Abstract
Our study first proposed that curcumin could protect human endothelial cells from the damage caused by oxidative stress via autophagy. Furthermore, our results revealed that curcumin causes some novel cellular mechanisms that promote autophagy as a protective effect. Pretreatment with curcumin remarkably improves the survival of human umbilical vein endothelial cells (HUVECs) from H2O2-induced viability loss, which specifically evokes an autophagic response. Exposed to H2O2, curcumin-treated HUVECs upregulate the level of microtubule-associated protein 1 light chain 3-II (LC3-II), the number of autophagosomes, and the degradation of p62. We show that this compound promotes BECN1 expression and inhibits the phosphatidylinositol 3-kinase (PtdIns3K)-AKT-mechanistic target of rapamycin (MTOR) signaling pathway. Curcumin can also reverse FOXO1 (a mediator of autophagy) nuclear localization along with causing an elevated level of cytoplasmic acetylation of FOXO1 and the interaction of acetylated FOXO1 and ATG7, under the circumstance of oxidative stress. Additionally, knockdown of FOXO1 by shRNA inhibits not only the protective effects that curcumin induced, but the autophagic process, from the quantity of LC3-II to the expression of RAB7. These results suggest that curcumin induces autophagy, indicating that curcumin has the potential for use as an autophagic-related antioxidant for prevention and treatment of oxidative stress. These data uncover a brand new protective mechanism involving FOXO1 as having a critical role in regulating autophagy in HUVECs, and suggest a novel role for curcumin in inducing a beneficial form of autophagy in HUVECs, which may be a potential multitargeted therapeutic avenue for the treatment of oxidative stress-related cardiovascular diseases.
Introduction
Curcumin is a hydrophobic polyphenol derived from turmeric: the rhizome of the herb Curcuma longa. Accumulating evidence suggests that curcumin is a highly pleiotropic molecule that modulates abundant targets, which influence numerous biochemical and molecular functions.Citation1,Citation2 Although this polyphenol was studied for several decades in particular with regard to its ability for modulating various targets through either gene expression or direct interaction, and hence its potential use in treating cancer, there are few studies that highlight the cardiovascular protective effects of this compound. It is reported that curcumin has an antioxidant effect on endothelial cells,Citation3 but a more specific mechanism remains to be uncovered.
Macroautophagy (hereafter referred to as autophagy) is an evolutionarily conserved catabolic mechanism used by most kinds of mammalian cells that involves the sequestration, transport, bulk degradation and recycling of cytoplasmic components, such as long-lived proteins and organelles.Citation4,Citation5 In cardiovascular cells, autophagy acts predominantly as a prosurvival pathway, protecting the cells from oxidative stress.Citation6-Citation8 So the induction of autophagy, which is correlated with enhanced cell survival and decreased apoptosis, might be a potential mechanism underlying the protective effects of curcumin against oxidative stress.
FOXO1 (also known as forkhead in rhabdomyosarcoma, or FKHR) is a member of the forkhead box O (FOXO) family, which a series of recent studies have demonstrated plays an important role in several intracellular functions, including metabolic regulation, longevity, cell cycle, cell death, stress response and tumor suppression.Citation9-Citation13 It is widely reported that FOXO1 either promotes or inhibits apoptosis in different cell types.Citation10,Citation14 Various experiments pointed out that these specific functions, in the case of FOXO1 factors, are in response to signals that induce the appropriate post-translational modification of FOXO1, and these post-translational modifications are the predominant mechanisms regulating FOXO1 transcriptional activities and modulating FOXO1 protein stability, DNA-binding activity, protein-protein interactions, and subcellular localization.Citation15 Upon oxidative stress, recent work has uncovered an important role for FOXO1 regulating autophagy, not only because FOXO1 can promote expression of several autophagy-related genes, but also the FOXO1 post-translational modifications can participate in the autophagic process.Citation16-Citation18 All evidence indicates that FOXO1 and autophagy are intimately related, but the precise molecular mechanisms underlying these processes still remain obscure.
Here, by examining how curcumin promotes autophagy flux in a dose-dependent manner in endothelial cells, and determining the important component in curcumin-mediated protection against oxidative stress, our study indicates that endothelial cells initiate an autophagic survival response during oxidative stress under curcumin pretreatment. Therefore, by induction of autophagy the survival response curcumin can potentiate its biological effects.
Results
Curcumin protected HUVECs from H2O2-induced apoptosis
Cell survival was tested following treatment with a range of concentrations (from 100 μmol/L to 1 mmol/L) of H2O2 and it was found that H2O2 significantly decreased cell survival in a dose-dependent manner (Fig. S1). According to the results, to provide a maximum dynamic range for quantifying protective vs. harmful responses, treatment with 200 μmol/L H2O2 for 4 h was chosen in the subsequent experiments. To evaluate whether curcumin protects HUVECs from oxidative stress, a CCK-8 assay was used to determine cell viability (). As illustrated, cells treated with 200 μmol/L H2O2 for 4 h showed typical characteristics of apoptosis, whereas curcumin (1, 5, 10 μmol/L) prevented H2O2-dependent damage, restoring cell survival following the oxidative stress.
Figure 1. The protective effects of curcumin on H2O2-induced cytotoxicity in HUVECs. (A) Effects of curcumin on the viability of HUVECs treated with H2O2. HUVECs were exposed to various concentrations of curcumin (1, 5, 10, 50 μmol/L) for 24 h. Then, cells were treated with H2O2 (200 μmol/L) for 4 h and cell viability was determined by CCK-8 assay. Values are expressed as the mean ± SD, n = 3. #p < 0.05 H2O2 alone group vs. H2O2-free group (Control); ***p < 0.001 vs. H2O2 alone group. (B and C) Treated HUVECs were subjected to TUNEL and Hoechst staining. (B) Representative staining with TUNEL. (C) The number of TUNEL-positive nuclei was expressed as a percentage of total nuclei detected by Hoechst staining. **p < 0.001 vs. H2O2 alone group. (D) Representative western blot analysis of cleaved caspase-3, caspase-9 and β-actin in curcumin (1, 5, 10 μmol/L)-treated HUVECs. (E) Densitometric analysis was used to quantify the levels of caspase-3 and (F) caspase-9 activation. Values were normalized against β-actin and presented as fold increase compared with the basal level (Control). Data was shown as mean ± SD, n = 3. ##p < 0.01, H2O2 alone group vs. H2O2-free group (Control); **p < 0.01, ***p < 0.001 vs. H2O2 alone group.
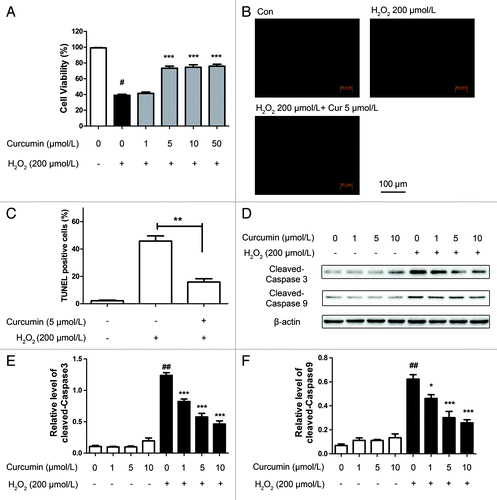
Based on the TUNEL assay and Hoechst staining, H2O2-treated cells exhibited the characteristics of the early stages of apoptotic cell death. Compared with cells without curcumin pretreatment that displayed a high level of TUNEL-positive staining, the number of TUNEL-positive cells of the curcumin-pretreated groups appeared to decline dramatically and dose dependently ().
