Abstract
Accidental or therapeutic exposure to ionizing radiation has severe physiological consequences and can result in cell death. We previously demonstrated that deficiency or blockade of the ubiquitously expressed receptor CD47 results in remarkable cell and tissue protection against ischemic and radiation stress. Antagonists of CD47 or its ligand THBS1/thrombospondin 1 enhance cell survival and preserve their proliferative capacity. However the signaling pathways that mediate this cell-autonomous radioprotection are unclear. We now report a marked increase in autophagy in irradiated T-cells and endothelial cells lacking CD47. Irradiated T cells lacking CD47 exhibit significant increases in formation of autophagosomes comprising double-membrane vesicles visualized by electron microscopy and numbers of MAP1LC3A/B+ puncta. Moreover, we observed significant increases in BECN1, ATG5, ATG7 and a reduction in SQSTM1/p62 expression relative to irradiated wild-type T cells. We observed similar increases in autophagy gene expression in mice resulting from blockade of CD47 in combination with total body radiation. Pharmacological or siRNA-mediated inhibition of autophagy selectively sensitized CD47-deficient cells to radiation, indicating that enhanced autophagy is necessary for the prosurvival response to CD47 blockade. Moreover, re-expression of CD47 in CD47-deficient T cells sensitized these cells to death by ionizing radiation and reversed the increase in autophagic flux associated with survival. This study indicates that CD47 deficiency confers cell survival through the activation of autophagic flux and identifies CD47 blockade as a pharmacological route to modulate autophagy for protecting tissue from radiation injury.
Introduction
Therapeutic or accidental exposure to ionizing radiation (IR) directly damages cellular macromolecules and leads to formation of reactive radicals that cause genomic instability and cell death. We previously demonstrated that a lack or blockade of CD47 results in profound protection of cells and normal tissue from death induced by IR treatment.Citation1,Citation2 CD47 is a widely expressed transmembrane receptor for two members of the signal regulatory protein (SIRP/SHPS) family and the matricellular protein THBS1.Citation3 Signaling through CD47 controls second messengers such as calcium, cAMP and cGMP in numerous cell types. In vascular cells CD47 inhibits the production and effector pathways of nitric oxide (NO) signaling. Consequently, CD47 signaling plays a major role in cardiovascular physiology by regulating tissue blood flow, platelet homeostasis and angiogenesis.Citation4 Agonists of this pathway such as THBS1 inhibit tumor growth and angiogenesis, but antagonists improve tissue survival of ischemic insults and radiation injuries.Citation2,Citation5−Citation7 Deficiency of CD47 or THBS1 or suppression of CD47 results in radioprotection of human endothelial cells in vitro and protects soft tissue and bone marrow in vivo.Citation1 Moreover, suppression of CD47 in combination with IR enhances the IR-induced delay of tumor regrowth for syngeneic melanoma and squamous carcinoma models.Citation1 Consistent with the known radioprotective activity of NO donors, the absence of CD47 also confers near complete resistance to high dose IR injury in primary null cells and in mouse tissues. However, our previous studies indicated that the radioprotective effect of CD47 suppression is independent of NO/cGMP signaling,Citation7 suggesting that an additional target of CD47 signaling mediates radioprotection. In our search to understand the selective protection of normal cells and tissue from IR with blockade or the absence of CD47, we found increased expression of genes of the autophagy pathway.
Autophagy is a cellular process that involves the lysosomal degradation of macromolecules or organelles within the cell.Citation8 Autophagy plays a role in cellular homeostasis, responses to infection and, potentially, as a cell death mechanism.Citation9 A hallmark of this process is the enclosure of a macromolecule or organelle by a double-membrane vesicle resulting in the formation of an autophagosome.Citation8,Citation10−Citation12 Cells activate autophagy in stress situations to increase survival.Citation13 On the other hand, IR treatment induces a decrease of MAP1LC3A/B (LC3) and the accumulation of SQSTM1 in tissue of mice exposed to IR.Citation14 Moreover, radiation-induced autophagy in cancer cells increases their survival and resistance to therapy.Citation15,Citation16 Conversely, blockade of this pathway can sensitize cells to IR. In this study we demonstrate a novel cytoprotective role of autophagy as a physiological response to IR and demonstrate that deficiency in CD47 increases the expression and function of autophagy associated genes as a protective mechanism from death induced by radiation exposure in cells and in mice.
Results
Deficiency of CD47 increases radioprotection of Jurkat T cells
Our previous studies demonstrated that lack of CD47 preserves survival of tissues and endothelial cells exposed to radiation.Citation1,Citation2 Lack of CD47 in the JinB8 clone of Jurkat T cells (CD47−) similarly conferred resistance to increasing doses of ionizing radiation as assessed by cell viability 72 h after exposure (). Lack of CD47 preserved > 85% viability at all the doses as measured by MTS assay. In contrast wild-type (WT) Jurkat T cells retained only 25% cell viability after irradiation at 40 Gy. To further confirm this protection, we exposed the same number of cells to a 20 Gy dose of IR and measured LDH release 24 h after exposure as a measure of cytotoxicity. We observed only a 10% increase in cytotoxicity in irradiated CD47− cells compared with a 40% increase in cytotoxicity of WT cells (). To determine whether the lack of CD47 preserves proliferative capacity, Jurkat T cells were plated in complete medium and exposed to a 20 Gy dose of radiation. WT and CD47− Jurkat cells showed no difference in crystal violet stainingCitation17,Citation18 at time 0 and similar 4-fold increases in cell numbers at day 10. On the other hand, irradiated cultures of WT cells showed only a 1.5-fold increase in proliferative capacity vs. a 4-fold increase in proliferative capacity in cells that lack CD47 (). Thus lacking CD47 preserves proliferative capacity after IR, demonstrated by the ability of these cells to undergo at least two cycles of cell division. Taken together these experiments demonstrate that the lack of CD47 protects Jurkat T cells from cell death associated with radiation treatment.
Figure 1. Radioprotection of CD47− Jurkat cells. (A) WT and CD47-deficient Jurkat cells were irradiated at the indicated doses, and cell viability was measured by MTS assay (n = 4; one-way ANOVA-Bonferroni post-test; *p < 0.05). (B) Cells were irradiated at 20 Gy, and cell cytotoxicity was measured by LDH release (n = 3; Student’s t-test, *p < 0.05). (C) Cells were irradiated at 20 Gy, and cell density was measured by crystal violet staining 10 d after radiation treatment (n = 3; one-way ANOVA-Bonferroni post-test *p < 0.05)
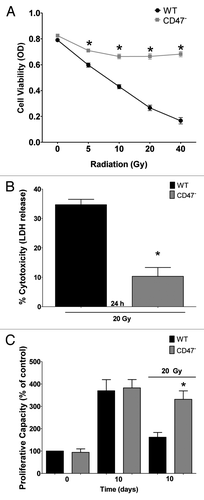
CD47 deficiency stimulates autophagosome formation after IR
Autophagosome formation is the characteristic morphological feature of the autophagic process. To determine if the absence of CD47 stimulates the formation of these structures, Jurkat cells in complete medium were exposed to a 10 Gy dose of IR. After 6 h, cells were fixed, sectioned and analyzed by electron microscopy (). Autophagosomes are defined as double- or single-membrane-bound structures containing undigested cellular components such as vacuoles and membranes as well as mitochondria, that have not fused with a lysosome.Citation19 We observed more than a 2-fold increase in autophagosome number in sections of irradiated CD47− Jurkat T cells when compared with WT irradiated counterparts (). The basal numbers of autophagosomes were not significantly different, indicating that the change in number is due to IR exposure. Since autophagosome formation is a normal homeostatic process, this observation does not suffice to prove that autophagy is activated. Autophagosome expansion is a response to activation of autophagy.Citation20 Under basal conditions, autophagosomes are about 150 nm in size, and activation results in expansion to 300–900 nm.Citation20 We measured autophagosome expansion using imaging software and observed a significant increase in autophagosome size when compared with unirradiated CD47− Jurkat T cells and irradiated WT cells (). Therefore, the lack of CD47 results in autophagosome expansion and activation of autophagy as a response to IR.
Figure 2. Deficiency of CD47 regulates autophagosome formation after IR. (A) Electron microscopy of Jurkat WT and CD47− 6 h post IR. (B) Autophagic vacuole (Av) size was measured from photographs taken from sections and measured using ImageJ software (n = 3 for each condition; Student’s t-test, *p < 0.05). (C) (Av) number was measured in sections of cells differentiating from lysosomal (L) structures (n = 3 for each condition, one-way ANOVA-Bonferroni post-test *p < 0.05). (D) Representative confocal microscopy images and quantification (E) of Jurkat WT and CD47− transfected with GFP-LC3 6 h post-IR.
