Abstract
When the levels of intracellular amino acids are high, RRAG GTPases recruit MTORC1 to lysosomes and promote its activation. We found that RRAGs also recruit specific MTORC1 substrates to the lysosomal surface, thus facilitating MTORC1-mediated phosphorylation and regulation. In particular, active RRAGs interact with the transcription factor EB (TFEB), the master regulator of a gene network that promotes lysosomal biogenesis and autophagy. Redistribution to lysosomes is critical for MTORC1-dependent inactivation of TFEB under nutrient-rich conditions. Therefore, RRAGs play a critical role coordinating nutrient availability and cellular clearance.
The transcription factor EB (TFEB) is a master regulator of lysosomal and autophagic gene expression. In basal conditions, TFEB resides in the cytoplasm. However, under specific circumstances, such as starvation, TFEB rapidly translocates to the nucleus and activates a transcriptional program that includes numerous genes associated with lysosomal biogenesis and function. TFEB also upregulates genes implicated in the major steps of the autophagy pathway, including formation of autophagosomes, fusion of autophagosomes with lysosomes and degradation of autophagosomal substrates. Therefore, TFEB provides coordinated transcriptional regulation of the two main degradative organelles in the cell, autophagosomes and lysosomes.
A critical question for understanding TFEB regulation is how cells link TFEB activation to nutrient availability. An important clue was provided by the recent finding by our laboratory, along with several others, that TFEB is regulated by MTORC1. Under nutrient-rich conditions, active MTORC1 directly phosphorylates TFEB at several residues, including serine 211 (S211). Phosphorylation in S211 promotes interaction of TFEB with the cytosolic chaperone YWHA (14-3-3) and consequent retention of TFEB in the cytosol. Upon inactivation of MTORC1 by starvation, the TFEB-YWHA complex dissociates and TFEB is free to enter the nucleus and activate lysosomal biogenesis and autophagy. Although these findings were very informative, there was an important question that remained to be answered. While TFEB appears diffuse in the cytosol, active MTORC1 localizes to the lysosomal surface. In the presence of nutrients (in particular, amino acids), MTORC1 is recruited to the lysosomal surface by an interaction with RRAGs GTPases and is then activated by RHEB GTPase. Therefore, the prediction is that TFEB must be recruited to lysosomes in order to be phosphorylated by active MTORC1, and this recruitment should be critical for proper TFEB regulation.
In the current study we identify the molecular machinery that regulates association of TFEB with lysosomal membranes, and further elucidate the cellular signals that coordinate nutrient availability with TFEB regulation. We find that TFEB associates with lysosomes in an amino acid-dependent manner through direct interaction with active RRAG GTPases. Changes in the levels of intracellular amino acids alter the nucleotide state of the RRAGs. When amino acids are abundant, RRAGs are active and promote recruitment of TFEB and MTORC1 to lysosomes. This allows MTORC1-dependent phosphorylation of TFEB and assures retention of TFEB in the cytosol under conditions in which autophagy is not required. In fully fed cells, TFEB continuously cycles between lysosomes and the cytosol. At any given time, a fraction of TFEB is associated with lysosomes, where it is phosphorylated by MTORC1 and sent back to the cytosol. The recruitment of TFEB to lysosomes is rapid and transient, and at steady-state most TFEB appears freely diffuse in the cytosol. In contrast, under starvation conditions, RRAGs and MTORC1 are inactivated and TFEB is free to be transported to the nucleus and promote expression of autophagic and lysosomal genes ().