To confirm whether H2O2-induced cell death was apoptotic, we then explored the impact of the expression of caspase-3 and caspase-9 by western blot analysis (). Significantly, the reduction of caspase-3 and caspase-9 activity was detected in the cells treated with curcumin, also in a dose-dependent manner, which is consistent with previous reports on the reduction of apoptosis by curcumin.
Curcumin promoted autophagy in HUVECs
To estimate the promotion of autophagy by curcumin in HUVECs, and separately evaluate the extent of autophagosome and autolysosome accumulation, we transfected HUVECs with a marker protein, mCherry-EGFP-LC3 tandem tagged fluorescent protein (tfLC3) and analyzed them by confocal microscopy. The green LC3 puncta primarily represent autophagosomes, whereas red LC3 puncta indicate both autophagosomes and autolysosomes, since mCherry retains its fluorescence even in the acidic environment of lysosomes, whereas GFP loses its fluorescence. The red puncta that overlayed with the green puncta and appeared yellow in merged images were thus indicators of autophagosomes, whereas the solely red puncta were indicative of autolysosomes.Citation19,Citation20 Not only were the numbers of green and red puncta both significantly higher after curcumin (1, 5, 10 μmol/L) treatment (), but the yellow puncta were also significantly increased after curcumin in the merged images, which meant increased autophagosomes. These results imply that autophagic flux was enhanced in response to curcumin in HUVECs.
Figure 2. Curcumin induces autophagy in HUVECs. (A) Confocal images of representative images of GFP and mCherry fluorescent puncta in HUVECs transfected with mCherry-EGFP-LC3 for 24 h, and treated with different concentrations (1, 5, 10 μmol/L) of curcumin for 24 h, and finally with H2O2 (200 μmol/L) for 4 h. (B) Quantification of GFP/mCherry double-positive and mCherry single-positive puncta per cell in control or cells treated with curcumin (1, 5, 10 μmol/L) for 24 h, then H2O2 (200 μmol/L) for 4 h. Data were the mean value of three independent experiments with each count of no less than 100 cells. Values are expressed as the mean ± SD, n = 3, *p < 0.05, ***p < 0.001 vs. H2O2 alone group. (C) western blot analysis of endogenous LC3 and p62 after challenge with curcumin of different concentrations (1, 5, 10 μmol/L) for 24 h and H2O2 (200 μmol/L) for 4 h revealed LC3-II accumulation and p62 degradation. (D) Bar charts show the quantification of endogenous LC3-II and (E) p62. Values are expressed as the mean ± SD, n = 3, **p < 0.01, ***p < 0.001 vs. H2O2 alone group.
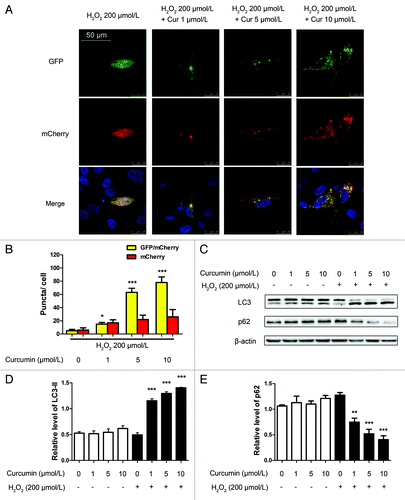
To confirm whether pretreatment with curcumin (1, 5, 10 μmol/L) and exposure to 200 μmol/L H2O2 triggered autophagy in HUVECs, we examined levels of the microtubule-associated protein 1 light chain 3-II (LC3-II), which is a LC3-phosphatidylethanolamine conjugate and an autophagosomal marker.Citation20 Western blot assay showed that the ratio of LC3-II/β-actin markedly increased in a dose-dependent manner with curcumin (1, 5, 10 μmol/L) treatment over 24 h then with 200 μmol/L H2O2 stimulation for 4 h in HUVECs (). Furthermore, it was accompanied by the loss of p62 which functions as an adaptor in autophagy ().Citation21
Curcumin downregulated the PtdIns3K-AKT-mTOR signaling pathway in HUVECs exposed to oxidative stress
Consistent with these results, we examined Ser473 phosphorylation of AKT, which measured both AKT-mTOR and mTORC2 activity.Citation22 Facing oxidative stress, pretreatment with curcumin (1, 5, 10 μmol/L) also decreased the level of phosphorylated AKT and mTOR in a dose-dependent manner (). Moreover, within 4 h, by observing the 200 μmol/L H2O2-injured and with 5 μmol/L curcumin group in terms of time, we found that phosphorylated AKT and mTOR were significantly reduced after 30 min (). These results suggest that 5 μmol/L curcumin inhibits the PtdIns3K-AKT-mTOR signaling pathway and induces autophagy.
Figure 3. Curcumin inhibits the PtdIns3K-AKT-mTOR signaling pathway. (A) HUVECs with different concentrations (1, 5, 10 μmol/L) of curcumin for 24 h stimulated with H2O2 (200 μmol/L) for 4 h. Western blots were analyzed for AKT, mTOR and their phosphorylation activity, and β-actin. (B) The quantification of phosphorylation of AKT and (C) phosphorylation of mTOR intensity. Values are expressed as the mean ± SD, n = 3; #p < 0.05, H2O2 alone group vs. H2O2-free group (Control); *p < 0.05, **p < 0.01, ***p < 0.001, vs. H2O2 alone group. (D) The HUVECs with 5 μmol/L curcumin for 24 h stimulated with H2O2 (200 μmol/L) for 4 h lysates at different time points were analyzed by western blot for detection of AKT, mTOR and their phosphorylation activity, and β-actin.
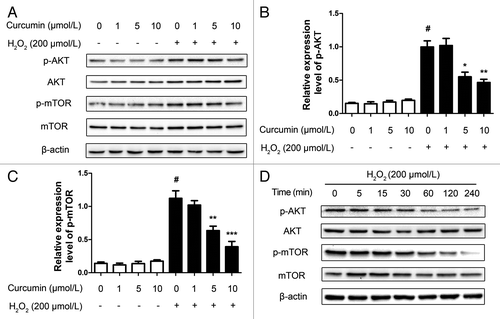
Curcumin mediated BECN1-BCL-2 interactions in HUVECs, and escalated the level of cytoplasmic acetylation of FOXO1 in autophagy
It has been shown that a BECN1-BCL-2 complex functions as a regulator of autophagic capacity, which promotes cell homeostasis.Citation23 To elucidate whether curcumin treatment affected BECN1 and BCL-2 expression, total cellular proteins were analyzed by western blot. The levels of BCL-2 remained unchanged over the period of H2O2 injury, but BECN1 levels increased (). To describe the possibility that the interaction between BCL-2 and BECN1 regulated curcumin-induced autophagy, the endogenous BECN1-BCL-2 complex was immunoprecipitated with antibody against BCL-2. The immunoprecipitation (IP) and western blots showed that the levels of BECN1 co-immunoprecipitating with BCL-2 steadily decreased for 4 h, also in a dose-dependent manner (). These results indicate that curcumin induces autophagy in HUVECs by upregulating BECN1 expression and weakening the interaction between BECN1 and BCL-2, so that the free BECN1 activated autophagy. Interestingly, the small but consistent increase in BECN1 levels at 4 h after 5 μmol/L curcumin pretreatment and H2O2 injury coincided with the reduction of cleaved caspase-3 and cleaved caspase-9. Altogether, the data imply that increased emancipation of BECN1 from the BECN1-BCL-2 complex delays the onset of apoptosis.
