromatography |
Introduction
Second generation (2G) ethanol, unlike gasoline, is a renewable energy source that can be produced from any kind of lignocellulosic residues such as sugarcane bagasse or corn stover. Ethanol derived from lignocellulosic materials is an ecofriendly liquid fuel, because the released CO2 after burning of ethanol is taken up by growing vegetal biomass through photosynthesis and thus makes no net contribution to the atmosphere.Citation1 However, bioethanol production cost is the major impeding factor for the setting up large scale biorefineries. The inefficient conversion of hemicellulosic hydrolysate is a main obstacle to the biorefineries for the large scale bioethanol production.Citation2
Sugarcane bagasse (SB) is the lignocellulosic material with the huge potential as a renewable feedstock for the bioethanol production in Brazil.Citation3 SB is a fibrous by-product generated after juice extraction in sugar and alcohol production units. Generally, 270–280 kg of bagasse is generated from one ton of sugarcane. SB is available in foreseeable amount round the year and has considerable amounts of carbohydrates (∼67%) in cell wall which can be converted into bioethanol and other value-added products.Citation4 With regards to its composition, it is primarily consisted of cellulose (42%), hemicellulose (25%) and lignin (19%).Citation5 According to Canilha et al.Citation6 the bioconversion of hemicellulose derived sugars into ethanol is an important criterion to achieve satisfactory 2G ethanol production.
The fermentable sugars from the lignocellulosic structure can be released through a pretreatment process. Dilute acid hydrolysis primarily cleaves the hemicellulose structure into monomeric constituents yielding mainly D-xylose in addition with other sugars and some undesired compounds like furans, phenolics and weak acid.Citation6 The ideal microorganism in the biorefinery is the one which can efficiently convert both xylose and glucose into ethanol with equal ethanol yields. Among the xylose utilizing microorganisms, the yeast Scheffersomyces stiptis (Syn. Pichia stipitis) is considered one of the most promising candidate which can efficiently convert hemicellulosic hydrolysates into ethanol.Citation7
Immobilized cells offer several unique advantages, such as easy separation and recovery of the biocatalyst, elimination of the inoculum preparation stage, and higher yields and productivities.Citation8,9 Immobilized cells can be reused in repeated batch, fed-batch and continuous fermentation operations. However, immobilized cells system presents some challenges such as mechanical strength of the carrier, diffusion of nutrients within carrier and leaching of cells.Citation10 In order to overcome from such problems, important parameters such as immobilized cell concentration and stirring needs to be investigated thoroughly during the fermentation process.
In the present study, efforts were made to investigate the influence of immobilized cells concentration and the stirring on the production of hemicellulosic ethanol from dilute acid hydrolysate of SB by Ca-alginate entrapped Scheffersomyces stipitis NRRL Y-7124.
Materials and Methods
Raw material and preparation of acid hydrolysate
Sugarcane bagasse was provided by the São Francisco Usina located in Sertãozinho/São Paulo, Brazil. Dilute acid pretreatment of SB was performed in a reactor of 100 L capacity. The SB was mixed with H2SO4 (98% purity) at a ratio of 100 mg H2SO4/ g of dry bagasse for 20 min at 121°C, using 10% substrate concentration.Citation11,12 After hydrolysis, the reaction mixture was cooled, recovered and was stored at 4°C. The hydrolysate was concentrated under a reduced pressure by a concentrator of 32L capacity at 70°C in order to obtain a xylose concentration of 30 g/L. This concentrated hydrolysate was subsequently detoxified following the method of Alves et al.Citation13 to remove inhibitors generated during dilute acid hydrolysis. This step involved 3 stages: raising the pH to 7.0 with calcium oxide; reducing the pH to 5.5 with phosphoric acid and adding activated charcoal (2.4% w/v) followed by incubation for 1h at 200 rpm and 30°C. After each stage, the hydrolysate was filtered under vacuum and finally it was autoclaved at 0.5 atm (110°C) for 15 min and was used in the fermentation reactions.
Microorganism and inoculum preparation
The inoculum of S. stipitis was prepared by transferring a loopful of strain from the slant into 125 ml Erlenmeyer flasks containing 50 ml of medium composed of 30.0 g/L of xylose, 10.0 g/L of yeast extract and 20.0 g/L of peptone (pH: 5.5). The flasks were incubated in a rotatory shaker (Innova 4000 Incubator Shaker, New Brunswick Scientific, Enfield, CT, USA) at 200 rpm and 30°C for 24 h. After 24 h of incubation, broth was centrifuged (Q222TM284, Quimis Aparelhos Científicos Ltda, SP, Brazil) at 3000 xg for 10 min at room temperature, followed by washing with sterilized water and used for immobilization.
