Abstract
The role of autophagy in necrotrophic fungal physiology and infection biology is poorly understood. We have studied autophagy in the necrotrophic plant pathogen Fusarium graminearum in relation to development of nonassimilating structures and infection. We identified an ATG8 homolog F. graminearum ATG8 whose first 116 amino acids before the predicted ATG4 cleavage site are 100% identical to Podospora anserina ATG8. We generated a ΔFgatg8 mutant by gene replacement and showed that this cannot form autophagic compartments. The strain forms no perithecia, has reduced conidia production and the aerial mycelium collapses after a few days in culture. The collapsing aerial mycelium contains lipid droplets indicative of nitrogen starvation and/or an inability to use storage lipids. The capacity to use carbon/energy stored in lipid droplets after a shift from carbon rich conditions to carbon starvation is severely inhibited in the ΔFgatg8 strain demonstrating autophagy-dependent lipid utilization, lipophagy, in fungi. Radial growth rate of the ΔFgatg8 strain is reduced compared with the wild type and the mutant does not grow over inert plastic surfaces in contrast to the wild type. The ability to infect barley and wheat is normal but the mutant is unable to spread from spikelet to spikelet in wheat. Complementation by inserting the F. graminearum atg8 gene into a region adjacent to the actin gene in ΔFgatg8 fully restores the WT phenotype. The results showed that autophagy plays a pivotal role for supplying nutrients to nonassimilating structures necessary for growth and is important for plant colonization. This also indicates that autophagy is a central mechanism for fungal adaptation to nonoptimal C/N ratios.
Introduction
Vegetative fungal hyphae can expand over large areas to assimilate nutrients from their growth environment whether it is soil or living creatures. The hyphae remain interconnected and nutrients may be transported over long distances to accommodate development of nonassimilating structures e.g., aerial mycelia, conidiophores, sexual reproductive organs or infection structures. These structures thus rely on supply of nutrients from the vegetative mycelium. The nutrients can either be mobilized by breaking down biomass in the vegetative mycelium through apoptosis or autophagyCitation1 and the nutrients translocated into these developing nonassimilating fungal structures.
Autophagy is an intracellular process for degradation and recycling of proteins, organelles and cytoplasm in eukaryotic organisms and is now known to be important for fungal survival, reproduction and infection.Citation1 It acts in the interface between death and survival by rescuing cells from starvation by remobilization of intracellular components.Citation2 It is typically activated by nitrogen starvation or adverse external conditions that may occur during developmental processes.Citation1 In contrast to apoptosis, autophagy does not normally lead to DNA breakdownCitation3 and is likely the most important process for recycling of biomass in fungi to support their survival and development.Citation1,Citation4
Bakers' yeast, Saccharomyces cerevisiae, has been the model organism for the study of autophagy. Autophagy is initiated by the formation of a single-membrane phagophore that envelopes cytoplasm and organelles. The phagophore elongates and the edges are sealed to form a spherical double membrane structure, the autophagosome. The autophagosome fuses with the vacuole, releasing an autophagic body and its content into the lumen of the vacuole for processing by vacuolar hydrolases.Citation5 Currently, more than 30 autophagy-related proteins (ATGs) that play a role in autophagy have been described.Citation6 They facilitate vesicle nucleation, phagophore formation, autophagosome expansion, docking and recycling. The ubiquitin like-ATG8 has been shown to be essential for autophagosome formationCitation7 and is often used as a biological marker for autophagy as it is associated with all stages of the process.
In addition to facilitate nutrient transfer in filamentous fungi, autophagy is also involved in stress-induced cell differentiation of reproductive structures. In the saprotrophic fungus Podospora anserina autophagy has been shown to be required for formation of female reproductive organsCitation8 and in Sordaria macrospora the morphology of the perithecia is altered in autophagy impaired mutants.Citation9 In the hemi-biotrophic plant pathogenic fungi Magnaporthe oryzae and Colletotrichum orbiculare autophagy has been shown to be significant for appressorium-mediated plant infection.Citation10,Citation11 Also in Ustilago maydis autophagy is important for fungal pathogenicity.Citation12 In the necrotrophic plant pathogenic fungus Fusarium graminearum the importance of autophagy for fungal growth and infection has not been studied in detail.
Fusarium graminearum is an ascomycete necrotrophic pathogen of cereal species and causes Fusarium head blight (FHB). The fungus produces mycotoxins such as deoxynivalenol (DON) and zearalenone (ZEA) which pose severe hazards to human and animal health and thus reduce grain quality. In barley F. graminearum attack the spike as soon as it erupts from the flag leaf sheath, near the time of pollination. The fungus infects individual spikelets through the brush hairs protruding from the seed tips, proceeds to the apical portions of the pericarp and the inner surface of the testa. In wheat, the fungus form infection cushionsCitation13 and spread by systemic growth through the rachis from one spikelet to another when inoculated by point inoculation of one spikelet.Citation14,Citation15 Autophagy could be important for both successful infection and spread between spikelets in wheat since the initial infection and the growth through the nutrient-poor rachis could need mobilization of recycled nutrients. The autophagy-associated lipase ATG15 from F. graminearum has been shown to be required for infection which support this hypothesis.Citation16 This secondary spread from one spikelet to another does not occur in infected barley.Citation17
Here we report on autophagy in F. graminearum. We identify and compare a putative F. graminearum atg8 with known atg8-genes of fungal origin and delete it by targeted gene replacement. The effect of deleting the Fgatg8 is studied in relation to linear growth rate, formation of aerial mycelium, use of storage lipid droplets, growth over an inert plastic surface, infection and formation of reproductive structures. The importance of autophagy for the ability of F. graminearum to infect wheat and barley is evaluated.
Results
The F. graminearum atg8 gene is highly similar to other fungal atg8 genes
The F. graminearum atg8 gene was identified in the genome database (www.broadinstitute.org) with the BLAST algorithm using the yeast atg8 ortholog amino acid sequence (GenBank accession no. CAA84899). The F. graminearum atg8 homolog, atg8 (Locus FGSG_10740.3), contains three exons, two introns and the predicted F. graminearum ATG8 protein is 118 amino acids (aa) long with a calculated molecular mass of 13.72 kDa. In S. cerevisiae the ATG8-preprotein is recognized and cleaved by ATG4 after a glycine close to the C-terminal to yield the active ATG8. In S. cerevisiae the cleavage is between amino acid 116 and 117. Comparison of the active part of the protein, preceding the putative ATG4 cleavage site, with known ATG8 from other fungi shows high homologies. Thus, F. graminearum ATG8 is 81% identical to ATG8 in S. cerevisiae, 97% identical to ATG8 in Magnaporthe grisea (accession no. ACJ06522588) and 100% identical to ATG8 in Podospora anserina (accession no. AF502254). Interestingly, in all four sequences exactly 116 aa preceded the ATG4 cleavage site.
The atg8 gene was successfully replaced in F. graminearum
The F. graminearum atg8 gene was replaced with a hygromycin resistance cassette using homologous recombination and A. tumefaciens transformation (). More than 100 hygromycin-resistant mutants were obtained and 20 were selected for PCR analyses. Sixteen of these had the correct replacement according to PCR analyses of the insertion border regions. DNA gel blot analysis of XhoI-digested genomic DNA from four strains revealed that these had the expected band shift from 5.4 kb to 7.5 kb showing that the larger hygromycin cassette had replaced atg8 and that only one insertion had occurred (). These four strains were used for initial characterization of atg8 mutants and subsequently one, named ΔFgatg8, was used for infection studies and gene complementation. The F. graminearum atg8 gene including the promoter was inserted into the region of the actin gene in the ΔFgatg8 strain and the complementation strain cΔFgatg8 was obtained (). Results of the initial phenotypic characterization of all four deletion strains and complementation constructs are available in .
