Abstract
Adipocytes differentiate and function in environments rich in extracellular matrix (ECM) proteins. The phenotypes of genetically modified mice have aided in recognizing the importance of ECM proteins and their modifiers, e.g., proteinases, in the regulation of obesity and metabolism. Most of the molecular mechanisms through which ECM proteins and modifiers regulate adipogenesis or adipocyte function have not been fully defined. Adipose tissue fibrosis may be a factor that links obesity to diabetes or cardiovascular disease risk in conjunction with tissue inflammation. Defining the molecular mechanisms through which the ECM environment regulates adipogenesis and adipocyte function should provide us with a better understanding of the disease link between obesity and diabetes or cardiovascular diseases.
Keywords: :
Adipose Tissue Fibrosis and Inflammation
Obesity increases the risk of diabetes, cardiovascular diseases and cancer. The molecular mechanisms underlying the link between obesity and the risk of developing metabolic and cardiovascular diseases, however, are still enigmatic. In addition to obesity, the loss of fat tissue (lipodystrophy) increases the risk of diabetes and cardiovascular diseases. The U- or J-curve relationship between body mass index (BMI) and the risk of diabetes and cardiovascular diseasesCitation1,Citation2 suggests the important role played by “balanced adiposity” in maintaining metabolic health. Why do the two opposite extremes of adiposity similarly increase the risk of diabetes and cardiovascular diseases? Some hints may be found in the biological processes common to both obesity and lipodystrophy. Among an array of biological processes, inflammation and fibrosis are two candidate biological processes that may explain the metabolic consequences of obesity and lipodystrophy.
In adipose tissues, mature adipocytes and their progenitor cells (preadipocytes) exist within a three-dimensional (3D) network of ECM proteins. Adipose tissue function is regulated by the physiological interaction between cells and a variety of ECM proteins. The collagen family is the largest group of ECM proteins.Citation3 The density and structure of collagens in organs are tightly regulated. The excess deposition of collagens in individuals with pathological conditions is defined as fibrosis, a hallmark of chronic tissue damage. Unlike the existence of fibrosis in the skin, lungs, liver or kidneys, the existence of fibrosis in adipose tissue has not been fully recognized. Recent reports, however, suggest that obese individuals display excess collagen deposition in adipose tissues.Citation4-Citation6 Notably, the excess collagen deposition in adipose tissues was observed along with inflammatory tissue damage, which is characterized by the infiltration of neutrophils, lymphocytes and macrophages. Thus, fibrotic tissue damage is perceived by many as a process secondary to tissue inflammation, whose pathological impact on obesity and metabolism has been extensively studied in recent years.Citation7-Citation9 Adding a layer of complexity to the comprehensive understanding of adipose tissue biology, inflammatory tissue damage characterized by macrophage infiltration can also be found in lipodystrophy.Citation10 Likewise, adipose tissue fibrosis has been observed in individuals with congenital lipodystrophy and in the dystrophic interscapular fat pads of partially lipodystrophic patients.Citation11,Citation12 Of note, LMNA mutations reported in the latter group lead to the paradoxical expansion of interscapular fat pads, which display fibrotic changes but not evidence of ongoing inflammatory processes, suggesting the possibility that fibrosis and inflammation are not always coupled.Citation12 The causal and temporo-spatial relationships between adipose tissue fibrosis and inflammation remain to be defined in the contexts of obesity and lipodystrophy. Despite these unresolved questions, a series of studies using gene targeting in rodents and mechanistic experiments at the cellular level have helped elucidate some of the genetic and molecular mechanisms involved in the regulation of adipose tissue ECM remodeling and function.
MMP-Dependent Type I Collagen Turnover during Adipose Tissue Development
Adipocytes differentiate and function in vivo within a 3D environment surrounded by a number of extracellular matrix (ECM) proteins ( and ). In the adipose tissue primordium, preadipocytes progressively change cell shape and accumulate lipid droplets in a space juxtaposed to collagen bundles.Citation13 Among the ECM proteins, collagens are the most abundant proteins that constitute interstitial fibers and pericellular basement membranes.Citation3 Type I collagen molecules, which exist mainly as [α1(I)]2α2(I) heterotrimers in a triple helix, are staggered and interwoven with each other to form thick collagen bundles.Citation14 Type I collagen bundles provide the major ECM framework necessary to sustain the structure and function of mesenchymal tissues. Although triple-helical type I collagen is highly resistant to proteolytic degradation, it can be cleaved and degraded by a set of matrix metalloproteinases (MMPs) in certain stages of development and chronic disease progression.Citation15 Of the 28 members of the MMP family,Citation16 MMP14 (membrane type 1 matrix metalloproteinase, MT1-MMP) plays a major role in the postnatal development of mesenchymal tissues, particularly bones and adipose tissues.Citation17,Citation18 The dominant role played by MMP14 is attributable to its collagenolytic activity tethered to the cell surface. Although the soluble collagenases of rodents, i.e., MMP2, MMP8, MMP13 and Mcol1a (an ortholog of human MMP1), are secreted as inactive zymogens, MMP14 is expressed as an active enzyme on the cell surface, as its inhibitory pro-domain is intracellularly removed by furin or furin-like proprotein convertases.Citation19,Citation20
Figure 1. Peri-adipocyte collagens. Left: the scanning electron micrograph of mouse inguinal fat pads; the group of round adipocytes are surrounded with collagen bundles. Middle: immunofluorescent staining of type I collagen (red); thick bundles of type I collagen surrounding the group of adipocytes as well as thinner fibers of type I collagen enwrapping individual adipocyte is displayed. Right: immunofluorescent staining of type IV collagen (green); type IV collagen is found as a component of basement membrane that enwraps each adipocytes; type IV collagen can also be found as the basement membrane underneath the layer of vascular endothelial cells.
