Abstract
Autophagy is now emerging as a spotlight in trafficking events that activate innate and adaptive immunity. It facilitates innate pathogen detection and antigen presentation, as well as pathogen clearance and lymphocyte homeostasis. In this review, we first summarize new insights into its functions in immunity, which underlie its associations with autoimmunity. As some lines of evidence are emerging to support its role in autoimmune and autoinflammatory diseases, we further discuss whether and how it affects autoimmune diseases including systemic lupus erythematosus, rheumatoid arthritis, diabetes mellitus and multiple sclerosis, as well as autoinflammatory diseases, such as Crohn disease and vitiligo.
Introduction
Autoimmune diseases (AID) arise due to a breach in central and peripheral immune tolerance, amplification of the autoimmune response, and local processes in the target organ that facilitate end-organ disease. This may be restricted to certain organs (e.g., in autoimmune thyroiditis), certain tissues in different places [e.g., antiglomerular basement membrane (GBM) disease which may affect the basement membrane in both the lung and the kidney],Citation1 or may be systemic such as rheumatoid arthritis (RA) and systemic lupus erythematosus (SLE), in which the loss of immune tolerance is systemic and disease manifestations can occur at a variety of different organs or tissues in the body.Citation2 Although the precise mechanisms of autoimmune diseases are unclear, certain pathogenic mechanisms are ultimately triggered during the disease progression, and dysfunctions involving major cell signaling pathways, i.e., the nuclear factor of kappa light polypeptide gene enhancer in B-cells 1 (NFKB1/NFκB) pathway and the inflammatory responses, are consistent features in most AID.Citation3,Citation4
Autophagy is now emerging as a spotlight in trafficking events that activate innate and adaptive immunity.Citation5 As a central player in the immunological control of pathogen clearance, it may also deliver antigens to major histocompatibility complex (MHC) compartments, regulate lymphocyte survival and homeostasis, and mediate cytokine production.Citation6-Citation9 Given the likely importance of autophagy in self-renovation for immunity, here we will review its immune functions and highlight its role in the pathogenesis of AID.
Mechanisms of Autophagy
Autophagy refers to a cellular degradation pathway that involves cytoplasmic content engulfment, delivery and degradation by the lysosome. At least three different forms termed macroautophagy, microautophagy and chaperone-mediated autophagy (CMA) have been identified. Of these forms, macroautophagy has the best-known impact upon human diseases, which will be the main focus of this review. The process of autophagy is as shown in . More details for this process are described extensively elsewhere.Citation10-Citation21
Figure 1. Schematic diagram of autophagy. In macroautophagy, the phagophores engulf the cytoplasmic constituents including organelles, and the resulting autophagosomes fuse with lysosomes, resulting in complete degradation of the sequestered cytoplasmic components by lysosomal hydrolases. In sequence, the cellular events during autophagy follow distinct stages: vesicle nucleation (formation of the phagophore), vesicle elongation and completion (growth and closure), fusion of the double-membrane autophagosome with the lysosome to form an autolysosome, and lysis of the autophagosome inner membrane and breakdown of its contents inside the autolysosome. Microautophagy, on the other hand, happens when lysosomes directly engulf cytoplasm by invaginating, protrusion and/or septation of the lysosomal limiting membrane. In CMA, specific cytosolic proteins are transported into lysosomes via a molecular chaperone/receptor complex composed of HSPA8/HSC70 and LAMP2A (the CMA receptor lysosome-associated membrane protein type-2A).
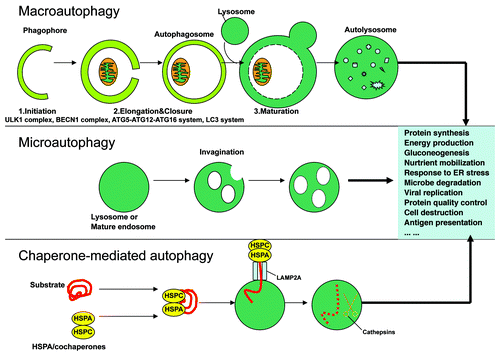
In brief, autophagy is tightly regulated to prevent its unbalanced activation. It can be upregulated in response to extra- or intracellular stress and signals such as starvation, growth factor deprivation, endoplasmic reticulum (ER) stress, accumulation of unfolded proteins and pathogen infection. Autophagosomes can be formed from different membrane sources including the ER,Citation22,Citation23 the Golgi complex,Citation24-Citation26 the mitochondria,Citation27,Citation28 and the plasma membrane.Citation14,Citation19,Citation29,Citation30 To date, at least 35 autophagy-related (ATG) genes have been identified.Citation31 Most of them contribute to autophagosome formation, and are conserved from yeast to human. In mammals, the “core” ATG proteins can be divided into five subgroups: the ATG13-RB1CC1/FIP200-ATG101-ULK1/ULK2 (equivalent of Atg1 in yeast) complex,11PIK3C3/VPS34-BECN1(Atg6) class III PtdIns3-kinase complex (which can be divided into at least three types, the ATG14-PIK3C3-PIK3R4/VPS15-BECN1, UVRAG (UV radiation resistance-associated gene)-PIK3C3-PIK3R4-BECN1 and KIAA0226/RUBICON-UVRAG-PIK3C3-PIK3R4-BECN1 complexes),Citation32-Citation34 ATG9-WIPI1 complex,Citation35-Citation37 ATG12 (ATG12–ATG5-ATG16L1) conjugation system,Citation38 and LC3 (Atg8) conjugation system.Citation12,Citation20,Citation39-Citation42 Each complex contributes to a different function during autophagy. Autophagy is impaired without any of these complexes or core gene products, indicating that a sequential reaction is indispensable for the autophagy process.Citation43 Post-translational modifications such as phosphorylation, ubiquitination and acetylation are thought to be important in regulating autophagy.
Physiological and Pathological Roles of Autophagy
The autophagy induced in response to nutrient deprivation is executed in a nonselective fashion, and its main purpose has long been recognized as a response to adapt to nutrient-poor conditions to maintain energy homeostasis. It preserves cellular bioenergetics by providing metabolic substrates (obtained through bulk cytoplasmic degradation), which maintains macromolecular synthesis and ATP production. Mice with systemic deletion of Atg3,Citation44 Atg5,Citation45 Atg7,Citation46 Atg9Citation47 or Atg16l1,Citation48 die immediately after birth and show reduced amino acid levels during the neonatal starvation period.Citation10,Citation17,Citation49 Although the exact reason for neonatal death in those knockout mice is still controversial, it demonstrates explicitly that amino acid supply through autophagy is important for infant survival. Later tissue-specific gene-targeting studies have revealed that autophagy functions with lineage specification.Citation10,Citation17,Citation49,Citation50
Recent studies, however, have shed light on other indispensable roles for starvation-independent or constitutive autophagy in cellular homeostasis. Autophagy also occurs constitutively at low levels even under nutrient-rich conditions. One of the best-characterized substrates of selective autophagy is SQSTM1/p62 (sequestosome 1). SQSTM1 directly interacts with LC3 on the phagophore through the LC3-interacting region, and then is incorporated into the autophagosome and degraded. When autophagy is defective, accumulation of SQSTM1 will enhance its function as a scaffold protein in several signaling pathways, e.g., NFKB1 signaling.Citation10,Citation51 Inclusion bodies with SQSTM1 can be observed in various neurodegenerative diseases and liver disorders. Other adaptive functions of autophagy are as follows: it works as a cellular housekeeper to eliminate defective proteins and organelles, to prevent abnormal protein aggregate accumulation, and to remove intracellular pathogens; it selectively delivers microbial genetic material and antigens to the innate and adaptive immune systems; it functions as a tumor suppressor in nontumor cells, but it favors tumor progression once tumors are established,Citation52,Citation53 owing to an increased metabolic demand; it also interplays with life/death decisions of the cell by self-cannibalism. Accordingly, it protects humans against diverse pathologies, including obesity, diabetes, neurodegeneration, aging, heart disease, infections, cancer and AID.Citation10,Citation16,Citation17,Citation54
Autophagy and Immunity
It is suggested autophagy has three principal types of contributions to the function of the immune system.Citation55 (1) Type I: Specialized immune processes. For example, by a process called xenophagy,Citation56 pathogens can be captured and eliminated. This will lead to pattern recognition receptors capture, and enhanced MHC presentation of antigens, including auto-antigens in some circumstances.Citation57 (2) Type II: Generic role in cellular homeostasis. This refers to the role of autophagy in controlling cellular homeostasis in all cell types. Intracellular organelles and energy will be normally controlled but with lineage specification as mentioned. For example, T cells are more dependent on autophagy whereas B2 cells are less sensitive. (3) Type III: Isolated ATG functions. This refers to gene-specific function instead of implementation of the entire autophagy pathway. For example, ATG12–ATG5 has been implicated in the inhibition of retinoic acid-inducible gene 1-like receptor (RLR) signaling, whereas ATG9 has been implicated in negatively regulating TBK1.
The many presently recognized roles of autophagy in innate and adaptive immunity have been steadily increasing in complexity (). There are complicated feedback loops between the autophagy pathway/proteins and immunity/inflammation; the autophagy proteins function in both the induction and suppression of immune and inflammatory responses, and, vice versa, immune and inflammatory signals function in both the induction and suppression of autophagy.Citation5,Citation7-Citation9,Citation55,Citation57-Citation62
Figure 2. The multifaceted roles of autophagy in immunity. The many currently recognized roles of autophagy in innate and adaptive immunity have been steadily increasing in complexity. Normal autophagy function contributes to balanced immunity responses, resulting in protection against disease. Imbalanced autophagy results in maladaptive responses and more severe disease. Here “diseasome” stresses more on convergence of different diseases as “every road leads to Rome” in which autophagy may be a common pathway.
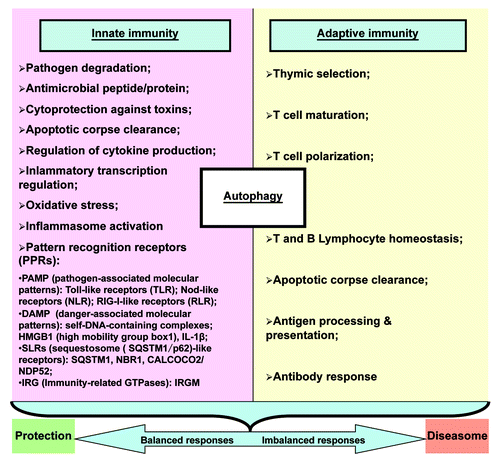
Autophagy as an Innate Immunity Mechanism
In keeping with its primary function as a cytoplasmic scavenger process, autophagy serves as a mechanism for the removal of intracellular pathogens, in a regulated manner. Autophagy proteins have diverse functions to benefit the host in the removal of invading pathogens, through xenophagy, phagolysosomal maturation, the recruitment of molecules that damage pathogen-derived membranes, and, possibly, many other as-yet undiscovered mechanisms.Citation63,Citation64 Beyond targeting intracellular pathogens for degradation, other functions such as the control of downstream inflammatory signaling by an increase in NFKB1-dependent cytokine production, ROS production, production of antimicrobial peptides, cell-cell receptor-ligand interactions,Citation65 and necrotic cell death in autophagy-deficient cellsCitation66 may have further beneficial effects in infected host cells. In signaling, diacylglycerol is beneficial to autophagy induction, whereas cAMP/PKA signaling may have the inverse effect.Citation67,Citation68 In addition, the autophagy pathway has a crucial role in resistance to infection by intracellular pathogens. The genetic deletion, mutation or knockdown of autophagy genes prevents viral, fungal and bacterial infection. For example, in humans, recent studies support a link between autophagy and resistance to tuberculosisCitation69-Citation71 and leprosy.Citation58,Citation72,Citation73
Although autophagy is a component of host defense, various pathogens have evolved several ways to adapt to host autophagy as reviewed elsewhere,Citation9,Citation64,Citation74 and even for their own self-serving purposes, e.g., viral replication.Citation75-Citation77 Bacteria can inhibit lysosomal fusion or maturation by residing in phagosomes, or camouflage themselves to avoid autophagic recognition. Such disguise or escape may shed light on a fundamental aspect of autoimmunity, i.e., discrimination between self and nonself.