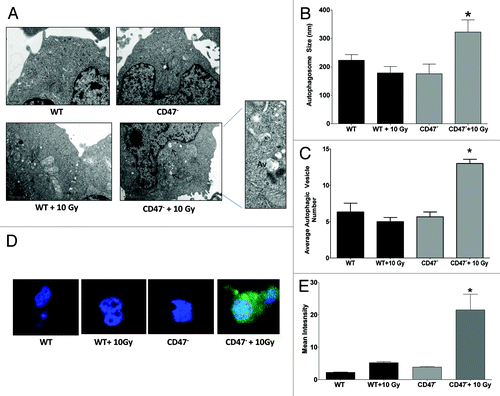
To further explore the role of CD47 in the formation of autophagosomes, we transfected the cells with a GFP-Tagged MAP1LC3A/B construct and treated in the same manner. LC3 is proteolyzed and lipidated to form LC3-II during the assembly of autophagosome membranes, and LC3 in characteristic punctate structures in the cell cytoplasm is a reliable molecular marker of autophagy.Citation21 As shown in representative images (), CD47− Jurkat T cells exposed to IR showed increased LC3 puncta formation. In contrast, we did not observe any change in the formation of these structures in WT cells. Quantification of confocal images showed a > 3-fold increase in GFP-LC3 intensity in sections of CD47− Jurkat cells when compared with untreated and WT controls (). We further confirmed our results by staining endogenous LC3-II using a fluorescent antibody (Fig. S1A) and observed increased expression of LC3-II in irradiated CD47− but not in WT cells. This demonstrates that the LC3 puncta pattern observed in is not an artifact of GFP-LC3 overexpression and suggested that the protection and survival of cells that lack CD47 may be due in part to activation of autophagosome formation and an increased autophagic process.
Because the CD47− Jurkat cell line was generated by chemical mutagenesisCitation22 we wanted to confirm that the altered LC3 puncta formation in response to IR is not due to a secondary mutation in this cell line or other mutations associated with transformation. Lung endothelial cells cultured from WT and CD47−/− mice were transfected with the GFP-LC3 plasmid and exposed to IR (Fig. S1B). WT endothelial cells showed minimal induction of LC3-containing puncta in response to IR, whereas the Cd47 null endothelial cells showed strong induction that resembled that observed in CD47− Jurkat cells. Therefore, targeted deletion of the Cd47 gene was sufficient to enhance induction of autophagosomes in response to IR, and this response as a result of CD47 loss is conserved in murine and human cells.
CD47 deficiency regulates protein expression of LC3-II and SQSTM1 to stimulate autophagy
To further understand how CD47 regulates the activation of autophagy after IR we cultured WT and CD47− Jurkat T cells in complete medium and exposed the cells to a 10 Gy dose of IR. Cultures were harvested at 2, 6 and 24 h post IR exposure, and lysates were analyzed for protein expression by western blot hybridization. Basal expression of proteolyzed and lipidated LC3-II was consistently increased in the CD47− Jurkat T cells (). After IR treatment, LC3-II levels in CD47− cells remained elevated relative to WT and stable at 2 h. Levels also increased in the WT over the non-IR control but not over those of the CD47− cells. LC3-II levels at 6 h remained significantly elevated in CD47− cells relative to the WT counterpart at the same time point and the WT control. At 24 h the levels of LC3-II were reduced to WT levels in the CD47− Jurkat cells, showing that autophagy was not sustained, which could lead to cell death.Citation23 These protein expression data correlate with the increase in autophagosome formation observed in and confirm that lacking CD47 increases autophagy.
Figure 3. Deficiency of CD47 increases the autophagic flux. WT and CD47-deficient Jurkat T cells were cultured in complete medium and exposed to a 10 Gy dose of ionizing radiation. Cells were harvested at 0, 2, 6 and 24 h after IR. Protein expression was assessed by western blot hybridization using specific antibodies to LC3 (A) and SQSTM1 (B). Data were quantified by densitometry and normalizing the signal to that of ACTB/β-actin in the respective cells (n = 3; one-way ANOVA-Bonferroni post-test, *p < 0.05).
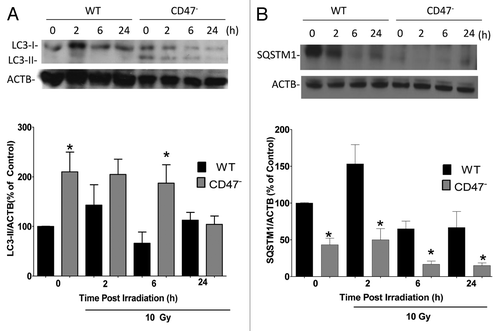
To further explore the role of CD47 in the autophagic flux, we assessed the expression of SQSTM1, which is preferentially degraded by autophagy.Citation19 Total cellular expression levels of SQSTM1 inversely correlate with autophagic activity.Citation21 Basal levels of SQSTM1 were significantly lower in CD47− Jurkat T cells when compared with their untreated WT counterparts (). Upon exposure to IR, SQSTM1 levels in WT cells increased moderately at 2 h and then returned to WT basal levels. A significant reduction in SQSTM1 levels was also observed after IR in CD47− cells at 6 h and 24 h, indicating that autophagy is selectively activated in the CD47− cells as a response to IR. These results indicate that the lack of CD47 activates the autophagic flux to protect Jurkat T cells from IR.
CD47 deficiency regulates BECN1, ATG5 and ATG7 to stimulate autophagy
To determine whether CD47 signaling regulates additional autophagy-related (ATG) genes, we assessed the transcriptional regulation and protein expression of BECN1, ATG5 and ATG7. Autophagy genes are regulated at the transcriptional level in responses to stress.Citation8 WT and CD47− Jurkat T cells were exposed to 10 Gy of radiation and harvested at similar time points as above. BECN1 is an early autophagy-related gene,Citation24 and we observed a significant difference in basal levels of BECN1 mRNA between WT and CD47− cells (). BECN1 mRNA levels in CD47− cells increased over 3-fold and 2-fold relative to WT at 6 h and 24 h, respectively, after exposure to IR. In contrast, BECN1 mRNA levels were not significantly altered by IR in WT cells. BECN1 protein expression, correspondingly, did not change after exposure to IR in WT cells (). However, we observed a > 50% increase of BECN1 protein expression at 2 h in the CD47− cells compared with WT irradiated counterparts at the same time point. At 6 h BECN1 protein expression remained significantly elevated compared with irradiated WT cells but no significant difference was observed at 24 h. This indicates that BECN1 may be upregulated in the CD47− Jurkat cell line as an early response after IR.
Figure 4. Deficiency of CD47 regulates BECN1, ATG5 and ATG7 gene expression. WT and CD47-deficient Jurkat T cells were cultured in complete medium and exposed to a 10 Gy dose of ionizing radiation. Cells were harvested at 0, 2, 6 and 24 h after IR. Protein and gene expression for BECN1 (A and D), ATG5 (B and E) and ATG7 (C and F) were measured by western blot hybridization and real time PCR, respectively (n = 3, Student’s t-test *p < 0.05).
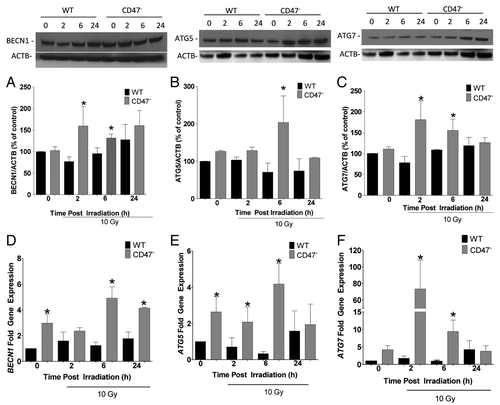
ATGs associate in different complexes to initiate and form the autophagosome. ATG5 interacts with ATG12 in a complex that is essential for autophagosome formation. ATG7 is an E1-like enzyme, and its expression leads to the activation of both LC3-II and ATG12.Citation9,Citation25 mRNA expression of the corresponding genes can be activated as a response to stress.Citation8 ATG5 mRNA levels were significantly elevated basally in the CD47− Jurkat cells when compared with WT (). Although no further increase occurred at 2 h, the levels remained elevated compared with WT cells at the same time point. mRNA levels remained significantly elevated at 6 h compared with the WT counterparts but were reduced to WT levels in the CD47− cells at 24 h. On the other hand, basal protein expression levels of ATG5 were not significantly different but increased over 50% after IR treatment when compared with the WT counterpart at 6 h (). Protein expression levels of ATG5 declined at 24 h and were not significant when compared with the irradiated WT counterpart.