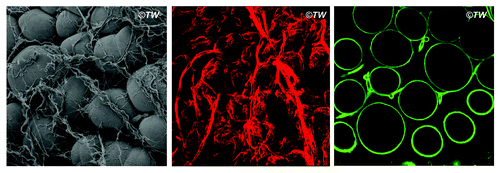
Figure 2. Peri-adipocyte ECM proteins. Each adipocyte is surrounded by basement membrane whose framework is defined by type IV collagen. The most abundant fibrillar structure is provided by the cross-linking of triple-helical type I collagen molecules (enlarged in the inset). Types V and VI collagens form micro-fibers that exist between type I collagen fibers as well as between cell surface and type I collagen fibers. Fibronectin is the key ECM protein that defines cell shape and contractility in close association with type I collagen. Matricelluar proteins, i.e., thrombospondins and SPARC, regulate the fibrilliogenesis of type I collagen and interact with multiple ECM proteins.
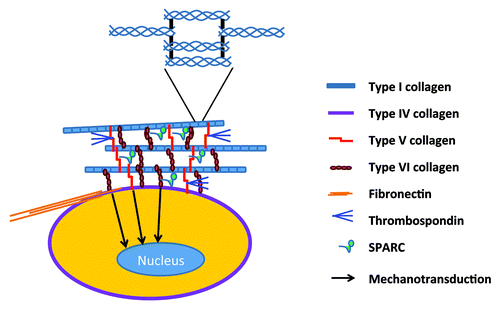
The complete loss of Mmp14 in mice leads to postnatal lipodystrophy and leptin-null status.Citation18 In vitro studies suggest that MMP15 (MT2-MMP) possesses pericellular collagenase activity similar to that of MMP14;Citation21-Citation23 however, the lipodystrophic phenotype of Mmp14-null mice is not rescued by Mmp15 because MMP15 is rarely expressed in adipose tissues. Cell-autonomous defects in adipogenic potential were confirmed by the observations of impaired in vitro adipogenesis within a 3D collagen environment and defective in vivo adipogenesis when Mmp14-null preadipocytes were transplanted into an MMP14-sufficient host.Citation18 Of note, Mmp14 is largely dispensable for adipogenesis under 2D culture conditions, suggesting that the interaction of MMP14 with type I collagen, particularly in a 3D environment, plays a central role in regulating adipocyte maturation. As such, MMP14 can be defined as an in vivo factor that is necessary for adipocyte maturation ().
Figure 3. MMP14 (MT1-MMP) as a 3D factor. MMP14 is required for the cytoskeletal rearrangement of preadipocytes in a 3D collagen environment (3D preadipocytes). MMP14-dependent cell shape regulation is closely linked to the adipogenic potential of preadipocytes in a 3D environment (3D adipocytes). The loss of MMP14 activity leads to the aberrant cell shape of preadipocytes and impaired 3D adipogenesis, which is coupled with the excess accumulation of undigested collagen fibers. MMP14 is not required for adipogenesis under 2D culture conditions (2D adipogenesis).
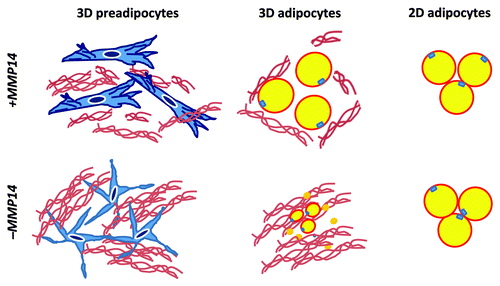
The inextricable relationship between the MMP family proteins and type I collagen has been further underscored by the developmental defects observed in mice that harbor an MMP-resistant Col1a1 gene mutation.Citation24,Citation25 This knock-in mutation (Col1a1r/r) renders triple-helical type I collagen molecules resistant to MMP-dependent hydrolysis and impairs anabolic bone remodeling.Citation25,Citation26 Although the gene targeting of Mmp2, which encodes the most abundant collagenase/gelatinase found in tissues and the circulation, results in only a subtle skeletal growth defect,Citation27 Mmp2 gene targeting in mice harboring the MMP-resistant mutant Col1a1 gene leads to a profound defect in postnatal development; this effect is similar to that observed with Mmp14-null mice.Citation28 MMP2 is activated by MMP14 on the cell surfaceCitation29 in a manner that depends on the physical interactions between MMP2, MMP14 and an endogenous MMP inhibitor, TIMP2.Citation30 MMP2 can also be activated by the other membrane-type MMPs, e.g., MMP15 (MT2-MMP) and MMP16 (MT3-MMP); indeed, the activation of MMP2 under normal conditions is barely affected by the Mmp14-null state in rodents.Citation31 These complex results suggest that the phenotypes observed in Mmp14-null mice are mostly independent of Mmp2; however, the proteolytic activity mediated by MMP2 may play a qualitatively distinct role, beyond that of collagenolysis, in certain stages of development and disease. Consistent with the non-overlapping roles played by MMP2 and MMP14, Mmp2 and Mmp14 double-knockout mice die at birth, which is a significantly more severe and lethal phenotype than that of Mmp14-null mice.Citation32 It is conceivable, however, that the roles played by MMPs in cleaving substrates other than ECM proteins, particularly chemokines and cytokines, may complicate the phenotypic consequences of targeting each MMP gene.Citation33-Citation35 Mmp2-deficient mice are more resistant to high-fat diet-induced weight gain than littermate wild-type miceCitation36; however, the direct role of MMP2 in regulating adipose tissue ECM remodeling and function is undefined.