Recent studies revealed overlaps between autophagy and innate immune signaling via TBK1 and IκB family of kinases, underscoring the cooperation of pro-inflammatory, immune and physiological signaling pathways in the control of autophagy.Citation57,Citation78-Citation80 Other intersections between autophagy and innate immunity come from studies on pattern recognition receptors, including pathogen-associated molecular patterns: Toll-like receptors, Nod-like receptors, RLRs; DAMPs (damage-associated molecular patterns): self-DNA-containing complexes, HMGB1 (high mobility group box1), IL1B/IL-1β, defensins; SLRs (SQSTM1-like receptors): SQSTM1, NBR1, CALCOCO2/NDP52;Citation57,Citation81immunity-related GTPases: immunity-related GTPase family, M (IRGM);Citation82 and inflammasomesCitation83-Citation85 (). The details of how pattern recognition receptors connect to autophagy have been elegantly reviewed elsewhere.Citation9,Citation57,Citation86 Pattern recognition receptors detect microbial components and induce innate immune responses; however, aberrant activation of immune responses often causes massive inflammation, leading to onset and/or promotion of autoimmune diseases.Citation87-Citation90 Of interest, autophagy often has a dual function. That is, SLRs endow autophagic machinery with the capacity to find its microbial targets in the cytoplasm, whereas they can, via proinflammatory signaling, induce other innate immunity mechanisms of the cell (e.g., promoting NFKB1 induction and CASP8 aggregation, activation and cell death);Citation91 DAMPs such as HMGB1 and IL1B can induce autophagy, whereas induction of autophagy can also promotes secretion of HMGB1 and IL1B.Citation92-Citation94 Such forward loops may explain some of the reasons why inflammation or autoimmunity persist and amplify.
Neutrophil extracellular traps (NETs) composed of DNA, histones and neutrophil antimicrobial peptides can trap and kill various bacterial, fungal and protozoal pathogens, and their release is one of the first lines of defense against pathogens.Citation95 Recent data suggest NETosis, a cell death program also called NET cell death, is associated with the formation of autophagosomes. Autophagy inhibition blocks the intracellular chromatin decondensation, which is necessary for NET formation and NETosis.Citation96,Citation97 NETs have recently been associated with infections, certain autoimmune diseases, such as SLE, anti-neutrophil antibody-associated small vessel vasculitis and autoinflammatory chronic granulomatous disease.Citation98-Citation100 In addition, autophagy may be involved in neutrophil-mediated, antibody-dependent cellular cytotoxicity.Citation101
Autophagy in Antigen Processing and Presentation
The major histocompatibility complex on human chromosome 6 represents one of the most important genetic loci for human autoimmune diseases. Specific alleles that differ from closely related alleles by only one or a few amino acids in the peptide binding groove are frequently strongly associated with disease susceptibility, raising the important question of which peptide presentation events are critical in disease initiation and progression.Citation102-Citation104 Classically, MHC class I molecules present intracellular antigens to CD8+ T cells, and MHC class II molecules present extracellular antigens to CD4+ T cells. However, professional antigen-presenting cells, such as dendritic cells, can process extracellular antigens for MHC class I presentation by a pathway called cross-presentation.Citation105 Vice versa, some nuclear and cytosolic antigens can be presented by MHC class II after intracellular processing.Citation106,Citation107
Autophagy has been implicated both in intracellular and extracellular antigen processing for MHC class II presentation to CD4+ T cells.Citation106 Autophagosomes fuse with lysosome to form autolysosomes, and can also fuse with late endosomes, such as multivesicular bodies, and MHC class II loading compartments, resulting in MHC class II presentation of the autophagic cargo.Citation108 In human B lymphoblastoid cell lines, mass spectrometry analysis of the MHC class II ligandome shows that 20% to 30% of self-class II epitopes are derived from cytosolic and nuclear proteins.Citation106 This self-antigen presentation via autophagy might be involved in autoimmune diseases.Citation109 Recent studies indicate self-derived,Citation110 pathogen-derived,Citation111,Citation112 and tumor antigensCitation113 can be presented by autophagy. Autophagy might assist with extracellular antigen processing through pathogen associated molecular patterns, i.e., toll-like receptorsCitation114 and NOD-like receptors.Citation115
The vast majority of peptides presented on MHC class I molecules are generated by the proteasome in the cytosol. However, some studies also suggest that autophagy might even assist MHC class I antigen presentation to CD8+ T cells.Citation116,Citation117 It was suggested that antigens could be degraded by proteases in the lytic vacuole after autophagy. Peptides generated in the lytic vacuole might return to the cytosol and then enter the classical pathway after proteasome trimming, or alternatively, they could stay in the endovacuolar pathway and be loaded onto MHC class I molecules. This mechanism seems to be induced during viral infection, and requires more time and more antigen than the classical pathway involving the proteasome in the cytosol. Thus, this process might be limited to times of “cellular emergency.”Citation118
Autophagy and T Cells
One major mechanism of tolerance induction is based on the elimination of autoreactive T cells in the thymus. The proposed model for how autophagy influences T cell repertoires accounts for the dual role of thymic epithelial cells, which play a role via endogenous antigen presentation in the MHC II-restricted fashion in both positive and negative selection.Citation119 By transplantation of Atg5−/− thymi into TCR transgenic or athymic recipient mice, positive selection of some but not all CD4+ T cell specificities and for all CD8+ T cell specificities is observed and Atg5 deficiency shapes the T cell repertoire toward multi-organ inflammation. Significantly, organs that appear particularly susceptible to autophagy-dependent autoimmunity show high baseline autophagy, which enhances endogenous MHC II antigen presentation, suggesting that an increase in MHC II-restricted presentation of self antigens has to be matched by autophagy-dependent tolerogenic selection mechanisms in the thymus.
Once T cells depart from the thymus, autophagy plays a role in their survival and homeostasis. A significant decrease in peripheral T and B lymphocyte numbers but with no alterations in the numbers of the myeloid lineage cells has been observed in Atg3-knockout, Atg5-knockout and Atg7-knockout mice.Citation120,Citation121 Defective clearance of mitochondria,Citation122-Citation126 and growth factor withdrawalCitation127 may be plausible explanations. In addition, although autophagic cell death is still a matter of debate, there is a report that T cells showing excessive autophagy undergo cell death with an autophagic component.Citation128
Autophagy and B Cells
Most B cells in peripheral lymphoid tissues are produced in adult bone marrow and are referred to as B-2 cells, which are generally considered to be mediators of adaptive immunity. A minor B cell subset called B-1 cells is part of the innate immune system.Citation129,Citation130 It has been suggested that B-1a, B-1b and B-2 B cells may develop from distinct cell lineages.Citation131 A study using B cell-specific deletion of Atg5 in mice has shown that autophagy plays a role in B cell development and homeostasis.Citation132 The data indicate that ATG5 is required for the maintenance of B-1a B cells, which normally have self-renewal capacity, but not B-1b or B-2 B cells, although sufficient pre-B cells survive to populate the periphery. The reason might be due to a role for ATG5 in cytokine-driven differentiation or cell survival after growth factor withdrawal.
A few studies also suggest that autoantibodies can stimulate autophagy. For example, sera from type 2 diabetic patients with neuropathy or a subpopulation of patients with neurogenic chronic intestinal pseudo-obstruction,Citation133-Citation137 are associated with increased levels of autophagosomes involving the FAS receptor complex. Similar autophagy activation was also observed in SH-SY5Y neurons treated by sera from active lupus patients.Citation133 These data might allow the hypothesis that autoimmune diseases correlate with unbalanced autophagy. But, to date, no clear link and precise mechanisms have been resolved with regard to autoantibodies from classical autoimmune disease in mediating autophagy.
Autophagy and Cytokines
Autophagy is regulated by immunologically relevant cytokines and cell surface receptors, with activation by IFNG/IFNγ, TNF/TNFα, NFKB, monocyte chemoattractant protein 1(CCL2),Citation138 Fcγ receptors, CD46, CD40 and inhibition by IL4, IL13, CLCF1, LIF, insulin-like growth factor-1 (IGF1), FGF2, CXCL12/SDF1, NFKB, STAT3 and BCL2.Citation9 As IFNG, a major Th1 cytokine, induces autophagy, whereas the Th2 cytokines IL4 and IL13 inhibit autophagy, it may demonstrate that autophagy is an effector of Th1/Th2 polarization, explaining why Th1 cytokines are protective and Th2 cytokines permissive when it comes to controlling intracellular pathogens.Citation61 Lines of evidence supporting the role of inflammatory cytokines in the regulation of autophagy may even indicate that the pro-inflammatory cytokine patterns usually observed in chronic autoimmune disorders ultimately disrupt the balance of autophagy in the affected organ.Citation62 Also, autophagy has positive contributions to the biogenesis and secretion of the proinflammatory cytokines such as IL1B,Citation92 IL18, adipocytokines,Citation139,Citation140 type I IFN,Citation141 TNF,Citation142 IL7Rα chain,Citation143etc., which may be a possible explanation for the vicious pathogenic cytokine milieu often observed in infectious and autoimmune diseases.
Autophagy in Autoimmune and Autoinflammatory Diseases
The multitiered connections between autophagy and immunity uncovered to date strongly supports the idea that autophagy may exert both beneficial and aggravating effects on immunity and inflammation. Such a switch between the dual functions of autophagy in disease has become a priority when considering disease pathogenesis, and further its potential therapeutic implications. Of utmost importance, an imbalanced function of autophagy has recently been linked to the causation and prevention of autoimmune and/or autoinflammatory diseases, which strongly supports a role of autophagy at the heart of immunity rather than as a bystander.
Example of an autoinflammatory disorder: Crohn disease
Crohn disease (CD) has widely been described as an autoimmune disease, and a newer view describes it as a disease of immune deficiency.Citation144,Citation145 This suggests an autoinflammatory rather than autoimmune origin of inflammation in Crohn disease.Citation146 Autoinflammatory disease is a brand new category of diseases that have several common characteristics of autoimmunity without involving autoantigen, marked by abnormally increased inflammation, mediated predominantly by the cells and molecules of the innate immune system, with a significant host predisposition. Both autoinflammatory diseases and autoimmune diseases result in flares of inflammation.Citation147,Citation148 A combination of environmental factors and genetic predisposition is believed to cause CD. And plenty of genetic risk factors have been discovered.Citation149-Citation151 Malfunctions in the innate immune system and Th17 system have been addressed. However, the discovery of the autophagy genes ATG16L1 and IRGM as risk factors for CD put autophagy in the spotlight in its pathogenesis.Citation152-Citation169
A single-nucleotide polymorphism (SNP) of ATG16L1 (rs2241880, threonine300 is replaced by alanine, T300A) has been associated with CD with evidence from several northern Caucasian CD cohorts.Citation150,Citation151,Citation166,Citation167 However, its role in the pathogenesis of CD disease is not simple; the association was not observed in Asian populations.Citation170 For specific CD subphenotypes, it appears to be associated only with disease of the small bowel.Citation171 So far, very little information is currently available about the functions of the human ATG16L1 protein, and even less about the consequences of its variations. Threonine 300 is located in the C-terminal WD repeat domain in ATG16L1, suggesting T300A would modify Atg16L1 conformation and interaction and be sufficient for conventional autophagy. However, T300A variants are fully competent in the formation of autophagosomes in both constitutive and starvation-induced conditions in ATG16L1−/−fibroblasts.172One study suggested that the ATG16L1T300A mutant is defective in autophagic sequestration of intracellular bacteria but not in canonical autophagy.Citation172 Another study, however, suggested that this mutant has intact activity in both canonical autophagy and antibacterial autophagy.Citation173 This subtle consequence of the T300A variation might be critically dependent on the cell system examined: human gut epithelial Caco2 cellsCitation172 vs. murine fibroblasts.Citation173 ATG16L1-deficient mice die within the first day of delivery, however, ATG16L1 hypomorphic mice, which express a very low level of Atg16L1, are viable and grossly normal but exhibit structurally abnormal Paneth cells with cytoplasmic lysozyme staining. These data suggest ATG16L1 has a role in maintaining the normal function of Paneth cells.Citation139,Citation174 ATG16L1-deficient Paneth cells exhibit not only defective granule exocytosis but also an unexpected gain of function including increased expression of genes involved in peroxisome proliferator-activated receptor signaling and lipid metabolism, of acute phase reactants and of two adipocytokines, leptin and adiponectin, known to directly influence intestinal injury responses. Similarly, CD patients homozygous for the ATG16L1 CD risk alleles display comparable Paneth cell granule defects and increased leptin levels.Citation139 Further study suggests that CD can be determined by a virus-plus-susceptibility gene interaction in combination with additional environmental factors and commensal bacteria, showing a possible reason why the disease occurs in only a small proportion of persons carrying common risk alleles of disease susceptibility genes.Citation174 In addition to these cell-intrinsic mechanisms, a link between autophagy and the immune system can also contribute to the pathogenesis of CD. This link occurs through a direct interaction between ATG16L1 and NOD2/CARD15 (which has the strongest association with Crohn disease) in the bacterial sensing pathway,Citation175,Citation176 the regulation of inflammatory cytokine productionCitation48,Citation177 and antigen presentation.Citation115
Mice that are null for Irgm1 develop normally, have no apparent phenotype and, yet, they are extremely susceptible to bacterial infection, all dying at 11–16 weeks postinfection. Previously, two polymorphisms of IRGM have been highly correlated with CD risk: a tag-SNP variation within the coding region (rs10065172, C313T);Citation178 and a 20-kb deletion upstream of the IRGM gene.Citation179 They are in perfect linkage disequilibrium (r2 = 1.0). Follow-up genetic studies of IRGM have revealed unexpected complexity. Resequencing of the IRGM open reading frames did not reveal any disease-associated coding variants, suggesting that the causal variants are regulatory rather than structural.Citation179,Citation180 A recent study shows rs10065172 alters a binding site for MIR196, which is strongly increased in colonic epithelial cells of individuals with CD compared with controls.Citation181 Downregulation of IRGM seen in colonic epithelial cells of individuals homozygous for the protective allele (rs10065172 C) is mediated by MIR196, but that is not seen in carriers of the risk variant (rs10065172 T) because the T allele disrupts the target site. Hypothetically, increased IRGM expression in colonic mucosa should be protective. However, IRGM overexpression actually reduces the efficacy of xenophagy, as an increase in the number of surviving intracellular bacteria is observed. Effective xenophagy may thus require optimal IRGM expression levels, both overexpression and underexpression being potentially harmful.Citation182 Alternatively, overexpression of specific IRGM isoforms induces cell death,Citation82 which could be the main inflammatory trigger. It is possible that the rs10065172 T allele contributes to the maintenance (rather than initiation) of chronic inflammation in the gut by disrupting a MIR196-dependent autoregulatory loop controlling IRGM expression, thereby promoting epithelial apoptosis.Citation183 Further genetic evidence, combined with functional data, is needed, as the association can also be observed with susceptibility to tuberculosis in African Americans,Citation184 but cannot be detected in Asian CD patients,Citation180 which may reflect gene-by-environment or gene-by-gene interactions.