Figure 1. (A) Disruption strategy for F. graminearum atg8 gene. Homologous recombination between the disruption cassette and the Fgatg8 gene inserted the hygromycin resistance gene (HYG) at the indicated sites. (B) DNA gel blot analysis of XhoI- digested genomic DNA using part of the atg8 gene and the hygromycin cassette as probe.

Figure 2. (A) Validation results of F. graminearum atg8 gene insertion in the ΔFgatg8 strain. Chromosome 4, 3157631–3159167 bp with sequence of geneticin resistance NPTII cassette and F. graminearum atg8 gene inserted by homologous recombination. (B) PCR analysis with primer pairs (1) upsActHFwd/nptIIRev, (2) nptIIFwd/Atg8upsFW and (3) Atg8dwnRev/dwnActHrRev. (C) DNA gel blot analysis with (4) SspI and HindII digested genomic DNA and primer pairs (5) nptIIFwd/nptIIRev was used as probe.
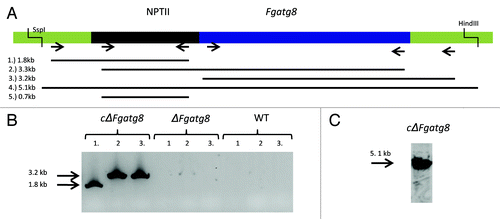
Deletion of atg8 prevents autophagy in F. graminearum
Autophagy in the ΔFgatg8 strain and the WT strain was studied by staining the rapamycin-treated or nutrient-starved hyphae with MDC as described in Veneault-Fourrey et al.Citation11 The WT strain showed induction of autophagic compartments under both conditions (). In the ΔFgatg8 strain, rapamycin treatment led to corrugated hyphae while the starvation treatment resulted in highly vacuolated hyphae. Treatment with MDC showed no signs of autophagy in the ΔFgatg8 strain (). These results suggest that autophagy is blocked in the ΔFgatg8 strain.
Figure 3. Formation of autophagic compartments in hyphae after induction by starvation or rapamycin treatment. F. graminearum WT (left panel) and ΔFgatg8 (right panel) mycelium were grown in YPD, YPD + rapamycin or in H2O and stained with monodansyl cadavarine (MDC). DIC and fluorescence images of the MDC staining are shown. Fluorescence images are taken using the same microscope and camera settings. Bars = 5 µm.
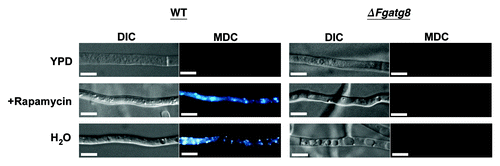
The ΔFgAgt8 strain has reduced ability to reallocate nutrients and support nonassimilating fungal structures
Colonies of WT and ΔFgatg8 strains growing on DFM media grew with the same density and color for the first days of incubation. When the mycelia covered almost the entire plate, after approximately 6 d, the aerial mycelium in the ΔFgatg8 strain collapsed leaving a totally flat mycelium (). The mutant show denser pigmentation probably due to the altered growth form with loss of the white aerial mycelium. The wild type showed normal aerial mycelium for at least 3–4 weeks of incubation (Fig. S2).
Figure 4. Aerial mycelium growth. Petri dishes with ΔFgatg8, WT and cΔFgatg8 strains cultivated on DFM media for 6 d at 22°C. Petri dishes = 9 cm diameter.
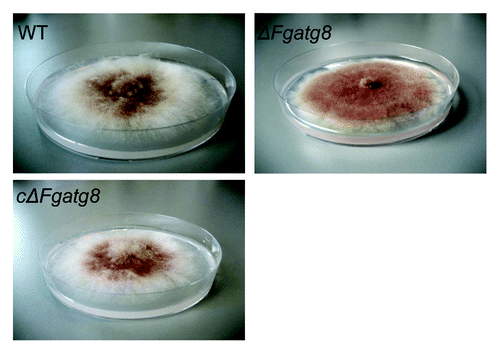
Linear growth rates of the WT strain and cΔFgatg8 strain were higher than ΔFgatg8 strain on all media tested (). On a rich medium (YPD, C/N ratio = 4.5Citation18) the difference in growth rates between the WT and the ΔFgatg8 strain was less than on a defined medium (DFM, C/N ratio = 22.8). To test if this difference was mainly caused by an inability to reallocate nitrogen from the old hyphae to the growing tip, growth on two defined media with high, DFMNO3 (C/N ratio = 21), and low nitrogen content, DFMNO3L (C/N ratio = 104), was analyzed. The growth rates of ΔFgatg8 strain on DFMNO3 and DFMNO3L were reduced by 64.1 ± 1.5% and 58.1 ± 0.5% (± SEM) compared with the WT. Thus, growth rate of the ΔFgatg8 strain was reduced most compared with the WT under the most nitrogen-limited growth conditions.
Table 1. Linear growth rate in mm/d at 19°C measured in growth pipettes with different media
The density of the aerial hyphae in the ΔFgatg8 strain was reduced compared with the WT strain when cultivated on DFM (; Figs. S2andS3). The ΔFgatg8 aerial mycelium was very low compared with the high and dense aerial mycelium produced by the WT and cΔFgatg8 strains (, left; Fig. S4). The aerial mycelium was investigated by microscopy at day 4 before the colonies covered the whole plates. The ΔFgatg8 strain aerial mycelium showed contorted hyphae that contained a high number of lipid droplets while no detectable lipid droplets were found in the WT and cΔFgatg8 aerial mycelium (; Fig. S4).
Figure 5. Scanned images of aerial mycelium on 18 x 18 mm cover glasses with an arrow to the left indicating the level of the agar surface (left image). The DIC microscope images (middle image) and corresponding fluorescence microscopy images (right image). Microscope images show a contorted thin mycelium containing lipid droplets for the ΔFgatg8-mutant while this is absent in WT and cΔFgatg8 strains. HCS LipidTOX Red staining and fluorescence microscopy confirms the presence of lipid droplets in the ΔFgatg8-mutant and their absence in the other strains. Bars = 20 µm.
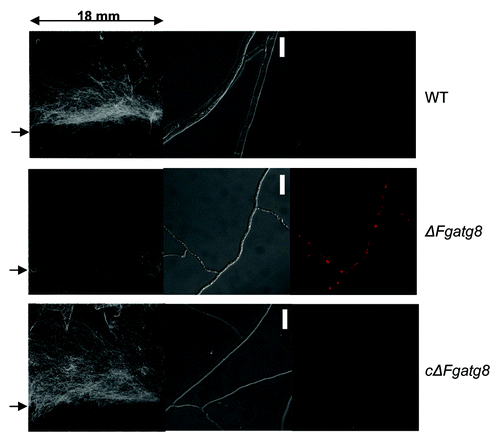
The use of storage lipid droplets in carbon-starved mycelium was investigated in a modified liquid DFM medium with only NO3 as nitrogen source. Two days of growth in medium containing double normal concentration of glucose (C/N ratio = 41.7) caused accumulation of lipid droplets in all strains. Replacing the liquid medium with a DFM medium containing 4 times the usual nitrogen concentration and no carbon source caused disappearance of the lipid droplets in WT and cΔFgatg8 strain after 48 h continued incubation. The ΔFgatg8 strain retained an abundance of lipid droplets under these conditions ().
Figure 6. Lipid droplets in mycelia of F. graminearum strains after 48 h incubation in 1/10 DFM with 2x glucose and NO3 as nitrogen source and a subsequent change in nutrient solution and a further 48 h incubation in four times NO3 with no glucose. Inserts shows parts of images of similar cultures after the first 48 h and before the shift to high N and no C medium to show that all treatments starts with the same amounts of lipid droplets after the initial incubation. The lipid droplets was stained with the fluorescent stain Nile Red and filters and image processing optimized for showing lipid droplets. Bars = 50 µm.