Type V and VI Collagens in Adipose Tissue Development and Obesity
Type V collagen molecules exist in the form of [α1(V)]2α2(V) or α1(V)α2(V)α3(V) heterotrimers.Citation37 These molecules constitute micro-fibers that are closely associated with thick type I collagen bundles.Citation38 Genetic mutations in Col5a1 and Col5a2 are found in families with Ehlers-Danlos syndrome,Citation39 underscoring the role played by type V collagen in regulating the elastic resilience of connective tissues in association with type I collagen. A previous study has shown that the loss of Col5a3, which is highly expressed in adipose tissues, muscles and pancreatic islets, leads to a reduced size of pancreatic islets and adipose tissues.Citation40 In that study, Col5a3-null adipocytes and skeletal muscles displayed insulin resistance due to the suppression of insulin-induced Glut4 translocation. The molecular mechanism through which the loss of Col5a3 negatively impacts adipocyte size and function, however, is undefined. In addition to modifying the tensile strength of type I collagen fibers, type V collagen also binds to thrombospondin 1, a matricellular ECM protein that is abundant in connective tissues and the circulation.Citation41 It is conceivable that type V collagen heterotrimers regulate the composition and density of ECM proteins in adipose tissues through their interactions with multiple ECM proteins.
Type VI collagen heterotrimers form microfibrils in the interface between the basement membrane and thick bundles of type I collagens.Citation42-Citation44 Col6a1-null mice are born and develop normally with no gross abnormalities.Citation45 As observed in Bethlem myopathy, which is linked to 2q37 or Col6a mutations in humans,Citation46,Citation47 these mice display a mild form of necrotizing myopathy. When Col6a1-deficient mice were crossed with ob/ob mice, Col6a1 deficiency was demonstrated to protect mice from the high-fat diet-induced impairment of glucose metabolism.Citation48 Although Col6a1-deficient ob/ob mice display a lower body weight relative to wild-type mice in the early stages of postnatal development, the fat mass of Col6a1-deficient ob/ob mice increases faster and catches up with that of ob/ob mice at 12 weeks of age. Although the consequence of whole-body Col6a1 deficiency is complex, affecting multiple organs and displaying reduced food intake and energy expenditure, it has been suggested that type VI collagen is a fibrotic component that restricts adipose tissue expandability. In humans, Col6a3 gene expression in adipose tissues was found to correlate with visceral adipose tissue mass and pro-inflammatory gene expression.Citation49 The causal roles played by type VI collagen in regulating adipogenesis, adipocyte hypertrophy, or adipocyte function, however, have not been fully addressed in vitro.
Non-Collagen ECM Proteins in Adipocyte Biology
Fibronectin inhibits 3T3 adipogenesis in conjunction with the regulation of cell shape and stretch.Citation50 The pericellular assembly of fibronectin molecules dictates cytoskeletal and ECM organization.Citation51 Fibronectin plays a critical role in embryonic mesoderm development and cardiovascular morphogenesis.Citation52,Citation53 Recently, Wang et al. demonstrated that soluble Pref-1 (DLK1) released from the cell surface interacts with fibronectin and inhibits adipogenesis by activating the Rac1-Erk1/2 pathway.Citation54 Pref-1 was initially identified as a member of the EGF-like family of proteins, which is highly expressed in preadipocytes and inhibits adipocyte differentiation.Citation55 The novel interaction identified between Pref-1 and fibronectin,Citation56 which is the major ligand of α5β1 integrin,Citation57 is intriguing in understanding the molecular mechanisms through which the interaction between cells and pericellular ECM proteins regulates adipogenesis and adipocyte function. Moreover, fibronectin assembly plays a key role in maintaining the fibrillar organization of type I collagen and thrombospondin 1.Citation58 Fibronectin can be cross-linked to an α1(I) collagen chain through factor XIIIa.Citation59 This close molecular interaction between fibronectin and type I collagen may play a major role in the ECM interactomes that may regulate adipocyte function. The most recent study from Spiegelman’s group suggests a role of a myokine, termed Irisin, in inducing the “browning” of subcutaneous fat and thermogenesis.Citation60 Irisin is a proteolytically cleaved fragment of Fndc5 mostly comprising a type III fibronectin domain (Fn3), which is commonly found in ECM proteins and cell surface receptors.Citation61 Fn3 promotes cell adhesion via activated β1 integrin.Citation62 Although the molecular mechanism through which Irisin induces the “browning” of adipose tissues remains undefined, it is intriguing to speculate that this molecule may regulate adipocyte function by fine-tuning cell-cell or cell-ECM interactions.