Along these lines, it would be critical to explore further genetic associations given that autophagy is a pathway, and interactions between the environment and the autophagic risk factors. A recent study thus suggests an association between a tSNP (rs12303764) in ULK1 and risk of CD.Citation163 However, no confirmed epistasis has been derived to date, in spite of a weak interaction with respect to ATG16L1 and NOD2 susceptibility variants.Citation167 In addition, the lack of association of these variants with CD in the Asian populations is intriguing.
Example of an autoimmune disease: Systemic lupus erythematosus
The pathogenic mechanisms of SLE—a prototypic systemic autoimmune disease— are incompletely understood, but are postulated to involve multiple diverse aspects in the dysregulated immune response system. Recent genome-wide association studies have improved our understanding of the genetic basis of SLE, especially since they have identified and robustly replicated several novel loci, which implicate newer layers of immunological disturbances or pathways involved in disease pathogenesis. Among the many convincingly established genetic associations, genetic variants within or near ATG5—a key autophagy gene required for the formation of autophagosomes—have been associated with SLE both in Caucasian and Chinese populations,Citation185-Citation188 which suggested that autophagy may also be involved in SLE pathogenesis.
However, both the genetic data and the pathogenesis mechanisms are insufficient. The PRDM1 (PR domain containing 1, with ZNF domain, also known as BLIMP1) -ATG5 gene region is in low linkage disequilibrium and associated patterns are diversified between Caucasians and Chinese. Thus, it seems that multiple independent signals are associated with SLE, including rs573775 (ATG5 intron 1),Citation185 rs2245214 (ATG5 intron 6)Citation186 and intergenic region of PRDM1-ATG5 (rs548234, rs6937876 and rs6568431).Citation185-Citation188 Although replication studies confirmed the association of ATG5 gene variants with SLE from Caucasians,Citation185,Citation186 the consistent association signal in both Caucasians and Chinese is from the intergenic region of PRDM1-ATG5,Citation188 which may obscure the role of ATG5 in SLE. In spite of this, eQTL analysis both in immortalized lymphoblasts and in lymphocytes from Chinese lupus patients as well as healthy controls, supported the idea that polymorphisms within the intergenic region of PRDM1-ATG5 may be through upregulating ATG5 gene expression, giving clues that ATG5 is a candidate gene of SLE.Citation188 Genotypes of intergenic gene polymorphisms correlated with ATG5 (cis-QTL effect) and its downstream gene expressions (trans-QTL effect), including many autophagy genes, type I IFN-related genes, apoptosis-related genes and NFKB-related genes, suggesting ATG5 has a multifaceted function in lupus pathogenesis ().Citation188 Anyhow, the genetic mechanism by which intergenic gene polymorphisms affects gene expression is lacking and a further fine mapping of ATG5 is urgent. From the viewpoint that autophagy is a pathway, the study also suggests that ATG7 and IRGM are susceptibility genes to SLE, and positive gene-gene interactions occur among ATG5, ATG7 and IRGM.Citation188 The association between IRGM gene polymorphisms and SLE can be also derived from a meta-analysis conducted between SLE and 16 autoimmune diseases, which aims to detect shared and disease-specific genetic loci.Citation189 All the above strongly indicate that autophagy is involved in SLE pathogenesis. The upregulation of ATG5 is consistent with the observations that complement-inactivated sera from patients of SLE can significantly activate autophagyCitation133 and that ATG5 expression elevates in autoimmune demyelination and multiple sclerosis (MS).Citation190
ATG5 may participate in the pathogenesis of SLE at multiple levels ().Citation191 It may be involved in pathogen clearance, ultraviolet (UV) irradiation resistance, and many other environmental trigger protections. For example, Atg5−/− fibroblasts are resistant to death from UV light.Citation192 This protein may be involved in the activation of innate immunity by delivering viral nucleic acids to endosomal compartments containing toll-like receptor 7, which signals the induction of type 1 IFN production—thus playing a central role in SLE pathogenesis.Citation193-Citation195 Plasmacytoid dendritic cells from Atg5 knockout mice produce less IFNA/IFNα in response to viral infections, which may underscore a role for viral and bacterial triggers in SLE.Citation141 Many other PPRs like HMGB1,Citation196 and DEFENSIN,Citation197 whose expression are higher in lupus patients, can activate autophagy as well.Citation93 The lack of efficient handling of apoptosis, secondary necrosis, and exposure of chromatin fragments may overcome tolerance to self-antigens and lead to SLE. Mice with dendritic cell-conditional deletion in Atg5, show a pronounced defect of processing and presentation of phagocytosed antigens containing toll-like receptor stimuli for MHC class II.Citation112 A more recent study directly investigating autophagy in T lymphocytes from both lupus-prone mouse models and human lupus patients by LC3 conversion assays and electron microscopy experiments indicated autophagy increased in lupus compared with that in normal controls and nonlupus autoimmune diseases. The data further support the idea that autophagy is genetically deregulated in lupus, promoting survival of autoimmune T cells.Citation198 Persistence of autoreactive T and B cell clones and their imbalanced crosstalk can also be interfered with by ATG5.Citation124,Citation132,Citation191,Citation199-Citation201 Excessive inflammation and tissue damage may further be sustained by production of inflammatory cytokines,Citation202 the recruitment of immune cells,Citation203-Citation205 and enhanced oxidative stress.Citation206,Citation207 In addition, nonimmune mechanisms may also contribute to end-stage organ damage.Citation208 For example, podocyte-specific deletion of Atg5 leads to a glomerulopathy, whereas human renal biopsies of patients with acquired proteinuric diseases often show elevated ATG3 expression, suggesting autophagy as a key homeostatic mechanism to maintain glomerular injury.Citation209 As lupus nephritis is a common characteristic of SLE, further organ or tissue intrinsic mechanisms involving autophagy are also needed.
Figure 3. The possible role of ATG5 in systemic lupus erythematosus (SLE). An overview of the many possible mechanisms by which ATG5 gene polymorphisms may contribute to the pathogenesis of a type of autoimmune disease.
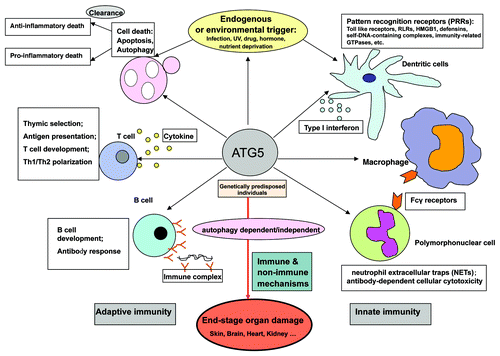
Of note, as discussed above, ATG5 may have its own autophagy-independent functions/Type III functions. It is suggested that autophagy-independent functions include: being a pro-apoptotic molecule translocating to mitochondria to trigger mitochondrial outer membrane permeabilization;Citation210,Citation211 to function as a suppressor of innate immune signaling in the form of the ATG12–ATG5 conjugate by interactions with the caspase recruitment domain of retinoic acid-inducible gene-1 (DDX58/RIG1) and interferon-β promoter stimulator (MAVS/IPS-1), contributing to RNA virus replication,Citation212 but to mediate intracellular killing of pathogens via autophagosome-independent processes such as GTPase trafficking.Citation213 In spite of its possibly specific function in such cellular processes, it would be cautious to assume that its association with SLE is due to its nonautophagy characteristics, as current understanding of the autophagy network is incomplete. Also, the above “nonautophagy” functions relate more with innate immunity rather than perturbations of adaptive immunity, which nevertheless laid the foundation of autoimmunity. In any case, systemic evaluations of ATG5 in SLE will be necessary and critical.
In addition, recent genetic studies also associate allelic variants of the HSPA/HSP70 genes with SLE in Caucasians, and correlate with the presence of autoantibodies to CALR/Ro and SSB/La.Citation214 This finding may indicate that CMA is also defective in SLE patients.Citation215,Citation216
Other autoimmune/autoinflammatory diseases
A few pieces of evidence are emerging to support the role of autophagy in other autoimmune disease, such as rheumatoid arthritis, diabetes mellitus, MS and other autoinflammatory diseases such as vitiligo.
Anti-citrullinated protein antibodies, which are the most specific autoantibody indicators in patients with RA, are present before clinical onset, which may indicate that the initial loss of immune tolerance to citrullinated proteins occurs as a consequence of an inflammatory event outside the joint. A recent study suggests the presentation of the citrullinated peptides but not of the unmodified peptides was associated with autophagy. The presentation was reduced by 3-methyladenine or by reduction in ATG5 expression.Citation110 Thus, ATG5 may also be involved in the pathogenesis of RA. Of interest, a recent large-scale replication study on RA also associated disease susceptibility with the PRDM1-ATG5 intergenic region, and this may further suggest a common mechanism of ATG5 in autoimmunity.Citation217 However, such genetic association is lacking in Asian populations, either with disease susceptibility or anticyclic citrullinated peptides antibody.Citation217
The majority of type 1 diabetes is an inflammatory autoimmune disease of the pancreas, resulting in a lack of insulin. Type 2 diabetes mellitus is characterized by insulin resistance and failure of pancreatic β-cells producing insulin. Deficiency of insulin by β-cells, or the insensitivity of its receptors plays a central role in all forms of diabetes mellitus. Recent studies strongly suggest that basal autophagy is important for maintenance of β-cell volume and function.Citation218,Citation219 Impaired autophagy has been linked with diabetes and acute pancreatitis.Citation219-Citation221 This process is considered to be an adaptive response, i.e., autophagy induction contributes to the beneficial metabolic effects of exercise.Citation222 However, a study also indicates autophagy can be stimulated by complement-inactivated sera from type 2 diabetic patients with neuropathy, which may suggest an autoantibody-mediated autophagy pathway in the pathogenesis of demise of neuronal tissue in type 2 diabetes.
Multiple sclerosis is currently believed to be an autoimmune-mediated disorder characterized by damage of myelin and axons by focal lymphocytic infiltration.Citation223 In MS, prolonged T cell survival and increased T cell proliferation have been linked to disease relapse and progression. In blood samples of experimental autoimmune encephalomyelitis mice—a widely used model of MS—elevated expression of ATG5 and IRGM1 have been observed.Citation190,Citation224 ATG5 is also higher in T cells as well as brain samples from active relapsing-remitting MS patients than that in nondiseased controls. IRGM may affect the survival of mature effector CD4+ T cells by protecting them from IFNG-inflicted apoptotic cell death, thus delaying a homeostatic mechanism that otherwise limits the duration and strength of the cell-mediated immunity response.Citation55,Citation224,Citation225 Thus, increased T cell expression of ATG5 and IRGM1 may contribute to inflammatory demyelination in MS.
As discussed above, UVRAG activates the BECN1-PIK3C3 complex, promoting autophagy. A small nonsegmental vitiligo cohort study from Korea suggests a possible association between UVRAG (rs1458836, rs7933235) and disease susceptibility, expanding the number of current disease associated autophagy genes.Citation226 As UV is strongly associated with SLE disease occurrence, association between UVRAG gene polymorphisms and SLE susceptibility will be of interest.
Conclusion
Recent studies highlight the role of autophagy in positively shaping the landscapes of innate and adaptive immunity. One key issue is to understand whether and how it affects immunity-related diseases. Plenty of evidence is emerging to support its role in autoimmune and autoinflammatory diseases. The most telling lines of evidence are from an unbiased method, by genome-wide association studies in human populations. Key findings include associations between polymorphisms in ATG16L1 and IRGM with autoinflammatory Crohn disease, and polymorphisms in/near ATG5 with autoimmune SLE. Clues are also surfacing to link autophagy with other autoimmune diseases, such as RA, diabetes mellitus, MS and other autoinflammatory diseases such as vitiligo. These findings will bolster the significance of the immunological role of autophagy in human disease, but also will broaden our vision and develop a scientific research foundation for the pathogenesis of autoimmune and autoinflammatory diseases.