The conjugation reaction of ATG proteins is mediated in part by the action of ATG7. Untreated CD47− cells had higher levels of ATG7 mRNA than WT (). Remarkably, we observed an over 60-fold increase in ATG7 mRNA levels in CD47− cells 2 h post IR, which gradually declined over 24 h. This reveals an early and dramatic CD47-dependent response to IR. Even though ATG7 mRNA levels declined after the 2 h time point, they remained significantly higher than in irradiated WT cells at 6 h. However, ATG7 levels in CD47− decreased and were not different from WT at 24 h. In contrast to the differences in mRNA levels, we did not observe basal differences of ATG7 protein expression between the WT and the CD47− Jurkat cell lines . However, we did observe a > 50% increase in irradiated CD47− Jurkat T cells at 2 h when compared with non-IR controls and a 3-fold increase when compared with the IR WT at the same time point. Even though levels of ATG7 began to decrease in CD47− cells, they remained significantly higher than the WT counterparts at 6 h but returned to basal levels at 24 h and were not different from WT ().
Pharmacological inhibition of autophagy sensitizes CD47-deficient Jurkat T cells to IR
To determine whether autophagy is necessary for the radioresistance of cells lacking CD47, we cultured Jurkat cells in complete medium in the presence of the autophagy inhibitors hydroxychloroquine (HCQ) or 3-methyladenine (3-MA). HCQ raises lysosomal pH, which leads to inhibition of autophagosome lysosome fusion and inhibition of lysosomal protein degradation. 3-MA inhibits autophagosome formation by inhibiting the PtdIns3K pathway.Citation10,Citation19 Cells were treated with inhibitors 1 h prior to irradiation at 20 Gy, and cell viability was examined 72 h after treatment. As observed in , treatment with HCQ sensitized CD47− cells to radiation treatment. In contrast, treatment of WT cells with HCQ did not further sensitize the cells to radiation. Treatment with 3-MA caused also sensitized CD47− cells to IR (). The selective sensitization of CD47− cells by these inhibitors further indicates that the observed radioprotection response requires an autophagic mechanism.
Figure 5. Pharmacological inhibition of autophagy sensitizes CD47-deficient Jurkat T cells to IR. WT and CD47-deficient Jurkat T cells were plated in complete medium in 96-well plates. One hour prior to IR, cells were treated with 25 µM hydroxychloroquine (A) or 5 mM 3-MA (B). Cell viability was measured using MTS reagent after 72 h (n = 3 in triplicate, one-way ANOVA-Bonferroni post-test *p < 0.0001)
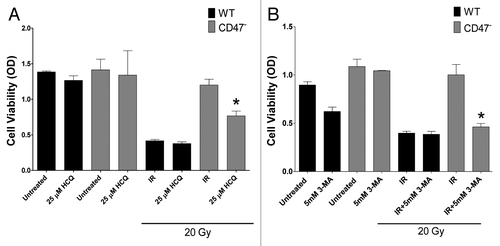
Blockade of ATG genes sensitizes CD47-deficient Jurkat cells to IR
We have observed that CD47 regulates the induction of ATG5 and ATG7 by IR. We also know that these genes are involved in the promotion of autophagy during cellular stress.Citation8 To determine whether the radioprotection effect is dependent on the expression of these genes, we transfected WT and CD47− Jurkat cells with specific siRNAs to silence ATG5 and ATG7. Knockdown of the genes was about 73% for ATG5 and 75% for ATG7 at the transcriptional level, and protein expression was also reduced as corroborated by western blot hybridization (Fig. S2A and B). Cells were plated in complete medium for 24 h before exposure to 20 Gy of IR and then assessed viability (), cytotoxicity (), and proliferative capacity (). In all three assays we observed a significant sensitization of CD47− cells to IR with the knockdown of ATG5 or ATG7. Moreover, blockade of these genes caused the accumulation of SQSTM1 in the cells (Fig. S2C). These results indicate that the increased survival, viability and proliferative capacity observed in CD47− cells after IR requires the activation of autophagy.
Figure 6. Silencing ATG5 and ATG7 sensitizes CD47-deficient Jurkat T cells to IR. WT and CD47− Jurkat T cells were plated in complete media and transfected with siRNAs against ATG5 and ATG7 or nonspecific scrambled control (SCR). Twenty-four hours after transfection cells were irradiated at 20 Gy. Cell viability was measured by MTS (A), cell cytotoxicity by LDH release (B), and proliferative capacity by crystal violet (n = 3 in triplicate; one-way ANOVA-Bonferroni post-test, *p < 0.001).
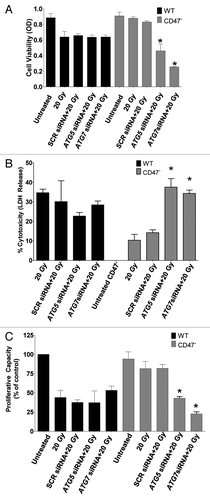
Blockade of CD47 increases autophagy in endothelial cells
To further demonstrate that the regulation of autophagy in absence of CD47 is not particular to the transformed Jurkat cell line, we measured autophagy in HUVEC cells with CD47 knockdown. Protein expression of SQSTM1 was reduced with blockade of CD47 when compared with untreated HUVEC. Levels of SQSTM1 remained elevated after exposure of HUVEC to IR, whereas CD47 blockade in combination with IR caused a further reduction in SQSTM1 protein expression (). On the other hand, treatment of HUVEC with CD47 morpholino alone increased BECN1, ATG5 and ATG7 protein levels when compared with the irradiated WT counterparts. BECN1 was further increased by exposure to IR but remained higher in cells treated with CD47 morpholino relative to control irradiated HUVEC (). Furthermore, in HUVEC we observed a 2-fold increase in BECN1 mRNA levels with blockade of CD47 (Fig. S3A) and 20-fold and 4-fold increases, respectively, in ATG5 (Fig. S3B) and ATG7 (Fig. S3C). Increased autophagy activation was confirmed by a 4-fold increase in LC3 puncta in HUVEC treated with CD47 morpholino in combination with IR (). Thus, regulation of autophagy genes by CD47 is conserved in nontransformed human endothelial cells and can be achieved without a total loss of CD47 expression.
Figure 7. Blockade of CD47 increases autophagy in endothelial cells. HUVEC were plated in complete medium, and one group was treated with 10 µM CD47 antisense morpholino. After 48 h, cells were exposed to a 10 Gy dose of IR, and monolayers were harvested 6 h after IR exposure. (A) Representative images of protein expression of SQSTM1, BECN1, ATG7 and ATG5 in HUVEC measured by western blot hybridization. (B) Confocal microscopy images and quantification (C) of HUVEC treated in the same manner and transfected with GFP-LC3.
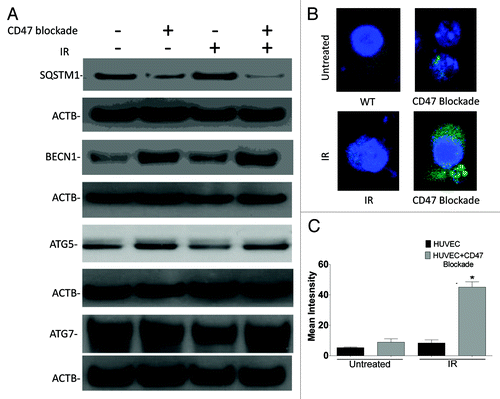
Re-expression of CD47 in the CD47− Jurkat cells reverses radioprotection and autophagic flux
To complement the CD47 knockdown studies in HUVEC, CD47− Jurkat cells were stably transfected with a plasmid to re-express CD47. We confirmed expression of CD47 by flow cytometry and western blot hybridization (). Re-expression of CD47 in the CD47− cells restored their sensitivity to IR (). Moreover, assessment of the autophagic flux by measuring SQSTM1 protein expression and LC3-II expression demonstrated that the observed activation of autophagic flux in CD47− T cells is reversed with re-expression of CD47 (). Re-expression of CD47 also prevented the increase in lipidation of LC3 and LC3 puncta formation induced by IR (). Re-expression of CD47 also restored induction of SQSTM1 by radiation to WT levels (). These data confirm that the radioprotection observed in the CD47− Jurkat cell line is due to the absence of CD47 rather than a secondary somatic mutation. Thus, CD47 regulates autophagy responses in both mouse and human cells in a manner that is consistent with the radioprotection observed by blocking this pathway using CD47 antagonists.
Figure 8. Re-expression of CD47 in CD47− Jurkat cells reverses radioprotection and reduces the autophagic flux. (A) Flow cytometry analysis using FITC-conjugated CD47 antibody B6H12 demonstrates re-expression of CD47 in CD47− Jurkat cells. (B) Western blot hybridization analysis of CD47 expression in Jurkat, CD47−, and CD47− transfected with a plasmid expressing CD47. (C) Re-expression of CD47 in CD47− cells sensitizes this cell line to IR as measured by MTS assay (n = 3 one way ANOVA, *p < 0.04). (D) WT, CD47− and CD47− + CD47 and were cultured in complete medium and harvested before and after exposure to a 10 Gy dose of IR. Protein expression was assessed by western blot hybridization using LC3 and SQSTM1 antibodies. (E) Representative confocal images and quantitative analysis show LC3 puncta formation of cells transfected with GFP-LC3.