Figure 1. Model of the mechanism of TFEB regulation by RRAG GTPases. In nutrient-rich conditions, active RRAGs promote recruitment of MTORC1 and TFEB to the lysosomal membrane. Active MTORC1 phosphorylates TFEB in several residues including S211. Phosphorylation of TFEB at S211 creates a binding site for YWHA (14-3-3). We propose that YWHA may mask the RRAG-binding domain in TFEB (represented in yellow) and subsequently sequester TFEB in the cytosol. Under starvation conditions, RRAG GTPases and MTORC1 are inactivated and TFEB can no longer redistribute to lysosomes. Consequently, the TFEB-YWHA complex dissociates, thus allowing transport of TFEB to the nucleus where it mediates the activation of a transcriptional network that promotes autophagy, lysosomal biogenesis and increased lysosomal degradation. In summary, TFEB localization and regulation are nutrient dependent. In fully fed cells TFEB continuously shuttles between the cytosol and lysosomes, whereas under nutrient-deprivation conditions, TFEB is transported from the cytosol to the nucleus.
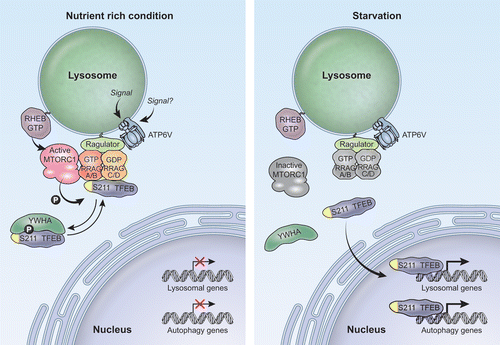
The interaction of TFEB with RRAGs requires the first 30 residues of TFEB and the switch regions of the RRAGs G domain. It is well established that the switch regions of small GTPases undergo profound conformational changes depending on their nucleotide-bound state. This may explain the high specificity of TFEB for active (but not inactive) RRAGs. When the binding of TFEB to RRAGs is prevented (for example by mutation of specific residues in the N-terminal portion of TFEB, depletion or inactivation of endogenous RRAGs, or overexpression of inactive RRAGs) TFEB is no longer able to redistribute to lysosomes, be phosphorylated by MTOR, or bind YWHA. This leads to dysfunctional accumulation of TFEB in the nucleus, and activation of autophagy under nutrient-rich conditions. Therefore, as predicted, recruitment of TFEB to lysosomes is critical for proper regulation of this transcription factor.
Accumulation of TFEB in the nucleus has also been observed under conditions in which lysosomal function is compromised (e.g., after incubation with chloroquine or in several cell models of lysosomal storage disorders), thus suggesting the existence of a pathway that is activated under lysosomal stress conditions. Recent work from the Sabatini lab showed that the lysosomal H+ transporting ATPase (ATP6V) is a component of the MTORC1 pathway that links activation of RRAG GTPases to the concentration of amino acids in the lumen of the lysosome. It is therefore plausible that other stimuli that affect the activity of ATPV6 (such as the changes in lysosomal pH induced by chloroquine) also influence the activation state of RRAGs and, thereby, the distribution of TFEB. Therefore, RRAGs and TFEB may be implicated in a broader pathway of cellular response to lysosomal stress.
Finally, this lysosome-to-nucleus regulatory mechanism is shared by other transcription factors. TFEB belongs to a family of basic helix-loop-helix leucine zipper transcription factors that also includes MITF, TFE3 and TFEC. Sequence alignment reveals a high degree of homology between the N-terminal portion of TFEB and some MITF isoforms including human isoforms 1 and 2, which are required for proliferation and survival of retinal pigment epithelial cells, osteoclasts, natural killer cells and mast cells. Similar to TFEB, MITF isoform 1 interacts with active RRAGs and is retained in the cytosol by MTORC1, thus suggesting a more general role of RRAGs in the regulation of gene expression.
In summary, our work identifies the molecular mechanism by which the RRAG complex provides temporal and spatial coordination of TFEB (and MITF) localization and activation. It also highlights the novel and critical role of lysosomes as key signaling centers that synchronize environmental cues, such as amino acid availability, with gene expression, energy production and cellular homeostasis.
Acknowledgments
This project was supported by the Intramural Research Program of the NIH, National Heart, Lung, and Blood Institute (NHLBI).
Disclosure of Potential Conflicts of Interest
No potential conflicts of interest were disclosed.