Abbreviations: | ||
AID | = | autoimmune diseases |
ATG16L1 | = | autophagy-related gene 16-like 1 |
CD | = | Crohn disease |
CMA | = | chaperone-mediated autophagy |
DAMPs | = | damage-associated molecular patterns |
ER | = | endoplasmic reticulum |
HMGB1 | = | high mobility group box 1 |
IGF1 | = | insulin-like growth factor-1 |
IRGM | = | immunity-related GTPase family M |
MHC | = | major histocompatibility complex |
MS | = | multiple sclerosis |
NETs | = | neutrophil extracellular traps |
NFKB1 | = | nuclear factor of kappa light polypeptide gene enhancer in B-cells 1 |
RA | = | rheumatoid arthritis |
RLR | = | retinoic acid-inducible gene 1-like receptor |
SLE | = | systemic lupus erythematosus |
SNP | = | single-nucleotide polymorphism |
TNF | = | tumor necrosis factor |
UV | = | ultraviolet |
Acknowledgments
This work was supported by grants from the Major State Basic Research Development Program of China (973 program, No.2012CB517700), National Natural Science Foundation of China (No. 30825021) and Natural Science Fund of China to the Innovation Research Group (81021004). We apologize to those authors whose publications could not be quoted in this review due to space limitations.
References
- Zhou XJ, Lv JC, Zhao MH, Zhang H. Advances in the genetics of anti-glomerular basement membrane disease. Am J Nephrol 2010; 32:482 - 90; http://dx.doi.org/10.1159/000321324; PMID: 20962523
- McGonagle D, McDermott MF. A proposed classification of the immunological diseases. PLoS Med 2006; 3:e297; http://dx.doi.org/10.1371/journal.pmed.0030297; PMID: 16942393
- Gerondakis S, Siebenlist U. Roles of the NF-kappaB pathway in lymphocyte development and function. Cold Spring Harb Perspect Biol 2010; 2:a000182; http://dx.doi.org/10.1101/cshperspect.a000182; PMID: 20452952
- Brown KD, Claudio E, Siebenlist U. The roles of the classical and alternative nuclear factor-kappaB pathways: potential implications for autoimmunity and rheumatoid arthritis. Arthritis Res Ther 2008; 10:212; http://dx.doi.org/10.1186/ar2457; PMID: 18771589
- Münz C. Enhancing immunity through autophagy. Annu Rev Immunol 2009; 27:423 - 49; http://dx.doi.org/10.1146/annurev.immunol.021908.132537; PMID: 19105657
- Levine B, Deretic V. Unveiling the roles of autophagy in innate and adaptive immunity. Nat Rev Immunol 2007; 7:767 - 77; http://dx.doi.org/10.1038/nri2161; PMID: 17767194
- Virgin HW, Levine B. Autophagy genes in immunity. Nat Immunol 2009; 10:461 - 70; http://dx.doi.org/10.1038/ni.1726; PMID: 19381141
- Deretic V. Autophagy in innate and adaptive immunity. Trends Immunol 2005; 26:523 - 8; http://dx.doi.org/10.1016/j.it.2005.08.003; PMID: 16099218
- Levine B, Mizushima N, Virgin HW. Autophagy in immunity and inflammation. Nature 2011; 469:323 - 35; http://dx.doi.org/10.1038/nature09782; PMID: 21248839
- Mizushima N, Komatsu M. Autophagy: renovation of cells and tissues. Cell 2011; 147:728 - 41; http://dx.doi.org/10.1016/j.cell.2011.10.026; PMID: 22078875
- Mizushima N. The role of the Atg1/ULK1 complex in autophagy regulation. Curr Opin Cell Biol 2010; 22:132 - 9; http://dx.doi.org/10.1016/j.ceb.2009.12.004; PMID: 20056399
- Chen Y, Klionsky DJ. The regulation of autophagy - unanswered questions. J Cell Sci 2011; 124:161 - 70; http://dx.doi.org/10.1242/jcs.064576; PMID: 21187343
- Mizushima N. Autophagy: process and function. Genes Dev 2007; 21:2861 - 73; http://dx.doi.org/10.1101/gad.1599207; PMID: 18006683
- Hamasaki M, Yoshimori T. Where do they come from? Insights into autophagosome formation. FEBS Lett 2010; 584:1296 - 301; http://dx.doi.org/10.1016/j.febslet.2010.02.061; PMID: 20188731
- He C, Klionsky DJ. Regulation mechanisms and signaling pathways of autophagy. Annu Rev Genet 2009; 43:67 - 93; http://dx.doi.org/10.1146/annurev-genet-102808-114910; PMID: 19653858
- Kundu M, Thompson CB. Autophagy: basic principles and relevance to disease. Annu Rev Pathol 2008; 3:427 - 55; http://dx.doi.org/10.1146/annurev.pathmechdis.2.010506.091842; PMID: 18039129
- Levine B, Kroemer G. Autophagy in the pathogenesis of disease. Cell 2008; 132:27 - 42; http://dx.doi.org/10.1016/j.cell.2007.12.018; PMID: 18191218
- Meijer AJ, Codogno P. Autophagy: regulation by energy sensing. Curr Biol 2011; 21:R227 - 9; http://dx.doi.org/10.1016/j.cub.2011.02.007; PMID: 21419990
- Rubinsztein DC, Shpilka T, Elazar Z. Mechanisms of autophagosome biogenesis. Curr Biol 2012; 22:R29 - 34; http://dx.doi.org/10.1016/j.cub.2011.11.034; PMID: 22240478
- Tanida I. Autophagy basics. Microbiol Immunol 2011; 55:1 - 11; http://dx.doi.org/10.1111/j.1348-0421.2010.00271.x; PMID: 21175768
- Weidberg H, Shvets E, Elazar Z. Biogenesis and cargo selectivity of autophagosomes. Annu Rev Biochem 2011; 80:125 - 56; http://dx.doi.org/10.1146/annurev-biochem-052709-094552; PMID: 21548784
- Mizushima N, Yoshimori T, Ohsumi Y. The role of Atg proteins in autophagosome formation. Annu Rev Cell Dev Biol 2011; 27:107 - 32; http://dx.doi.org/10.1146/annurev-cellbio-092910-154005; PMID: 21801009
- Tooze SA, Yoshimori T. The origin of the autophagosomal membrane. Nat Cell Biol 2010; 12:831 - 5; http://dx.doi.org/10.1038/ncb0910-831; PMID: 20811355
- van der Vaart A, Griffith J, Reggiori F. Exit from the Golgi is required for the expansion of the autophagosomal phagophore in yeast Saccharomyces cerevisiae. Mol Biol Cell 2010; 21:2270 - 84; http://dx.doi.org/10.1091/mbc.E09-04-0345; PMID: 20444982
- Geng J, Klionsky DJ. The Golgi as a potential membrane source for autophagy. Autophagy 2010; 6:950 - 1; http://dx.doi.org/10.4161/auto.6.7.13009; PMID: 20729630
- van der Vaart A, Reggiori F. The Golgi complex as a source for yeast autophagosomal membranes. Autophagy 2010; 6:800 - 1; http://dx.doi.org/10.4161/auto.6.6.12575; PMID: 20714226
- Hailey DW, Rambold AS, Satpute-Krishnan P, Mitra K, Sougrat R, Kim PK, et al. Mitochondria supply membranes for autophagosome biogenesis during starvation. Cell 2010; 141:656 - 67; http://dx.doi.org/10.1016/j.cell.2010.04.009; PMID: 20478256
- Luo S, Chen Q, Cebollero E, Xing D. Mitochondria: one of the origins for autophagosomal membranes?. Mitochondrion 2009; 9:227 - 31; http://dx.doi.org/10.1016/j.mito.2009.04.004; PMID: 19398041
- Militello RD, Colombo MI. A membrane is born: origin of the autophagosomal compartment. Curr Mol Med 2011; 11:197 - 203; http://dx.doi.org/10.2174/156652411795243441; PMID: 21375493
- Ravikumar B, Moreau K, Jahreiss L, Puri C, Rubinsztein DC. Plasma membrane contributes to the formation of pre-autophagosomal structures. Nat Cell Biol 2010; 12:747 - 57; http://dx.doi.org/10.1038/ncb2078; PMID: 20639872
- Homma K, Suzuki K, Sugawara H. The Autophagy Database: an all-inclusive information resource on autophagy that provides nourishment for research. Nucleic Acids Res 2011; 39:Database issue D986 - 90; http://dx.doi.org/10.1093/nar/gkq995; PMID: 20972215
- Liang XH, Jackson S, Seaman M, Brown K, Kempkes B, Hibshoosh H, et al. Induction of autophagy and inhibition of tumorigenesis by beclin 1.. Nature 1999; 402:672 - 6; http://dx.doi.org/10.1038/45257; PMID: 10604474
- Pattingre S, Tassa A, Qu X, Garuti R, Liang XH, Mizushima N, et al. Bcl-2 antiapoptotic proteins inhibit Beclin 1-dependent autophagy. Cell 2005; 122:927 - 39; http://dx.doi.org/10.1016/j.cell.2005.07.002; PMID: 16179260
- Ciechomska IA, Goemans GC, Skepper JN, Tolkovsky AM. Bcl-2 complexed with Beclin-1 maintains full anti-apoptotic function. Oncogene 2009; 28:2128 - 41; http://dx.doi.org/10.1038/onc.2009.60; PMID: 19347031
- Young ARJ, Chan EYW, Hu XW, Köchl R, Crawshaw SG, High S, et al. Starvation and ULK1-dependent cycling of mammalian Atg9 between the TGN and endosomes. J Cell Sci 2006; 119:3888 - 900; http://dx.doi.org/10.1242/jcs.03172; PMID: 16940348
- Webber JL, Tooze SA. New insights into the function of Atg9. FEBS Lett 2010; 584:1319 - 26; http://dx.doi.org/10.1016/j.febslet.2010.01.020; PMID: 20083107
- Proikas-Cezanne T, Ruckerbauer S, Stierhof YD, Berg C, Nordheim A. Human WIPI-1 puncta-formation: a novel assay to assess mammalian autophagy. FEBS Lett 2007; 581:3396 - 404; http://dx.doi.org/10.1016/j.febslet.2007.06.040; PMID: 17618624
- Mizushima N, Noda T, Yoshimori T, Tanaka Y, Ishii T, George MD, et al. A protein conjugation system essential for autophagy. Nature 1998; 395:395 - 8; http://dx.doi.org/10.1038/26506; PMID: 9759731
- Nakatogawa H, Suzuki K, Kamada Y, Ohsumi Y. Dynamics and diversity in autophagy mechanisms: lessons from yeast. Nat Rev Mol Cell Biol 2009; 10:458 - 67; http://dx.doi.org/10.1038/nrm2708; PMID: 19491929
- Youle RJ, Narendra DP. Mechanisms of mitophagy. Nat Rev Mol Cell Biol 2011; 12:9 - 14; http://dx.doi.org/10.1038/nrm3028; PMID: 21179058
- Kabeya Y, Mizushima N, Ueno T, Yamamoto A, Kirisako T, Noda T, et al. LC3, a mammalian homologue of yeast Apg8p, is localized in autophagosome membranes after processing. EMBO J 2000; 19:5720 - 8; http://dx.doi.org/10.1093/emboj/19.21.5720; PMID: 11060023
- Tanida I, Ueno T, Kominami E. LC3 conjugation system in mammalian autophagy. Int J Biochem Cell Biol 2004; 36:2503 - 18; http://dx.doi.org/10.1016/j.biocel.2004.05.009; PMID: 15325588
- Ichimura Y, Kirisako T, Takao T, Satomi Y, Shimonishi Y, Ishihara N, et al. A ubiquitin-like system mediates protein lipidation. Nature 2000; 408:488 - 92; http://dx.doi.org/10.1038/35044114; PMID: 11100732
- Sou YS, Waguri S, Iwata J, Ueno T, Fujimura T, Hara T, et al. The Atg8 conjugation system is indispensable for proper development of autophagic isolation membranes in mice. Mol Biol Cell 2008; 19:4762 - 75; http://dx.doi.org/10.1091/mbc.E08-03-0309; PMID: 18768753
- Kuma A, Hatano M, Matsui M, Yamamoto A, Nakaya H, Yoshimori T, et al. The role of autophagy during the early neonatal starvation period. Nature 2004; 432:1032 - 6; http://dx.doi.org/10.1038/nature03029; PMID: 15525940
- Komatsu M, Waguri S, Ueno T, Iwata J, Murata S, Tanida I, et al. Impairment of starvation-induced and constitutive autophagy in Atg7-deficient mice. J Cell Biol 2005; 169:425 - 34; http://dx.doi.org/10.1083/jcb.200412022; PMID: 15866887
- Saitoh T, Fujita N, Hayashi T, Takahara K, Satoh T, Lee H, et al. Atg9a controls dsDNA-driven dynamic translocation of STING and the innate immune response. Proc Natl Acad Sci U S A 2009; 106:20842 - 6; http://dx.doi.org/10.1073/pnas.0911267106; PMID: 19926846