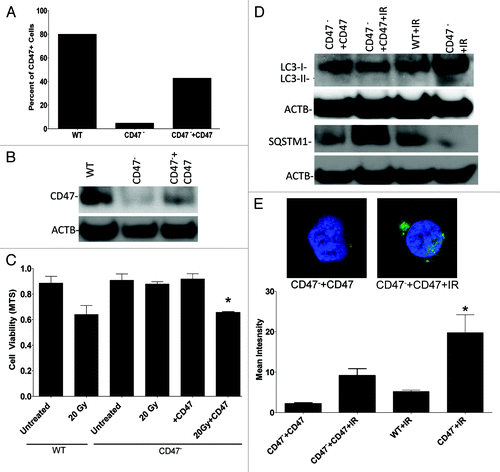
In vivo suppression of CD47 is radioprotective via autophagy
Blockade of CD47 with antisense morpholinos in mice protects soft tissues exposed to IR from apoptotic cell death.Citation1 Lung tissue is very sensitive to therapeutic doses of IR, and the autophagic process is regulated in this tissue after IR exposure.Citation14,Citation15 To determine if protection from apoptosis by CD47 blockade correlates with increased autophagy in vivo, mice were treated IP with CD47 morpholino in saline or saline alone and exposed to total body IR (TBI). As demonstrated in , lung tissue harvested after 24 h from control mice exposed to TBI showed a 5-fold increase in apoptotic nuclei as measured by TUNEL staining. This apoptotic response was inhibited in mice treated with CD47 morpholino before TBI. Basal cell death was not significantly altered by treatment with morpholino alone (). The reduced cell death in irradiated mice treated with CD47 morpholino was associated with a 3-fold increase in Lc3 levels (), a > 4-fold increase in Becn1 (), a > 1.5 fold increase in Atg5 (), and an over 6-fold increase in Atg7 () mRNA expression at 24 h when compared with tissue of mice exposed to IR alone. IR treatment alone caused slight increases in expression of the same autophagy genes when compared with untreated WT animals. Blockade of CD47 in the absence of TBI either had no effect or caused insignificant changes in the expression of these genes. The expression of each of these genes in lung tissue of mice treated with the CD47 morpholino was markedly reduced 48 h after irradiation compared with the 24 h time point (). Thus, the maximal autophagy response coincides with the observed suppression of apoptosis at 24 h. Moreover, immunohistochemical staining with a SQSTM1 antibody () revealed that SQSTM1 expression was significantly reduced in lung tissue of mice treated with IR and blockade of CD47 (), further confirming that the in vivo radioprotection observed in this tissue is associated with activation of autophagy. Together these data reinforce our observations in cultured endothelial cells and CD47− Jurkat cells, and provide a mechanistic basis for our previous findings that blockade of CD47 protects mouse tissues from cell death triggered by IR.
Figure 9. Suppression of CD47 upregulates autophagy and inhibits apoptotic lung tissue cell death in vivo after exposure to total body IR. WT mice were treated with saline or 10 µM CD47 morpholino. After 48 h of treatment, a group of each was exposed to TBI and sacrificed 24 h or 48 h after IR exposure. (A) TUNEL staining of representative sections of lung tissue from mice treated with saline and CD47 morpholino with or without exposure to IR fluorescent nuclei are indicative of apoptotic cells. (B) Quantitative analysis of TUNEL positive nuclei in lung tissue from control and morpholino-treated mice. (C) Immunohistochemical analysis and quantification (D) of SQSTM1 expression in lung tissue of control and irradiated mice treated with CD47 morpholino. Measurement of mRNA levels of LC3 (E) Becn1 (F), Atg5 (G), and Atg7 (H) in lung tissue of mice exposed to total body irradiation after 24 h otherwise noted at 48 h (n = 3, *p < 0.05).
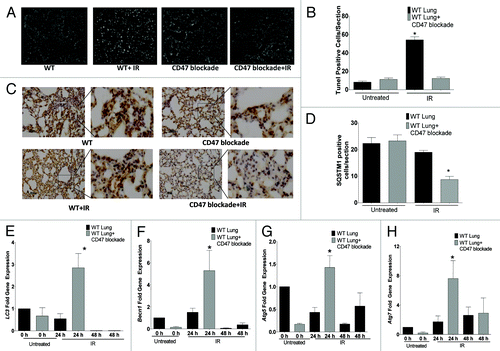
Discussion
We showed for the first time that the effects of CD47 on cell and tissue radiosensitivity are mediated by the regulation of autophagy. Jurkat T cells lacking CD47 reproduce the radioprotected phenotypes we previously reported for CD47 null mouse cells and tissues and human endothelial cells with pharmacological blockade of CD47,Citation1,Citation2 thus providing a convenient cell model to examine the biochemical mechanism by which CD47 confers radioprotection and preserves the proliferative capacity of cells. Increased survival of irradiated CD47− Jurkat T cells and CD47−/− murine lung endothelial cells is associated with increases in autophagosome formation and LC3 puncta formation and protein expression. Degradation of SQSTM1 protein correspondingly increases, indicating that CD47 signaling normally limits cell survival of IR stress by preventing an autophagic flux. Correspondingly, increased expression of BECN1, ATG5 and ATG7 is observed in CD47− Jurkat T cells when compared with their WT counterparts. Enhanced autophagy responses can be reproduced by temporary suppression of CD47 expression in HUVEC using a CD47 morpholino and reversed in CD47− cells by re-expressing CD47. Moreover, suppression of CD47 with the antisense morpholino reduces the percentage of apoptotic cells in lung tissue of mice exposed to IR and is associated with increased expression of autophagy-related genes. These results are consistent with previous studies showing an association between induction of autophagy genes and radioprotection of normal tissue.Citation26 Here we provide the first evidence that CD47 is a master regulator of this autophagic response to IR. Because the radioresistance of CD47− cells can be overcome by treatment with pharmacological inhibitors of autophagy or silencing of autophagy-related genes, autophagy is necessary for the radioprotection conferred by lack of CD47 or its pharmacological blockade.
Cells responding to stress coordinate the activation of autophagy at the transcriptional level to preserve survival.Citation8 Our data indicate that, while CD47 basally regulates autophagy-related genes at the level of their mRNA expression, functional induction of autophagy requires a cell stress such as IR. Notably, we observed CD47-dependent basal differences in mRNA expression for BECN1, ATG5 and ATG7, whereas functional changes in protein expression were observed only after irradiation in CD47− cells. This is consistent with the IR-induced increases in mRNA and protein expression of autophagy-related genes in HUVEC only when CD47 expression was suppressed.
Furthermore, electron microscopy shows increased autophagosome formation and expansion as a consequence of IR, and LC3 fluorescent staining clearly indicates that the LC3 puncta increase after IR in CD47-deficient T cells and endothelial cells.
Taken together, these data suggest that CD47− cells have an increased capacity to stabilize and store mRNA of autophagy-related genes and are thereby primed to rapidly activate autophagy in case of stress. Similar mRNA priming without altered protein expression is well established during T cell activation responses and is mediated mainly by the presence of Internal Ribosome Entry Sites (IRES),Citation27 but is not limited to this cell type.Citation28,Citation29 Several cell types regulate proteins that function during periods of cellular stress that are translated from mRNAs that contain an IRES. IRES in these mRNAs may ensure that their translation is not compromised during stress conditions.Citation30 This regulation of translation by IRES is observed in many eukaryotic cellsCitation28 and is implicated in the integrated stress response, which can modulate protein biosynthesis during stress signals, including endoplasmic reticulum stress, amino acid deprivation and oxidative stress.Citation27 We speculate that autophagy-related genes may contain IRES, and CD47 thereby regulates the integrated stress response to allow translation and activation of the autophagy machinery during stress such as IR.