Cell immobilization
S. stipitis cells were immobilized by entrapment in calcium alginate gel. The cell suspension was added to a solution of sodium alginate (sodium salt of alginic acid from brown algae, Fluka analytical, Switzerland). Prior to cells addition, it was autoclaved at 111°C for 15 min. The cell suspension (3.0, 6.5 or 10.0 g/L of cells concentration) was added into the alginate solution (2%) and spheres of gel were produced by dripping this suspension in sterilized calcium chloride solution maintained under gentle stirring with a concentration of 0.1 M.Citation14 shows the method employed for the preparation of immobilized cells. The beads containing immobilized cells were maintained in calcium chloride solution at 4°C for 12 hours. After this period, the beads were washed by sterile distilled water and subsequently used in fermentative processes.
Fermentation medium and conditions
The hemicellulosic hydrolysate was supplemented with peptone (5.0 g/L), yeast extract (3.0 g/L), CaCl2 (0.1 g/L) and (NH4)2SO4 (2.0 g/L).Citation14 Fermentation assays were carried out in 125 ml Erlenmeyer flasks containing 40 ml of production medium and 10 ml of immobilized biocatalyst. Fermentation assays were performed in an incubator shaker at 30°C for 72 h at different stirring levels according to the design of experiment.
Determination of cell concentration and stirring effect
To investigate the influence of cell concentration and stirring and their interactions, full factorial design of experiments (22) were performed. The responsive variables were ethanol production (g/l), xylose to ethanol conversion yield (g/g) and ethanol productivity (g/l/h). Experiments using the parameters of the central point were carried out 4 times. shows the selected independent process variables with their coded value as per the experimental matrix of full 22 factorial design. The analysis of statistical tests was performed using the software- STATGRAPHICS 16.1.11 and DESIGN-EXPERT 8.0.7.1.
Table 1 Matrix of 22 full factorial design with 4 replicates at the central point to evaluate the influence of immobilized cell concentration (X1) and stirring (X2) on different response variables
Analytical methods
Xylose, glucose and ethanol concentration were analyzed by HPLC (Schimadzu LC-10 AD (Kyoto, Japan) with column equipped with BIO-RAD Aminex HPX-87H (300 × 7.8 mm) coupled to a detector of refractive index RID-6A, with eluent 0.01 N sulfuric acid at a flow rate of 0.6 mL·min−1, column temperature of 45°C and injected volume of 20 μL. Before the passing of samples with HPLC, the samples were filtered through a Sep Pak C18 filter. The concentration of cells was determined by turbidimetry using spectrophotometer (Beckman DU 640 B Fullerton, CA, USA) and correlated with the dry weight of cells (g/L) through a calibration curve.
Results
Considering the importance of continuous ethanol production by immobilized cells, a 22 full factorial was designed to investigate the effect of immobilized cell concentration and stirring. The results (volumetric ethanol production, g/l; ethanol yield, Yp/s and ethanol productivity, Qp) obtained after 48 hours of fermentation are shown in .
Table 2 Response variables of 22 full factorial design with 4 replications at the central point to evaluate the influence of immobilized cell concentration (X1) and stirring (X2) on ethanol production after 48 hours of process
In all experiments, it was observed that the yeast was able to assimilate the available xylose in the fermentation medium for the production of ethanol. Ethanol production showed substantially varying content from 4.62 g/L in assay#7 to 8.9 g/L in assay#2. The second experiment (+1 and -1 for cell concentration and stirring, respectively) stands out presenting the best results among the trials of the design of experiment, with ethanol yield (Yp/s) of 0.33 g/g and volumetric productivity (Qp) of 0.19 g/L.h. The estimated effects of each factor for responsive variables are shown in .