Figure 4. Curcumin promotes BECN1 expression and mediates BECN1-BCL-2 interactions in HUVECs and escalated cytoplasmic deacetylation of the FOXO1 level in autophagy. (A) Representative western blot analysis for BECN1 and β-actin in curcumin-treated HUVECs stressed by H2O2 (200 μmol/L). Total cell lysates from different curcumin concentration-treated HUVECs were collected and subjected to western blot analysis. (B) Densitometric analysis was used to quantify the levels of BECN1. Values are expressed as the mean ± SD, n = 3; *p < 0.05, vs. H2O2 alone group. (C) Co-immunoprecipitation of BECN1 and BCL-2 in HUVECs. Cells were treated as above, the cell lysate was extracted for co-immunoprecipitation with anti-BCL-2, followed by probing with anti-BECN1. Western blot analyzed the expression of acetylation of FOXO1, phosphorylation of FOXO1and SIRT1, (D and E) either with different concentrations (1, 5, 10 μmol/L) of curcumin for 24 h stimulated with H2O2 (200 μmol/L) for 4 h, (F) or 5 μmol/L curcumin for 24 h stimulated with H2O2 (200 μmol/L) for 4 h at different time points of H2O2 (200 μmol/L) stimulation. Values are expressed as the mean ± SD, n = 3; *p < 0.05, **p < 0.01 vs. H2O2 alone group.
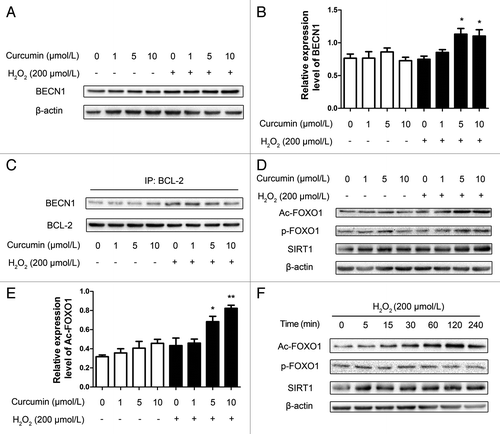
In the cytoplasm, the changes of phosphorylation and acetylation of FOXO1 were assayed by western blot (). Acetylation of FOXO1 (Ac-FOXO1) was increased dose dependently after oxidative stress under the influence of curcumin. Meanwhile, there was no obvious change in the phosphorylation of FOXO1. From the western blot results of different time points, it could be seen that the acetylation of FOXO1 started to be enhanced at 1 h (), but there was no significant change in the phosphorylation of FOXO1.
Curcumin prevented FOXO1 nuclear localization in the induction of oxidative stress by H2O2
To evaluate the effect of oxidative stress on endogenous FOXO1 subcellular localization, western blot results of cellular fractionation, from nucleus and cytoplasm separately, and the ratio of nucleus/cytoplasm was analyzed. Both showed that curcumin inhibited the nuclear localization of FOXO1 (). To confirm these results, cultured HUVECs were treated with H2O2. Oxidative stress by H2O2 treatment caused FOXO1 to translocate from the cytoplasm to the nucleus, resulting in predominant nuclear localization of FOXO1 indicative of activation, in contrast with the group pretreated with curcumin (). Meanwhile, HUVECs treated with 5 μmol/L curcumin for 24 h, at different time points of H2O2 (200 μmol/L) stimulation (30 min, 1 h, 2 h and 4 h), demonstrated a significant repression of FOXO1 nuclear localization. FOXO1 was localized to the cytoplasm and perinuclear region, compared with the group exposed to oxidative stress, which only caused predominant nuclear localization of FOXO1 within 1 h, and lasted for 4 h (, for statistical results see Fig. S3).
Figure 5. Curcumin prevents endogenous FOXO1 nuclear localization in the induction of oxidative stress by H2O2. (A) Cell fractionation of HUVECs curcumin-treated and stressed by H2O2 (200 μmol/L) was performed separately to analyze FOXO1 cellular localization. Histone 1 (H1) is the loading control for nuclear protein, and β-actin is the loading control for cytoplasmic protein. (B) The quantification of the FOXO1 cellular localization was analyzed by the ratio of nucleus/cytoplasm. Values are expressed as the mean ± SD, n = 3; #p < 0.05, H2O2 alone group vs. H2O2-free group (Control); **p < 0.01, ***p < 0.001 vs. H2O2 alone group. (C) Using immunofluorescence microscopy, representative images of fluorescent images of HUVECs showing endogenous FOXO1 nuclear localization, compared with the inhibition of this process with curcumin treatment. (D) The fluorescent relative quantitative analysis of nuclear/cytoplasm FOXO1. Values are expressed as the mean ± SD, n = 3; ***p < 0.001 vs. H2O2 alone group. (E) HUVECs treated with 5 μmol/L curcumin for 24 h, at different time points of H2O2 (200 μmol/L) stimulation (30 min, 1 h, 2 h and 4 h), were immunostained with FOXO1 (red) antibodies. FOXO1 proteins are localized to the cytoplasm and perinuclear region. Nuclei were labeled by DAPI. Oxidative stress causes predominant nuclear localization of FOXO1 within 1 h, and lasts for 4 h.
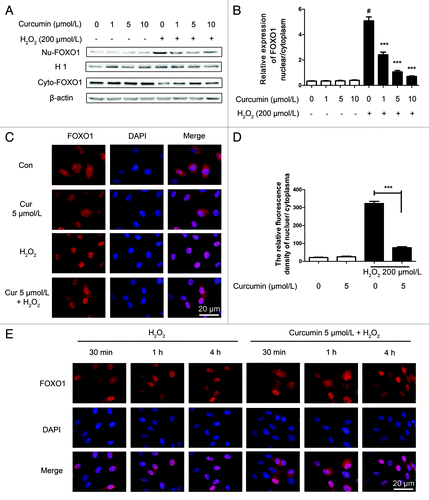
Knockdown of FOXO1 inhibited curcumin-induced autophagy
We utilized a short hairpin-based RNA interference vector construct to silence human FOXO1 gene expression in HUVECs. In preliminary screening, transient transfection of the shRNA pSUPER.GFP/neo expression vector specifically reduced the amount of FOXO1 protein (shFOXO1) detectable in HUVECs, by 70–80% after 48 h transfection, compared with a control group transiently transfected with nontargeting scramble shRNA (shScr) as measured by western blotting (Fig. S4). To test whether curcumin-induced protection was dependent on autophagy, we determined whether the inhibition of autophagy by 3-methyladenine (3-MA) or the shFOXO1 plasmid affected the curcumin-induced protection in HUVECs. We had HUVECs tranfected with pSUPER-FOXO1 and pSUPER-Scramble vector separately for 24 h, then treated the cells with curcumin for 24 h, one of which was given 10 mmol/L of 3-MA, and finally injured by exposure to H2O2 for 4 h. Compared with the unstressed groups, the cell viability of curcumin treatment shScr showed a significant recovery from oxidative stress; however, the same treatment of curcumin in shFOXO1 and shScr with 3-MA groups did not show improved survival ().