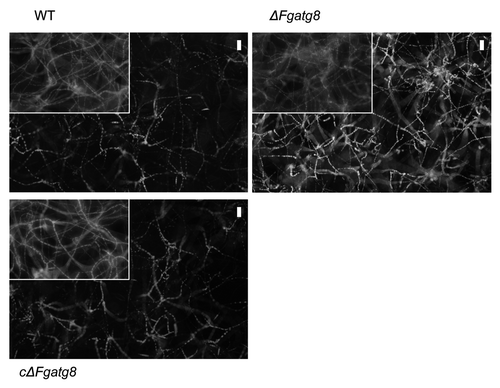
Growth over inert surface is virtually absent in the ΔFgatg8 strain. When an agar plug with mycelium cut from the growing edge of cultures was placed on a DFM plug in a plastic Petri dish the WT and cΔFgatg8 strains showed extensive mycelium growth over the plastic surface after 3 d growth whereas the ΔFgatg8 strain had hardly grown from the agar plug (; and).
Formation of asexual and sexual spores is reduced or absent in the ΔFgatg8 strain
The ΔFgatg8 strain can produce viable conidia. However, the number of macroconidia produced after 19 d when the plate was completely covered was considerably lower in ΔFgatg8 than in the WT and cΔFgatg8 strains ().
Figure 8. Conidia production of the different strains per DFM plate incubated 19 d at room temperature (22°C).
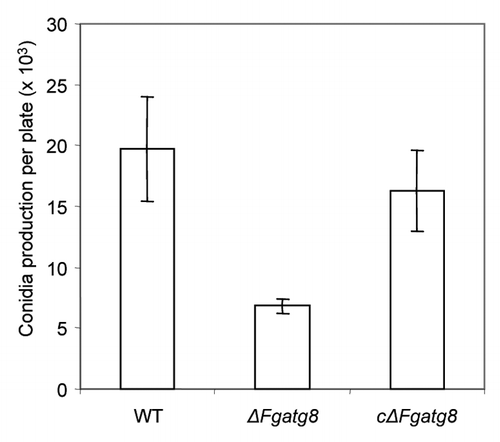
F. graminearum is homothallic and readily forms perithecia by self-fertilization. The ΔFgatg8 strain does not form perithecia under conditions where the WT and cΔFgatg8 produces high numbers. In attempted crosses between ΔFgatg8 and WT perithecia were not found, while in crosses between cΔFgatg8 and WT both perithecia and viable ascospore offsprings were found suggesting that the mutant lacking atg8 is unable to engage in sexual reproduction.
The ΔFgatg8 strain shows delayed infection of barley and inability to spread from spikelet to spikelet in wheat
The pathogenicity of the ΔFgatg8 strain on barley was examined by spray inoculation of barley spikes at flowering. The WT and the ΔFgatg8 strain developed similar symptoms of FHB (). After 4 d FHB symptoms were detected in plants infected with both the mutant and the WT strain and after 17 d almost all grains were heavily infected (). The WT strain reached the maximum infection level at 14–17 d after infection (dai), with an average infection of 14.2 (± 1.1) grains per spike, whereas the maximum infection level in the ΔFgatg8 strain was slightly delayed and was reached at 17 dai, with 11.6 (± 0.9) infected grains per spike.
Figure 9. Infection of barley spikes with F. graminearum, (A) WT and (B) ΔFgatg8 mutant 10 d after spray inoculation.
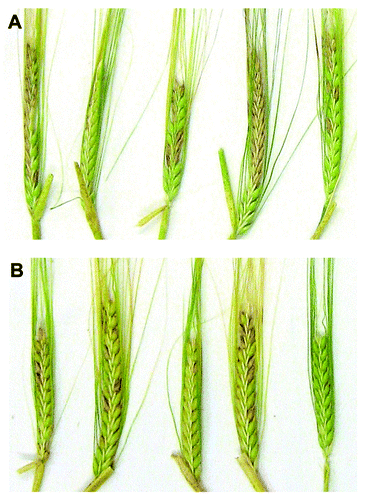
Figure 10. Comparison of F. graminearum, WT and ΔFgatg8 mutant infection of barley spikes over time.
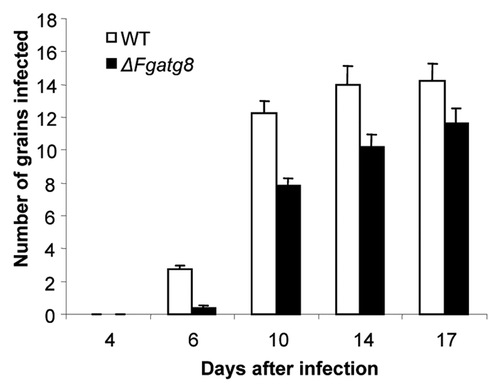
When wheat plants were point inoculated, the ΔFgatg8 and the WT strain displayed a clear difference in the development of FHB. In plants infected by WT and cΔFgatg8 the infection spread from the point of inoculation to most of the spike (). In the plants infected with the ΔFgatg8 the infection was typically only present in the grains that were point inoculated. Thus the ΔFgatg8 strain has reduced ability to spread from the point of inoculation in wheat heads ().
Figure 11. Wheat spikes 21 d after point inoculation. Arrows indicate infection localized to the point of inoculation for the ΔFgatg8 mutant.
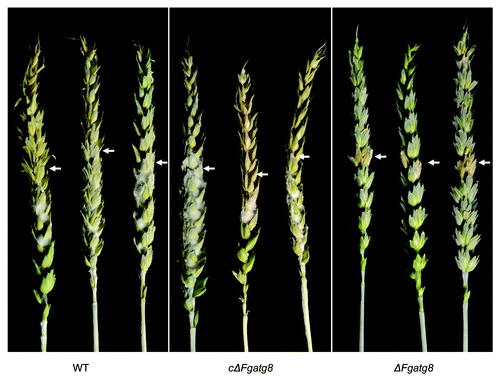
Deoxynivalenol (DON) has been reported to be important for spread of infection in wheat spikes.Citation19 To determine if the reduced infection by ΔFgatg8 could be ascribed to lack of toxin production DON, zearalenone (ZEA) and aurofusarin (AUR) levels were determined. Concentrations of DON in WT were 0.44, 0.16 and 0.19 μg/mL on YMA, PDA and CzID media respectively. ΔFgatg8 DON production was 6%, 88% and 7% of WT concentration. The production of DON in cΔFgatg was 89%, 176%, 93% compared with the WT in the three respective media. ZEA concentrations in WT were 8,5, 38 and 0.7 μg/mL. Production of ZEA in ΔFgatg8 was reduced to 2%, 19% and 18% of WT ZEA production. ZEA for cΔFgatg8 was 265%, 100% and 127% compared with WT. Production of AUR was similar in all the strains.
Discussion
The F. graminearum atg8 gene shows high similarity to atg8 genes from other organisms as previously shown.Citation6 The predicted amino acid sequence is 100% identical to ATG8 in P. anserina for the first 116 amino acids preceding the predicted C-terminal ATG4 cleavage site.Citation20 The F. graminearum atg8 gene was replaced by a hygromycin cassette and we could show that the ΔFgatg8 strain does not form autophagic compartments as seen by MDC staining () after starvation or treatment with rapamycin that induces these structures in the WT. This stain is not exclusive to autophagosomesCitation21 but usually reveals a clear difference between wild-type and atg8 deletions mutants.Citation1 A complementation strain, cΔFgatg8 was constructed by insertion of F. graminearum atg8 including its own promoter in a position with no open reading frames but in vicinity of the actin gene to ensure that the chromatin structure is open for transcription ().