Table 3 Estimated effects of cell concentration (X1) and stirring (X2) on the response Variables
presents the analysis of variance (ANOVA) and significance level for the response variables analyzed. Stirring was significant at 95% of confidence level (p-value <0.05) for all studied response variables. Statistical analysis showed negative effect on all studied variables (ethanol production, Yp/s and Qp). Higher levels of ethanol (ethanol yield, Yp/s and ethanol productivity, Qp) was obtained at low levels of stirring (Fig. 2). The interaction between the factors was significant at 95% confidence level (p-value <0.05) for ethanol production and Qp. On the other hand, for the variable Yp/s, the interaction between cell concentration and stirring was significant at 90% confidence level (p-value<0.1).
Table 4 Analysis of variance and significance of the process variables- cell concentration (X1) and stirring (X2) for response variables (Ethanol production, ethanol yield, ethanol productivity and xylose consumption)
The effect of immobilized cell concentration was not significant at 95% confidence for any studied response variable (p-value>0.05). However, it was significant for ethanol and Qp, with 90% of confidence having a positive effect on these variables. This fact confirmed the negative effect on ethanol production due to the cumulative effect of interaction of the factors. shows the response surface and corresponding contour lines of investigated factors. It was observed that low stirring and higher cell concentrations had a positive effect on ethanol production.
Figure 2. Pareto charts of independent variables and their interactions on each response variable (ethanol production, ethanol yield and ethanol productivity).
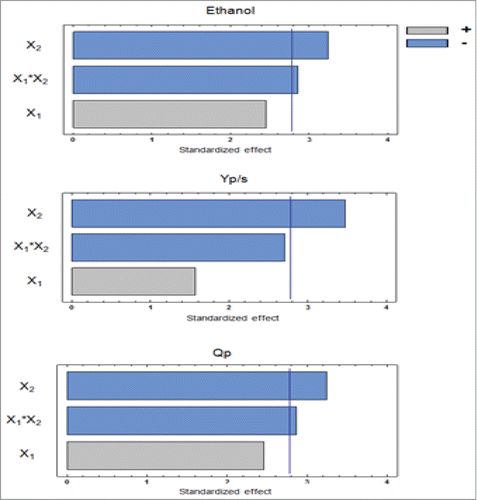
Figure 3. Response surface plots and corresponding contour lines showing the relationship between responsive variables (ethanol production, ethanol yield and ethanol productivity) and process variables (immobilized cell concentration and stirring).
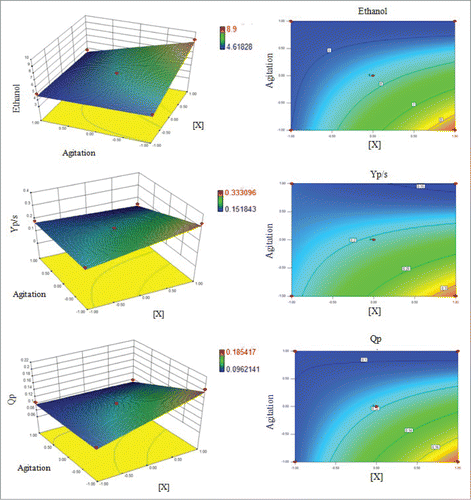
According to the analysis of variance (ANOVA), estimated effects and Pareto charts, it can be seen that stirring is the major factor that influences the process, being significant for all response variables. The interaction between stirring and cell concentration was found to be another important factor, which implies on fix fermentation stirring at its negative level (100 rpm). The use of higher cell concentrations in fermentation process is an important factor and in immobilized systems, higher amount of cells provide advantage since they provide higher yields and productivities within shorter fermentation times.Citation15
Chandel et al.Citation8 found that cells of Saccharomyces cerevisiae VS3 immobilized in Saccharum spontaneum stalks showed a maximum of 21.66 ± 0.62 g/L of ethanol with yield (0.43 ± 0.02 g/g) in the Ist cycle of fermentation which remained same up to 8th cycle of fermentation. After 8th cycle of fermentation, reduction in ethanol production was observed. The higher ethanol yield in immobilized cell systems is due to higher utilization of sugars from the lignocellulose hydrolysates due to the presence of high cell density in the carrier. The difference in ethanol productivity may also be attributed to the different environment and morphology of immobilized yeast cell mass and its effects on apparent viscosity of the medium that alters mass transfer and mixing characteristics.Citation16 shows microscope view of Ca-alginate matrix containing cells of S. stiptis after conditioning time. Yeast cells are deeply entrapped within the matrix, showing the feasibility of calcium alginate beads as a viable carrier for the repetitive use in fermentation cycles.
Figure 4. Microscope picture of a ca-alginate bead containing cells of immobilized S. stipites (400x).