Figure 6. Knockdown of FOXO1 inhibited curcumin-induced autophagy. HUVECs were transfected with shFOXO1, and transfected with shScramble (shScr) as a control. After curcumin-treated HUVECs were stressed by H2O2 (200 μmol/L), (A) cell viability was examined, and (B) immunoblots and densitometry were used to analyze endogenous LC3, p62, caspase-3 and caspase-9 expression, with β-actin as a loading control. (C and D) HUVECs treated similarly were subjected to TUNEL staining. (C) Representative staining with TUNEL is shown. (D) The number of TUNEL-positive nuclei was expressed as a percentage of total nuclei detected by Hoechst staining. Values are expressed as the mean ± SD, n = 3; #p < 0.05, shScr with curcumin vs. shScr control (basal line); *p < 0.05, **p < 0.01, ***p < 0.001 vs. shScr with curcumin group.
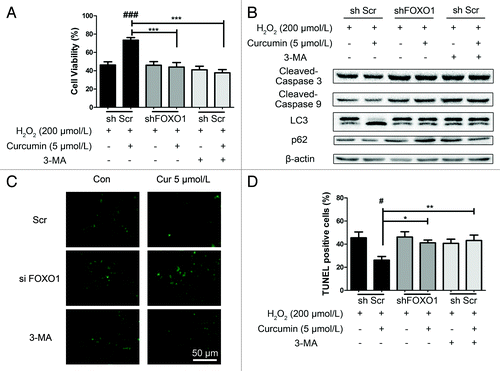
To further confirm this result, we observed the level of endogenous LC3 and p62 in these groups. Curcumin triggered an autophagic LC3-II increase in the cells transfected with shScr vector, whereas the other groups, which did not have an improvement in cell viability, still showed LC3-I in the stressed HUVECs. Furthermore, discernible activation of cleaved caspase-3 and cleaved caspase-9 was observed in the 3-MA groups, as well as the shFOXO1 groups. In contrast, a lower rate of HUVEC apoptosis was induced by curcumin in the shScr group (, for statistical results see Fig. S5A–E). Parallel experiments with TUNEL staining () again showed an increase in apoptosis of shFOXO1 HUVECs and scramble cells treated with 3-MA, regardless of the presence of curcumin. Together, these data suggest that interfering with either the autophagic responses or shFOXO1 in endothelial cells can eliminate the cytoprotective effects of curcumin.
Curcumin upregulated RAB7 expression, and the interaction of acetylated FOXO1 and ATG7 during autophagy
Curcumin could increase autophagic flux to prolong cell viability, so we examined the expression of RAB5 and RAB7, both of which belong to the small GTPase protein family, which is required for the final maturation of late autophagic vacuoles and fusion with lysosomes.Citation24,Citation25 There was little change in the expression of RAB5 (), which was also an important promoting factor in autophagic vacuole fusion. However, both the mRNA of RAB7 and protein expression of RAB7 increased significantly during curcumin-induced autophagy (), in HUVECs when either FOXO1 expression or autophagy were inhibited.Citation26 Our results imply that curcumin might mainly promote autophagy by increasing RAB7 expression, but not RAB5.
Figure 7. Curcumin upregulates RAB7 expression during autophagy. (A and B) RAB7 and RAB5 mRNA expression were evaluated by quantitative polymerase chain reaction (qPCR). Relative mRNA expression level is shown. The gene encoding glyceraldehyde-3-phosphate dehydrogenase (GAPDH) was used as the internal control. (A) There was little change of RAB5 mRNA level, but (B) the mRNA of RAB7 increased significantly. (C) Immunoblots and (D) densitometric analyses of RAB7 and RAB5 are shown, which was consistent with the data for RAB7 mRNA above. And there was no change in RAB5 protein level. Values are expressed as the mean ± SD, n = 3; #p < 0.05, ###p < 0.001, shScr with curcumin vs. shScr control (basal line); ***p < 0.001 vs. shScr with curcumin group. (E) Co-immunoprecipitation of ATG7 and the acetylated FOXO1 in HUVECs. Cells were treated as indicated, then the cell lysate was extracted for co-immunoprecipitation with anti-ATG7, followed by probing with anti-acetylated FOXO1. The interaction between ATG7 and acetylated FOXO1 accumulated in the shScr group, but neither in the shFOXO1 groups nor the shScr with 3-MA groups.
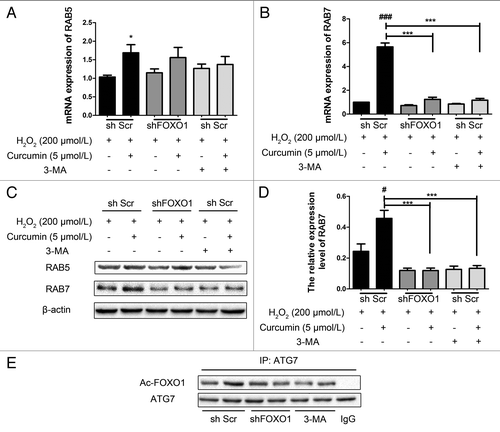
Additionally, with the increase in acetylation of FOXO1, the interaction of acetylated FOXO1 and ATG7 also increased (). This result was consistent with a previous report that promoting the interaction of acetylated FOXO1 and ATG7 could accelerate the process of autophagy ().Citation18
Figure 8. Regulation of curcumin-induced autophagy in HUVECs. During oxidative stress curcumin protects HUVEC survival via autophagy, controlling the following aspects to promote the autophagic process: Curcumin downregulates the PtdIns3K-AKT-mTOR signaling pathway, mediates BECN1-BCL-2 interactions, and interferes with FOXO1 nuclear localization and FOXO1-related proteins.
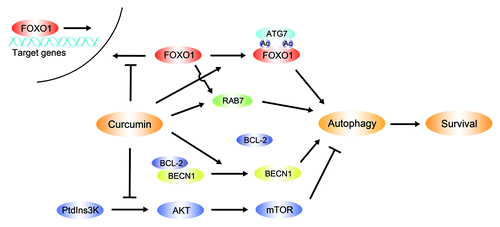
Discussion
Oxidative stress plays a central role in a number of human physiological and pathological processes, including cancer, neurodegenerative diseases, chronic inflammation, ischemic injury, type II diabetes and arteriosclerosis.Citation27-Citation31 Curcumin, a polyphenol with antioxidant and antiinflammatory properties, has beneficial effects on human vascular endothelial cell with its capability of neutralizing reactive oxygen species including superoxide, hydroxyl radical and nitric oxide.Citation3,Citation32,Citation33 In this study, for the first time, we found that curcumin protected vascular endothelial cell survival from oxidative stress by inducing autophagy. As reported, basal levels of autophagy are essential for efficient removal and recycling of damaged cytoplasmic contents, enbodying its significance to cellular homeostasis.Citation34 Our data showed that curcumin can induce a beneficial autophagic process to protect the endothelial cells.