Moreover, others have shown that autophagy can be regulated by post-transcriptional mRNA stabilization of autophagy-related genes. In HeLa cells, ULK1, LC3B and ATG12 are stabilized at the mRNA level to maintain a pool of ATG transcripts without taxing cells energy demands for de novo synthesis and processing of mRNA.Citation31 Post-transcriptional regulation of mRNA is also in observed for ATG7 and BECN1.Citation32,Citation33 Similarly, our data in Jurkats and HUVEC suggests that deficiency or blockade of CD47 primes cells by stabilizing mRNA to engage the autophagy machinery in an efficient manner to insure cell survival. Such basal effects of CD47 on mRNA levels for certain autophagy genes may contribute to the radioresistence of CD47− cells and the advantages of CD47-null mice in recovering from various forms of ischemic stress and ischemia/reperfusion injury.Citation1,Citation34
Re-expression of CD47 in the Jurkat CD47-deficient cell line reversed the autophagic flux observed in the untransfected cells, demonstrating that CD47 acutely controls autophagy. Our data clearly demonstrate that autophagy is necessary for the radioprotection caused by loss or blockades of CD47. The autophagy inhibitors 3-MA and HCQ sensitize CD47− Jurkat cells to IR. Since pharmacological inhibitors of autophagy can have nonspecific effects,Citation21 we confirmed these results by silencing two ATG genes to specifically inhibit autophagy.Citation19 Our data show that CD47 negatively regulates expression of ATG5 and ATG7 at the level of mRNA and protein expression. Mice deficient in either Atg5 or Atg7 are viable but can die early due to a defective autophagosome formation during a period of starvation before breast feeding is engaged.Citation35 Therefore, these genes are essential for activation of the autophagic process and important in development and the maintenance of cell viability. Moreover, silencing these two genes sensitized cultured cells to IR.Citation25 Silencing of ATG5 and ATG7 similarly sensitized CD47− Jurkat cells to IR, indicating that this pathway is essential for the protection of CD47− cells from IR. Because these ATG genes are involved in the formation of the phagophore assembly site and the formation and elongation of the autophagosome,Citation21 it can be inferred that blocking these steps is sufficient to sensitize cells to IR and that CD47 broadly regulates autophagy. Our studies indicate that CD47 regulates this pathway upstream of ATG5 and ATG7, but inhibition at other levels cannot be excluded. Furthermore, inhibition of autophagy ablates the protective effects of CD47 deficiency or pharmacological inhibition of CD47 shown in this study and in previous publications.Citation1,Citation2 The activation of autophagy in normal tissue is an important mechanism for the maintenance of cellular homeostasis, and its activation is viewed as an advantageous adaptive response in cases of tissue and cellular stress.Citation8,Citation36
The association between tissue radioprotection and an increase in autophagic flux suggests that autophagy-inducing drugs such as the FDA approved carbamazepine,Citation37 could be useful radioprotectors.Citation26 This drug increased the survival of mice exposed to lethal doses of radiation and preserved clonogenic survival of hematopoetic progenitors.Citation26 Others have shown that IR can lead to dysregulation of the autophagic flux, and that accumulation of SQSTM1 is associated with IR treatment in lung tissue.Citation15 Lung tissue is very sensitive to therapeutic doses or IR. In the same study, treatment with the clinically approved radiomitigator Amifostine increases autophagic gene expression. Notably, Amifostine treatment increases the levels of LC3 but not BECN1 after IR exposure. Amifostine treatment has limited cytoprotective efficacy in certain clinical situations,Citation38 which may be due to partial or shortened regulation of the autophagic flux. On the other hand, our results demonstrate that deficiency or blockade of CD47 regulates expression of multiple autophagy genes that control several stages of the autophagic process. Blockade of CD47 in mice exposed to total body irradiation causes an increase in LC3, BECN1, ATG5 and ATG7 in irradiated lung tissue, and this increase is associated with a reduction in lung tissue apoptotic cell death. Here we also demonstrate that blockade with CD47 with morpholino alone does not affect viability of cells and tissue, corroborating our previous reports. Therefore targeting CD47 may prove to be a better strategy for radioprotection due to its broad regulation of the autophagic flux in cells and tissue. It is important to highlight that the activation of autophagy gene protein expression in vitro and in vivo is not sustained, which could lead to cellular death.Citation14 Together with the survival data, this indicates that the observed regulation of autophagy genes protects cells and tissues that lack CD47.
Autophagy may also be the mechanism by which the absence or suppression of CD47 prevents apoptosis in irradiated tissues.Citation1 Ligation of CD47 is known to induce apoptotic and nonapoptotic death in several cell types including activated T cells,Citation39 B-cell chronic lymphocytic leukemia,Citation40,Citation41 promyelocytic leukemia,Citation42 multiple myeloma,Citation43 breast carcinoma,Citation44 and monocytes and dendritic cells.Citation45 Jurkat T cells deficient of CD47 are resistant to Fas-mediated cell death and are sensitized to death by ligation of Fas and re-expression of CD47.Citation39 Induction of T cell death by CD47 also involves activation of BNIP3. BNIP3 is a BCL2 family member that contains a BH3 domain with pro-apoptotic function, but BNIP3 also is essential for autophagy stimulated by 6-thioguanine or 5-fluorouracil.Citation46 Therefore, BNIP3 is one target of CD47 that could control the balance between cell apoptosis and autophagy. Our data identify BECN1 as another CD47 signaling target that regulates BCL2 family member interactions to increase cell survival. BCL2 is a potent anti-apoptotic protein, and induction of its interacting partner BECN1 by loss of CD47 may be a master regulator of the fate decision between apoptotic cell death and protective autophagy.
Activation of autophagy in malignancies such as cancer can lead to genomic instability, tumor progression and resistance to treatment.Citation47 Therefore, blocking CD47 in cancer could lead to resistance to radiation treatment. However, we have previously shown that blockade of CD47 does not protect tumor cells from the effects of ionizing radiation.Citation1 Moreover, we have also shown that blockade of CD47 increases the radiation-induced growth delay in two syngeneic mouse tumor models.Citation1 Therefore, CD47 blockade combined with IR reduces tumor burden in mice. Elevated expression of CD47 is a negative prognostic factor in certain cancers, and blockade of CD47 is beneficial in mouse tumor models. Therefore, CD47 may play an important role in the regulation of autophagy in some cancers but this remains to be confirmed.
Our findings indicate that genetic deletion or temporary suppression of CD47 renders cells nearly immune to radiation-induced cell death and that this cytoprotection depends on an autophagic response. Mice that lack CD47 or its ligand THBS1 are profoundly resistant to a variety of ischemic injuries including soft tissue ischemia and ischemia/reperfusion,Citation48 liver and renal ischemia/reperfusion injury,Citation34,Citation49 spinal cord injury,Citation50 focal cerebral ischemiaCitation51 and radiation. This makes CD47 blockade or agents that downregulate its expression attractive approaches to treat ischemic injuries by inducing autophagy. Other agents that transiently activate autophagy in situations of cellular stress may also prove beneficial to improve tissue survival and overall homeostasis.
Materials and Methods
Cell culture
Jurkat T lymphoma cells and the CD47-deficient (CD47−) Jurkat somatic mutant clone JinB8Citation22 were routinely cultured in RPMI 1640 (Invitrogen, 11875-093) medium supplemented with 10% FBS (Gemini BioProducts, GBP-100106), penicillin/streptomycin and glutamine (Invitrogen, 15070-063, complete growth medium) at 37°C and 5% CO2. Human umbilical vein endothelial cells (HUVEC) and murine lung endothelial cells from WT and CD47 null mice were grown in endothelial cell growth medium-2 in a humidified 37°C incubator with 5% CO2
In vitro radiation experiments
Jurkat T cells were plated in complete growth medium in 96-well plates or 60 mm culture plates. Irradiation was done on a Precision X-Ray X-Rad 320 (operating at kV/10 mA with a 2-mm aluminum filter). The dose rate at 50 cm from the X-Ray source was 242 cGy/minute as determined by multiple thermoluminescent dosimeter readings.
Cell viability assay
WT and CD47− cells were plated at a density of 7 × 103 cells/well in 96-well plates 24 h after plating cells were exposed to a single dose of radiation and allowed to incubate another 72 h at 37°C. For siRNA experiments cell viability was determined by [3-(4,5-dimethylthiazol-2-yl)-5-(3-carboxymethoxyphenyl)-2-(4-sulfophenyl)-2H-tetrazolium (MTS) reduction using the CellTiter 96 Aqueous One Solution cell proliferation assay (Promega, G5421) as per the manufacturer’s instructions, and absorbance at 490 nm was determined with a 96-well plate based spectrophotometer (Dynatech).
Cytotoxicity assay
Jurkat T cells (1 × 104 per well) were seeded into 96-well plates in 200 μL of complete growth medium and exposed to 20 Gy IR. IR-induced cytotoxicity was measured via lactate dehydrogenase (LDH) released due to cell death. LDH release was quantified in cell culture supernatant using the CytoTox 96® Non-Radioactive Cytotoxicity Assay following manufacturer’s instructions (Promega, G1780)
Measurement of proliferative capacity
Proliferative capacity was determined as described previously.Citation17,Citation18 Briefly, 2 × 105 cells were plated in 24-well plates in complete media and either exposed to 10 Gy IR or left untreated as controls. Ten days after radiation treatment, cells were harvested by centrifugation, and medium was removed and the well washed with 1X PBS. Then cells were stained with crystal violet for 15 min and washed in PBS in several centrifugation steps. Cells were left to dry overnight at room temperature. To the dry wells, 100 µl of citrate buffer was added and incubated for 5 min at room temperature. Relative cell density was quantified by spectrophotometry.