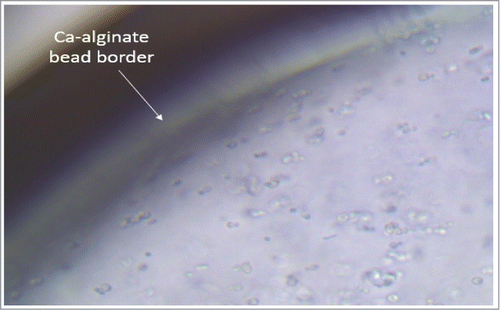
The advantage of applying higher cell concentration in fermentation processes is important parameters which affect ethanol production. Agbogbo et al.Citation17 observed that high initial cell concentration increase the rate of xylose assimilation and ethanol production by the free cells of the yeast S. stipitis CBS6054 in synthetic medium, showing ethanol yield of 0.38 g/g with 6.5 g/L of ethanol production. Matsushika and SawayamaCitation18 evaluated the influence of different initial concentrations of recombinant yeast S. cerevisiae MA-R4 on xylose assimilation and concluded that high cell concentrations lead to an increase in xylose assimilation and ethanol production. They also observed the maximum rate of xylose consumption and ethanol production (2.58 g/L.h to 0.83 g/L.h, respectively) using 13.1 g/L of free cells. However, in some cases, use of high cell concentrations may favor the formation of by-products such as xylitol, impairing the production of ethanol. In the present study, we did not observe the formation of polyol during the fermentation.
Stirring is a parameter of great importance in a fermentation process, interfering directly in the nutrients uptake rate and oxygen transfer.Citation19 The stirring provides better medium homogenization and maintain the gradient between the inside and outside of the cell, facilitating the entry of nutrients and gases exit and other products of cellular catabolism.Citation20 According to Skoog and Hahn-HägerdalCitation21 the available amount of oxygen in fermentation broth plays an important role in the metabolism of the yeast S. stipitis directly influencing the cell growth, redox balance, functionality of the mitochondria and the generation of energy for the transport of xylose. Gamarra et al.Citation22 also found that stirring is responsible for the rapid elimination of CO2 and ethanol from the spheres in immobilized systems.
Silva et al.Citation23 investigated the influence of the stirring on ethanol production by the yeast S. stipitis from rice straw hydrolysate and observed a deviation of yeast metabolism for the production of biomass with the increase of the agitation. The authors also found that the carbon source available in the process is divided between biomass formation and product formation, which is strongly influenced by the oxygen availability in the system. Rodmui et al.Citation20 analyzed the effect of different values of stirring (from 0 to 200 rpm) for ethanol production by the mixed culture of S. cerevisiae and Candida tropicalis from a mixture of commercial xylose and glucose. They observed that higher levels of ethanol were obtained at lower stirring, reaching maximum level at 50 rpm (Yp/s, 0.38 g/g). Thus, the low agitation rates (less than 100 rpm) may favor the high ethanol formation in relation to biomass formation. Low agitation is also important to minimize the damage of carrier ensuring the carrier immobilization stability due to lower shear tensions.
The assay#2 with the fixed conditions (10 g/L of cells and 100 rpm) showed the high ethanol production of 8.9 g/L, Yp/s of 0.33 g/g and Qp of 0.185 g/L.h. These values are very similar to other studies using the free cells of yeast S. stipitis. Scordia et al.Citation24 observed an ethanol yield of 0.33 g/g and a productivity of 0.17 g/L.h from giant reed (Arundo donax L.) hemicellulosic hydrolysate after 48 hrs of fermentation by free cells of S. stipitis CBS6054. Silva et al.Citation25 used the free cells of S. stipitis NRRL Y-7124 and obtained Yp/s of 0.33 g/g and Qp of 0.24 g/L.h from synthetic medium containing commercial xylose, glucose and arabinose. Canilha et al.Citation26 obtained a yield of 0.30 g/g and a productivity of 0.13 g/L.h of ethanol using free cells of S. stipitis DSM 3651 grown in SB hemicellulosic hydrolysate. Therefore, experimental conditions used in the present work can serve as a benchmark studies for the future work conducted with repeated batch or continuous process to improve yields and productivity.