Based on our model, using HUVECs treated with curcumin over 24 h, then stimulated by 200 μmol/L H2O2 for 4 h, we demonstrated that (1) curcumin suppresses the PtdIns3K-AKT-mTOR signaling pathway to promote autophagy; (2) curcumin promotes the dissociation of BECN1 and BCL-2, and the increased free BECN1 in the cytoplasm triggers the autophagic process; and (3) the presence of curcumin also prevents FOXO1 nuclear localization under conditions of oxidative stress, and enhances the level of acetylation of FOXO1. Together, activation of autophagy, deacetylation of FOXO1 and inhibition of FOXO1 nuclear localization are part of an essential adaptive mechanism for HUVECs maintaining viability under conditions of oxidative stress.
It is still a matter of debate as to whether natural products, such as resveratrol, not only suppress cancer cells by inducing apoptosis but also protect normal cells from oxidative stress via an autophagic process.Citation22,Citation35 In the present study, curcumin induced autophagy in HUVECs based not only on morphology, but also on molecular biology. Thus, curcumin plays a role of protector in normal cells.
Regulation of the subcellular localization and transcriptional activity of FOXO proteins is achieved predominantly by post-translational modifications including phosphorylation, acetylation and ubiquitination.Citation15 It is still unknown whether, among the various cellular responses to the FOXO1 transcription factor such as cell cycle arrest, resistance to oxidative stress, apoptosis, or atrophy, there is modulation of a series of specific target genes, or the effects are restricted to specific tissues, cell types or different stresses. Specifically, in neurons and lymphocytes, primarily, the translocation of FOXO1 from the cytoplasm to the nucleus induced by oxidative stress triggers cell death.Citation36-Citation38 But, there is no report about what response FOXO1 will take when curcumin-treated endothelial cells experience oxidative stress.
According to our findings, FOXO1 was localized to the nuclei following treatment with 200 μmol/L H2O2. Moreover, curcumin can partly reverse this process by enhancing the acetylation of the FOXO1 level in the cytoplasm, and can directly prolong cell longevity either by promoting cell survival or by inhibiting apoptosis, or both. It is reported that acetylation by CBP at the positively charged basic residues in the FOXO1 DNA-binding domain can interfere with FOXO1 binding to a target DNA, which prevents FOXO1-mediated transcription. Interestingly, acetylation promotes the phosphorylation of FOXO1 via the PtdIns3K-AKT-mTOR signaling pathway.Citation39 Consistent with our findings, it demonstrates that increasing the acetylation of FOXO1 destabilizes the FOXO1-DNA complex, attenuates transcriptional activity leading to apoptosis, and consequently enhances phosphorylation of FOXO1 by AKT. As to this study, the hypothesis perfectly explains that as acetylation of FOXO1 feeds back to regulate AKT and phosphorylate FOXO1, there was no obvious change in the phosphorylation of FOXO1 (Thr 24, Ser 256, Ser 319), which should have been attenuated by the negative regulation of activated AKT (Ser 473).
In addition, treatment of curcumin maintained SIRT1 at a stable level. Although, as an interesting candidate, SIRT1 plays roles in several cellular processes, such as energy homeostasis, cellular survival, and autophagic degradation,Citation40 the link between autophagy and SIRT1 still remains obscure. Interestingly, unlike other polyphenolic compounds that boost the activity of SIRT1 in vitro,Citation41,Citation42 curcumin in our research maintained SIRT1 at a basal level. In short, the elevation of total acetylated FOXO1 in HUVECs was regulated through various pathways or proteins, directly or indirectly. It is possible that SIRT1 and CBP/p300, an acetyltransferase, co-participated in the regulation of acetylated FOXO1. In other words, the expression of acetylated FOXO1 resulted from the counterbalance of acetyltransferases and deacetylases.
After shFOXO1 was transfected into HUVECs, the protective autophagy induced by curcumin was inhibited when the cells faced oxidative stress, the same result as the group treated with 3-MA. The curcumin-induced autophagy was suppressed by either shFOXO1 or 3-MA, as evidenced by decreased LC3-II, accumulated p62 and increased activity of caspase-3. Furthermore, shFOXO1 enhanced LC3-II, but not LC3-I, which was consistent with what has been suggested before that shFOXO1 inhibits autophagic flux primarily at the level of autophagosome-lysosome fusion.Citation17
What is more, our results show that RAB7, which is required for the initiation of the early-to-late endosome transition, plays an important role in the FOXO1-mediated autophagic process triggered by curcumin. Under the circumstances that HUVECs initiate autophagy, curcumin could also upregulate the expression of RAB7, which contributes to the transport of late endosomes and lysosomes in the autophagic-endocytic pathway.Citation25,Citation43 These findings suggest that curcumin accelerates the endothelial cell autophagic process by enhancing RAB7 expression. In addition, we confirmed that FOXO1 and RAB7 co-mediate the autophagy induced by curcumin, although how they interact is still unknown.
No matter to what extent autophagy is detrimental or beneficial, the main goals for further studies will be to differentiate ‘protective autophagy’ from ‘apoptotic autophagy’ and to point out the key function of FOXO1 in each of them. As FOXO factors are involved in a variety of cellular functions, it is yet to be uncovered whether FOXO1 targets different subsets of genes under different circumstances or in different cell types. These studies enable us to titrate the participation of curcumin-induced HUVEC autophagy and FOXO1-dependent events, especially post-translational modifications, under oxidative stress.
In some types of human normal cells, autophagy acts predominantly as a prosurvival pathway, protecting the cells from oxidative stress; however, in human endothelial cells, the precise molecular mechanisms underlying these processes still remain obscure. This study indicates that curcumin can initiate an autophagic survival response against oxidative stress, and in these processes curcumin affects autophagy-related events, such as: promoting activation of BECN1, inhibiting the PtdIns3K-AKT-mTOR signaling pathway, preventing FOXO1 nuclear localization and mediating FOXO1-induced autophagy. Our findings suggest a novel role for curcumin in inducing a beneficial form of autophagy in HUVECs, which may be a potential multitargeted therapeutic avenue for the treatment of oxidative stress-related cardiovascular diseases.
Materials and Methods
Cell culture and treatment
The investigation conformed to the principles outlined in the Declaration of Helsinki.Citation44 HUVECs were isolated from umbilical cords and cultured according to Jaffe et al.Citation45 Cells were cultured in M199 containing some special supplements as previously described.Citation46 HUVECs were characterized by immunofluorescence staining for von Willebrand factor and by microscopy observation of typical cobblestone morphology. The cells were pretreated with curcumin (1, 5 and 10 μmol/L) supplemented with 5% FBS (Gibco) for 24 h, after which the medium was removed and replaced with medium containing 5% FBS and 200 μmol/L H2O2. After an additional 4 h incubation, cells were assessed as described later.
Cytotoxicity assays
Cell viability was determined by colorimetric assay with CCK-8 (Dojindo, CK04). Briefly, while being cultured on 96-well cell culture plate, cells were pretreated with various concentrations of curcumin for 24 h and followed by treatment with H2O2. To measure cell viability, 10 μL CCK-8 assay solution was added to 100 mL medium per well and incubated for a further 4 h. Then the optical densities of the wells were read on a microplate reader (Molecular Devices). The percentage of living cells was calculated by the ratio of optical density of the experimental wells to that of the normal wells.