Secreted protein, acidic and rich in cysteine (SPARC) is a matricellular ECM proteinCitation63 that indirectly contributes to the formation of the structural framework of mesenchymal tissues. Sparc-null mice display increased adipose tissue size and higher expandability in response to the consumption of a high-fat diet.Citation64,Citation65 The phenotype is attributable, at least partly, to the role played by SPARC in regulating the density and structure of type I collagen fibrils.Citation66,Citation67 Conversely, the loss of Col1a1 gene expression in fibroblasts (embryonic fibroblasts isolated from Mov-13 mice) impairs the extracellular accumulation of SPARC but not the extracellular accumulation of fibronectin or type III collagen.Citation67 Moreover, the loss of SPARC interferes with the cell-mediated contraction of collagen gels, which can be rescued with exogenously added recombinant SPARC protein, suggesting that SPARC plays paracrine and endocrine roles. SPARC was found to suppress adipogenesis and promote osteogenesis by regulating the intra-nuclear content of β-catenin.Citation68 Thus, SPARC, in association with type I collagen, can be a potent modifier of Wnt signaling in adipocytes, which is a major pathway in the regulation of adipogenesis and adipocyte gene expression.Citation69,Citation70
Thrombospondin 1 and 2 (THBS1 and THBS2) belong to the group of matricellular ECM proteins.Citation63 THBS1 is a large glycoprotein that contains multiple functional domains, including a coiled-coil domain for oligomerization, an N-terminal laminin G-like domain (LG), a thrombospondin type 1 repeat (TSR), an epidermal growth factor-like domain, type 3 repeats and a C-terminal lectin-like domain. THBS1 binds to a number of ECM proteins, cell surface proteins, and growth factors. THBS1 binds to collagens, particularly type V collagenCitation41; however, the metabolic significance of this interaction is undefined. Given the positive role of type V collagen in adipose tissue development,Citation40 the specific interaction between THBS1 and type V collagen could be a biological modifier of adipocyte function. THBS1 expression in adipose tissue is significantly elevated in obese diabetic humans, suggesting its role in the progression of metabolic syndrome.Citation71 The loss of Thbs1 protects mice from diet-induced obesityCitation72; however, the role of Thbs1 in regulating peripheral insulin resistance remains elusive in light of the complex effects exerted by this protein on multiple organs and tissues, including pancreatic islets.Citation73 The effect of the Thbs1 gene on obesity and metabolism appears to be variable depending on genetic and environmental factors; another group reported a subtle effect or almost no effect of Thbs1 gene targeting on adipose tissue size.Citation74 THBS2 shares most of the functional domains found in THBS1 except for the N-terminal domain, which displays distinct sequences. Thbs2-null mice have fragile skin and an abnormal thickening of cortical bones in association with dysregulated type I collagen fibrillogenesis.Citation75 A previous study showed that Thbs2-null mesenchymal cells displayed better lipid accumulation than wild-type cells during adipogenesis despite the gene expression of adipocyte markers being almost identical to that of wild-type cells.Citation76 In this study, increased fat mass was selectively found in female mice fed the control diet but not those fed a high-fat diet, and no significant difference was found in male mice under any nutritional conditions. Another group demonstrated that Thbs2-null male mice are modestly protected from high-fat diet-induced obesity, but no metabolic benefit was observed.Citation77 Despite these studies, the roles played by either THBS1 or THBS2 in the regulation of adipogenesis and adipocyte function remain to be defined at the molecular and cellular levels.
Matrix Elasticity and Geometry in Adipocyte Function
Recently, the role of geometrical constraints in regulating cell fate and differentiation has been highlighted. The differentiation of human mesenchymal cells into the cells of adipocyte or osteoblast lineage can be controlled by cell shape.Citation78 Stretched cells with a flattened cell shape differentiate more easily into osteoblasts, whereas small and round-shaped cells differentiate into adipocytes. The study showed that the cell shape-dependent regulation of lineage commitment was reversed by dominant-negative or active RhoA, suggesting that the effects of cell shape serve as upstream initiators of RhoA-dependent mechanotransduction. Nonetheless, it is difficult to determine the specific effect of matrix elasticity or 3D geometry in the in vivo environment that is intricately regulated by ECM protein composition and density.Citation79 Despite the importance of physical constraints imposed by a 3D meshwork of collagen, the layer of collagen itself can exert inhibitory effects on the induction of a set of adipocyte genes in conjunction with suppressed pro-transcriptional histone mark modification.Citation80 The use of non-ECM nanomaterials that may bypass the interaction between cells and ECM proteins may aid in dissecting the signaling pathways that are regulated by physical parameters, such as cell shape, force, and pressure.
Future Directions
The critical role played by ECM proteins and their modifying enzymes (proteinases) in the regulation of adipogenesis and adipocyte function has been recognized in animal models. Gene targeting or knockdown has been used to determine the biological role of ECM proteins and proteinases. The pericellular ECM composition and its remodeling are physically linked to the cytoskeleton, which is further linked to the nuclear structure. This hard-wired interaction between ECM proteins and nuclear structure is considered to play a central role in the gene regulation and phenotype switching of cells during their adaptation to the tissue environment. Due to the multiple players and interactions in ECM biology, we must advance our understanding of the complex cell-ECM interactome by combining the evolving system biology and discovery-oriented reductionist approaches. The comprehensive understanding of the genetic architecture that underlies the interactomes of ECM proteins and their modifiers should help us define the pathogenesis of adipose tissue fibrosis and inflammation, which are closely linked to diabetes and cardiovascular diseases ().
Figure 4. Nutritional injury and genetics in adipose tissue pathology. Nutritional injury, such as high-fat diet, unravels genetic predispositions of individuals. Adipose tissues can maintain healthy tissue function through physiological tissue regeneration in response to nutritional injury; however, in certain individuals, adipose tissues are genetically predisposed to fibrosis (overhealing) or inflammation (chronic wound).
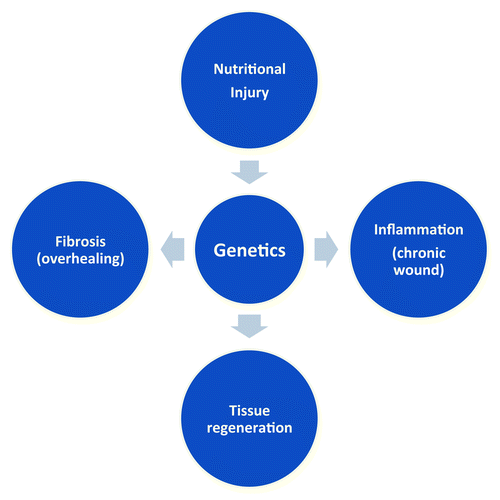
Acknowledgments
I thank Mayumi Inoue at the University of Michigan for discussion. The scanning electron micrograph was obtained at University of Michigan Microscopy and Image Analysis Laboratory (MIL). This work was supported by grants from National Institutes of Health (K08DK078801 and R03DK091433 to T.-H. Chun and P60DK020572 to the Michigan Diabetes Research Training Center) and Department of Internal Medicine, University of Michigan (to T.-H.C.).
