Abstract
Selective autophagy, mediated by Atg8 binding proteins, has not been extensively studied in plants. Plants possess a large gene family encoding multiple isoforms of the Atg8 protein. We have recently reported the identification of two new, closely homologous Arabidopsis thaliana plant proteins that bind the Arabidopsis Atg8f protein isoform. These two proteins are specific to plants and have no homologs in nonplant organisms. The expression levels of the genes encoding these proteins are elevated during carbon starvation and also during late stages of seed development. Exposure of young seedlings to carbon starvation induces the production of a newly identified compartment decorated by these Atg8-binding proteins. This compartment dynamically moves along the endoplasmic reticulum membrane and is also finally transported into the vacuole. Enhanced or suppressed expression of these Atg8-binding proteins respectively enhances or suppresses seed germination under suboptimal germination conditions, indicating that they contribute to seed germination vigor.
Keywords: :
Being sessile organisms that cannot escape from environmental stresses, plants apparently use the autophagy machinery quite extensively for the turnover of specific proteins during the course of plant development and upon exposure to various biological perturbations, particularly stresses that cause energy deprivation. Bulk autophagy has been characterized in plants, with knockout mutants in most of the plant genes encoding the core autophagy machinery displaying higher sensitivity than wild-type plants to carbon and nitrogen starvation, and in some cases also possessing accelerated senescence, impaired response to abiotic stresses and pathogen attacks as well as defective fertilization due to impaired pollen germination. Selective autophagy may also be used in plants to pass through various physiological and developmental switches. However, very few cases of selective autophagy have been demonstrated in plants. We, hence, set out to find selective autophagy targets specific to plants.
Selective autophagy mediators are characterized by their ability to bind Atg8. Nine Atg8 homologs exist in the model plant Arabidopsis thaliana and, therefore, we decided to focus on AtAtg8f as a representative. In a yeast two-hybrid assay using AtAtg8f as bait, we discovered two closely homologous plant-specific proteins, termed autophagy-interacting proteins 1 and 2 (ATI1 and ATI2). Both the ATI1 and ATI2 proteins contain two putative Atg8-interacting motifs (termed AIMs) spanned by a predicted transmembrane domain. The presence of an AIM in the ATI's sequences further strengthens the possibility of their interaction with Atg8 and their possible involvement in selective autophagy. In addition, ATI1 and ATI2 expression level is increased during carbon starvation, a common autophagy-inducing stress. These two proteins were also proven to bind the AtAtg8f protein by an in vivo bifluorescence complementation (BiFC) assay.
ATI1 and ATI2 localize to the endoplasmic reticulum (ER) under normal growth conditions (). However, exposure of seedlings to carbon starvation stimulates the association of these proteins with the surface of newly identified bodies (termed ATI1 bodies) that can also be detected in transmission images of wild-type plants (). ATI1 bodies are distinct from Golgi, peroxisomes and mitochondria and display very low colocalization frequency with autophagosomes, suggesting that the interaction between ATI1/2 and AtAtg8f may be a transient one. ATI1 bodies do not seem to contain ER lumen, as demonstrated by the absence of HDEL/KDEL-tagged fluorescent proteins inside them, suggesting that they may be involved in the transport of membrane proteins rather than ER soluble proteins. It is, however, also possible that these bodies may transport nonprotein components from the ER to the vacuole. The ATI1 bodies appear to move along the ER membrane network and to be further transported into the vacuole (), indicating that they apparently mediate the transport of specific ER components into the vacuole.
Figure 1. A schematic summary of the results presented in our work. (A) Under favorable conditions, ATI1/ATI2 are localized to the ER membrane. (B) Upon exposure to stress, the proteins become associated with spherical bodies (ATI1 bodies) that move dynamically on the ER network. (C) ATI1 bodies are transported to the vacuole. A cylinder marks the transmembrane domain of ATI1/ATI2 and the two putative AIMs are marked on both sides of the ER membrane. Abbreviations: ER, endoplasmic reticulum; AIM, Atg8-interacting motif.
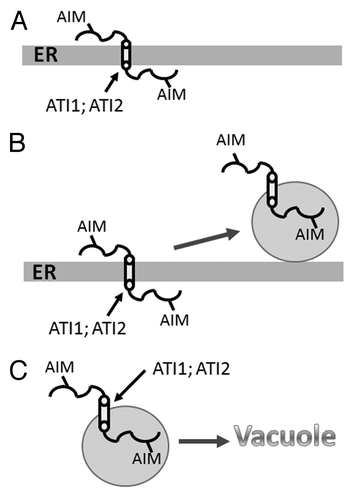
One of the most important developmental switches in plants is the transition of seeds from their maturation stage (when the seeds mature on the mother plant) to the germination stage (when mature seeds become germination competent). During the stage of seed development, the seeds should maintain a “germination-incompetent” status in order to prevent their precocious germination on the mother plant on rainy days or in humid conditions. This is particularly executed by the accumulation of the germination-inhibiting hormone abscisic acid (ABA). After seeds have matured and become ready to germinate, the ABA hormone is turned over and replaced by another, germinating-promoting hormone called gibberellin, whose synthesis is initiated during late seed development and/or early seed germination. The expression of ATI1 and ATI2 in developing and germinating seeds is concomitant with the pattern of ABA accumulation and degradation. Our research showed that overexpression of the ATI1 gene stimulates germination, while the combined suppression of the ATI1 and ATI2 genes inhibits seed germination on medium supplemented with the germination-suppressing hormone ABA. These results indicate that in seeds exposed to conditions that suppress germination, the newly identified compartments defined by ATI1 and ATI2 may act in either: (1) removal of germination-inhibiting ER-associated compounds by their transport for disposal inside the vacuole; and/or (2) transport of germination-promoting components to the vacuole, the compartment where they may apparently function. Such processes may promote seedling establishment and vigor even under harsh environmental conditions.
More work is needed in order to determine the precise role of ATI1/2 in seed development and germination. In addition to the accumulation of ABA, developing seeds also synthesize large amounts of various storage proteins whose degradation during the subsequent stage of seed germination provides the amino acids needed to fuel germination. These massive amounts of storage proteins are generally folded inside the ER and are then transported to the storage vacuole where they assemble to form protein bodies. A number of studies provided evidence supporting the direct transport of the storage proteins from the ER to the vacuole by a process that resembles autophagy, and such a potential autophagy-mediated transport is supported by the fact that genes encoding many proteins of the core autophagy machinery are induced during seed development. Whether ATI1 and ATI2 also participate in the massive transport of the storage proteins to the vacuole is an interesting subject for future research.
Acknowledgments
Our research was supported by grants from The Israel Science Foundation (Grant 764/07), the J & R Center for Scientific Research at The Weizmann Institute of Science, and the Israeli Ministry of Agriculture. G.G. is an incumbent of the Bronfman Chair of Plant Science at The Weizmann Institute of Science.