Conclusion
Given the many challenges in bioconversion of hemicellulosic hydrolysates into ethanol with the appropriate yield, the use of immobilized cells shows the improvement in economically viable fermentation process. The stirring in the fermentation process is the crucial factor that influences the production of ethanol by immobilized cells. This parameter shows the extreme importance of oxygen availability to the microorganisms and stability of the immobilization support. In this study, S. stipitis NRRL Y-7124 showed maximum ethanol production (ethanol yield of 0.33 g/g and ethanol productivity of 0.185 g/l/h) from sugarcane bagasse hemicellulosic hydrolysate using 10.0 g/L of immobilized cells and 100 rpm.
Disclosure of Potential Conflicts of Interest
No potential conflicts of interest were disclosed.
Funding
Authors thank to Fundação de Amparo à Pesquisa do Estado de São Paulo (FAPESP–BIOEN Project grants: 2008/57926–4 and 2010/13828–9), Conselho Nacional de Desenvolvimento Científico e Tecnológico (CNPq) and Coordenação de Aperfeiçoamento de Pessoal de Nível Superior (CAPES) for the financial support.
References
- Goldemberg J. Ethanol for a sustainable energy future. Sci 2007; 315:808–810; http://dx.doi.org/10.1126/science.1137013
- Balat M, Balat H. Recent trends in global production and utilization of bio-ethanol fuel. Appl Ener 2009; 86: 2273–2282; http://dx.doi.org/10.1016/j.apenergy.2009.03.015
- Canilha L, Santos VTO, Rocha GJM, Silva JBA, Giulietti M, Silva SS, Felipe MGA, Ferraz A, Milagres AMF, Carvalho W. A study on the pretreatment of a sugarcane bagasse sample with dilute sulfuric acid. J Ind Microbiol Biotechnol 2011; 38:1467-1475; PMID:21210180; http://dx.doi.org/10.1007/s10295-010-0931-2
- Chandel AK, Silva SS, Carvalho W, Singh OV. Sugarcane bagasse and leaves: Foreseeable biomass of biofuel and bio-products. J Chem Technol Biotechnol 2012; 87: 11-20; http://dx.doi.org/10.1002/jctb.2742
- Rezende CA, Lima MA, Maziero P, Azevedo ER, Garcia W, Polikarpov I. Chemical and morphological characterization of sugarcane bagasse submitted to a delignification process for enhanced enzymatic digestibility. Biotechnol Biofuels 2011; 4:54; PMID:22122978; http://dx.doi.org/10.1186/1754-6834-4-54
- Canilha L, Chandel AK, Milessi TSS, Antunes FAF, Freitas WL, Felipe MGA, Silva SS. Bioconversion of sugarcane biomass into ethanol: An overview about composition, pretreatment methods, detoxification of hydrolysates, enzymatic saccharification, and ethanol fermentation. J Biomed Biotechnol 2012; 1-15; PMID:23251086; http://dx.doi.org/10.1155/2012/989572
- Lin TH, Huang GF, Guo GL, Hwang WS, Huang SL. Pilot-scale ethanol production from rice straw hydrolysates using xylose-fermenting Pichia stipitis. Bioresour Technol 2012; 116:314-319; PMID:22537402; http://dx.doi.org/10.1016/j.biortech.2012.03.089
- Chandel AK, Narasu ML, Chandrasekhar G, Manikeyam A, Rao LV. Use of Saccharum spontaneum (wild sugarcane) as biomaterial for cell immobilization and modulated ethanol production by thermotolerant Saccharomyces cerevisiae VS3. Bioresour Technol 2009; 100: 2404-2410; PMID:19114303; http://dx.doi.org/10.1016/j.biortech.2008.11.014
- Zhao J, Xia L. Ethanol production from corn stover hemicellulosic hydrolysate using immobilized recombinant yeast cells. Biochem Eng J 2010; 49: 28-32; http://dx.doi.org/10.1016/j.bej.2009.11.007
- Carvalho W, Canilha L, Silva SS. Semi-continuous xylose-to-xylitol bioconversion by Ca-alginate entrapped yeast cells in a stirred tank reactor. Bioproc Biosystem Eng 2008; 31:493-498; http://dx.doi.org/10.1007/s00449-007-0187-z
- Silva DDV, Felipe MGA, Mancilha IM, Silva SS. Evaluation of inoculum of Candida guilliermondii grown in presence of glucose on xylose reductase and xylitol dehydrogenase activities and xylitol production during batch fermentation of sugarcane bagasse hydrolysate. Appl Biochem Biotechnol 2005; 121-124: 427-437; PMID:15917619
- Milessi TSS, Antunes FAF, Chandel AK, Silva SS. Rice bran extract: an inexpensive nitrogen source for the production of 2G ethanol from sugarcane bagasse hydrolysates. 3 Biotech 2013; 3:373-379; http://dx.doi.org/10.1007/s13205-012-0098-9
- Alves LA, Felipe MGA, Silva JBA, Silva SS, Prata AMR. Pretreatment of sugarcane bagasse hemicellulose hydrolisate for xylitol production by Candida guilliermondii. Appl Biochem Biotechnol 1998; 70/72:89-98; PMID:9779572; http://dx.doi.org/10.1007/BF02920126
- Milessi TSS, Chandel AK, Antunes FAF, Silva SS. Immobilization of Scheffersomyces stipitis cells with calcium alginate beads: A sustainable method for hemicellulosic ethanol production from sugarcane bagasse hydrolysate. Bioethanol 2013; 1-8. http://dx.doi.org/10.2478/bioeth-2013-0002
- Nagodawawithana TW, Steinkraus KH. Influence of the rate of ethanol production and accumulation on the viability of Saccharomyces cerevisiae in “Rapid Fermentation”. Appl Env Microbiol 1976; 31:158-162.