References
- Zheng W, McLerran DF, Rolland B, Zhang X, Inoue M, Matsuo K, et al. Association between body-mass index and risk of death in more than 1 million Asians. N Engl J Med 2011; 364:719 - 29; http://dx.doi.org/10.1056/NEJMoa1010679; PMID: 21345101
- Berrington de Gonzalez A, Hartge P, Cerhan JR, Flint AJ, Hannan L, MacInnis RJ, et al. Body-mass index and mortality among 1.46 million white adults. N Engl J Med 2010; 363:2211 - 9; http://dx.doi.org/10.1056/NEJMoa1000367; PMID: 21121834
- Ricard-Blum S. The collagen family. Cold Spring Harb Perspect Biol 2011; 3:a004978; http://dx.doi.org/10.1101/cshperspect.a004978; PMID: 21421911
- Henegar C, Tordjman J, Achard V, Lacasa D, Cremer I, Guerre-Millo M, et al. Adipose tissue transcriptomic signature highlights the pathological relevance of extracellular matrix in human obesity. Genome Biol 2008; 9:R14; http://dx.doi.org/10.1186/gb-2008-9-1-r14; PMID: 18208606
- Divoux A, Tordjman J, Lacasa D, Veyrie N, Hugol D, Aissat A, et al. Fibrosis in human adipose tissue: composition, distribution, and link with lipid metabolism and fat mass loss. Diabetes 2010; 59:2817 - 25; http://dx.doi.org/10.2337/db10-0585; PMID: 20713683
- Sbarbati A, Osculati F, Silvagni D, Benati D, Galiè M, Camoglio FS, et al. Obesity and inflammation: evidence for an elementary lesion. Pediatrics 2006; 117:220 - 3; http://dx.doi.org/10.1542/peds.2004-2854; PMID: 16396883
- Schenk S, Saberi M, Olefsky JM. Insulin sensitivity: modulation by nutrients and inflammation. J Clin Invest 2008; 118:2992 - 3002; http://dx.doi.org/10.1172/JCI34260; PMID: 18769626
- Lumeng CN, Saltiel AR. Inflammatory links between obesity and metabolic disease. J Clin Invest 2011; 121:2111 - 7; http://dx.doi.org/10.1172/JCI57132; PMID: 21633179
- Gregor MF, Hotamisligil GS. Inflammatory mechanisms in obesity. Annu Rev Immunol 2011; 29:415 - 45; http://dx.doi.org/10.1146/annurev-immunol-031210-101322; PMID: 21219177
- Herrero L, Shapiro H, Nayer A, Lee J, Shoelson SE. Inflammation and adipose tissue macrophages in lipodystrophic mice. Proc Natl Acad Sci U S A 2010; 107:240 - 5; http://dx.doi.org/10.1073/pnas.0905310107; PMID: 20007767
- Gandotra S, Le Dour C, Bottomley W, Cervera P, Giral P, Reznik Y, et al. Perilipin deficiency and autosomal dominant partial lipodystrophy. N Engl J Med 2011; 364:740 - 8; http://dx.doi.org/10.1056/NEJMoa1007487; PMID: 21345103
- Béréziat V, Cervera P, Le Dour C, Verpont M-C, Dumont S, Vantyghem M-C, et al, Lipodystrophy Study Group. LMNA mutations induce a non-inflammatory fibrosis and a brown fat-like dystrophy of enlarged cervical adipose tissue. Am J Pathol 2011; 179:2443 - 53; http://dx.doi.org/10.1016/j.ajpath.2011.07.049; PMID: 21945321
- Napolitano L. The differentiation of white adipose cells: an electron microscopy study. J Cell Biol 1963; 18:663 - 79; http://dx.doi.org/10.1083/jcb.18.3.663; PMID: 14064115
- Perumal S, Antipova O, Orgel JPRO. Collagen fibril architecture, domain organization, and triple-helical conformation govern its proteolysis. Proc Natl Acad Sci U S A 2008; 105:2824 - 9; http://dx.doi.org/10.1073/pnas.0710588105; PMID: 18287018
- Stamenkovic I. Extracellular matrix remodelling: the role of matrix metalloproteinases. J Pathol 2003; 200:448 - 64; http://dx.doi.org/10.1002/path.1400; PMID: 12845612
- Greenlee KJ, Werb Z, Kheradmand F. Matrix metalloproteinases in lung: multiple, multifarious, and multifaceted. Physiol Rev 2007; 87:69 - 98; http://dx.doi.org/10.1152/physrev.00022.2006; PMID: 17237343
- Holmbeck K, Bianco P, Caterina J, Yamada S, Kromer M, Kuznetsov SA, et al. MT1-MMP-deficient mice develop dwarfism, osteopenia, arthritis, and connective tissue disease due to inadequate collagen turnover. Cell 1999; 99:81 - 92; http://dx.doi.org/10.1016/S0092-8674(00)80064-1; PMID: 10520996
- Chun TH, Hotary KB, Sabeh F, Saltiel AR, Allen ED, Weiss SJ. A pericellular collagenase directs the 3-dimensional development of white adipose tissue. Cell 2006; 125:577 - 91; http://dx.doi.org/10.1016/j.cell.2006.02.050; PMID: 16678100
- Yana I, Weiss SJ. Regulation of membrane type-1 matrix metalloproteinase activation by proprotein convertases. Mol Biol Cell 2000; 11:2387 - 401; PMID: 10888676
- Pei D, Weiss SJ. Furin-dependent intracellular activation of the human stromelysin-3 zymogen. Nature 1995; 375:244 - 7; http://dx.doi.org/10.1038/375244a0; PMID: 7746327
- Chun TH, Sabeh F, Ota I, Murphy H, McDonagh KT, Holmbeck K, et al. MT1-MMP-dependent neovessel formation within the confines of the three-dimensional extracellular matrix. J Cell Biol 2004; 167:757 - 67; http://dx.doi.org/10.1083/jcb.200405001; PMID: 15545316