The ΔFgatg8 strain produced less conidia than the WT and cΔFgatg8 () and no perithecia was formed under normally inducing conditions. Reduced conidia formation has previously been observed in autophagy-deficient mutantsCitation22 of P. anserina,Citation8,Citation23 A. oryzaeCitation24 and A. fumigatus.Citation25 Also the inability to form sexual spores has been reported.Citation8,Citation22 Vacuolization of vegetative mycelium is a way to support conidiation through recycling of nutrients.Citation4,Citation26 Since autophagy channels cytoplasm through the vacuole to provide recycled nutrients, a deficiency in this process probably hinders conidiophore development through lack of energy and nutrients.
This study is the first to show that loss of autophagy results in apparently normal development of aerial hyphae that then collapse after 6 d growth in defined media. The hyphae are at this stage filled with lipid droplets (and). This suggests that a surplus of carbon accumulates in the mutant or that it is unable to process carbon stored in lipid droplets. Glycerol is an essential component of the mechanism by which fungal hyphae regulate and maintain turgor.Citation22,Citation27,Citation28 If the ΔFgatg8 strain is unable to form free glycerol required to maintain turgor pressure the aerial mycelium will most likely collapse. Thus it appears as if lack of autophagy in F. graminearum leads to an inability to utilize the stored lipids. Linear growth rate was lower for ΔFgatg8 strains than for the WT and cΔFgatg8 strains and the difference was more pronounced on the minimal media than on the rich medium (). The growing tip is dependent on nutrients from older hyphal compartments as well as from nutrient uptake.Citation4,Citation29 Thus the larger difference in growth rate between ΔFgatg8 strains and the WT strain on the high C/N ratio media could indicate that autophagic recycling is needed to obtain nutrients from the old hyphal compartments to be transported to actively growing hyphal tips. Nitrogen appears to be the most critical requirement for fungi growing on DFM as lipid droplets are formed in the wild type under this nutrient regime.
An important feature for fungal growth is the ability to breach inert areas in search for nutrients. F. graminearum is very able in this respect. The WT and cΔFgatg8 strains can cover the bottom of a plastic Petri dish from a 1 cm diameter inoculated agar plug with DFM in a few days () while the ΔFgatg8 strain is unable to do so. The results can best be explained by an inability of the mutant to keep the turgor pressure required for development of exploratory hyphae due to loss of cytoplasmic glycerol generated through lipid breakdown.
As stated above the accumulation of lipid droplets in the aerial mycelium of ΔFgatg8 strains could be caused either by a relative surplus of carbon and a lack of some other nutrients or an inability to process lipids. Since autophagy has been shown to be involved in lipid droplet breakdownCitation30 the ΔFgatg8 strain was compared with the WT and cΔFgatg8 strains. The results show that the ΔFgatg8 strain has lost its ability to use stored lipid droplets under severe carbon starvation as the WT and cΔFgatg8 strains used most of the lipid droplets ().
This implies that autophagy-dependent lipid breakdown is required to generate glycerol needed to keep turgor pressure in aerial mycelia and mycelia growing over hydrophobic surfaces. Autophagy has recently been shown to be involved in lipid droplet breakdown, lipophagy, in mammalian cells.Citation30 Our results thus further emphasize that autophagy is an essential key for lipid removal from cells and that fungi may be good model organisms to study this important process that appears to be central to the human metabolic syndrome and its associated pathologies.Citation30 Nutrient limitations influence mycotoxin synthesis and their production is dependent on use of stored carbon resources. The observed reduction in mycotoxin production in ΔFgatg8 is also of interest since this could be due to a failure to efficiently recycle carbon/energy from stores under conditions that normally would trigger secondary metabolite production.
The ΔFgatg8 strain could infect both barley and wheat. The infection of barley was slightly delayed but otherwise similar to the WT strain ( and ). F. graminearum has recently been shown to produce infection cushionsCitation13 and the delay in barley infection could be due to reduced turgor pressure in ΔFgatg8. The appressoria forming fungus M. grisea requires high turgor pressures to penetrate the host surface.Citation22 In wheat the ΔFgatg8 strain had lost the ability to grow from one spikelet to the next through the rachis (). The rachis is mainly composed of vascular tissues and poor in nutrients.Citation31 Thus the inability of infecting hyphae to pass the rachis could be due to lack of nutrient supply. The rachis does present a defense barrier in wheat as illustrated by the requirement of the mycotoxin deoxynivalenol (DON) to suppress deposition of callose and it is likely that the process is energy requiring. The autophagy-related lipase FgATG15 has previously been shown to be required for infection by F. graminearum in agreement with these results.Citation16 The low production of DON in ΔFgatg8 probably also contributes to the reduced pathogenicity in wheat.Citation32,Citation33
In conclusion this is the first report on autophagy in a necrotrophic plant pathogenic fungus and we show that autophagy is important for successful infection of wheat. As observed for other fungi autophagy is required for sporulation and sexual reproduction but here it is also shown to be essential for the use of storage lipids. Aerial hyphal collapse and the inability to break down lipid droplets suggest that maintenance of turgor pressure requires autophagy-dependent recycling of nutrients.
Materials and Methods
Fungal strains and standard growth conditions
F. graminearum wild-type strains PH1 (NRRL31084) and 00–676 (NRRL 34097)Citation34 were obtained from the Agricultural Research Service Culture Collection, National Center for Agricultural Utilization Research, Peoria, IL, USA. F. graminearum 1104–14Citation35 was kindly provided by Erik Lysøe, Plant Health and Plant Protection, Bioforsk, Noway. Wild-type and the ΔFgatg8 strains were stored in 10% glycerol (VWR, Herlev, Denmark, 24388.364) at -70°C. Strains were grown on YPD agar media (1% yeast extract, Merck 1.03753), 2% peptone, Merck, 1.07213, 1% dextrose, Merck, 108337, 2% agar, Merck, 1.01614) or on minimal DFM mediumCitation36 if not otherwise stated. For DNA extraction, strains were grown in liquid YPD at room temperature, (RT~22°C), harvested and stored at -70°C until use.
Construction of the Fgatg8 disruption mutant
Fusarium graminearum atg8 gene was identified in the F. graminearum genome database (www.broadinstitute.org) using the BLAST algorithm and the yeast ATG8 amino acid sequence (GenBank accession no. CAA84899). The USER cloning system described in Frandsen et al.Citation37 was used to generate Fgatg8 deletion strains. The upstream region of the F. graminearum atg8 gene was amplified by PCR using the primers Atg8O1 and Atg8O2 and the downstream region using the primers Atg8A3 and Atg8A4 (See for all primers used in this study). The amplified fragments were introduced into the pRF-HU2 vectorCitation37 flanking the hygromycin resistance cassette. The cloning results were confirmed by sequencing. The replacement construct was electroporated into Agrobacterium tumefaciens LBA4404 and A. tumefaciens-mediated transformation was performed as described in Malz et al.Citation36 DNA was prepared from approximately 1 g of fungal biomass of the WT and the Fgatg8 deletion strains as described in Tobiasen et al.Citation38 Selected mutants were analyzed for correct homologous recombination by PCR using the primers Atg8T3/ Hyg1 and Hyg2/Atg8T4. The replacement of the F. graminearum atg8 gene by the hygromycin gene was confirmed by DNA gel blotting of XhoI (Biolabs, R0146) digested genomic DNA using a 2.9 kb gene specific probe for atg8 and the hygromycin cassette which was generated from pΔFgatg8 using the primers Atg8O1 and Atg8A4.
Table 2. Primers used for cloning and result validation. Underline in the primers indicates specific overhangs containing a single 2-deoxyuridine nucleoside or added restriction sites.