Terminal deoxynucleotidyl transferase dUTP nick end labeling (TUNEL) assay
Treated cells were measured using the terminal deoxynucleotidyl transferase dUTP nick end labeling (TUNEL) assay (Roche, 1684795) according to the manufacturer’s protocol. Slides were washed in phosphate-buffered saline (PBS) and counterstained with 1 μmol/L of Hoechst 33258 (Sigma, 861405). The percentages of apoptotic nuclei were calculated by determining the number of Hoechst-stained nuclei that were positive for TUNEL staining, and approximately 100 nuclei were counted in each section. Fluorescence micrographs were taken by means of a fluorescence microscope (Olympus BX-51).
Immunoblot analysis and immunoprecipitation assay
Using the compartmental protein extraction kit (Millipore, 2145), cytoplasmic and nuclear proteins were isolated from HUVECs. Cellular fractionation was done according to the manufacturer’s protocol. Antibodies against AKT (9272), phospho-AKT (4060), LC3 (No. 3868), mTOR (2983), phospho-mTOR (5536), FOXO1 (2880), phospho-FOXO1 (9461) and SIRT1 (2496) were from Cell Signaling Technology. Antibodies against p62 (AP2183b), ATG7 (AJ1065a), RAB7 (AP8665b), and RAB5 (AP6974b) were from Abgent. Acetylated FOXO1 (sc-49473) antibody was from Santa Cruz. 3-methyladenine (3-MA) (M9281) and β-actin antibody (A8481) were from Sigma. Equal amount of protein was subjected to SDS- PAGE on 8–15% polyacrylamide gel and transferred to polyvinylidene difluoride membrane (Millipore, HATF09025). The membrane with blotted protein was blocked for 1 h with blocking buffer containing 5% nonfat dry milk and 0.05% Tween-20 in Tris-buffered saline (TBS-T), followed by incubation with antibodies diluted 1:1,000 in blocking buffer overnight at 4°C. Then, the membrane was washed three times with TBS-T for 30 min and incubated at room temperature for 1 h with diluted (1:2,000) secondary horseradish peroxidase-conjugated goat anti-rabbit IgG (Santa Cruz). Whole-cell lysate proteins were used for immunoprecipitation with the indicated antibodies. 1–3 μg of antibody was added to 1 mL of cell lysate, which was incubated at 4°C overnight. After the addition of Protein A/G-agarose beads, the incubation was continued for 1 h. Immunoprecipitates were extensively washed with lysis buffer and eluted with SDS loading buffer by boiling for 5 min. Detection of the bands was done using the ChemiDoc XRS System (Bio-Rad). The relative intensity of each band was normalized to the band of β-actin respectively.
Construction of shRNA expression vector plasmids
A pSUPER.GFP/neo vector (Oligoengine, VEC-PBS-0006) kindly contributed from Prof. Yu-Xin Yin (Peking University Health Science Center, Beijing, China) was used for construction of short hairpin expression constructs to silence human FOXO1 (shFOXO1). We designed the FOXO1-specific and scrambled siRNA sequences. The sense strand oligo (5′-GATCCCCGCTTAGACTGTGACATGGAATTTCAAGAGAATTCCATGTCACAGTCTAAGCTTTTTA-3′) and antisense strand oligo (5′-TCGAGAAAAAGCTTAGACTGTGACATGGAATTCTCTTGAAATTCCATGTCACAGTCTAAGCGGG-3′) were annealed, and the resulting duplex was inserted into the pSUPER.GFP/neo vector using the BglII and XhoI restriction sites. A control construct (sense- GATCCCCGCTTGTTCGTTGGTAACTACATTCAAGAGATGTAGTTACCAACGAACAAGCTTTTTA; antisense- AGCTTAAAAAGCTTGTTCGTTGGTAACTACATCTCTTGAATGTAGTTACCAACGAACAAGCGGG) that expresses a nontargeting scramble shRNA sequence (shScr) was prepared in a similar manner. Recombinant plasmid constructs were verified by DNA sequencing analysis.
Cell transfection
HUVEC cells were transfected with an mCherry-EGFP-LC3B plasmid which was a gift of Prof. Jayanta Debnath (Addgene plasmid, 22418) at 70% to 80% confluence using Lipofectamine LTX and PLUS Reagents (Invitrogen, 15338-100) according to the manufacturer’s recommendations, which offered transfection efficiency of 20% to 10%. Briefly, Lipofectamine LTX and plasmid DNA were diluted and mixed in Opti-MEM (Invitrogen, 31985-062), the DNA-Lipofectamine LTX- PLUS reagents complexes were applied to the cultures, and incubation continued for 4 h at 37°C, after which the transfection medium was replaced with fresh medium containing 15% (vol/vol) FBS. Thirty-six hours later, cells were ready to be treated.Citation47,Citation48 The same method was used to transfect the shRNA plasmids.
Immunofluorescence and confocal microscopy
Immunofluorescence staining was performed as previously described using antibodies against FOXO1 (1:200 dilution for all antibodies) and DyLight 594 (Thermo Scientific, 35561) anti-rabbit IgG antibody. DAPI was used to visualize the nuclei. Morphological alterations in HUVECs were observed and documented by a fluorescence microscope (Olympus BX-51). Fields were chosen randomly from various sections to ensure objectivity of sampling. Morphometric analyses were performed with the Olympus DP-Soft analysis software. The images of HUVECs transfected with mCherry-EGFP-LC3 plasmid were obtained with a Leica TCS SP5 laser scanning confocal microscope equipped with 40 × objective lens. To quantify the number of autophagic puncta (GFP/mCherry, free mCherry) per cell, confocal microscopy images were binarized to black and white images by Quantity One (Bio-Rad) and then converted to centroids for scoring automatically by the image processing tool kit.
RNA isolation and quantitative PCR
Total RNA was extracted from cultured HUVECs with TRIzol (Invitrogen, 15596-026), and cDNA was synthesized with SuperScript III First-Strand Synthesis System (Invitrogen, 18080-051) following the manufacturer’s instructions. Real time-PCR was performed with THUNDERBIRD SYBR qPCR Mix reagent (TOYOBO, QPS-201) in a real-time PCR system (Stratagene). The following primer pairs were used: RAB7 (sense 5′-GGCCGCGTTTGAAGGATGACCT-3′; antisense 5′-CAGAACGCAGCAGTCTGCACCT-3′); RAB5 (sense 5′-GGCTTGCTGCGGTCTCAGGTTT-3′; antisense 5′-TGCCAACAGCGGACTCTCCCA-3′; GAPDH (sense 5′-TCTTTTGCGTCGCCAGCCGAG-3′; antisense 5′-TCCCGTTCTCAGCCTTGACGGT-3′).
Statistical analysis
Data were presented as means ± SD. Differences between groups were analyzed by one-way ANOVA; means of two groups were compared using Student’s t-test (paired, 2-tailed), with a two-tailed p value < 0.05 considered to indicate statistical significance. The number of experimental samples used in each group is presented in the figure legends.