Transfection of siRNAs to ATG5 and ATG7
For siRNA experiments, WT and JinB8 Jurkat cells were transfected with siRNAs specific for ATG5, ATG7 or siRNA control using Oligofectamine (Invitrogen, 12252011). siRNA oligonucleotides were designed using the Ambion Silencer siRNA Construction Kit (Ambion, AM1620). Once cells were transfected, cultures were exposed to a 20 Gy dose of IR. Cells were analyzed for viability using MTS, cytotoxicity measuring LDH and proliferative capacity via the crystal violet assay.
Electron microscopy
Cell pellets were fixed in 2.5% glutaraldehyde, postfixed in 0.5% osmium tetroxide, dehydrated and embedded in Spurs epoxy resin. Ultrathin sections (90 nm) were made and double-stained with uranyl acetate and lead citrate, and viewed with a Philips CM10 transmission electron microscope (Phillips Electronics).
Confocal microscopy
WT, CD47-deficient cells and HUVEC were transfected with GFP-LC3 plasmid (Addgene 22405) using the Amaxa nucleofection kit (Lonza). Transfection was performed for 24 h before treatment with IR. HUVECs were transfected with CD47 morpholino (Gene Tools) at the time of GFP-LC3 transfection. Primary endothelial cells were isolated from lungs of WT or CD47−/− mice and transfected with GFP-LC3 using Oligofectamine following the manufacturer’s instructions. For antibody staining of LC3-II, WT and CD47-deficient Jurkat cells (50,000 cells per well) were pelleted and resuspended in 4% paraformaldehyde and allowed to fix for 15 min at room temperature. Fixed cells were washed with PBS and permeabilized/blocked with stain buffer (0.1% Triton X-100/1% BSA/PBS) for 2 min. Cells were then washed 3 times in PBS and incubated with anti-LC3 antibody (1:50 dilution, Nanotools, 0231–100/LC3–5F10) in stain buffer for 1 h at room temperature. Cells were washed 3 times in PBS and incubated with Alexafluor-488 goat anti-mouse secondary antibody (1:500, Invitrogen, A-11001) in stain buffer for 1 h at room temperature. Cells were washed 5 times in PBS and resuspended in Vectashield mounting medium with DAPI (Vector Laboratories, H-1200) and added to the wells of an 8‐chambered Lab‐tek coverslips. Cells were allowed to settle to the bottom of the wells for 30 min at 4°C before imaging on the confocal microscope. Confocal images were acquired on a Zeiss LSM 510 NLO confocal system (Carl Zeiss) with a 63 × Plan‐Apochromat 1.4 NA oil immersion objective. Z‐stacked images were acquired at 512 × 512 pixels per frame using 8‐bit pixel depth for each channel and line averaging set to 4 collected sequentially in a multitrack, three-channel mode.
Western blot hybridization
Cell lysates were obtained by centrifuging the cell suspension and solubilizing the pellets in Triton lysis buffer with protease inhibitor cocktail (Roche, 05 892 791 001). Protein concentrations were measured using a bicinchoninic acid (BCA) protein assay (Pierce, 23225). Proteins were separated by gel electrophoresis on BisTris 4–12% SDS gels (Invitrogen NP0322PK2, NP0321PK2) and transferred to hydrophobic polyvinylidene difluoride membranes (Millipore, IPVH15150). Nonspecific binding was blocked by incubation with Blotto (American Bio Analytical, AB10109-01000). Membranes were probed with primary antibodies to BECN1 (Cell Signaling, 3738), ATG5 (Cell Signaling, 2630), ATG7 (Cell Signaling, 2631), LC3 (Nano Tools, 0231-100/LC3-5F10), or SQSTM1 (MBL International PM045) and treated with polyclonal horseradish peroxidase-conjugated secondary antibodies (R&D Systems PPS029). Immunoreactive products were visualized using chemiluminescence reagents (Super Signal Femto West or Pico West, Pierce 34094, 34079) and quantified by densitometry. Protein loading was determined by using an antibody to ACTB/β-actin (Sigma, A5316). Protein expression is represented as the ratio of the protein of interest over actin.
Quantitative real-time PCR
Jurkat cells were plated in 60-mm dishes in complete medium. Cells were exposed to a single dose of IR (10 Gy) and harvested at 0, 2, 6 and 24 h. Total RNA was isolated using the TRIzol (Invitrogen, 15596-026) following the manufacturer’s instructions. For each analysis, 5 µg of RNA was reverse-transcribed using the SuperSciript III first strand synthesis kit (Invitrogen, 18080-051). Quantitative real-time PCR was performed using SYBR Green (Thermo Scientific, AB-1158A) on an MJ Research Opticon I instrument (BioRad). Human HPRT1 (IDT Technologies, HPRT1 (NM_000194) (5′-ATT GTA ATG ACC AGT CAA CAG GG-3′/5′-GCA TTG TTT TGC CAG TGT CAA-3′) mRNA levels served as an internal control. The results were quantified as Ct values, where Ct is defined as the threshold cycle of PCR at which the amplified product is first detected and expressed as fold gene expression (the ratio of target/control).
Plasmid construction and stable transfection of CD47 in CD47-deficient cells was performed as follows: Plasmid CD47-GFP containing human CD47 isoform 2 (NM_002020.4) fused to EGFP in plasmid vector pEGFPN3Citation52 was obtained from Dr. Dennis Discher, and used to construct an internal V5 epitope tag in CD47. PCR primer pairs M185 ATTCTCGAGATGTGGCCCCTGGTAGCGG (XhoI underlined), M190 CGTAGAATCTAGACCGAGGAGAGGGTTAGGGATAGGCTTACCAACACGATATTTT AGCTCGATGATCG (XbaI underlined) and M191 GGTAAGCCTATCCCTAACCCTCTCCTCGGTCTAGATAGCACGGTTTCATGG TTTTCTCCAAATG (Xba1 underlined), M182 ACTAGTCTATCAGTTATTCCTAGGAGG (Spe1 underlined) with pCD47-GFP as template, were used to produce PCR products of the 5′ proximal and distal regions of the CD47 gene, respectively. Each PCR product was cloned into pCR2.1 TOPO (Invitrogen, K4560-01) and sequenced to ensure no errors had occurred during amplification. To construct the V5 tagged CD47 expression vector, pCD47-GFP was digested with XhoI and NotI, and the base vector was gel purified using standard techniques.Citation53 The proximal CD47 fragment was released from the pCR2.1 vector using XhoI-XbaI and the distal fragment released with XbaI-NotI digestion. The NotI restriction site was obtained from the pCR2.1 vector. The CD47 iV5 vector was constructed by a three-way ligation by incubation of the three purified DNA fragments with DNA ligase overnight at 14°C. Individual clones were sequenced using primer M185 to ensure no errors had occurred during amplification. This procedure resulted in the insertion of an internal V5 epitope tag located nine amino acids before the first transmembrane region in the CD47 molecule (KYR132GGKPIPNLLGLDST VSWFSPNEN; V5 epitope underlined). Cells were washed twice with PBS and stained with FITC conjugated antibodies in staining buffer (PBS, 0.5% BSA, 0.02% sodium azide) at 4°C for 30 min. After two washes with PBS and fixing with 500 μl of 1% paraformaldehyde (Electron Microscopy Sciences, 30525-89-4), cells were incubated with B6H12 antibody to CD47. Cells were analyzed by a FACS Caliber flow cytometer (Becton Dickinson). Flow cytometry data were then analyzed using FlowJo software (TreeStar). Jurkat cells were gated based on cell optical characteristics (FSC vs. SSC)
Animal model of total body irradiation
Age and sex matched C57BL/6 mice were injected intraperitoneally with 750 µl of 10 µM of CD47 morpholino (Gene Tools) and exposed to a single dose of IR at 7.6 Gy using a Therapax DXT300 X-ray irradiator (Pantak, Inc. using 2.0-mm Al filtration (300 kVp) at a dose rate of 2.53 Gy/min. C57BL/6 (NCI) were sacrificed 24 h after irradiation exposure. Mice were housed in a pathogen-free environment and had ad libitum access to standard rat chow and water. Care and handling of animals was in compliance with standards established by the Animal Care and Use Committee of the National Cancer Institute.
Immunohistochemistry
Staining for SQSTM1 was done as previously describedCitation1 utilizing a SQSTM1/p62 antibody (MBL International PM045). Tissue sections were prepared by American HistoLabs.