The complementation of ΔFgatg8 strain with F. graminearum atg8 gene
The transformation vector for the complementation of ΔFgatg8 strain with F. graminearum atg8 gene was generated using the USER cloning system.Citation37 The atg8 gene with its own promoter and terminator was amplified using primers Atg8upsFW/Atg8dwnREV and inserted into vector U-GOAL, flanking the geneticin resistance cassette NPTII. The U-GOAL vector is a modified version of the HU2 vectorCitation37 with homolog sequences in the region of the actin gene. This region (Ph1, Chromosome 4, 3157631–3159167 bp) was selected for targeted integration into the ΔFgatg8 PH1 strain and it was amplified with primers actinO1/actinO2 and actinO3/actinO4. Agrobacterium mediated transformation of ΔFgatg8 and the PH1 wild-type strains was performed as described above. The insertion of atg8 was confirmed by DNA gel blotting of SspI (Biolabs, R0312) and HindII (Biolabs, R0104) digested genomic DNA using a 671bp specific NPTII probe (). Primers used for validation of correct insertion are upsActHFwd, dwnActHrew, nptIIRev, nptIIFwd, Atg8upsFW and Atg8dwnREV ().
Analysis of autophagy by MDC in F. graminearum
To study autophagy 4 × 104 WT or ΔFgatg8 strain conidia were added to 10 ml YPD medium and incubated for 22 h at RT. Rapamycin (CalBiochem, 553210), inducer of autophagy, was added to a final concentration of 100 nM or alternatively the mycelium was washed and transferred to H2O. In both cases the cultures were left for 4 h prior to staining with monodansyl cadavarine (MDC) (Sigma-Aldrich, D-4008) at a final concentration of 50µM for 30 min in the dark. After washing with water, samples were observed under DIC and epifluorescence microscopy using DAPI filter.
Linear growth rate on agar in growth pipettes
F. graminearum, WT and ΔFgatg8 strains were cultivated on YPD, DFM, DFMNO3 or DFMNO3L agar media in growth pipettes. DFMNO3 and DFMNO3L is DFM where 10 mM asparagine (Fluka, 11150) has been replaced by 20 mM or 4 mM NaNO3 (Merck, 1.06537) respectively. Growth pipettes was introduced as a simplified version of growth tubes.Citation39,Citation40 The technique is here further refined with an improved way for preparation and inoculation of the pipettes so that it can become a routine for linear growth rate measurements (for the preparation of growth pipettes see supplementary information, (Suppl. Protocol; Fig. S7). The growth pipettes were incubated at 19°C and the position of the hyphal front was marked every 3–4 d until the fastest growing strains had grown the whole pipette length. Linear growth rates were calculated by linear regression of colony edge positions from the first marking vs. incubation time.
Aerial mycelium development
Cover glasses were inserted in DFM agar medium and plugs of the WT or ΔFgatg8 strains were placed in the center of the Petri dish and incubated at 22°C. When aerial mycelium had formed on the cover glasses, these were removed and scanned or stained. The same scanning technique as for the growth over plastic surfaces was used. Water containing 1 µg/ml HCS LipidTOX Red (Invitrogen, H34476) was used to stain lipid droplets in the hyphae and visualized by fluorescence microscopy using a Rhodamine filter.
Usage of storage lipid droplets in carbon starving mycelium
One-hundred microliters of 105 macroconidia/ml of the ΔFgatg8 strain. WT or cΔFgatg8 strain was added to wells in a 96-well microtiter plate (Iwaki, Japan, 3860–096) and incubated at 22°C for 48 h. The medium was 1/10 DFM containing nitrate instead of asparagine as nitrogen source. To stimulate the formation of storage lipids the glucose content was twice the normal level in 1/10 DFM. After the 48 h incubation the nutrient solution half of the samples were replaced with 1/10 DFM without glucose and 4 times the level of nitrogen to promote the use of stored lipids. After further 48h incubation 20 µl Nile Red (Sigma-Aldrich, N-3013) staining solution was added to each well. Nile Red was used since it distributed better and thus worked better in the wells than HCS LipidTOX Red. The main disadvantage with Nile Red as a fluorescent stain for staining lipid droplets is the wide emission spectra making it difficult to use in combination with other fluorescent stains. The Nile Red solution was made just before use by mixing 30 µl stock (1 mg/ml Nile Red in DMSO) with 1 ml water. After 5 min incubation the samples were analyzed using the FITC filter in a Nikon TE 300 (Nikon, Japan) inverted fluorescence inverted microscope. Images were taken using a 10 × lens and a Nikon D200 (Nikon, Japan) camera body attached to the front of the microscope. The same exposure settings where used for all images. The use of the 10 megapixel D200 camera and recording in the 12 bit raw Nikon NEF format made it possible to record color images with enough pixel resolution and dynamic range to get representative images of many hyphae at the same time. The original images are too large to be shown in print. To show that there is no bias in the selection of “representative hyphae” for presentation, images are shown containing the largest possible amount of hyphae while the lipid droplets still can be seen as small dots. Nile Red stains most of the hyphae red while lipid droplets are golden yellow when using the standard FITC filter in a fluorescence microscope. To show lipid droplets clearly, obtained images were converted into 16 bit Tiff RGB stacks and only the green channel containing the yellow signal characteristic for the lipid droplets were used for the final images. Images were processed using the open source Java freeware ImageJ 1.37v (http://rsbweb.nih.gov/ij/).
Growth over inert plastic surface and scanning images
The strains were cultivated on DFM agar medium and incubated at RT. From the edges the colonies 13 mm diameter plugs were cut out and transferred to the bottom of empty 9 cm diameter polystyrene Petri dishes and incubated in a moist chamber for 3 d at 22°C. After incubation, images of the fungal growth over the Petri dishes were recorded using a standard flatbed scanner (CanoScan 9950F, Canon, Japan) at high resolution (1200 dpi). To get dark-field like images the scanning was done with the scanner lid open in a dark room and adjusting the contrast with the histogram function. The same settings for the histogram function was used for all images. To calculate the radial growth over the plastic surface the edge of the colony was traced using the open source Java freeware ImageJ 1.37v (http://rsbweb.nih.gov/ij/) and the colony area outside the agar plug measured to be able to calculate the mean radial increase that would have created the observed colony size for a circular colony.
Formation of asexual and sexual spores
Macroconidia of ΔFgatg8 strain, WT and cΔFgatg8 strain were produced by inoculating DFM plates and allowing them to grow for 19 d until the whole plate was completely covered and all spore production was complete. Spores was washed from the agar by transferring the colonies to 250 ml E-flasks filled with 50 ml water and shaken for 30 min on a rotary shaker (KS 260 Basic, IKA, Staufen Germany) at 200 rpm. To count the numbers of spores in the solution, 200 µl aliquots of the spore suspension was transferred to wells in a 96 well microtiter plate (Iwaki, 3860-096) and allowed to settle for 1 h prior to counting in an inverted microscope.
Sexual crosses were performed using the F. graminearum, PH-1, 1104–14, 00–676 and ΔFgatg8 strains. The strains to be crossed were placed on opposite sides of carrot agarCitation41 plates. The cultures were incubated for 7 d, at 20°C with a continuous exposure to a mix of cool white light and fluorescent black light (Black light-blue F18W / BLB-T8 from Sylvania, Danvers, MA, USA). Fertilization was induced by spreading 2 ml 2.5% aqueous Tween 60 per plate with a sterile Drigalsky spatula. The incubation continued for 21 d after which time perithecia had developed. The perithecia were isolated using a dissection microscope and a sterile scalpel and transferred to an Eppendorf tube containing 1 ml water. The perithecia were crushed using forceps and 20 µl spore suspension was spread on YPD agar plates containing 75 µg/ml hygromycin (Sigma-Aldrich, H-0654).