Abbreviations: | ||
HUVEC | = | human umbilical vein endothelial cell |
GFP | = | green fluorescent protein |
LC3 | = | microtubule-associated protein 1 light chain 3 |
TUNEL | = | terminal eoxynucleotidyl transferase dUTP nick end labeling |
MTOR | = | mechanistic target of rapamycin |
MTORC | = | mechanistic target of rapamycin complex |
shRNA | = | short hairpin RNA |
CBP | = | CREB binding protein |
3-MA | = | 3-methyladenine |
Additional material
Download Zip (738.5 KB)Acknowledgments
This work was supported by the National Natural Science Foundation of China (No. 81020108031, 30572202, 30973558, 30772571, 30901815, 30901803), the Major Specialized Research Fund from the Ministry of Science and Technology in China (No. 2009ZX09103-144) and Research Fund from Ministry of Education of China (No.111 Projects No. B07001).
Disclosure of Potential Conflicts of Interest
No potential conflicts of interest were disclosed.
References
- Aggarwal BB, Sethi G, Baladandayuthapani V, Krishnan S, Shishodia S. Targeting cell signaling pathways for drug discovery: an old lock needs a new key. J Cell Biochem 2007; 102:580 - 92; http://dx.doi.org/10.1002/jcb.21500; PMID: 17668425
- Goel A, Kunnumakkara AB, Aggarwal BB. Curcumin as “Curecumin”: from kitchen to clinic. Biochem Pharmacol 2008; 75:787 - 809; http://dx.doi.org/10.1016/j.bcp.2007.08.016; PMID: 17900536
- Motterlini R, Foresti R, Bassi R, Green CJ. Curcumin, an antioxidant and anti-inflammatory agent, induces heme oxygenase-1 and protects endothelial cells against oxidative stress. Free Radic Biol Med 2000; 28:1303 - 12; http://dx.doi.org/10.1016/S0891-5849(00)00294-X; PMID: 10889462
- Mizushima N, Levine B, Cuervo AM, Klionsky DJ. Autophagy fights disease through cellular self-digestion. Nature 2008; 451:1069 - 75; http://dx.doi.org/10.1038/nature06639; PMID: 18305538
- Rubinsztein DC. The roles of intracellular protein-degradation pathways in neurodegeneration. Nature 2006; 443:780 - 6; http://dx.doi.org/10.1038/nature05291; PMID: 17051204
- Nguyen TM, Subramanian IV, Kelekar A, Ramakrishnan S. Kringle 5 of human plasminogen, an angiogenesis inhibitor, induces both autophagy and apoptotic death in endothelial cells. Blood 2007; 109:4793 - 802; http://dx.doi.org/10.1182/blood-2006-11-059352; PMID: 17272502
- Xie Y, You SJ, Zhang YL, Han Q, Cao YJ, Xu XS, et al. Protective role of autophagy in AGE-induced early injury of human vascular endothelial cells. Mol Med Report 2011; 4:459 - 64; PMID: 21468592
- Ge D, Jing Q, Meng N, Su L, Zhang Y, Zhang S, et al. Regulation of apoptosis and autophagy by sphingosylphosphorylcholine in vascular endothelial cells. J Cell Physiol 2011; 226:2827 - 33; http://dx.doi.org/10.1002/jcp.22632; PMID: 21302284
- Nemoto S, Finkel T. Redox regulation of forkhead proteins through a p66shc-dependent signaling pathway. Science 2002; 295:2450 - 2; http://dx.doi.org/10.1126/science.1069004; PMID: 11884717
- Accili D, Arden KC. FoxOs at the crossroads of cellular metabolism, differentiation, and transformation. Cell 2004; 117:421 - 6; http://dx.doi.org/10.1016/S0092-8674(04)00452-0; PMID: 15137936
- Barthel A, Schmoll D, Unterman TG. FoxO proteins in insulin action and metabolism. Trends Endocrinol Metab 2005; 16:183 - 9; http://dx.doi.org/10.1016/j.tem.2005.03.010; PMID: 15860415
- Greer EL, Brunet A. FOXO transcription factors at the interface between longevity and tumor suppression. Oncogene 2005; 24:7410 - 25; http://dx.doi.org/10.1038/sj.onc.1209086; PMID: 16288288
- Salih DA, Brunet A. FoxO transcription factors in the maintenance of cellular homeostasis during aging. Curr Opin Cell Biol 2008; 20:126 - 36; http://dx.doi.org/10.1016/j.ceb.2008.02.005; PMID: 18394876
- Burgering BM, Kops GJ. Cell cycle and death control: long live Forkheads. Trends Biochem Sci 2002; 27:352 - 60; http://dx.doi.org/10.1016/S0968-0004(02)02113-8; PMID: 12114024
- Vogt PK, Jiang H, Aoki M. Triple layer control: phosphorylation, acetylation and ubiquitination of FOXO proteins. Cell Cycle 2005; 4:908 - 13; http://dx.doi.org/10.4161/cc.4.7.1796; PMID: 15917664
- Sengupta A, Molkentin JD, Paik JH, DePinho RA, Yutzey KE. FoxO transcription factors promote cardiomyocyte survival upon induction of oxidative stress. J Biol Chem 2011; 286:7468 - 78; http://dx.doi.org/10.1074/jbc.M110.179242; PMID: 21159781
- Hariharan N, Maejima Y, Nakae J, Paik J, Depinho RA, Sadoshima J. Deacetylation of FoxO by Sirt1 Plays an Essential Role in Mediating Starvation-Induced Autophagy in Cardiac Myocytes. Circ Res 2010; 107:1470 - 82; http://dx.doi.org/10.1161/CIRCRESAHA.110.227371; PMID: 20947830
- Zhao Y, Yang J, Liao W, Liu X, Zhang H, Wang S, et al. Cytosolic FoxO1 is essential for the induction of autophagy and tumour suppressor activity. Nat Cell Biol 2010; 12:665 - 75; http://dx.doi.org/10.1038/ncb2069; PMID: 20543840
- Kimura S, Noda T, Yoshimori T. Dissection of the autophagosome maturation process by a novel reporter protein, tandem fluorescent-tagged LC3. Autophagy 2007; 3:452 - 60; PMID: 17534139
- Klionsky DJ, Abeliovich H, Agostinis P, Agrawal DK, Aliev G, Askew DS, et al. Guidelines for the use and interpretation of assays for monitoring autophagy in higher eukaryotes. Autophagy 2008; 4:151 - 75; PMID: 18188003
- Rubinsztein DC, Cuervo AM, Ravikumar B, Sarkar S, Korolchuk V, Kaushik S, et al. In search of an “autophagomometer”. Autophagy 2009; 5:585 - 9; http://dx.doi.org/10.4161/auto.5.5.8823; PMID: 19411822
- Pankiv S, Clausen TH, Lamark T, Brech A, Bruun JA, Outzen H, et al. p62/SQSTM1 binds directly to Atg8/LC3 to facilitate degradation of ubiquitinated protein aggregates by autophagy. J Biol Chem 2007; 282:24131 - 45; http://dx.doi.org/10.1074/jbc.M702824200; PMID: 17580304
- Gurusamy N, Lekli I, Mukherjee S, Ray D, Ahsan MK, Gherghiceanu M, et al. Cardioprotection by resveratrol: a novel mechanism via autophagy involving the mTORC2 pathway. Cardiovasc Res 2010; 86:103 - 12; http://dx.doi.org/10.1093/cvr/cvp384; PMID: 19959541