Terminal deoxynucleotidyl transferase dUTP nick end labeling (TUNEL) staining
Tissues of mice were extracted 24 h post IR treatment and were fixed immediately in 10% formalin. TUNEL staining was performed in paraffin-embedded sections using the Roche in situ fluorescein detection kit (Roche, 11684795910) following the manufacturer’s instructions.
Statistics
Results are presented as the mean ± SD with significance calculated by the Student’s t-test or ANOVA analysis as appropriate Graphpad Prism software. Significance was assigned for a p value ≤ 0.05.
Abbreviations: | ||
3-MA | = | 3-methyladenine |
ATG | = | autophagy related |
BCA | = | bicinchoninic acid |
BCL2 | = | B-cell lymphoma 2 |
BNIP3 | = | BCL2/adenovirus protein-interacting protein 3 |
cAMP | = | cyclic adenosine monophosphate |
CD47(-) | = | cluster of differentiation 47 deficient |
CD47M | = | CD47 morpholino |
cGMP | = | cyclic guanosine monophosphate |
FBS | = | fetal bovine serum |
FDA | = | Food and Drug Administration |
HCQ | = | hydroxychloroquine |
HUVEC | = | human umbilical endothelial cell |
IR | = | ionizing radiation |
IRES | = | internal ribosome entry sites |
LDH | = | lactate dehydrogenase |
MAP1LC3A/B | = | microtubule-associated protein 1 light chain 3 |
NO | = | nitric oxide |
SCR | = | scrambled |
SHPS1 | = | SH2 domain-containing protein tyrosine phosphatase substrate 1 |
SIRP | = | signal regulatory protein |
WT | = | wild type |
Additional material
Download Zip (439.4 KB)Acknowledgments
This work was supported by the Intramural Research Program of the National Institutes of Health, National Cancer Institute, Center for Cancer Research (D.D.R., D.A.W., M.T.) and an NCI Director’s Career Development Innovation Award (D.R.S.-P.).
Disclosure of Potential Conflicts of Interest
No potential conflicts of interest were disclosed.
Supplemental Materials
Supplemental materials may be found here: www.landesbioscience.com/journals/autophagy/article/21562
References
- Maxhimer JB, Soto-Pantoja DR, Ridnour LA, Shih HB, Degraff WG, Tsokos M, et al. Radioprotection in normal tissue and delayed tumor growth by blockade of CD47 signaling. Sci Transl Med 2009; 1:ra7; http://dx.doi.org/10.1126/scitranslmed.3000139; PMID: 20161613
- Isenberg JS, Maxhimer JB, Hyodo F, Pendrak ML, Ridnour LA, DeGraff WG, et al. Thrombospondin-1 and CD47 limit cell and tissue survival of radiation injury. Am J Pathol 2008; 173:1100 - 12; http://dx.doi.org/10.2353/ajpath.2008.080237; PMID: 18787106
- Roberts DD, Miller TW, Rogers NM, Yao M, Isenberg JS. The matricellular protein thrombospondin-1 globally regulates cardiovascular function and responses to stress via CD47. Matrix Biol 2012; 31:162 - 9; http://dx.doi.org/10.1016/j.matbio.2012.01.005; PMID: 22266027
- Isenberg JS, Martin-Manso G, Maxhimer JB, Roberts DD. Regulation of nitric oxide signalling by thrombospondin 1: implications for anti-angiogenic therapies. Nat Rev Cancer 2009; 9:182 - 94; http://dx.doi.org/10.1038/nrc2561; PMID: 19194382
- Isenberg JS, Qin Y, Maxhimer JB, Sipes JM, Despres D, Schnermann J, et al. Thrombospondin-1 and CD47 regulate blood pressure and cardiac responses to vasoactive stress. Matrix Biol 2009; 28:110 - 9; http://dx.doi.org/10.1016/j.matbio.2009.01.002; PMID: 19284971
- Isenberg JS, Romeo MJ, Maxhimer JB, Smedley J, Frazier WA, Roberts DD. Gene silencing of CD47 and antibody ligation of thrombospondin-1 enhance ischemic tissue survival in a porcine model: implications for human disease. Ann Surg 2008; 247:860 - 8; http://dx.doi.org/10.1097/SLA.0b013e31816c4006; PMID: 18438125
- Maxhimer JB, Shih HB, Isenberg JS, Miller TW, Roberts DD. Thrombospondin-1/CD47 blockade following ischemia-reperfusion injury is tissue protective. Plast Reconstr Surg 2009; 124:1880 - 9; http://dx.doi.org/10.1097/PRS.0b013e3181bceec3; PMID: 19952644
- He C, Klionsky DJ. Regulation mechanisms and signaling pathways of autophagy. Annu Rev Genet 2009; 43:67 - 93; http://dx.doi.org/10.1146/annurev-genet-102808-114910; PMID: 19653858
- Klionsky DJ. Autophagy. Curr Biol 2005; 15:R282 - 3; http://dx.doi.org/10.1016/j.cub.2005.04.013; PMID: 15854889
- Klionsky DJ, Abeliovich H, Agostinis P, Agrawal DK, Aliev G, Askew DS, et al. Guidelines for the use and interpretation of assays for monitoring autophagy in higher eukaryotes. Autophagy 2008; 4:151 - 75; PMID: 18188003
- Reggiori F, Klionsky DJ. Autophagosomes: biogenesis from scratch?. Curr Opin Cell Biol 2005; 17:415 - 22; http://dx.doi.org/10.1016/j.ceb.2005.06.007; PMID: 15978794
- Yorimitsu T, Klionsky DJ. Autophagy: molecular machinery for self-eating. Cell Death Differ 2005; 12:Suppl 2 1542 - 52; http://dx.doi.org/10.1038/sj.cdd.4401765; PMID: 16247502
- Cook KL, Shajahan AN, Clarke R. Autophagy and endocrine resistance in breast cancer. Expert Rev Anticancer Ther 2011; 11:1283 - 94; http://dx.doi.org/10.1586/era.11.111; PMID: 21916582
- Zois CE, Giatromanolaki A, Sivridis E, Papaiakovou M, Kainulainen H, Koukourakis MI. “Autophagic flux” in normal mouse tissues: focus on endogenous LC3A processing. Autophagy 2011; 7:1371 - 8; http://dx.doi.org/10.4161/auto.7.11.16664; PMID: 21997374
- Zois CE, Giatromanolaki A, Kainulainen H, Botaitis S, Torvinen S, Simopoulos C, et al. Lung autophagic response following exposure of mice to whole body irradiation, with and without amifostine. Biochem Biophys Res Commun 2011; 404:552 - 8; http://dx.doi.org/10.1016/j.bbrc.2010.12.024; PMID: 21145309
- Paglin S, Hollister T, Delohery T, Hackett N, McMahill M, Sphicas E, et al. A novel response of cancer cells to radiation involves autophagy and formation of acidic vesicles. Cancer Res 2001; 61:439 - 44; PMID: 11212227
- Franken NA, Rodermond HM, Stap J, Haveman J, van Bree C. Clonogenic assay of cells in vitro. Nat Protoc 2006; 1:2315 - 9; http://dx.doi.org/10.1038/nprot.2006.339; PMID: 17406473
- Suk K, Sipes DG, Erickson KL. Enhancement of B-cell translocation gene-1 expression by prostaglandin E2 in macrophages and the relationship to proliferation. Immunology 1997; 91:121 - 9; http://dx.doi.org/10.1046/j.1365-2567.1997.00235.x; PMID: 9203975
- Mizushima N, Yoshimori T, Levine B. Methods in mammalian autophagy research. Cell 2010; 140:313 - 26; http://dx.doi.org/10.1016/j.cell.2010.01.028; PMID: 20144757
- Klionsky DJ, Emr SD. Autophagy as a regulated pathway of cellular degradation. Science 2000; 290:1717 - 21; http://dx.doi.org/10.1126/science.290.5497.1717; PMID: 11099404
- Levine B, Ranganathan R. Autophagy: Snapshot of the network. Nature 2010; 466:38 - 40; http://dx.doi.org/10.1038/466038a; PMID: 20596005
- Reinhold MI, Green JM, Lindberg FP, Ticchioni M, Brown EJ. Cell spreading distinguishes the mechanism of augmentation of T cell activation by integrin-associated protein/CD47 and CD28. Int Immunol 1999; 11:707 - 18; http://dx.doi.org/10.1093/intimm/11.5.707; PMID: 10330276
- Singh K, Matsuyama S, Drazba JA, Almasan A. Autophagy-dependent senescence in response to DNA damage and chronic apoptotic stress. Autophagy 2012; 8:236 - 51; http://dx.doi.org/10.4161/auto.8.2.18600; PMID: 22240589