- Sabeh F, Li XY, Saunders TL, Rowe RG, Weiss SJ. Secreted versus membrane-anchored collagenases: relative roles in fibroblast-dependent collagenolysis and invasion. J Biol Chem 2009; 284:23001 - 11; http://dx.doi.org/10.1074/jbc.M109.002808; PMID: 19542530
- Ota I, Li X-Y, Hu Y, Weiss SJ. Induction of a MT1-MMP and MT2-MMP-dependent basement membrane transmigration program in cancer cells by Snail1. Proc Natl Acad Sci U S A 2009; 106:20318 - 23; http://dx.doi.org/10.1073/pnas.0910962106; PMID: 19915148
- Wu H, Byrne MH, Stacey A, Goldring MB, Birkhead JR, Jaenisch R, et al. Generation of collagenase-resistant collagen by site-directed mutagenesis of murine pro alpha 1(I) collagen gene. Proc Natl Acad Sci U S A 1990; 87:5888 - 92; http://dx.doi.org/10.1073/pnas.87.15.5888; PMID: 2165607
- Zhao W, Byrne MH, Wang Y, Krane SM. Osteocyte and osteoblast apoptosis and excessive bone deposition accompany failure of collagenase cleavage of collagen. J Clin Invest 2000; 106:941 - 9; http://dx.doi.org/10.1172/JCI10158; PMID: 11032854
- Zhao W, Byrne MH, Boyce BF, Krane SM. Bone resorption induced by parathyroid hormone is strikingly diminished in collagenase-resistant mutant mice. J Clin Invest 1999; 103:517 - 24; http://dx.doi.org/10.1172/JCI5481; PMID: 10021460
- Itoh T, Ikeda T, Gomi H, Nakao S, Suzuki T, Itohara S. Unaltered secretion of β-amyloid precursor protein in gelatinase A (matrix metalloproteinase 2)-deficient mice. J Biol Chem 1997; 272:22389 - 92; http://dx.doi.org/10.1074/jbc.272.36.22389; PMID: 9278386
- Egeblad M, Shen HCJ, Behonick DJ, Wilmes L, Eichten A, Korets LV, et al. Type I collagen is a genetic modifier of matrix metalloproteinase 2 in murine skeletal development. Dev Dyn 2007; 236:1683 - 93; http://dx.doi.org/10.1002/dvdy.21159; PMID: 17440987
- Sato H, Takino T, Okada Y, Cao J, Shinagawa A, Yamamoto E, et al. A matrix metalloproteinase expressed on the surface of invasive tumour cells. Nature 1994; 370:61 - 5; http://dx.doi.org/10.1038/370061a0; PMID: 8015608
- Wang Z, Juttermann R, Soloway PD. TIMP-2 is required for efficient activation of proMMP-2 in vivo. J Biol Chem 2000; 275:26411 - 5; http://dx.doi.org/10.1074/jbc.M001270200; PMID: 10827175
- Filippov S, Koenig GC, Chun T-H, Hotary KB, Ota I, Bugge TH, et al. MT1-matrix metalloproteinase directs arterial wall invasion and neointima formation by vascular smooth muscle cells. J Exp Med 2005; 202:663 - 71; http://dx.doi.org/10.1084/jem.20050607; PMID: 16147977
- Oh J, Takahashi R, Adachi E, Kondo S, Kuratomi S, Noma A, et al. Mutations in two matrix metalloproteinase genes, MMP-2 and MT1-MMP, are synthetic lethal in mice. Oncogene 2004; 23:5041 - 8; http://dx.doi.org/10.1038/sj.onc.1207688; PMID: 15064723
- Rodríguez D, Morrison CJ, Overall CM. Matrix metalloproteinases: what do they not do? New substrates and biological roles identified by murine models and proteomics. Biochim Biophys Acta 2010; 1803:39 - 54; http://dx.doi.org/10.1016/j.bbamcr.2009.09.015; PMID: 19800373
- Page-McCaw A, Ewald AJ, Werb Z. Matrix metalloproteinases and the regulation of tissue remodelling. Nat Rev Mol Cell Biol 2007; 8:221 - 33; http://dx.doi.org/10.1038/nrm2125; PMID: 17318226
- Parks WC, Wilson CL, López-Boado YS. Matrix metalloproteinases as modulators of inflammation and innate immunity. Nat Rev Immunol 2004; 4:617 - 29; http://dx.doi.org/10.1038/nri1418; PMID: 15286728
- Van Hul M, Lijnen HR. A functional role of gelatinase A in the development of nutritionally induced obesity in mice. J Thromb Haemost 2008; 6:1198 - 206; http://dx.doi.org/10.1111/j.1538-7836.2008.02988.x; PMID: 18433461
- Niyibizi C, Fietzek PP, van der Rest M. Human placenta type V collagens. Evidence for the existence of an alpha 1(V) alpha 2(V) alpha 3(V) collagen molecule. J Biol Chem 1984; 259:14170 - 4; PMID: 6501291
- Linsenmayer TF, Gibney E, Igoe F, Gordon MK, Fitch JM, Fessler LI, et al. Type V collagen: molecular structure and fibrillar organization of the chicken alpha 1(V) NH2-terminal domain, a putative regulator of corneal fibrillogenesis. J Cell Biol 1993; 121:1181 - 9; http://dx.doi.org/10.1083/jcb.121.5.1181; PMID: 8501123
- Burrows NP, Nicholls AC, Yates JR, Gatward G, Sarathachandra P, Richards A, et al. The gene encoding collagen alpha1(V)(COL5A1) is linked to mixed Ehlers-Danlos syndrome type I/II. J Invest Dermatol 1996; 106:1273 - 6; http://dx.doi.org/10.1111/1523-1747.ep12348978; PMID: 8752669
- Huang G, Ge G, Wang D, Gopalakrishnan B, Butz DH, Colman RJ, et al. α3(V) collagen is critical for glucose homeostasis in mice due to effects in pancreatic islets and peripheral tissues. J Clin Invest 2011; 121:769 - 83; http://dx.doi.org/10.1172/JCI45096; PMID: 21293061