- Saitoh T, Fujita N, Jang MH, Uematsu S, Yang BG, Satoh T, et al. Loss of the autophagy protein Atg16L1 enhances endotoxin-induced IL-1β production. Nature 2008; 456:264 - 8; http://dx.doi.org/10.1038/nature07383; PMID: 18849965
- Ichimura Y, Komatsu M. Pathophysiological role of autophagy: lesson from autophagy-deficient mouse models. Exp Anim 2011; 60:329 - 45; http://dx.doi.org/10.1538/expanim.60.329; PMID: 21791873
- Komatsu M, Ichimura Y. Selective autophagy regulates various cellular functions. Genes Cells 2010; 15:923 - 33; http://dx.doi.org/10.1111/j.1365-2443.2010.01433.x; PMID: 20670274
- Komatsu M, Ichimura Y. Physiological significance of selective degradation of p62 by autophagy. FEBS Lett 2010; 584:1374 - 8; http://dx.doi.org/10.1016/j.febslet.2010.02.017; PMID: 20153326
- Yang S, Wang X, Contino G, Liesa M, Sahin E, Ying H, et al. Pancreatic cancers require autophagy for tumor growth. Genes Dev 2011; 25:717 - 29; http://dx.doi.org/10.1101/gad.2016111; PMID: 21406549
- Rosenfeldt MT, Ryan KM. The multiple roles of autophagy in cancer. Carcinogenesis 2011; 32:955 - 63; http://dx.doi.org/10.1093/carcin/bgr031; PMID: 21317301
- Sridhar S, Botbol Y, Macian F, Cuervo AM. Autophagy and disease: always two sides to a problem. J Pathol 2012; 226:255 - 73; http://dx.doi.org/10.1002/path.3025; PMID: 21990109
- Deretic V. Autophagy in immunity and cell-autonomous defense against intracellular microbes. Immunol Rev 2011; 240:92 - 104; http://dx.doi.org/10.1111/j.1600-065X.2010.00995.x; PMID: 21349088
- Levine B. Eating oneself and uninvited guests: autophagy-related pathways in cellular defense. Cell 2005; 120:159 - 62; http://dx.doi.org/10.1016/S0092-8674(05)00043-7; PMID: 15680321
- Deretic V. Autophagy as an innate immunity paradigm: expanding the scope and repertoire of pattern recognition receptors. Curr Opin Immunol 2012; 24:21 - 31; http://dx.doi.org/10.1016/j.coi.2011.10.006; PMID: 22118953
- Delgado M, Singh S, De Haro S, Master S, Ponpuak M, Dinkins C, et al. Autophagy and pattern recognition receptors in innate immunity. Immunol Rev 2009; 227:189 - 202; http://dx.doi.org/10.1111/j.1600-065X.2008.00725.x; PMID: 19120485
- Dupont N, Temime-Smaali N, Lafont F. How ubiquitination and autophagy participate in the regulation of the cell response to bacterial infection. Biol Cell 2010; 102:621 - 34; http://dx.doi.org/10.1042/BC20100101; PMID: 21077843
- Saitoh T, Akira S. Regulation of innate immune responses by autophagy-related proteins. J Cell Biol 2010; 189:925 - 35; http://dx.doi.org/10.1083/jcb.201002021; PMID: 20548099
- Deretic V. Multiple regulatory and effector roles of autophagy in immunity. Curr Opin Immunol 2009; 21:53 - 62; http://dx.doi.org/10.1016/j.coi.2009.02.002; PMID: 19269148
- Lleo A, Invernizzi P, Selmi C, Coppel RL, Alpini G, Podda M, et al. Autophagy: highlighting a novel player in the autoimmunity scenario. J Autoimmun 2007; 29:61 - 8; http://dx.doi.org/10.1016/j.jaut.2007.06.003; PMID: 17693057
- Sumpter RJ Jr., Levine B. Autophagy and innate immunity: triggering, targeting and tuning. Semin Cell Dev Biol 2010; 21:699 - 711; http://dx.doi.org/10.1016/j.semcdb.2010.04.003; PMID: 20403453
- Shahnazari S, Brumell JH. Mechanisms and consequences of bacterial targeting by the autophagy pathway. Curr Opin Microbiol 2011; 14:68 - 75; http://dx.doi.org/10.1016/j.mib.2010.11.001; PMID: 21112809
- Andrade RM, Wessendarp M, Gubbels MJ, Striepen B, Subauste CS. CD40 induces macrophage anti-Toxoplasma gondii activity by triggering autophagy-dependent fusion of pathogen-containing vacuoles and lysosomes. J Clin Invest 2006; 116:2366 - 77; http://dx.doi.org/10.1172/JCI28796; PMID: 16955139
- Dupont N, Lacas-Gervais S, Bertout J, Paz I, Freche B, Van Nhieu GT, et al. Shigella phagocytic vacuolar membrane remnants participate in the cellular response to pathogen invasion and are regulated by autophagy. Cell Host Microbe 2009; 6:137 - 49; http://dx.doi.org/10.1016/j.chom.2009.07.005; PMID: 19683680
- Shahnazari S, Yen W-L, Birmingham CL, Shiu J, Namolovan A, Zheng YT, et al. A diacylglycerol-dependent signaling pathway contributes to regulation of antibacterial autophagy. Cell Host Microbe 2010; 8:137 - 46; http://dx.doi.org/10.1016/j.chom.2010.07.002; PMID: 20674539
- Shahnazari S, Namolovan A, Mogridge J, Kim PK, Brumell JH. Bacterial toxins can inhibit host cell autophagy through cAMP generation. Autophagy 2011; 7:957 - 65; http://dx.doi.org/10.4161/auto.7.9.16435; PMID: 21606683
- Intemann CD, Thye T, Niemann S, Browne EN, Amanua Chinbuah M, Enimil A, et al. Autophagy gene variant IRGM -261T contributes to protection from tuberculosis caused by Mycobacterium tuberculosis but not by M. africanum strains. PLoS Pathog 2009; 5:e1000577; http://dx.doi.org/10.1371/journal.ppat.1000577; PMID: 19750224
- Che N, Li S, Gao T, Zhang Z, Han Y, Zhang X, et al. Identification of a novel IRGM promoter single nucleotide polymorphism associated with tuberculosis. Clin Chim Acta 2010; 411:1645 - 9; http://dx.doi.org/10.1016/j.cca.2010.06.009; PMID: 20547146
- Kumar D, Nath L, Kamal MA, Varshney A, Jain A, Singh S, et al. Genome-wide analysis of the host intracellular network that regulates survival of Mycobacterium tuberculosis.. Cell 2010; 140:731 - 43; http://dx.doi.org/10.1016/j.cell.2010.02.012; PMID: 20211141
- Zhang FR, Huang W, Chen SM, Sun LD, Liu H, Li Y, et al. Genomewide association study of leprosy. N Engl J Med 2009; 361:2609 - 18; http://dx.doi.org/10.1056/NEJMoa0903753; PMID: 20018961
- Pinheiro RO, de Souza Salles J, Sarno EN, Sampaio EP. Mycobacterium leprae-host-cell interactions and genetic determinants in leprosy: an overview. Future Microbiol 2011; 6:217 - 30; http://dx.doi.org/10.2217/fmb.10.173; PMID: 21366421
- Deretic V, Levine B. Autophagy, immunity, and microbial adaptations. Cell Host Microbe 2009; 5:527 - 49; http://dx.doi.org/10.1016/j.chom.2009.05.016; PMID: 19527881
- Ogawa M, Mimuro H, Yoshikawa Y, Ashida H, Sasakawa C. Manipulation of autophagy by bacteria for their own benefit. Microbiol Immunol 2011; 55:459 - 71; http://dx.doi.org/10.1111/j.1348-0421.2011.00343.x; PMID: 21707736
- Dreux M, Chisari FV. Viruses and the autophagy machinery. Cell Cycle 2010; 9:1295 - 307; http://dx.doi.org/10.4161/cc.9.7.11109; PMID: 20305376
- Kim HJ, Lee S, Jung JU. When autophagy meets viruses: a double-edged sword with functions in defense and offense. Semin Immunopathol 2010; 32:323 - 41; http://dx.doi.org/10.1007/s00281-010-0226-8; PMID: 20865416
- Wild P, Farhan H, McEwan DG, Wagner S, Rogov VV, Brady NR, et al. Phosphorylation of the autophagy receptor optineurin restricts Salmonella growth. Science 2011; 333:228 - 33; http://dx.doi.org/10.1126/science.1205405; PMID: 21617041
- Criollo A, Senovilla L, Authier H, Maiuri MC, Morselli E, Vitale I, et al. The IKK complex contributes to the induction of autophagy. EMBO J 2010; 29:619 - 31; http://dx.doi.org/10.1038/emboj.2009.364; PMID: 19959994
- Comb WC, Cogswell P, Sitcheran R, Baldwin AS. IKK-dependent, NF-κB-independent control of autophagic gene expression. Oncogene 2011; 30:1727 - 32; http://dx.doi.org/10.1038/onc.2010.553; PMID: 21151171
- Itakura E, Mizushima N. p62 Targeting to the autophagosome formation site requires self-oligomerization but not LC3 binding. J Cell Biol 2011; 192:17 - 27; http://dx.doi.org/10.1083/jcb.201009067; PMID: 21220506
- Singh SB, Ornatowski W, Vergne I, Naylor J, Delgado M, Roberts E, et al. Human IRGM regulates autophagy and cell-autonomous immunity functions through mitochondria. Nat Cell Biol 2010; 12:1154 - 65; http://dx.doi.org/10.1038/ncb2119; PMID: 21102437
- Nakahira K, Haspel JA, Rathinam VA, Lee SJ, Dolinay T, Lam HC, et al. Autophagy proteins regulate innate immune responses by inhibiting the release of mitochondrial DNA mediated by the NALP3 inflammasome. Nat Immunol 2011; 12:222 - 30; http://dx.doi.org/10.1038/ni.1980; PMID: 21151103
- Zhou R, Yazdi AS, Menu P, Tschopp J. A role for mitochondria in NLRP3 inflammasome activation. Nature 2011; 469:221 - 5; http://dx.doi.org/10.1038/nature09663; PMID: 21124315
- Shi CS, Shenderov K, Huang NN, Kabat J, Abu-Asab M, Fitzgerald KA, et al. Activation of autophagy by inflammatory signals limits IL-1β production by targeting ubiquitinated inflammasomes for destruction. Nat Immunol 2012; 13:255 - 63; http://dx.doi.org/10.1038/ni.2215; PMID: 22286270
- Saitoh T, Akira S. Regulation of innate immune responses by autophagy-related proteins. J Cell Biol 2010; 189:925 - 35; http://dx.doi.org/10.1083/jcb.201002021; PMID: 20548099
- Münz C, Lünemann JD, Getts MT, Miller SD. Antiviral immune responses: triggers of or triggered by autoimmunity?. Nat Rev Immunol 2009; 9:246 - 58; http://dx.doi.org/10.1038/nri2527; PMID: 19319143
- Rouse BT, Sehrawat S. Immunity and immunopathology to viruses: what decides the outcome?. Nat Rev Immunol 2010; 10:514 - 26; http://dx.doi.org/10.1038/nri2802; PMID: 20577268
- Mills KH. TLR-dependent T cell activation in autoimmunity. Nat Rev Immunol 2011; 11:807 - 22; PMID: 22094985
- Kawasaki T, Kawai T, Akira S. Recognition of nucleic acids by pattern-recognition receptors and its relevance in autoimmunity. Immunol Rev 2011; 243:61 - 73; http://dx.doi.org/10.1111/j.1600-065X.2011.01048.x; PMID: 21884167
- Shi CS, Kehrl JH. TRAF6 and A20 regulate lysine 63-linked ubiquitination of Beclin-1 to control TLR4-induced autophagy. Sci Signal 2010; 3:ra42; http://dx.doi.org/10.1126/scisignal.2000751; PMID: 20501938
- Dupont N, Jiang S, Pilli M, Ornatowski W, Bhattacharya D, Deretic V. Autophagy-based unconventional secretory pathway for extracellular delivery of IL-1β. EMBO J 2011; 30:4701 - 11; http://dx.doi.org/10.1038/emboj.2011.398; PMID: 22068051
- Tang D, Kang R, Livesey KM, Cheh CW, Farkas A, Loughran P, et al. Endogenous HMGB1 regulates autophagy. J Cell Biol 2010; 190:881 - 92; http://dx.doi.org/10.1083/jcb.200911078; PMID: 20819940
- Skinner M. Autophagy: in the hands of HMGB1. Nat Rev Mol Cell Biol 2010; 11:756 - 7; http://dx.doi.org/10.1038/nrm2994; PMID: 20924397
- Brinkmann V, Reichard U, Goosmann C, Fauler B, Uhlemann Y, Weiss DS, et al. Neutrophil extracellular traps kill bacteria. Science 2004; 303:1532 - 5; http://dx.doi.org/10.1126/science.1092385; PMID: 15001782