Plant growth, inoculation and disease assessment
Single barley (Hordeum vulgare L. cv Scarlet) and wheat (Triticum aestivum cv Leguan) plants were grown in soil in 13-cm pots in growth chambers at 17 ± 3°C and 16 h light period. Light was supplied from Master HPI-T Plus 400 W lamps (Philips). The plants were watered every second day with a nutrient solution.Citation42 In barley, inoculations were conducted on spikes 7–8 d after their emergence from the flag sheath. A macroconidial suspension (4 × 104 conidia/ml) of either WT or the ΔFgatg8 strain was applied by spray inoculation to the entire spike. To obtain a humid environment for the infection, the spikes were sprayed with water before the inoculation. To obtain good infections and avoid cross contamination, the spikes were enclosed in small plastic bags for 72 h. As a control, water sprayed spikes at the same stage of development were also bagged. Infections were evaluated at 6, 10, 14 and 17 d after inoculation.
In wheat, spikes were point-inoculated at anthesis with either the WT or the ΔFgatg8 strain by placing a droplet (10 µl) of conidia suspension (4 × 104 conidia/ml) or water within the palea and lemma of two basal florets of one spikelet in the middle of the wheat spike (modified from Jenczmionka et al.Citation43) All inoculated spikes were enclosed in small plastic bags for 72 h. Infections were evaluated at 7, 13, 17, 21, 25 and 34 d after inoculation.
Production of secondary metabolites
WT, ΔFgatg8 and cΔFgatg8 were grown on yeast malt agar (YMA, Oxoid, CM920), potato dextrose agar (PDA, Merck, 1.10130) and on Czapec dox agar (Difco, 233818) with iprodione (Sigma-Aldrich, PS1052) and dichloran (Fluka, 36010) (CzID)Citation44 for 17 d at 25°C in the dark. Extraction was performed according to Smedsgaard et al. 1997.Citation45 Liquid chromatography was performed on an Agilent 1100 LC system (Agilent Technologies, Waldbronn, Germany). Twenty microliters of extract was injected and separated on a Gemini C6-Phenyl 3 μm 2-mm i.d. × 50-mm column (Phenomenex, Torrance, CA) using a flow of 0.300 mL per minute and a gradient consisting of water (A) and 95% acetonitrile (B), which both were buffered with 1% acetic acid and 5 mM ammonium acetate. The gradient started at 0% B and was increased lineally to 100% in 11 min, then held for 3 min before decreasing again to 0% in one minute and maintained for a further 3 min. The LC was coupled to a Quattro LC triple mass spectrometer (Waters-Micromass) operating in the negative mode. The parental ion for DON was 355.2 amu [M+CH3COO]- and quantifier and qualifier ions were 265.2 and 59.2 amu respectively. For zearalenone the parental ion was 317.2 [M-H]- and 131 and 171 amu quantifier and qualifier ions. Aurofusarin was analyzed using 569.1 amu [M-H]- using 482.8 and 427 amu as quantifier and qualifier ions. Concentrations of DON (Sigma-Aldrich, D0156) and ZEA (Sigma-Aldrich, Z2125) were quantified using a 2-fold dilution series ranging from 0.16 to 5.0 μg/mL, whereas aurofusarin was measured relatively in the samples as no reference standard was available.
Figure S1. Colonies on DFM agar 6 d after plug transfer. Incubated at 22°C. Colonies are photographed sideways to show the limited development of the aerial mycelium in the deletion mutants and the restoration of it in the complemented strains. Petri dishes = 9 cm diameter. For explanation of the strains see .
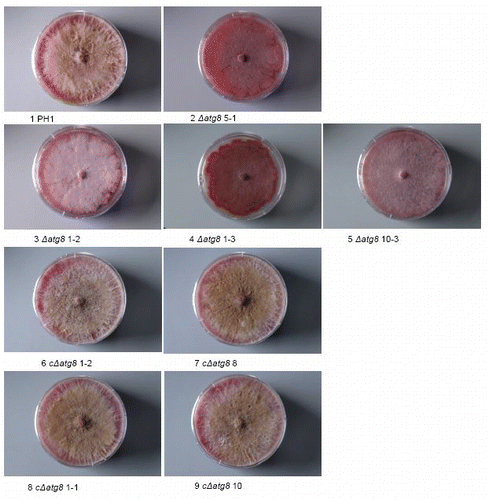
Figure S2. Colonies grown on DFM agar 26 d after plug transfer. Incubated at 22°C. Colonies are side. lit to show the limited development of the aerial mycelium in the deletion mutants and the restoration of it in the complemented strains. Petri dishes = 9 cm diameter. For explanation of the strains see .
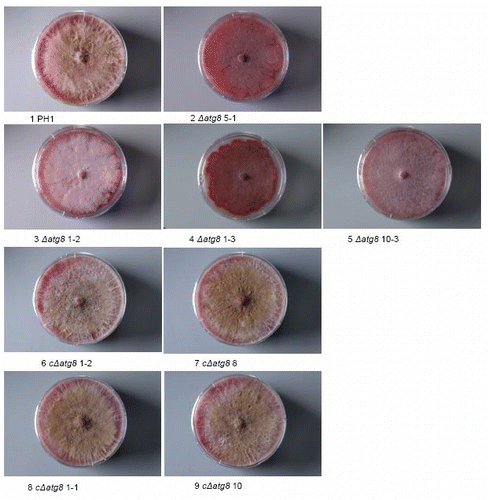
Figure S3. Aerial mycelium development on 18 × 18 mm (image size) cover glasses inserted in DFM agar (bottom part without aerial mycelium) after 4 d of growth. For explanation of the strains see .
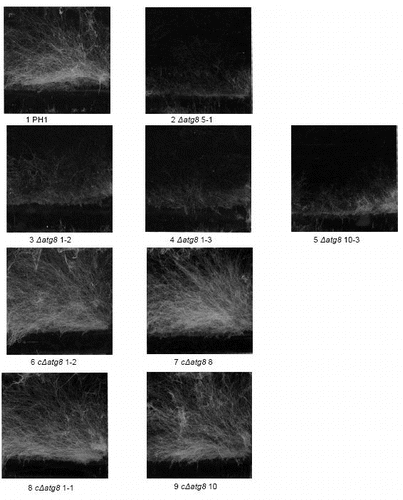
Figure S4. Lipid droplet formation in the aerial mycelium growing on cover glasses after 4 d. Stain = HCS LipidTOX Red. DIC image (left) showing the mycelium and right the corresponding section seen by fluorescence mycroscopy. The fluorescence images were exposed the same length of time and the resulting digital images treated in exactly the same way. Size bars = 20 μm. For explanation of the strains see .
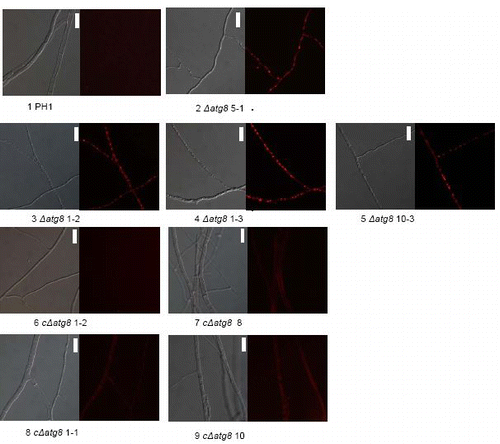
Figure S5. Growth over inert plastic surface 3 d after plug transfer incubated at 22°C in moist chamber. Petri dishes = 9 cm diameter. For explanation of the strains see .
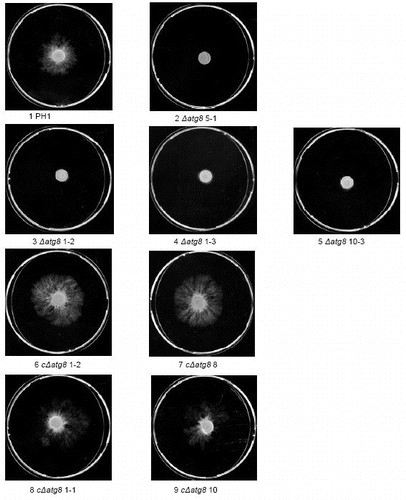
Figure S6. Radial extension measurements by determining the area of plastic colonized after 3 d on a plastic surface from images like in and then calculating what radial growth is needed to produce a completely circular colony with the same area. Error bars = SEM. For strain No explanation see slide 1.