- Mumby SM, Raugi GJ, Bornstein P. Interactions of thrombospondin with extracellular matrix proteins: selective binding to type V collagen. J Cell Biol 1984; 98:646 - 52; http://dx.doi.org/10.1083/jcb.98.2.646; PMID: 6693501
- Bonaldo P, Russo V, Bucciotti F, Doliana R, Colombatti A. Structural and functional features of the alpha 3 chain indicate a bridging role for chicken collagen VI in connective tissues. Biochemistry 1990; 29:1245 - 54; http://dx.doi.org/10.1021/bi00457a021; PMID: 2322559
- Kobayasi T, Karlsmark T. Type V and VI collagen for cohesion of dermal fibrillar structures. J Submicrosc Cytol Pathol 2006; 38:103 - 8; PMID: 17784637
- Keene DR, Engvall E, Glanville RW. Ultrastructure of type VI collagen in human skin and cartilage suggests an anchoring function for this filamentous network. J Cell Biol 1988; 107:1995 - 2006; http://dx.doi.org/10.1083/jcb.107.5.1995; PMID: 3182942
- Bonaldo P, Braghetta P, Zanetti M, Piccolo S, Volpin D, Bressan GM. Collagen VI deficiency induces early onset myopathy in the mouse: an animal model for Bethlem myopathy. Hum Mol Genet 1998; 7:2135 - 40; http://dx.doi.org/10.1093/hmg/7.13.2135; PMID: 9817932
- Jöbsis GJ, Keizers H, Vreijling JP, de Visser M, Speer MC, Wolterman RA, et al. Type VI collagen mutations in Bethlem myopathy, an autosomal dominant myopathy with contractures. Nat Genet 1996; 14:113 - 5; http://dx.doi.org/10.1038/ng0996-113; PMID: 8782832
- Speer MC, Tandan R, Rao PN, Fries T, Stajich JM, Bolhuis PA, et al. Evidence for locus heterogeneity in the Bethlem myopathy and linkage to 2q37. Hum Mol Genet 1996; 5:1043 - 6; http://dx.doi.org/10.1093/hmg/5.7.1043; PMID: 8817344
- Khan T, Muise ES, Iyengar P, Wang ZV, Chandalia M, Abate N, et al. Metabolic dysregulation and adipose tissue fibrosis: role of collagen VI. Mol Cell Biol 2009; 29:1575 - 91; http://dx.doi.org/10.1128/MCB.01300-08; PMID: 19114551
- Pasarica M, Gowronska-Kozak B, Burk D, Remedios I, Hymel D, Gimble J, et al. Adipose tissue collagen VI in obesity. J Clin Endocrinol Metab 2009; 94:5155 - 62; http://dx.doi.org/10.1210/jc.2009-0947; PMID: 19837927
- Spiegelman BM, Ginty CA. Fibronectin modulation of cell shape and lipogenic gene expression in 3T3-adipocytes. Cell 1983; 35:657 - 66; http://dx.doi.org/10.1016/0092-8674(83)90098-3; PMID: 6686086
- Singh P, Carraher C, Schwarzbauer JE. Assembly of fibronectin extracellular matrix. Annu Rev Cell Dev Biol 2010; 26:397 - 419; http://dx.doi.org/10.1146/annurev-cellbio-100109-104020; PMID: 20690820
- Georges-Labouesse EN, George EL, Rayburn H, Hynes RO. Mesodermal development in mouse embryos mutant for fibronectin. Dev Dyn 1996; 207:145 - 56; http://dx.doi.org/10.1002/(SICI)1097-0177(199610)207:2<145::AID-AJA3>3.0.CO;2-H; PMID: 8906418
- George EL, Baldwin HS, Hynes RO. Fibronectins are essential for heart and blood vessel morphogenesis but are dispensable for initial specification of precursor cells. Blood 1997; 90:3073 - 81; PMID: 9376588
- Wang Y, Zhao L, Smas C, Sul HS. Pref-1 interacts with fibronectin to inhibit adipocyte differentiation. Mol Cell Biol 2010; 30:3480 - 92; http://dx.doi.org/10.1128/MCB.00057-10; PMID: 20457810
- Smas CM, Sul HS. Pref-1, a protein containing EGF-like repeats, inhibits adipocyte differentiation. Cell 1993; 73:725 - 34; http://dx.doi.org/10.1016/0092-8674(93)90252-L; PMID: 8500166
- Wang Y, Zhao L, Smas C, Sul HS. Pref-1 interacts with fibronectin to inhibit adipocyte differentiation. Mol Cell Biol 2010; 30:3480 - 92; http://dx.doi.org/10.1128/MCB.00057-10; PMID: 20457810
- Hynes RO. Integrins: bidirectional, allosteric signaling machines. Cell 2002; 110:673 - 87; http://dx.doi.org/10.1016/S0092-8674(02)00971-6; PMID: 12297042
- Sottile J, Hocking DC. Fibronectin polymerization regulates the composition and stability of extracellular matrix fibrils and cell-matrix adhesions. Mol Biol Cell 2002; 13:3546 - 59; http://dx.doi.org/10.1091/mbc.E02-01-0048; PMID: 12388756
- Mosher DF, Schad PE. Cross-linking of fibronectin to collagen by blood coagulation Factor XIIIa. J Clin Invest 1979; 64:781 - 7; http://dx.doi.org/10.1172/JCI109524; PMID: 38260
- Boström P, Wu J, Jedrychowski MP, Korde A, Ye L, Lo JC, et al. A PGC1-α-dependent myokine that drives brown-fat-like development of white fat and thermogenesis. Nature 2012; 481:463 - 8; http://dx.doi.org/10.1038/nature10777; PMID: 22237023
- Bork P, Doolittle RF. Proposed acquisition of an animal protein domain by bacteria. Proc Natl Acad Sci U S A 1992; 89:8990 - 4; http://dx.doi.org/10.1073/pnas.89.19.8990; PMID: 1409594