- He C, Levine B. The Beclin 1 interactome. Curr Opin Cell Biol 2010; 22:140 - 9; http://dx.doi.org/10.1016/j.ceb.2010.01.001; PMID: 20097051
- Wilson EN, Bristol ML, Di X, Maltese WA, Koterba K, Beckman MJ, et al. A switch between cytoprotective and cytotoxic autophagy in the radiosensitization of breast tumor cells by chloroquine and vitamin D. Horm Cancer 2011; 2:272 - 85; http://dx.doi.org/10.1007/s12672-011-0081-7; PMID: 21887591
- Kim H, Bernard ME, Flickinger J, Epperly MW, Wang H, Dixon TM, et al. The autophagy-inducing drug carbamazepine is a radiation protector and mitigator. Int J Radiat Biol 2011; 87:1052 - 60; http://dx.doi.org/10.3109/09553002.2011.587860; PMID: 21728759
- Scheu S, Stetson DB, Reinhardt RL, Leber JH, Mohrs M, Locksley RM. Activation of the integrated stress response during T helper cell differentiation. Nat Immunol 2006; 7:644 - 51; http://dx.doi.org/10.1038/ni1338; PMID: 16680145
- Qin X, Sarnow P. Preferential translation of internal ribosome entry site-containing mRNAs during the mitotic cycle in mammalian cells. J Biol Chem 2004; 279:13721 - 8; http://dx.doi.org/10.1074/jbc.M312854200; PMID: 14739278
- Rzymski T, Harris AL. The unfolded protein response and integrated stress response to anoxia. Clin Cancer Res 2007; 13:2537 - 40; http://dx.doi.org/10.1158/1078-0432.CCR-06-2126; PMID: 17473181
- Lang KJ, Kappel A, Goodall GJ. Hypoxia-inducible factor-1alpha mRNA contains an internal ribosome entry site that allows efficient translation during normoxia and hypoxia. Mol Biol Cell 2002; 13:1792 - 801; http://dx.doi.org/10.1091/mbc.02-02-0017; PMID: 12006670
- Khambu B, Uesugi M, Kawazoe Y. Translational repression stabilizes messenger RNA of autophagy-related genes. Genes Cells 2011; 16:857 - 67; http://dx.doi.org/10.1111/j.1365-2443.2011.01532.x; PMID: 21790910
- Bose JK, Huang CC, Shen CK. Regulation of autophagy by neuropathological protein TDP-43. J Biol Chem 2011; 286:44441 - 8; http://dx.doi.org/10.1074/jbc.M111.237115; PMID: 22052911
- Zhu H, Wu H, Liu X, Li B, Chen Y, Ren X, et al. Regulation of autophagy by a beclin 1-targeted microRNA, miR-30a, in cancer cells. Autophagy 2009; 5:816 - 23; PMID: 19535919
- Isenberg JS, Maxhimer JB, Powers P, Tsokos M, Frazier WA, Roberts DD. Treatment of liver ischemia-reperfusion injury by limiting thrombospondin-1/CD47 signaling. Surgery 2008; 144:752 - 61; http://dx.doi.org/10.1016/j.surg.2008.07.009; PMID: 19081017
- Moreau K, Luo S, Rubinsztein DC. Cytoprotective roles for autophagy. Curr Opin Cell Biol 2010; 22:206 - 11; http://dx.doi.org/10.1016/j.ceb.2009.12.002; PMID: 20045304
- Kroemer G, Mariño G, Levine B. Autophagy and the integrated stress response. Mol Cell 2010; 40:280 - 93; http://dx.doi.org/10.1016/j.molcel.2010.09.023; PMID: 20965422
- Hidvegi T, Ewing M, Hale P, Dippold C, Beckett C, Kemp C, et al. An autophagy-enhancing drug promotes degradation of mutant alpha1-antitrypsin Z and reduces hepatic fibrosis. Science 2010; 329:229 - 32; http://dx.doi.org/10.1126/science.1190354; PMID: 20522742
- Eisbruch A. Amifostine in the treatment of head and neck cancer: intravenous administration, subcutaneous administration, or none of the above. J Clin Oncol 2011; 29:119 - 21; http://dx.doi.org/10.1200/JCO.2010.31.5051; PMID: 21115854
- Sarfati M, Fortin G, Raymond M, Susin S. CD47 in the immune response: role of thrombospondin and SIRP-alpha reverse signaling. Curr Drug Targets 2008; 9:842 - 50; http://dx.doi.org/10.2174/138945008785909310; PMID: 18855618
- Sagawa M, Shimizu T, Fukushima N, Kinoshita Y, Ohizumi I, Uno S, et al. A new disulfide-linked dimer of a single-chain antibody fragment against human CD47 induces apoptosis in lymphoid malignant cells via the hypoxia inducible factor-1α pathway. Cancer Sci 2011; 102:1208 - 15; http://dx.doi.org/10.1111/j.1349-7006.2011.01925.x; PMID: 21401803
- Bras M, Yuste VJ, Roué G, Barbier S, Sancho P, Virely C, et al. Drp1 mediates caspase-independent type III cell death in normal and leukemic cells. Mol Cell Biol 2007; 27:7073 - 88; http://dx.doi.org/10.1128/MCB.02116-06; PMID: 17682056
- Saumet A, Slimane MB, Lanotte M, Lawler J, Dubernard V. Type 3 repeat/C-terminal domain of thrombospondin-1 triggers caspase-independent cell death through CD47/alphavbeta3 in promyelocytic leukemia NB4 cells. Blood 2005; 106:658 - 67; http://dx.doi.org/10.1182/blood-2004-09-3585; PMID: 15784731
- Kikuchi Y, Uno S, Kinoshita Y, Yoshimura Y, Iida S, Wakahara Y, et al. Apoptosis inducing bivalent single-chain antibody fragments against CD47 showed antitumor potency for multiple myeloma. Leuk Res 2005; 29:445 - 50; http://dx.doi.org/10.1016/j.leukres.2004.09.005; PMID: 15725479
- Manna PP, Frazier WA. CD47 mediates killing of breast tumor cells via Gi-dependent inhibition of protein kinase A. Cancer Res 2004; 64:1026 - 36; http://dx.doi.org/10.1158/0008-5472.CAN-03-1708; PMID: 14871834
- Johansson U, Higginbottom K, Londei M. CD47 ligation induces a rapid caspase-independent apoptosis-like cell death in human monocytes and dendritic cells. Scand J Immunol 2004; 59:40 - 9; http://dx.doi.org/10.1111/j.0300-9475.2004.01355.x; PMID: 14723620
- Zeng X, Kinsella TJ. BNIP3 is essential for mediating 6-thioguanine- and 5-fluorouracil-induced autophagy following DNA mismatch repair processing. Cell Res 2010; 20:665 - 75; http://dx.doi.org/10.1038/cr.2010.40; PMID: 20368736
- White E, DiPaola RS. The double-edged sword of autophagy modulation in cancer. Clin Cancer Res 2009; 15:5308 - 16; http://dx.doi.org/10.1158/1078-0432.CCR-07-5023; PMID: 19706824
- Isenberg JS, Hyodo F, Pappan LK, Abu-Asab M, Tsokos M, Krishna MC, et al. Blocking thrombospondin-1/CD47 signaling alleviates deleterious effects of aging on tissue responses to ischemia. Arterioscler Thromb Vasc Biol 2007; 27:2582 - 8; http://dx.doi.org/10.1161/ATVBAHA.107.155390; PMID: 17916772
- Thakar CV, Zahedi K, Revelo MP, Wang Z, Burnham CE, Barone S, et al. Identification of thrombospondin 1 (TSP-1) as a novel mediator of cell injury in kidney ischemia. J Clin Invest 2005; 115:3451 - 9; http://dx.doi.org/10.1172/JCI25461; PMID: 16294224
- Myers SA, DeVries WH, Andres KR, Gruenthal MJ, Benton RL, Hoying JB, et al. CD47 knockout mice exhibit improved recovery from spinal cord injury. Neurobiol Dis 2011; 42:21 - 34; http://dx.doi.org/10.1016/j.nbd.2010.12.010; PMID: 21168495
- Jin G, Tsuji K, Xing C, Yang YG, Wang X, Lo EH. CD47 gene knockout protects against transient focal cerebral ischemia in mice. Exp Neurol 2009; 217:165 - 70; http://dx.doi.org/10.1016/j.expneurol.2009.02.004; PMID: 19233173
- Subramanian S, Boder ET, Discher DE. Phylogenetic divergence of CD47 interactions with human signal regulatory protein alpha reveals locus of species specificity. Implications for the binding site. J Biol Chem 2007; 282:1805 - 18; http://dx.doi.org/10.1074/jbc.M603923200; PMID: 17098740
- Sambrook J. Molecular Cloning: A Laboratory Manual. NY: Cold Spring Harbor Laboratory Press NY, 2001.