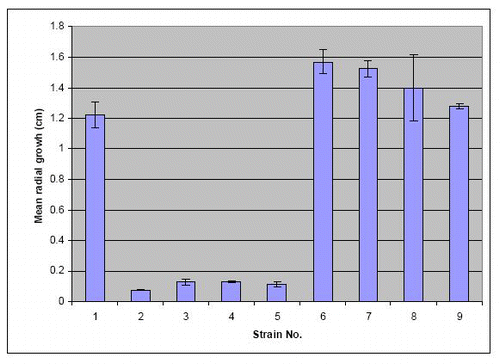
Figure S7. Preparation of fungal race pipettes showing the new techniques. Pipettes just poured and capped with sterile Eppendorf tubes (A). Inoculation of pipettes with the aid of a sterile toothpick (B). The final capped tube with capping Eppendorph tube secured with cello tape (C) (lid cut off the tube). Race pipette after incubation and readings (D) (markings were mainly done on the underside of this pipette).
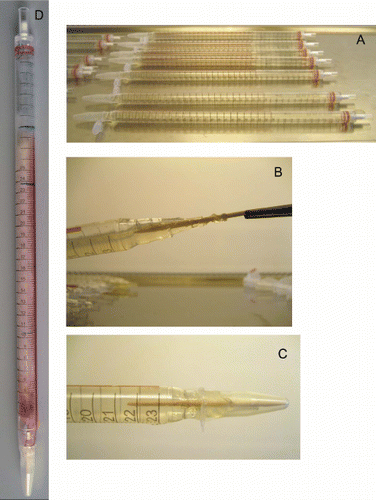
Table S1. Key to strain codes used in supplement compared with in the article. The codes of the three strains referred to in the article are in brackets
Abbreviations: | ||
aa | = | amino acids |
BLAST | = | basic local alignment search tool |
CzID | = | Czapek dox agar supplemented with iprodione and dichloran |
d | = | days |
DAPI | = | 4′,6-diamidino-2-phenylindole |
DFM | = | defined fusarium medium |
DIC | = | differential interference contrast |
DMSO | = | dimethyl sulfoxide |
DNA | = | deoxyribonucleic acid |
DON | = | deoxynivalenol |
FHB | = | Fusarium head blight |
FITC | = | fluorescein isothiocyanate |
MDC | = | monodansylcadaverine |
PCR | = | polymerase chain reaction |
PDA | = | potato dextrose agar |
RT | = | room temperature |
WT | = | wild type |
YMA | = | yeast malt agar |
YPD | = | yeast extract peptone dextrose |
ZEA | = | zearalenone |
Additional material
Download Zip (1.6 MB)Acknowledgments
This work was supported by a grant from the Villum Kann Rasmussen Foundation.
Disclosure of Potential Conflicts of Interest
There is no conflict of interest that I should disclose that could be perceived to bias our work, acknowledging all financial support and any other personal connections.
References
- Pollack JK, Harris SD, Marten MR. Autophagy in filamentous fungi. Fungal Genet Biol 2009; 46:1 - 8; http://dx.doi.org/10.1016/j.fgb.2008.10.010; PMID: 19010432
- Klionsky DJ, Emr SD. Cell biology - Autophagy as a regulated pathway of cellular degradation. Science 2000; 290:1717 - 21; http://dx.doi.org/10.1126/science.290.5497.1717; PMID: 11099404
- Bursch W. The autophagosomal-lysosomal compartment in programmed cell death. Cell Death Differ 2001; 8:569 - 81; http://dx.doi.org/10.1038/sj.cdd.4400852; PMID: 11536007
- Veses V, Richards A, Gow NAR. Vacuoles and fungal biology. Curr Opin Microbiol 2008; 11:503 - 10; http://dx.doi.org/10.1016/j.mib.2008.09.017; PMID: 18935977
- Farré JC, Krick R, Subramani S, Thumm M. Turnover of organelles by autophagy in yeast. Curr Opin Cell Biol 2009; 21:522 - 30; http://dx.doi.org/10.1016/j.ceb.2009.04.015; PMID: 19515549
- Meijer WH, van der Klei IJ, Veenhuis M, Kiel JAKW. ATG genes involved in non-selective autophagy are conserved from yeast to man, but the selective Cvt and pexophagy pathways also require organism-specific genes. Autophagy 2007; 3:106 - 16; PMID: 17204848
- Xie Z, Nair U, Klionsky DJ. Atg8 controls phagophore expansion during autophagosome formation. Mol Biol Cell 2008; 19:3290 - 8; http://dx.doi.org/10.1091/mbc.E07-12-1292; PMID: 18508918
- Pinan-Lucarré B, Paoletti M, Dementhon K, Coulary-Salin B, Clave C. Autophagy is induced during cell death by incompatibility and is essential for differentiation in the filamentous fungus Podospora anserina. Mol Microbiol 2003; 47:321 - 33; http://dx.doi.org/10.1046/j.1365-2958.2003.03208.x; PMID: 12519185
- Nolting N, Bernhards Y, Poggeler S. SmATG7 is required for viability in the homothallic ascomycete Sordaria macrospora. Fungal Genet Biol 2009; 46:531 - 42; http://dx.doi.org/10.1016/j.fgb.2009.03.008; PMID: 19351563
- Asakura M, Ninomiya S, Sugimoto M, Oku M, Yamashita S, Okuno T, et al. Atg26-Mediated Pexophagy Is Required for Host Invasion by the Plant Pathogenic Fungus Colletotrichum orbiculare. Plant Cell 2009; 21:1291 - 304; http://dx.doi.org/10.1105/tpc.108.060996; PMID: 19363139
- Veneault-Fourrey C, Barooah M, Egan M, Wakley G, Talbot NJ. Autophagic fungal cell death is necessary for infection by the rice blast fungus. Science 2006; 312:580 - 3; http://dx.doi.org/10.1126/science.1124550; PMID: 16645096
- Nadal M, Gold SE. The autophagy genes ATG8 and ATG1 affect morphogenesis and pathogenicity in Ustilago maydis. Mol Plant Pathol 2010; 11:463 - 78; http://dx.doi.org/10.1111/j.1364-3703.2010.00620.x; PMID: 20618705
- Boenisch MJ, Schafer W. Fusarium graminearum forms mycotoxin producing infection structures on wheat. BMC Plant Biol 2011; 11:110; http://dx.doi.org/10.1186/1471-2229-11-110; PMID: 21798058
- Ribichich KF, Lopez SE, Vegetti AC. Histopathological spikelet changes produced by Fusarium graminearum in susceptible and resistant wheat cultivars. Plant Dis 2000; 84:794 - 802; http://dx.doi.org/10.1094/PDIS.2000.84.7.794
- Wanjiru WM, Kang ZS, Buchenauer H. Importance of cell wall degrading enzymes produced by Fusarium graminearum during infection of wheat heads. Eur J Plant Pathol 2002; 108:803 - 10; http://dx.doi.org/10.1023/A:1020847216155
- Nguyen LN, Bormann J, Le GTT, Stärkel C, Olsson S, Nosanchuk JD, et al. Autophagy-related lipase FgATG15 of Fusarium graminearum is important for lipid turnover and plant infection. Fungal Genet Biol 2011; 48:217 - 24; http://dx.doi.org/10.1016/j.fgb.2010.11.004; PMID: 21094265
- Jansen C, von Wettstein D, Schafer W, Kogel KH, Felk A, Maier FJ. Infection patterns in barley and wheat spikes inoculated with wild-type and trichodiene synthase gene disrupted Fusarium graminearum. Proc Natl Acad Sci USA 2005; 102:16892 - 7; http://dx.doi.org/10.1073/pnas.0508467102; PMID: 16263921
- Martínez-Force E, Benítez T. Effects of varying media, temperature, and growth rates on the intracellular concentrations of yeast amino acids. Biotechnol Prog 1995; 11:386 - 92; http://dx.doi.org/10.1021/bp00034a004; PMID: 7654310