- Chi-Rosso G, Gotwals PJ, Yang J, Ling L, Jiang K, Chao B, et al. Fibronectin type III repeats mediate RGD-independent adhesion and signaling through activated β1 integrins. J Biol Chem 1997; 272:31447 - 52; http://dx.doi.org/10.1074/jbc.272.50.31447; PMID: 9395478
- Bornstein P, Sage EH. Matricellular proteins: extracellular modulators of cell function. Curr Opin Cell Biol 2002; 14:608 - 16; http://dx.doi.org/10.1016/S0955-0674(02)00361-7; PMID: 12231357
- Nie J, Bradshaw AD, Delany AM, Sage EH. Inactivation of SPARC enhances high-fat diet-induced obesity in mice. Connect Tissue Res 2011; 52:99 - 108; http://dx.doi.org/10.3109/03008207.2010.483747; PMID: 20615096
- Bradshaw AD, Graves DC, Motamed K, Sage EH. SPARC-null mice exhibit increased adiposity without significant differences in overall body weight. Proc Natl Acad Sci U S A 2003; 100:6045 - 50; http://dx.doi.org/10.1073/pnas.1030790100; PMID: 12721366
- Bradshaw AD, Puolakkainen P, Dasgupta J, Davidson JM, Wight TN, Helene Sage E. SPARC-null mice display abnormalities in the dermis characterized by decreased collagen fibril diameter and reduced tensile strength. J Invest Dermatol 2003; 120:949 - 55; http://dx.doi.org/10.1046/j.1523-1747.2003.12241.x; PMID: 12787119
- Iruela-Arispe ML, Vernon RB, Wu H, Jaenisch R, Sage EH. Type I collagen-deficient Mov-13 mice do not retain SPARC in the extracellular matrix: implications for fibroblast function. Dev Dyn 1996; 207:171 - 83; http://dx.doi.org/10.1002/(SICI)1097-0177(199610)207:2<171::AID-AJA5>3.0.CO;2-E; PMID: 8906420
- Nie J, Sage EH. SPARC functions as an inhibitor of adipogenesis. J Cell Commun Signal 2009; 3:247 - 54; http://dx.doi.org/10.1007/s12079-009-0064-4; PMID: 19798596
- Ross SE, Hemati N, Longo KA, Bennett CN, Lucas PC, Erickson RL, et al. Inhibition of adipogenesis by Wnt signaling. Science 2000; 289:950 - 3; http://dx.doi.org/10.1126/science.289.5481.950; PMID: 10937998
- Rosen ED, MacDougald OA. Adipocyte differentiation from the inside out. Nat Rev Mol Cell Biol 2006; 7:885 - 96; http://dx.doi.org/10.1038/nrm2066; PMID: 17139329
- Varma V, Yao-Borengasser A, Bodles AM, Rasouli N, Phanavanh B, Nolen GT, et al. Thrombospondin-1 is an adipokine associated with obesity, adipose inflammation, and insulin resistance. Diabetes 2008; 57:432 - 9; http://dx.doi.org/10.2337/db07-0840; PMID: 18057090
- Li Y, Tong X, Rumala C, Clemons K, Wang S. Thrombospondin1 deficiency reduces obesity-associated inflammation and improves insulin sensitivity in a diet-induced obese mouse model. PLoS One 2011; 6:e26656; http://dx.doi.org/10.1371/journal.pone.0026656; PMID: 22039525
- Olerud J, Mokhtari D, Johansson M, Christoffersson G, Lawler J, Welsh N, et al. Thrombospondin-1: an islet endothelial cell signal of importance for β-cell function. Diabetes 2011; 60:1946 - 54; http://dx.doi.org/10.2337/db10-0277; PMID: 21617177
- Voros G, Lijnen HR. Deficiency of thrombospondin-1 in mice does not affect adipose tissue development. J Thromb Haemost 2006; 4:277 - 8; http://dx.doi.org/10.1111/j.1538-7836.2005.01696.x; PMID: 16409488
- Kyriakides TR, Zhu Y-H, Smith LT, Bain SD, Yang Z, Lin MT, et al. Mice that lack thrombospondin 2 display connective tissue abnormalities that are associated with disordered collagen fibrillogenesis, an increased vascular density, and a bleeding diathesis. J Cell Biol 1998; 140:419 - 30; http://dx.doi.org/10.1083/jcb.140.2.419; PMID: 9442117
- Shitaye HS, Terkhorn SP, Combs JA, Hankenson KD. Thrombospondin-2 is an endogenous adipocyte inhibitor. Matrix Biol 2010; 29:549 - 56; http://dx.doi.org/10.1016/j.matbio.2010.05.006; PMID: 20561899
- Van Hul M, Frederix L, Lijnen HR. Role of Thrombospondin-2 in Murine Adipose Tissue Angiogenesis and Development. Obesity (Silver Spring) 2011; In press http://dx.doi.org/10.1038/oby.2011.260; PMID: 21818150
- McBeath R, Pirone DM, Nelson CM, Bhadriraju K, Chen CS. Cell shape, cytoskeletal tension, and RhoA regulate stem cell lineage commitment. Dev Cell 2004; 6:483 - 95; http://dx.doi.org/10.1016/S1534-5807(04)00075-9; PMID: 15068789
- Guilak F, Cohen DM, Estes BT, Gimble JM, Liedtke W, Chen CS. Control of stem cell fate by physical interactions with the extracellular matrix. Cell Stem Cell 2009; 5:17 - 26; http://dx.doi.org/10.1016/j.stem.2009.06.016; PMID: 19570510
- Sato-Kusubata K, Jiang Y, Ueno Y, Chun T-H. Adipogenic histone mark regulation by matrix metalloproteinase 14 in collagen-rich microenvironments. Mol Endocrinol 2011; 25:745 - 53; http://dx.doi.org/10.1210/me.2010-0429; PMID: 21436261