- Pattingre S, Tassa A, Qu X, Garuti R, Liang XH, Mizushima N, et al. Bcl-2 antiapoptotic proteins inhibit Beclin 1-dependent autophagy. Cell 2005; 122:927 - 39; http://dx.doi.org/10.1016/j.cell.2005.07.002; PMID: 16179260
- Jäger S, Bucci C, Tanida I, Ueno T, Kominami E, Saftig P, et al. Role for Rab7 in maturation of late autophagic vacuoles. J Cell Sci 2004; 117:4837 - 48; http://dx.doi.org/10.1242/jcs.01370; PMID: 15340014
- Yu X, Prekeris R, Gould GW. Role of endosomal Rab GTPases in cytokinesis. Eur J Cell Biol 2007; 86:25 - 35; http://dx.doi.org/10.1016/j.ejcb.2006.10.002; PMID: 17157409
- Fouraux MA, Deneka M, Ivan V, van der Heijden A, Raymackers J, van Suylekom D, et al. Rabip4′ is an effector of rab5 and rab4 and regulates transport through early endosomes. Mol Biol Cell 2004; 15:611 - 24; http://dx.doi.org/10.1091/mbc.E03-05-0343; PMID: 14617813
- Jain SK, McVie R, Duett J, Herbst JJ. Erythrocyte membrane lipid peroxidation and glycosylated hemoglobin in diabetes. Diabetes 1989; 38:1539 - 43; http://dx.doi.org/10.2337/diabetes.38.12.1539; PMID: 2583378
- Brownlee M. Biochemistry and molecular cell biology of diabetic complications. Nature 2001; 414:813 - 20; http://dx.doi.org/10.1038/414813a; PMID: 11742414
- Mueller CF, Laude K, McNally JS, Harrison DG. ATVB in focus: redox mechanisms in blood vessels. Arterioscler Thromb Vasc Biol 2005; 25:274 - 8; http://dx.doi.org/10.1161/01.ATV.0000149143.04821.eb; PMID: 15514203
- Vaziri ND, Rodríguez-Iturbe B. Mechanisms of disease: oxidative stress and inflammation in the pathogenesis of hypertension. Nat Clin Pract Nephrol 2006; 2:582 - 93; http://dx.doi.org/10.1038/ncpneph0283; PMID: 17003837
- Shibamoto Y. [Radiation-induced endocrinopathy]. Nihon Rinsho 2006; Suppl 3 575 - 8; PMID: 17022611
- Kowluru RA, Kanwar M. Effects of curcumin on retinal oxidative stress and inflammation in diabetes. Nutr Metab (Lond) 2007; 4:8; http://dx.doi.org/10.1186/1743-7075-4-8; PMID: 17437639
- Sreejayan N, Rao MNA. Nitric oxide scavenging by curcuminoids. J Pharm Pharmacol 1997; 49:105 - 7; http://dx.doi.org/10.1111/j.2042-7158.1997.tb06761.x; PMID: 9120760
- Terman A, Dalen H, Eaton JW, Neuzil J, Brunk UT. Mitochondrial recycling and aging of cardiac myocytes: the role of autophagocytosis. Exp Gerontol 2003; 38:863 - 76; http://dx.doi.org/10.1016/S0531-5565(03)00114-1; PMID: 12915208
- Chen Q, Ganapathy S, Singh KP, Shankar S, Srivastava RK. Resveratrol induces growth arrest and apoptosis through activation of FOXO transcription factors in prostate cancer cells. PLoS One 2010; 5:e15288; http://dx.doi.org/10.1371/journal.pone.0015288; PMID: 21179458
- Brunet A, Bonni A, Zigmond MJ, Lin MZ, Juo P, Hu LS, et al. Akt promotes cell survival by phosphorylating and inhibiting a Forkhead transcription factor. Cell 1999; 96:857 - 68; http://dx.doi.org/10.1016/S0092-8674(00)80595-4; PMID: 10102273
- Zheng WH, Kar S, Quirion R. Insulin-like growth factor-1-induced phosphorylation of the forkhead family transcription factor FKHRL1 is mediated by Akt kinase in PC12 cells. J Biol Chem 2000; 275:39152 - 8; http://dx.doi.org/10.1074/jbc.M002417200; PMID: 10995739
- Dijkers PF, Birkenkamp KU, Lam EW, Thomas NS, Lammers JW, Koenderman L, et al. FKHR-L1 can act as a critical effector of cell death induced by cytokine withdrawal: protein kinase B-enhanced cell survival through maintenance of mitochondrial integrity. J Cell Biol 2002; 156:531 - 42; http://dx.doi.org/10.1083/jcb.200108084; PMID: 11815629
- Matsuzaki H, Daitoku H, Hatta M, Aoyama H, Yoshimochi K, Fukamizu A. Acetylation of Foxo1 alters its DNA-binding ability and sensitivity to phosphorylation. Proc Natl Acad Sci U S A 2005; 102:11278 - 83; http://dx.doi.org/10.1073/pnas.0502738102; PMID: 16076959
- Kiffin R, Kaushik S, Zeng M, Bandyopadhyay U, Zhang C, Massey AC, et al. Altered dynamics of the lysosomal receptor for chaperone-mediated autophagy with age. J Cell Sci 2007; 120:782 - 91; http://dx.doi.org/10.1242/jcs.001073; PMID: 17284523
- Mattson MP, Cheng A. Neurohormetic phytochemicals: Low-dose toxins that induce adaptive neuronal stress responses. Trends Neurosci 2006; 29:632 - 9; http://dx.doi.org/10.1016/j.tins.2006.09.001; PMID: 17000014
- Howitz KT, Bitterman KJ, Cohen HY, Lamming DW, Lavu S, Wood JG, et al. Small molecule activators of sirtuins extend Saccharomyces cerevisiae lifespan. Nature 2003; 425:191 - 6; http://dx.doi.org/10.1038/nature01960; PMID: 12939617
- Vanlandingham PA, Ceresa BP. Rab7 regulates late endocytic trafficking downstream of multivesicular body biogenesis and cargo sequestration. J Biol Chem 2009; 284:12110 - 24; http://dx.doi.org/10.1074/jbc.M809277200; PMID: 19265192
- World Medical Association Declaration of Helsinki. World Medical Association Declaration of Helsinki. Recommendations guiding physicians in biomedical research involving human subjects. Cardiovasc Res 1997; 35:2 - 3; http://dx.doi.org/10.1016/S0008-6363(97)00109-0; PMID: 9302340
- Jaffe EA, Nachman RL, Becker CG, Minick CR. Culture of human endothelial cells derived from umbilical veins. Identification by morphologic and immunologic criteria. J Clin Invest 1973; 52:2745 - 56; http://dx.doi.org/10.1172/JCI107470; PMID: 4355998
- Wu HL, Li YH, Lin YH, Wang R, Li YB, Tie L, et al. Salvianolic acid B protects human endothelial cells from oxidative stress damage: a possible protective role of glucose-regulated protein 78 induction. Cardiovasc Res 2009; 81:148 - 58; http://dx.doi.org/10.1093/cvr/cvn262; PMID: 18815184
- Kojima Y, Ishida T, Sun L, Yasuda T, Toh R, Rikitake Y, et al. Pitavastatin decreases the expression of endothelial lipase both in vitro and in vivo. Cardiovasc Res 2010; 87:385 - 93; http://dx.doi.org/10.1093/cvr/cvp419; PMID: 20045866