- Remijsen Q, Vanden Berghe T, Wirawan E, Asselbergh B, Parthoens E, De Rycke R, et al. Neutrophil extracellular trap cell death requires both autophagy and superoxide generation. Cell Res 2011; 21:290 - 304; http://dx.doi.org/10.1038/cr.2010.150; PMID: 21060338
- Mitroulis I, Kambas K, Chrysanthopoulou A, Skendros P, Apostolidou E, Kourtzelis I, et al. Neutrophil extracellular trap formation is associated with IL-1β and autophagy-related signaling in gout. PLoS One 2011; 6:e29318; http://dx.doi.org/10.1371/journal.pone.0029318; PMID: 22195044
- Remijsen Q, Kuijpers TW, Wirawan E, Lippens S, Vandenabeele P, Vanden Berghe T. Dying for a cause: NETosis, mechanisms behind an antimicrobial cell death modality. Cell Death Differ 2011; 18:581 - 8; http://dx.doi.org/10.1038/cdd.2011.1; PMID: 21293492
- Hakkim A, Fürnrohr BG, Amann K, Laube B, Abed UA, Brinkmann V, et al. Impairment of neutrophil extracellular trap degradation is associated with lupus nephritis. Proc Natl Acad Sci U S A 2010; 107:9813 - 8; http://dx.doi.org/10.1073/pnas.0909927107; PMID: 20439745
- Phillipson M, Kubes P. The neutrophil in vascular inflammation. Nat Med 2011; 17:1381 - 90; http://dx.doi.org/10.1038/nm.2514; PMID: 22064428
- Bakema JE, Ganzevles SH, Fluitsma DM, Schilham MW, Beelen RH, Valerius T, et al. Targeting FcαRI on polymorphonuclear cells induces tumor cell killing through autophagy. J Immunol 2011; 187:726 - 32; http://dx.doi.org/10.4049/jimmunol.1002581; PMID: 21653835
- Wucherpfennig KW, Sethi D. T cell receptor recognition of self and foreign antigens in the induction of autoimmunity. Semin Immunol 2011; 23:84 - 91; http://dx.doi.org/10.1016/j.smim.2011.01.007; PMID: 21306912
- Trowsdale J. The MHC, disease and selection. Immunol Lett 2011; 137:1 - 8; http://dx.doi.org/10.1016/j.imlet.2011.01.002; PMID: 21262263
- Rai E, Wakeland EK. Genetic predisposition to autoimmunity--what have we learned?. Semin Immunol 2011; 23:67 - 83; http://dx.doi.org/10.1016/j.smim.2011.01.015; PMID: 21288738
- Amigorena S, Savina A. Intracellular mechanisms of antigen cross presentation in dendritic cells. Curr Opin Immunol 2010; 22:109 - 17; http://dx.doi.org/10.1016/j.coi.2010.01.022; PMID: 20171863
- Dengjel J, Schoor O, Fischer R, Reich M, Kraus M, Müller M, et al. Autophagy promotes MHC class II presentation of peptides from intracellular source proteins. Proc Natl Acad Sci U S A 2005; 102:7922 - 7; http://dx.doi.org/10.1073/pnas.0501190102; PMID: 15894616
- Crotzer VL, Blum JS. Autophagy and intracellular surveillance: Modulating MHC class II antigen presentation with stress. Proc Natl Acad Sci U S A 2005; 102:7779 - 80; http://dx.doi.org/10.1073/pnas.0503088102; PMID: 15911750
- Schmid D, Pypaert M, Münz C. Antigen-loading compartments for major histocompatibility complex class II molecules continuously receive input from autophagosomes. Immunity 2007; 26:79 - 92; http://dx.doi.org/10.1016/j.immuni.2006.10.018; PMID: 17182262
- Gannage M, Münz C. MHC presentation via autophagy and how viruses escape from it. Semin Immunopathol 2010; 32:373 - 81; http://dx.doi.org/10.1007/s00281-010-0227-7; PMID: 20857294
- Ireland JM, Unanue ER. Autophagy in antigen-presenting cells results in presentation of citrullinated peptides to CD4 T cells. J Exp Med 2011; 208:2625 - 32; http://dx.doi.org/10.1084/jem.20110640; PMID: 22162830
- Paludan C, Schmid D, Landthaler M, Vockerodt M, Kube D, Tuschl T, et al. Endogenous MHC class II processing of a viral nuclear antigen after autophagy. Science 2005; 307:593 - 6; http://dx.doi.org/10.1126/science.1104904; PMID: 15591165
- Lee HK, Mattei LM, Steinberg BE, Alberts P, Lee YH, Chervonsky A, et al. In vivo requirement for Atg5 in antigen presentation by dendritic cells. Immunity 2010; 32:227 - 39; http://dx.doi.org/10.1016/j.immuni.2009.12.006; PMID: 20171125
- Dörfel D, Appel S, Grünebach F, Weck MM, Müller MR, Heine A, et al. Processing and presentation of HLA class I and II epitopes by dendritic cells after transfection with in vitro-transcribed MUC1 RNA. Blood 2005; 105:3199 - 205; http://dx.doi.org/10.1182/blood-2004-09-3556; PMID: 15618468
- Sanjuan MA, Dillon CP, Tait SW, Moshiach S, Dorsey F, Connell S, et al. Toll-like receptor signalling in macrophages links the autophagy pathway to phagocytosis. Nature 2007; 450:1253 - 7; http://dx.doi.org/10.1038/nature06421; PMID: 18097414
- Cooney R, Baker J, Brain O, Danis B, Pichulik T, Allan P, et al. NOD2 stimulation induces autophagy in dendritic cells influencing bacterial handling and antigen presentation. Nat Med 2010; 16:90 - 7; http://dx.doi.org/10.1038/nm.2069; PMID: 19966812
- English L, Chemali M, Duron J, Rondeau C, Laplante A, Gingras D, et al. Autophagy enhances the presentation of endogenous viral antigens on MHC class I molecules during HSV-1 infection. Nat Immunol 2009; 10:480 - 7; http://dx.doi.org/10.1038/ni.1720; PMID: 19305394
- Jagannath C, Lindsey DR, Dhandayuthapani S, Xu Y, Hunter RL Jr., Eissa NT. Autophagy enhances the efficacy of BCG vaccine by increasing peptide presentation in mouse dendritic cells. Nat Med 2009; 15:267 - 76; http://dx.doi.org/10.1038/nm.1928; PMID: 19252503
- Chemali M, Radtke K, Desjardins M, English L. Alternative pathways for MHC class I presentation: a new function for autophagy. Cell Mol Life Sci 2011; 68:1533 - 41; http://dx.doi.org/10.1007/s00018-011-0660-3; PMID: 21390546
- Nedjic J, Aichinger M, Emmerich J, Mizushima N, Klein L. Autophagy in thymic epithelium shapes the T-cell repertoire and is essential for tolerance. Nature 2008; 455:396 - 400; http://dx.doi.org/10.1038/nature07208; PMID: 18701890
- Mortensen M, Ferguson DJ, Edelmann M, Kessler B, Morten KJ, Komatsu M, et al. Loss of autophagy in erythroid cells leads to defective removal of mitochondria and severe anemia in vivo. Proc Natl Acad Sci U S A 2010; 107:832 - 7; http://dx.doi.org/10.1073/pnas.0913170107; PMID: 20080761
- Stephenson LM, Miller BC, Ng A, Eisenberg J, Zhao Z, Cadwell K, et al. Identification of Atg5-dependent transcriptional changes and increases in mitochondrial mass in Atg5-deficient T lymphocytes. Autophagy 2009; 5:625 - 35; http://dx.doi.org/10.4161/auto.5.5.8133; PMID: 19276668
- Mizushima N, Levine B. Autophagy in mammalian development and differentiation. Nat Cell Biol 2010; 12:823 - 30; http://dx.doi.org/10.1038/ncb0910-823; PMID: 20811354
- Pua HH, Guo J, Komatsu M, He YW. Autophagy is essential for mitochondrial clearance in mature T lymphocytes. J Immunol 2009; 182:4046 - 55; http://dx.doi.org/10.4049/jimmunol.0801143; PMID: 19299702
- Jia W, He YW. Temporal regulation of intracellular organelle homeostasis in T lymphocytes by autophagy. J Immunol 2011; 186:5313 - 22; http://dx.doi.org/10.4049/jimmunol.1002404; PMID: 21421856
- Jia W, Pua HH, Li QJ, He YW. Autophagy regulates endoplasmic reticulum homeostasis and calcium mobilization in T lymphocytes. J Immunol 2011; 186:1564 - 74; http://dx.doi.org/10.4049/jimmunol.1001822; PMID: 21191072
- Stephenson LM, Miller BC, Ng A, Eisenberg J, Zhao Z, Cadwell K, et al. Identification of Atg5-dependent transcriptional changes and increases in mitochondrial mass in Atg5-deficient T lymphocytes. Autophagy 2009; 5:625 - 35; http://dx.doi.org/10.4161/auto.5.5.8133; PMID: 19276668
- Pua HH, Dzhagalov I, Chuck M, Mizushima N, He YW. A critical role for the autophagy gene Atg5 in T cell survival and proliferation. J Exp Med 2007; 204:25 - 31; http://dx.doi.org/10.1084/jem.20061303; PMID: 17190837
- Li C, Capan E, Zhao Y, Zhao J, Stolz D, Watkins SC, et al. Autophagy is induced in CD4+ T cells and important for the growth factor-withdrawal cell death. J Immunol 2006; 177:5163 - 8; PMID: 17015701
- Dorshkind K, Montecino-Rodriguez E. Fetal B-cell lymphopoiesis and the emergence of B-1-cell potential. Nat Rev Immunol 2007; 7:213 - 9; http://dx.doi.org/10.1038/nri2019; PMID: 17318232
- Baumgarth N. The double life of a B-1 cell: self-reactivity selects for protective effector functions. Nat Rev Immunol 2011; 11:34 - 46; http://dx.doi.org/10.1038/nri2901; PMID: 21151033
- Barber CL, Montecino-Rodriguez E, Dorshkind K. Developmental relationships between B-1 and B-2 progenitors. Cell Cycle 2011; 10:3810 - 1; http://dx.doi.org/10.4161/cc.10.22.18190; PMID: 22071629
- Miller BC, Zhao Z, Stephenson LM, Cadwell K, Pua HH, Lee HK, et al. The autophagy gene ATG5 plays an essential role in B lymphocyte development. Autophagy 2008; 4:309 - 14; PMID: 18188005
- Towns R, Kabeya Y, Yoshimori T, Guo C, Shangguan Y, Hong S, et al. Sera from patients with type 2 diabetes and neuropathy induce autophagy and colocalization with mitochondria in SY5Y cells. Autophagy 2005; 1:163 - 70; http://dx.doi.org/10.4161/auto.1.3.2068; PMID: 16874076
- von Gunten S, Simon HU. Autophagic-like cell death in neutrophils induced by autoantibodies. Autophagy 2007; 3:67 - 8; PMID: 17102587
- Towns R, Guo C, Shangguan Y, Hong S, Wiley JW. Type 2 diabetes with neuropathy: autoantibody stimulation of autophagy via Fas. Neuroreport 2008; 19:265 - 9; http://dx.doi.org/10.1097/WNR.0b013e3282f4cb50; PMID: 18303564
- Towns R, Pietropaolo M, Wiley JW. Stimulation of autophagy by autoantibody-mediated activation of death receptor cascades. Autophagy 2008; 4:715 - 6; PMID: 18560272
- de Giorgio R, Volta U, Stanghellini V, Cogliandro RF, Barbara G, Corinaldesi R, et al. Neurogenic chronic intestinal pseudo-obstruction: antineuronal antibody-mediated activation of autophagy via Fas. Gastroenterology 2008; 135:601 - 9; http://dx.doi.org/10.1053/j.gastro.2008.05.034; PMID: 18582468
- Kolattukudy PE, Niu J. Inflammation, endoplasmic reticulum stress, autophagy, and the monocyte chemoattractant protein-1/CCR2 pathway. Circ Res 2012; 110:174 - 89; http://dx.doi.org/10.1161/CIRCRESAHA.111.243212; PMID: 22223213
- Cadwell K, Liu JY, Brown SL, Miyoshi H, Loh J, Lennerz JK, et al. A key role for autophagy and the autophagy gene Atg16l1 in mouse and human intestinal Paneth cells. Nature 2008; 456:259 - 63; http://dx.doi.org/10.1038/nature07416; PMID: 18849966
- Saitoh T, Fujita N, Jang MH, Uematsu S, Yang BG, Satoh T, et al. Loss of the autophagy protein Atg16L1 enhances endotoxin-induced IL-1β production. Nature 2008; 456:264 - 8; http://dx.doi.org/10.1038/nature07383; PMID: 18849965