- Bai GH, Desjardins AE, Plattner RD. Deoxynivalenol-nonproducing fusarium graminearum causes initial infection, but does not cause disease spread in wheat spikes. Mycopathologia 2002; 153:91 - 8; http://dx.doi.org/10.1023/A:1014419323550; PMID: 12000132
- Kirisako T, Ichimura Y, Okada H, Kabeya Y, Mizushima N, Yoshimori T, et al. The reversible modification regulates the membrane-binding state of Apg8/Aut7 essential for autophagy and the cytoplasm to vacuole targeting pathway. J Cell Biol 2000; 151:263 - 76; http://dx.doi.org/10.1083/jcb.151.2.263; PMID: 11038174
- Deng YZ, Ramos-Pamplona M, Naqvi NI. Methods for functional analysis of macroautophagy in filamentous fungi. Methods Enzymol 2008; 451:295 - 310; http://dx.doi.org/10.1016/S0076-6879(08)03220-5; PMID: 19185728
- Liu XH, Lu JP, Zhang L, Dong B, Min H, Lin FC. Involvement of a Magnaporthe grisea serine/threonine kinase gene, MgATG1, in appressorium turgor and pathogenesis. Eukaryot Cell 2007; 6:997 - 1005; http://dx.doi.org/10.1128/EC.00011-07; PMID: 17416896
- Pinan-Lucarré B, Baiguerie A, Clave C. Accelerated cell death in Podospora autophagy mutants. Eukaryot Cell 2005; 4:1765 - 74; http://dx.doi.org/10.1128/EC.4.11.1765-1774.2005; PMID: 16278443
- Kikuma T, Ohneda M, Arioka M, Kitamoto K. Functional analysis of the ATG8 homologue Aoatg8 and role of autophagy in differentiation and germination in Aspergillus oryzae. Eukaryot Cell 2006; 5:1328 - 36; http://dx.doi.org/10.1128/EC.00024-06; PMID: 16896216
- Richie DL, Fuller KK, Fortwendel J, Miley MD, McCarthy JW, Feldmesser M, et al. Unexpected link between metal ion deficiency and autophagy in Aspergillus fumigatus. Eukaryot Cell 2007; 6:2437 - 47; http://dx.doi.org/10.1128/EC.00224-07; PMID: 17921348
- Righelato RC, Trinci APJ, Pirt SJ, Peat A. The influence of maintenance energy and growth rate on the metabolic activity, morphology and conidiation of Penicillium chrysogenum. J Gen Microbiol 1968; 50:399 - 412; PMID: 5652074
- Lew RR. How does a hypha grow? The biophysics of pressurized growth in fungi. Nat Rev Microbiol 2011; 9:509 - 18; http://dx.doi.org/10.1038/nrmicro2591; PMID: 21643041
- Weber RWS, Wakley GE, Thines E, Talbot NJ. The vacuole as central element of the lytic system and sink for lipid droplets in maturing appressoria of Magnaporthe grisea. Protoplasma 2001; 216:101 - 12; http://dx.doi.org/10.1007/BF02680137; PMID: 11732192
- Ryan FJ, Beadle GW, Tatum EL. The tube method of measuring the growth rate of Neurospora. Am J Bot 1943; 30:784 - 99; http://dx.doi.org/10.2307/2437554
- Singh R, Kaushik S, Wang YJ, Xiang YQ, Novak I, Komatsu M, et al. Autophagy regulates lipid metabolism. Nature 2009; 458 1131 5; PMID: 19779424
- Halloran GM, Lee JW. Plant Nitrogen Distribution in Wheat Cultivars. Aust J Agric Res 1979; 30:779 - 89; http://dx.doi.org/10.1071/AR9790779
- Ilgen P, Maier FJ, Schäfer W. Trichothecenes and lipases are host-induced and secreted virulence factors of Fusarium graminearum. Cereal Res Commun 2008; 36:421 - 8; http://dx.doi.org/10.1556/CRC.36.2008.Suppl.B.35
- Gardiner DM, Kazan K, Manners JM. Novel genes of Fusarium graminearum that negatively regulate deoxynivalenol production and virulence. Mol Plant Microbe Interact 2009; 22:1588 - 600; http://dx.doi.org/10.1094/MPMI-22-12-1588; PMID: 19888824
- Gale LR, Bryant JD, Calvo S, Giese H, Katan T, O'Donnell K, et al. Chromosome complement of the fungal plant pathogen Fusarium graminearum based on genetic and physical mapping and cytological observations. Genetics 2005; 171:985 - 1001; http://dx.doi.org/10.1534/genetics.105.044842; PMID: 16079234
- Langseth W, Bernhoft A, Rundberget T, Kosiak B, Gareis M. Mycotoxin production and cytotoxicity of Fusarium strains isolated from Norwegian cereals. Mycopathologia 1998-1999; 144:103 - 13; http://dx.doi.org/10.1023/A:1007016820879; PMID: 10481290
- Malz S, Grell MN, Thrane C, Maier FJ, Rosager P, Felk A, et al. Identification of a gene cluster responsible for the biosynthesis of aurofusarin in the Fusarium graminearum species complex. Fungal Genet Biol 2005; 42:420 - 33; http://dx.doi.org/10.1016/j.fgb.2005.01.010; PMID: 15809006
- Frandsen RJN, Andersson JA, Kristensen MB, Giese H. Efficient four fragment cloning for the construction of vectors for targeted gene replacement in filamentous fungi. BMC Mol Biol 2008; 9:70; http://dx.doi.org/10.1186/1471-2199-9-70; PMID: 18673530
- Tobiasen C, Aahman J, Ravnholt KS, Bjerrum MJ, Grell MN, Giese H. Nonribosomal peptide synthetase (NPS) genes in Fusarium graminearum, F. culmorum and F. pseudograminearium and identification of NPS2 as the producer of ferricrocin. Curr Genet 2007; 51:43 - 58; http://dx.doi.org/10.1007/s00294-006-0103-0; PMID: 17043871
- Ryan FJ, Beadle GW, Tatum EL. The tube method of measuring the growth rate of Neurospora. Am J Bot 1943; 30:784 - 99; http://dx.doi.org/10.2307/2437554
- White B, Woodward D. A simple method for making disposable race tubes. Fungal Genet Newsl 1995; 42:79
- Klittich C, Leslie JF. Nitrate Reduction Mutants of Fusarium moniliforme (Gibberella fujikuroi). Genetics 1988; 118:417 - 23; PMID: 17246415
- Skov J, Lemmens M, Giese H. Role of a Fusarium culmorum ABC transporter (FcABC1) during infection of wheat and barley. Physiol Mol Plant Pathol 2004; 64:245 - 54; http://dx.doi.org/10.1016/j.pmpp.2004.09.005
- Jenczmionka NJ, Maier FJ, Losch AP, Schafer W. Mating, conidiation and pathogenicity of Fusarium graminearum, the main causal agent of the head-blight disease of wheat, are regulated by the MAP kinase gpmk1. Curr Genet 2003; 43:87 - 95; PMID: 12695848
- Samson RA, Houbraken J, Thrane U, Frisvad JC, Andersen B. Food and indoor fungi. CBS “KNAW” Fungal Biodiversity Centre, Utrecht, The Netherlands, 2010.
- Smedsgaard J. Micro-scale extraction procedure for standardized screening of fungal metabolite production in cultures. J Chromatogr A 1997; 760:264 - 70; http://dx.doi.org/10.1016/S0021-9673(96)00803-5; PMID: 9062989