Abstract
MTOR, a central regulator of autophagy, is involved in cancer and cardiovascular and neurological diseases. Modulating the MTOR signaling balance could be of great significance for numerous diseases. No chemical activators of MTOR have been found, and the urgent challenge is to find novel MTOR downstream components. In previous studies, we found a chemical small molecule, 3-benzyl-5-((2-nitrophenoxy) methyl)–dihydrofuran-2(3H)-one (3BDO), that inhibited autophagy in human umbilical vein endothelial cells (HUVECs) and neuronal cells. Here, we found that 3BDO activated MTOR by targeting FKBP1A (FK506-binding protein 1A, 12 kDa). We next used 3BDO to detect novel factors downstream of the MTOR signaling pathway. Activation of MTOR by 3BDO increased the phosphorylation of TIA1 (TIA1 cytotoxic granule-associated RNA binding protein/T-cell-restricted intracellular antigen-1). Finally, we used gene microarray, RNA interference, RNA-ChIP assay, bioinformatics, luciferase reporter assay, and other assays and found that 3BDO greatly decreased the level of a long noncoding RNA (lncRNA) derived from the 3′ untranslated region (3′UTR) of TGFB2, known as FLJ11812. TIA1 was responsible for processing FLJ11812. Further experiments results showed that FLJ11812 could bind with MIR4459 targeting ATG13 (autophagy-related 13), and ATG13 protein level was decreased along with 3BDO-decreased FLJ11812 level. Here, we provide a new activator of MTOR, and our findings highlight the role of the lncRNA in autophagy.
Introduction
MTOR (mechanistic target of rapamycin [serine/threonine kinase]/FK506 binding protein 12-rapamycin associated protein 1), controls many cellular processes, including apoptosis, autophagy, translation, energy metabolism, and inflammation.Citation1 Under normal conditions, MTOR activity is regulated by growth factors, insulin, and amino acids.Citation2 Dysfunction of MTOR is a risk factor of various diseases such as Alzheimer disease, cancer, cystic kidney disease, and cardiovascular complications of diabetes. To treat these diseases, pharmacological agents that can regulate MTOR have been developed and are helpful in improving many diseases. Rapamycin and its derivatives (rapalogs), inhibitors of MTOR, have been evaluated for their immunosuppressive and antiproliferative properties and as anticancer agents.Citation3,Citation4 In addition, newly developed pharmaceuticals targeting MTOR may increase MTOR suppression and be useful in associated diseases.Citation5 However, in some cases, inhibition of MTOR has negative effects on certain diseases.Citation6 For example, in normal physiological processes, the RPS6KB1 (ribosomal protein S6 kinase, 70 kDa, polypeptide 1)-mediated negative feedback loop can inhibit growth-factor–induced tumorigenesis. However, suppression of MTOR complex 1 (MTORC1) activity by rapamycin could relieve the RPS6KB1-mediated negative feedback, thus increasing the PI3K-AKT pathway, for increased cell growth, survival, and migration.Citation7 In endothelial progenitor cells, rapamycin at relatively low concentrations increased the rates of apoptosis.Citation8 A decreased MTOR signal pathway with reduced RPS6KB1 activity results in hypoinsulinemia, loss of glucose tolerance, and decreased pancreatic β-cell size.Citation9 These symptoms mentioned above could be relieved by increased MTOR signaling with the resulting increased phosphorylated RPS6KB1 and EIF4EBP1 (eukaryotic translation initiation factor 4E-binding protein 1) in pancreatic βcells in mice.Citation10 The extended use of MTOR kinase inhibitors damages tissues and impairs metabolism.Citation11 A possible avenue to solve the existing problems is the development of small chemical molecules that selectively modulate MTOR kinase. However, to our knowledge, we lack a chemical activator of MTOR.
We have previously found that 3-benzyl-5-((2-nitrophenoxy) methyl)–dihydrofuran-2(3H)-one is a compound with the potential to eliminate chloroquine-induced autophagy in human umbilical vein endothelial cells.Citation12 Subsequently, we have demonstrated that 3BDO suppressed lipopolysaccharide-induced HUVEC autophagic injury by downregulating the protein levels of NUPR1 (nuclear protein, transcriptional regulator, 1) and TP53 (tumor protein p53), TP53 nuclear translocation and reactive oxygen species overproduction.Citation13 Therefore, 3BDO might be beneficial for ameliorating autophagy in cardiovascular diseases. However, the target of 3BDO was not known.
In addition, we studied the effects of 3BDO on autophagy in neuronal cells caused by amyloid-peptide (Aβ). In vitro studies showed that 3BDO inhibited excessive Aβ (25 to 35) peptide-induced autophagy in PC12 neuronal cells and increased the phosphorylation of RPS6KB1.Citation14 In vivo experiments showed that 3BDO had a good safety profile. 3BDO treatment could significantly reduce the number of autophagosomes and improve neuronal function in App and Psen1 transgenic mice.Citation15 So 3BDO may be a useful compound to investigate autophagy in Alzheimer disease. From these and other data, we speculated that 3BDO might activate MTOR as an antagonist of rapamycin.