- Lee HK, Lund JM, Ramanathan B, Mizushima N, Iwasaki A. Autophagy-dependent viral recognition by plasmacytoid dendritic cells. Science 2007; 315:1398 - 401; http://dx.doi.org/10.1126/science.1136880; PMID: 17272685
- Kleinnijenhuis J, Oosting M, Plantinga TS, van der Meer JW, Joosten LA, Crevel RV, et al. Autophagy modulates the Mycobacterium tuberculosis-induced cytokine response. Immunology 2011; 134:341 - 8; http://dx.doi.org/10.1111/j.1365-2567.2011.03494.x; PMID: 21978003
- McLeod IX, Zhou X, Li QJ, Wang F, He YW. The class III kinase Vps34 promotes T lymphocyte survival through regulating IL-7Rα surface expression. J Immunol 2011; 187:5051 - 61; http://dx.doi.org/10.4049/jimmunol.1100710; PMID: 22021616
- Marks DJ, Harbord MW, MacAllister R, Rahman FZ, Young J, Al-Lazikani B, et al. Defective acute inflammation in Crohn’s disease: a clinical investigation. Lancet 2006; 367:668 - 78; http://dx.doi.org/10.1016/S0140-6736(06)68265-2; PMID: 16503465
- Coulombe F, Behr MA. Crohn’s disease as an immune deficiency?. Lancet 2009; 374:769 - 70; http://dx.doi.org/10.1016/S0140-6736(09)61576-2; PMID: 19733770
- Lalande JD, Behr MA. Mycobacteria in Crohn’s disease: how innate immune deficiency may result in chronic inflammation. Expert Rev Clin Immunol 2010; 6:633 - 41; http://dx.doi.org/10.1586/eci.10.29; PMID: 20594136
- Kiss MH, Magalhães CS. Autoinflammatory diseases: mimics of autoimmunity or part of its spectrum? Case presentation. J Clin Immunol 2008; 28:Suppl 1 S84 - 9; http://dx.doi.org/10.1007/s10875-008-9179-2; PMID: 18351446
- McDermott MF, Aksentijevich I. The autoinflammatory syndromes. Curr Opin Allergy Clin Immunol 2002; 2:511 - 6; http://dx.doi.org/10.1097/00130832-200212000-00006; PMID: 14752334
- Wellcome Trust Case Control Consortium. Genome-wide association study of 14,000 cases of seven common diseases and 3,000 shared controls. Nature 2007; 447:661 - 78; http://dx.doi.org/10.1038/nature05911; PMID: 17554300
- Anderson CA, Boucher G, Lees CW, Franke A, D’Amato M, Taylor KD, et al. Meta-analysis identifies 29 additional ulcerative colitis risk loci, increasing the number of confirmed associations to 47. Nat Genet 2011; 43:246 - 52; http://dx.doi.org/10.1038/ng.764; PMID: 21297633
- Franke A, McGovern DP, Barrett JC, Wang K, Radford-Smith GL, Ahmad T, et al. Genome-wide meta-analysis increases to 71 the number of confirmed Crohn’s disease susceptibility loci. Nat Genet 2010; 42:1118 - 25; http://dx.doi.org/10.1038/ng.717; PMID: 21102463
- Brest P, Corcelle EA, Cesaro A, Chargui A, Belaïd A, Klionsky DJ, et al. Autophagy and Crohn’s disease: at the crossroads of infection, inflammation, immunity, and cancer. Curr Mol Med 2010; 10:486 - 502; http://dx.doi.org/10.2174/156652410791608252; PMID: 20540703
- Stappenbeck TS, Rioux JD, Mizoguchi A, Saitoh T, Huett A, Darfeuille-Michaud A, et al. Crohn disease: a current perspective on genetics, autophagy and immunity. Autophagy 2011; 7:355 - 74; http://dx.doi.org/10.4161/auto.7.4.13074; PMID: 20729636
- Khor B, Gardet A, Xavier RJ. Genetics and pathogenesis of inflammatory bowel disease. Nature 2011; 474:307 - 17; http://dx.doi.org/10.1038/nature10209; PMID: 21677747
- Fritz T, Niederreiter L, Adolph T, Blumberg RS, Kaser A. Crohn’s disease: NOD2, autophagy and ER stress converge. Gut 2011; 60:1580 - 8; http://dx.doi.org/10.1136/gut.2009.206466; PMID: 21252204
- Meixlsperger S, Münz C. Morbus Crohn--a disease of failing macroautophagy in the immune system?. Int Immunol 2009; 21:1205 - 11; http://dx.doi.org/10.1093/intimm/dxp096; PMID: 19762453
- Cadwell K, Stappenbeck TS, Virgin HW. Role of autophagy and autophagy genes in inflammatory bowel disease. Curr Top Microbiol Immunol 2009; 335:141 - 67; http://dx.doi.org/10.1007/978-3-642-00302-8_7; PMID: 19802564
- Bekpen C, Xavier RJ, Eichler EE. Human IRGM gene “to be or not to be”. Semin Immunopathol 2010; 32:437 - 44; http://dx.doi.org/10.1007/s00281-010-0224-x; PMID: 20737271
- Plantinga TS, Joosten LA, Netea MG. ATG16L1 polymorphisms are associated with NOD2-induced hyperinflammation. Autophagy 2011; 7:1074 - 5; http://dx.doi.org/10.4161/auto.7.9.15867; PMID: 21673517
- Massey DC, Parkes M. Genome-wide association scanning highlights two autophagy genes, ATG16L1 and IRGM, as being significantly associated with Crohn’s disease. Autophagy 2007; 3:649 - 51; PMID: 17921695
- Cotterill L, Payne D, Levinson S, McLaughlin J, Wesley E, Feeney M, et al. Replication and meta-analysis of 13,000 cases defines the risk for interleukin-23 receptor and autophagy-related 16-like 1 variants in Crohn’s disease. Can J Gastroenterol 2010; 24:297 - 302; PMID: 20485703
- Zhang HF, Qiu LX, Chen Y, Zhu WL, Mao C, Zhu LG, et al. ATG16L1 T300A polymorphism and Crohn’s disease susceptibility: evidence from 13,022 cases and 17,532 controls. Hum Genet 2009; 125:627 - 31; http://dx.doi.org/10.1007/s00439-009-0660-7; PMID: 19337756
- Henckaerts L, Cleynen I, Brinar M, John JM, Van Steen K, Rutgeerts P, et al. Genetic variation in the autophagy gene ULK1 and risk of Crohn’s disease. Inflamm Bowel Dis 2011; 17:1392 - 7; http://dx.doi.org/10.1002/ibd.21486; PMID: 21560199
- Klionsky DJ. Crohn’s disease, autophagy, and the Paneth cell. N Engl J Med 2009; 360:1785 - 6; http://dx.doi.org/10.1056/NEJMcibr0810347; PMID: 19369659
- Fisher SA, Tremelling M, Anderson CA, Gwilliam R, Bumpstead S, Prescott NJ, et al, Wellcome Trust Case Control Consortium. Genetic determinants of ulcerative colitis include the ECM1 locus and five loci implicated in Crohn’s disease. Nat Genet 2008; 40:710 - 2; http://dx.doi.org/10.1038/ng.145; PMID: 18438406
- Rioux JD, Xavier RJ, Taylor KD, Silverberg MS, Goyette P, Huett A, et al. Genome-wide association study identifies new susceptibility loci for Crohn disease and implicates autophagy in disease pathogenesis. Nat Genet 2007; 39:596 - 604; http://dx.doi.org/10.1038/ng2032; PMID: 17435756
- Hampe J, Franke A, Rosenstiel P, Till A, Teuber M, Huse K, et al. A genome-wide association scan of nonsynonymous SNPs identifies a susceptibility variant for Crohn disease in ATG16L1. Nat Genet 2007; 39:207 - 11; http://dx.doi.org/10.1038/ng1954; PMID: 17200669
- Yano T, Kurata S. An unexpected twist for autophagy in Crohn’s disease. Nat Immunol 2009; 10:134 - 6; http://dx.doi.org/10.1038/ni0209-134; PMID: 19148195
- Cadwell K, Liu JY, Brown SL, Miyoshi H, Loh J, Lennerz JK, et al. A key role for autophagy and the autophagy gene Atg16l1 in mouse and human intestinal Paneth cells. Nature 2008; 456:259 - 63; http://dx.doi.org/10.1038/nature07416; PMID: 18849966
- Ng SC, Tsoi KK, Kamm MA, Xia B, Wu J, Chan FK, et al. Genetics of inflammatory bowel disease in Asia: Systematic review and meta-analysis. Inflamm Bowel Dis 2012; 18:1164 - 76; http://dx.doi.org/10.1002/ibd.21845; PMID: 21887729
- Chapman JM, Onnie CM, Prescott NJ, Fisher SA, Mansfield JC, Mathew CG, et al. Searching for genotype-phenotype structure: using hierarchical log-linear models in Crohn disease. Am J Hum Genet 2009; 84:178 - 87; http://dx.doi.org/10.1016/j.ajhg.2008.12.015; PMID: 19185283
- Kuballa P, Huett A, Rioux JD, Daly MJ, Xavier RJ. Impaired autophagy of an intracellular pathogen induced by a Crohn’s disease associated ATG16L1 variant. PLoS One 2008; 3:e3391; http://dx.doi.org/10.1371/journal.pone.0003391; PMID: 18852889
- Fujita N, Saitoh T, Kageyama S, Akira S, Noda T, Yoshimori T. Differential involvement of Atg16L1 in Crohn disease and canonical autophagy: analysis of the organization of the Atg16L1 complex in fibroblasts. J Biol Chem 2009; 284:32602 - 9; http://dx.doi.org/10.1074/jbc.M109.037671; PMID: 19783656
- Cadwell K, Patel KK, Maloney NS, Liu TC, Ng AC, Storer CE, et al. Virus-plus-susceptibility gene interaction determines Crohn’s disease gene Atg16L1 phenotypes in intestine. Cell 2010; 141:1135 - 45; http://dx.doi.org/10.1016/j.cell.2010.05.009; PMID: 20602997
- Travassos LH, Carneiro LA, Ramjeet M, Hussey S, Kim YG, Magalhães JG, et al. Nod1 and Nod2 direct autophagy by recruiting ATG16L1 to the plasma membrane at the site of bacterial entry. Nat Immunol 2010; 11:55 - 62; http://dx.doi.org/10.1038/ni.1823; PMID: 19898471
- Travassos LH, Carneiro LA, Girardin S, Philpott DJ. Nod proteins link bacterial sensing and autophagy. Autophagy 2010; 6:409 - 11; http://dx.doi.org/10.4161/auto.6.3.11305; PMID: 20200479
- Plantinga TS, Crisan TO, Oosting M, van de Veerdonk FL, de Jong DJ, Philpott DJ, et al. Crohn’s disease-associated ATG16L1 polymorphism modulates pro-inflammatory cytokine responses selectively upon activation of NOD2. Gut 2011; 60:1229 - 35; http://dx.doi.org/10.1136/gut.2010.228908; PMID: 21406388
- Parkes M, Barrett JC, Prescott NJ, Tremelling M, Anderson CA, Fisher SA, et al, Wellcome Trust Case Control Consortium. Sequence variants in the autophagy gene IRGM and multiple other replicating loci contribute to Crohn’s disease susceptibility. Nat Genet 2007; 39:830 - 2; http://dx.doi.org/10.1038/ng2061; PMID: 17554261
- McCarroll SA, Huett A, Kuballa P, Chilewski SD, Landry A, Goyette P, et al. Deletion polymorphism upstream of IRGM associated with altered IRGM expression and Crohn’s disease. Nat Genet 2008; 40:1107 - 12; http://dx.doi.org/10.1038/ng.215; PMID: 19165925
- Prescott NJ, Dominy KM, Kubo M, Lewis CM, Fisher SA, Redon R, et al. Independent and population-specific association of risk variants at the IRGM locus with Crohn’s disease. Hum Mol Genet 2010; 19:1828 - 39; http://dx.doi.org/10.1093/hmg/ddq041; PMID: 20106866
- Brest P, Lapaquette P, Souidi M, Lebrigand K, Cesaro A, Vouret-Craviari V, et al. A synonymous variant in IRGM alters a binding site for miR-196 and causes deregulation of IRGM-dependent xenophagy in Crohn’s disease. Nat Genet 2011; 43:242 - 5; http://dx.doi.org/10.1038/ng.762; PMID: 21278745
- Brest P, Lapaquette P, Mograbi B, Darfeuille-Michaud A, Hofman P. Risk predisposition for Crohn disease: a “ménage à trois” combining IRGM allele, miRNA and xenophagy. Autophagy 2011; 7:786 - 7; http://dx.doi.org/10.4161/auto.7.7.15595; PMID: 21508684
- Georges M. The long and winding road from correlation to causation. Nat Genet 2011; 43:180 - 1; http://dx.doi.org/10.1038/ng0311-180; PMID: 21350497