- Johansen CL, Coolen L, Hunik JH. Influence of morphology on product formation in Aspergillus awamori during submerged fermentation. Biotechnol Prog 1998; 14: 233-240; PMID:9548774; http://dx.doi.org/10.1021/bp980014x
- Agbogbo FK, Coward-Kelly G, Torry-Smith M, Wenger K, Jeffries TW. The effect of initial cell concentration on xylose fermentation by Pichia stipitis. Appl Biochem Biotechnol 2007; 136/140:653-662.
- Matsushika A, Sawayama S. Effect of initial cell concentration on ethanol production by flocculent Saccharomyces cerevisiae with xylose-fermenting ability. Appl Biochem Biotechnol 2010; 162:1952-1960; PMID:20432070; http://dx.doi.org/10.1007/s12010-010-8972-6
- Liu R, Shen F. Impacts of main factors on bioethanol fermentation from stalk juice of sweet sorghum by immobilized Saccharomyces cerevisiae (CICC 1308). Bioresour Technol 2008; 99: 847-854; PMID:17360181; http://dx.doi.org/10.1016/j.biortech.2007.01.009
- Rodmui A, Kongkiattikajorn J, Dandusitapun Y. Optimization of agitation conditions for maximum ethanol production by co-culture. Kasetsart J 2008; 42:285-293.
- Skoog K, Hahn-Hagerdahl B. Effect of oxygenation on xylose fermentation by Pichia stipitis. Appl Environ Microbiol 1990; 56:3389-3394; PMID:16348343
- Gamarra JA, Cuevas CM, Lescano G. Production of ethanol by a stirred catalystic basket reactor with immobilized yeast cells. J Ferm Technol 1986; 64:25-28; http://dx.doi.org/10.1016/0385-6380(86)90053-1
- Silva JPA, Mussato SI, Roberto IC. The influence of initial xylose cencentration, agitation, and aeration on ethanol production by Pichia stipitis from rice straw hemicellulosic hydrolysate. Appl Biochem Biotechnol 2010; 162:1306-1315; PMID:19946760; http://dx.doi.org/10.1007/s12010-009-8867-6
- Scordia D, Cosentino SL, Lee JW, Jeffries TW. Bioconversion of giant reed (Arundo donax L.) hemicelluloses hydrolysate to ethanol by Scheffersomyces stipitis CBS 6054. Biomass Bioener 2012; 39:296-305; http://dx.doi.org/10.1016/j.biombioe.2012.01.023
- Silva JPA, Mussatto SL, Roberto IC, Teixeira JA. Fermentation medium and oxygen transfer conditions that maximize the xylose conversion to ethanol by Pichia stipitis. Renewable Ener 2012; 37: 259-265; http://dx.doi.org/10.1016/j.renene.2011.06.032
- Canilha L, Carvalho W, Felipe MGA, Silva JBA, Giulietti M. Ethanol production from sugarcane bagasse hydrolysate using Pichia stipitis. Appl Biochem Biotechnol 2010; 161:84-92; PMID:19802721; http://dx.doi.org/10.1007/s12010-009-8792-8