- King KY, Lew JD, Ha NP, Lin JS, Ma X, Graviss EA, et al. Polymorphic allele of human IRGM1 is associated with susceptibility to tuberculosis in African Americans. PLoS One 2011; 6:e16317; http://dx.doi.org/10.1371/journal.pone.0016317; PMID: 21283700
- Harley JB, Alarcón-Riquelme ME, Criswell LA, Jacob CO, Kimberly RP, Moser KL, et al, International Consortium for Systemic Lupus Erythematosus Genetics (SLEGEN). Genome-wide association scan in women with systemic lupus erythematosus identifies susceptibility variants in ITGAM, PXK, KIAA1542 and other loci. Nat Genet 2008; 40:204 - 10; http://dx.doi.org/10.1038/ng.81; PMID: 18204446
- Gateva V, Sandling JK, Hom G, Taylor KE, Chung SA, Sun X, et al. A large-scale replication study identifies TNIP1, PRDM1, JAZF1, UHRF1BP1 and IL10 as risk loci for systemic lupus erythematosus. Nat Genet 2009; 41:1228 - 33; http://dx.doi.org/10.1038/ng.468; PMID: 19838195
- Han JW, Zheng HF, Cui Y, Sun LD, Ye DQ, Hu Z, et al. Genome-wide association study in a Chinese Han population identifies nine new susceptibility loci for systemic lupus erythematosus. Nat Genet 2009; 41:1234 - 7; http://dx.doi.org/10.1038/ng.472; PMID: 19838193
- Zhou XJ, Lu XL, Lv JC, Yang HZ, Qin LX, Zhao MH, et al. Genetic association of PRDM1-ATG5 intergenic region and autophagy with systemic lupus erythematosus in a Chinese population. Ann Rheum Dis 2011; 70:1330 - 7; http://dx.doi.org/10.1136/ard.2010.140111; PMID: 21622776
- Ramos PS, Criswell LA, Moser KL, Comeau ME, Williams AH, Pajewski NM, et al, International Consortium on the Genetics of Systemic Erythematosus. A comprehensive analysis of shared loci between systemic lupus erythematosus (SLE) and sixteen autoimmune diseases reveals limited genetic overlap. PLoS Genet 2011; 7:e1002406; http://dx.doi.org/10.1371/journal.pgen.1002406; PMID: 22174698
- Alirezaei M, Fox HS, Flynn CT, Moore CS, Hebb AL, Frausto RF, et al. Elevated ATG5 expression in autoimmune demyelination and multiple sclerosis. Autophagy 2009; 5:152 - 8; http://dx.doi.org/10.4161/auto.5.2.7348; PMID: 19066443
- Zhou XJ, Lu XL, Nath SK, Lv JC, Zhu SN, Yang HZ, et al, International Consortium on the Genetics of Systemic Lupus Erythematosus. Gene-gene interaction of BLK, TNFSF4, TRAF1, TNFAIP3, and REL in systemic lupus erythematosus. Arthritis Rheum 2012; 64:222 - 31; http://dx.doi.org/10.1002/art.33318; PMID: 21905002
- Wang Y, Singh R, Massey AC, Kane SS, Kaushik S, Grant T, et al. Loss of macroautophagy promotes or prevents fibroblast apoptosis depending on the death stimulus. J Biol Chem 2008; 283:4766 - 77; http://dx.doi.org/10.1074/jbc.M706666200; PMID: 18073215
- Koutouzov S, Mathian A, Dalloul A. Type-I interferons and systemic lupus erythematosus. Autoimmun Rev 2006; 5:554 - 62; http://dx.doi.org/10.1016/j.autrev.2006.05.002; PMID: 17027892
- Rönnblom L, Alm GV, Eloranta ML. Type I interferon and lupus. Curr Opin Rheumatol 2009; 21:471 - 7; http://dx.doi.org/10.1097/BOR.0b013e32832e089e; PMID: 19525849
- Zhou XJ, Lv JC, Cheng WR, Yu L, Zhao MH, Zhang H. Association of TLR9 gene polymorphisms with lupus nephritis in a Chinese Han population. Clin Exp Rheumatol 2010; 28:397 - 400; PMID: 20497632
- Urbonaviciute V, Fürnrohr BG, Meister S, Munoz L, Heyder P, De Marchis F, et al. Induction of inflammatory and immune responses by HMGB1-nucleosome complexes: implications for the pathogenesis of SLE. J Exp Med 2008; 205:3007 - 18; http://dx.doi.org/10.1084/jem.20081165; PMID: 19064698
- Bennett L, Palucka AK, Arce E, Cantrell V, Borvak J, Banchereau J, et al. Interferon and granulopoiesis signatures in systemic lupus erythematosus blood. J Exp Med 2003; 197:711 - 23; http://dx.doi.org/10.1084/jem.20021553; PMID: 12642603
- Gros F, Arnold J, Page N, Décossas M, Korganow AS, Martin T, et al. Macroautophagy is deregulated in murine and human lupus T lymphocytes. Autophagy 2012; 8; In press PMID: 22522825
- Wang C, Khalil M, Ravetch J, Diamond B. The naive B cell repertoire predisposes to antigen-induced systemic lupus erythematosus. J Immunol 2003; 170:4826 - 32; PMID: 12707365
- Nedjic J, Aichinger M, Mizushima N, Klein L. Macroautophagy, endogenous MHC II loading and T cell selection: the benefits of breaking the rules. Curr Opin Immunol 2009; 21:92 - 7; http://dx.doi.org/10.1016/j.coi.2009.01.013; PMID: 19246181
- Pua HH, Dzhagalov I, Chuck M, Mizushima N, He Y-W. A critical role for the autophagy gene Atg5 in T cell survival and proliferation. J Exp Med 2007; 204:25 - 31; http://dx.doi.org/10.1084/jem.20061303; PMID: 17190837
- Chang YP, Tsai CC, Huang WC, Wang CY, Chen CL, Lin YS, et al. Autophagy facilitates IFN-gamma-induced Jak2-STAT1 activation and cellular inflammation. J Biol Chem 2010; 285:28715 - 22; http://dx.doi.org/10.1074/jbc.M110.133355; PMID: 20592027
- Bell BD, Walsh CM. Coordinate regulation of autophagy and apoptosis in T cells by death effectors: FADD or foundation. Autophagy 2009; 5:238 - 40; http://dx.doi.org/10.4161/auto.5.2.7512; PMID: 19077534
- Zhang Y, Morgan MJ, Chen K, Choksi S, Liu ZG. Induction of autophagy is essential for monocyte-macrophage differentiation. Blood 2012; 119:2895 - 905; http://dx.doi.org/10.1182/blood-2011-08-372383; PMID: 22223827
- Mihalache CC, Simon HU. Autophagy regulation in macrophages and neutrophils. Exp Cell Res 2012; 318:1187 - 92; http://dx.doi.org/10.1016/j.yexcr.2011.12.021; PMID: 22245582
- Alves JD, Grima B. Oxidative stress in systemic lupus erythematosus and antiphospholipid syndrome: a gateway to atherosclerosis. Curr Rheumatol Rep 2003; 5:383 - 90; http://dx.doi.org/10.1007/s11926-003-0029-1; PMID: 12967525
- Tal MC, Sasai M, Lee HK, Yordy B, Shadel GS, Iwasaki A. Absence of autophagy results in reactive oxygen species-dependent amplification of RLR signaling. Proc Natl Acad Sci U S A 2009; 106:2770 - 5; http://dx.doi.org/10.1073/pnas.0807694106; PMID: 19196953
- Anders HJ, Schlondorff DO. Innate immune receptors and autophagy: implications for autoimmune kidney injury. Kidney Int 2010; 78:29 - 37; http://dx.doi.org/10.1038/ki.2010.111; PMID: 20428100
- Hartleben B, Gödel M, Meyer-Schwesinger C, Liu S, Ulrich T, Köbler S, et al. Autophagy influences glomerular disease susceptibility and maintains podocyte homeostasis in aging mice. J Clin Invest 2010; 120:1084 - 96; http://dx.doi.org/10.1172/JCI39492; PMID: 20200449
- Yousefi S, Perozzo R, Schmid I, Ziemiecki A, Schaffner T, Scapozza L, et al. Calpain-mediated cleavage of Atg5 switches autophagy to apoptosis. Nat Cell Biol 2006; 8:1124 - 32; http://dx.doi.org/10.1038/ncb1482; PMID: 16998475
- Kroemer G, Levine B. Autophagic cell death: the story of a misnomer. Nat Rev Mol Cell Biol 2008; 9:1004 - 10; http://dx.doi.org/10.1038/nrm2529; PMID: 18971948
- Jounai N, Takeshita F, Kobiyama K, Sawano A, Miyawaki A, Xin KQ, et al. The Atg5 Atg12 conjugate associates with innate antiviral immune responses. Proc Natl Acad Sci U S A 2007; 104:14050 - 5; http://dx.doi.org/10.1073/pnas.0704014104; PMID: 17709747
- Zhao Z, Fux B, Goodwin M, Dunay IR, Strong D, Miller BC, et al. Autophagosome-independent essential function for the autophagy protein Atg5 in cellular immunity to intracellular pathogens. Cell Host Microbe 2008; 4:458 - 69; http://dx.doi.org/10.1016/j.chom.2008.10.003; PMID: 18996346
- Fürnrohr BG, Wach S, Kelly JA, Haslbeck M, Weber CK, Stach CM, et al. Polymorphisms in the Hsp70 gene locus are genetically associated with systemic lupus erythematosus. Ann Rheum Dis 2010; 69:1983 - 9; http://dx.doi.org/10.1136/ard.2009.122630; PMID: 20498198
- Page N, Gros F, Schall N, Décossas M, Bagnard D, Briand JP, et al. HSC70 blockade by the therapeutic peptide P140 affects autophagic processes and endogenous MHCII presentation in murine lupus. Ann Rheum Dis 2011; 70:837 - 43; http://dx.doi.org/10.1136/ard.2010.139832; PMID: 21173017
- Page N, Gros F, Schall N, Briand JP, Muller S. A therapeutic peptide in lupus alters autophagic processes and stability of MHCII molecules in MRL/lpr B cells. Autophagy 2011; 7:539 - 40; http://dx.doi.org/10.4161/auto.7.5.14845; PMID: 21282971
- Raychaudhuri S, Thomson BP, Remmers EF, Eyre S, Hinks A, Guiducci C, et al, BIRAC Consortium, YEAR Consortium. Genetic variants at CD28, PRDM1 and CD2/CD58 are associated with rheumatoid arthritis risk. Nat Genet 2009; 41:1313 - 8; http://dx.doi.org/10.1038/ng.479; PMID: 19898481
- Ebato C, Uchida T, Arakawa M, Komatsu M, Ueno T, Komiya K, et al. Autophagy is important in islet homeostasis and compensatory increase of beta cell mass in response to high-fat diet. Cell Metab 2008; 8:325 - 32; http://dx.doi.org/10.1016/j.cmet.2008.08.009; PMID: 18840363
- Fujitani Y, Kawamori R, Watada H. The role of autophagy in pancreatic beta-cell and diabetes. Autophagy 2009; 5:280 - 2; http://dx.doi.org/10.4161/auto.5.2.7656; PMID: 19158492
- Mareninova OA, Hermann K, French SW, O’Konski MS, Pandol SJ, Webster P, et al. Impaired autophagic flux mediates acinar cell vacuole formation and trypsinogen activation in rodent models of acute pancreatitis. J Clin Invest 2009; 119:3340 - 55; PMID: 19805911
- Gukovsky I, Gukovskaya AS. Impaired autophagy underlies key pathological responses of acute pancreatitis. Autophagy 2010; 6:428 - 9; http://dx.doi.org/10.4161/auto.6.3.11530; PMID: 20215882
- He C, Bassik MC, Moresi V, Sun K, Wei Y, Zou Z, et al. Exercise-induced BCL2-regulated autophagy is required for muscle glucose homeostasis. Nature 2012; 481:511 - 5; http://dx.doi.org/10.1038/nature10758; PMID: 22258505
- Compston A, Coles A. Multiple sclerosis. Lancet 2008; 372:1502 - 17; http://dx.doi.org/10.1016/S0140-6736(08)61620-7; PMID: 18970977
- Xu H, Wu ZY, Fang F, Guo L, Chen D, Chen JX, et al. Genetic deficiency of Irgm1 (LRG-47) suppresses induction of experimental autoimmune encephalomyelitis by promoting apoptosis of activated CD4+ T cells. FASEB J 2010; 24:1583 - 92; http://dx.doi.org/10.1096/fj.09-137323; PMID: 20056715
- Furtado GC, Marcondes MC, Latkowski JA, Tsai J, Wensky A, Lafaille JJ. Swift entry of myelin-specific T lymphocytes into the central nervous system in spontaneous autoimmune encephalomyelitis. J Immunol 2008; 181:4648 - 55; PMID: 18802067
- Jeong TJ, Shin MK, Uhm YK, Kim HJ, Chung JH, Lee MH. Association of UVRAG polymorphisms with susceptibility to non-segmental vitiligo in a Korean sample. Exp Dermatol 2010; 19:e323 - 5; http://dx.doi.org/10.1111/j.1600-0625.2009.01039.x; PMID: 20163458