Despite the advances in delineating the roles of the MTOR signal pathway in autophagy, the current challenge is to find unknown downstream members involved in this signaling. Chemical genetics is an emerging field; small chemical molecules are used to penetrate the cell as probes to affect cell physiological processes. The technique provides new insights into novel factors modulating specific biological processes and has had great impact on diverse areas of cell biology.Citation16
In this study, we used 3BDO to further investigate genes involved downstream of the MTOR signaling pathway. We performed a microarray assay (Table S1) and found that the expression of FLJ11812 (GenBank accession number AK021874.1), located in the 3′ untranslated region (3′UTR) of transforming growth factor, β 2 (TGFB2), was greatly downregulated in response to 3BDO. The microarray assay result of 3BDO effect on the TGFB2 or other TGF family members was also shown in the Table S2. Prior to our work, the change of FLJ11812 expression as a transcript was also observed in many microarray assays,Citation17-Citation26 but its function and action mechanism has not been further studied. In addition, to our knowledge, the relation between autophagy and long noncoding RNAs are largely unclear. Here, we aimed to investigate whether FLJ11812 is an lncRNA, and how it is processed, as well as its detailed mechanism of action in autophagy.
Results
3BDO was targeted to FKBP1A (FK506-binding protein 1A, 12 kDa) and activated MTOR
According to our previous report, the small-molecule compound 3BDO greatly inhibits autophagy in HUVECs,Citation12,Citation13 so we deduced that the 3BDO might activate the MTOR pathway as an antagonist of rapamycin. We used molecular docking to predict whether 3BDO could bind with FKBP1A, the direct target of rapamycin.Citation27,Citation28 3BDO might form hydrogen bonds with TYR82A and ILE56A sites in FKBP1A (), the 2 amino acid sites for rapamycin binding with FKBP1A. Therefore, 3BDO might occupy the rapamycin-binding site and activate the MTOR signaling pathway.
Figure 1. 3BDO failed to activate the MTOR signaling in FKBP1A protein-overexpressed HUVECs. (A) In silico docking of 3BDO into the hydrophobic pocket of FKBP1A and surface view of docked 3BDO-FKBP1A molecule. Hydrogen-bond network of the docked 3BDO-FKBP1A molecule. (B) Western blot analysis of EIF4EBP1, phosphorylated EIF4EBP1 (p-EIF4EBP1, Thr37/46), RPS6KB1, and phosphorylated RPS6KB1 (p-RPS6KB1, Thr389) in HUVECs treated with or without 3BDO (60 μM) for 24 h after overexpression of FKBP1A. (C) Densitometry results of ratio of p-RPS6KB1 to total RPS6KB1 and p-EIF4EBP1 to total EIF4EBP1. pCMV6 phosphorylation was set to 1. *P < 0.05, **P < 0.01, #P > 0.05, n = 3.
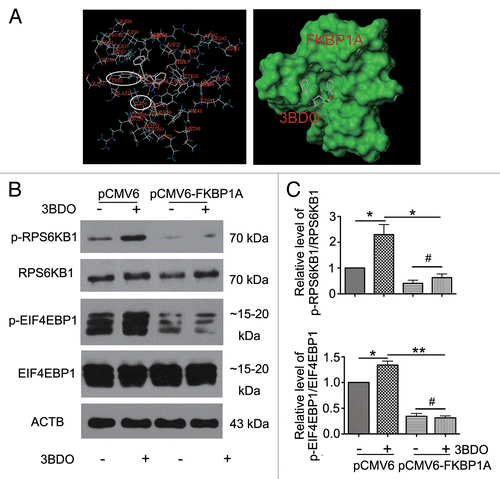
Next, we overexpressed FKBP1A cDNA in HUVECs and treated them with or without 3BDO to detect the phosphorylation of MTOR substrates RPS6KB1 and EIF4EBP1. Phosphorylation of RPS6KB1 and EIF4EBP1 was significantly increased by 3BDO with vector alone but suppressed with FKBP1A overexpression (). Therefore, 3BDO could target FKBP1A and activate the MTOR signaling pathway.
Antagonism between 3BDO and rapamycin in modulation of MTOR and RPS6KB1 phosphorylation and autophagy in HUVECs
To understand the antagonism between 3BDO and rapamycin, we examined the effect of 3BDO on MTOR and RPS6KB1 phosphorylation in the presence or absence of rapamycin. First, we examined the phosphorylation of MTOR (both Ser2448 and Ser2481)Citation29 and RPS6KB1 (p-RPS6KB1; Thr389) in HUVECs treated with 3BDO (60 μM), rapamycin (10 μM) or both for 6 h. Levels of p-MTOR (Ser2448) and p-RPS6KB1 were decreased with rapamycin; however, rapamycin failed to decrease the phosphorylation of MTOR and RPS6KB1 in the presence of 3BDO (). These data revealed that 3BDO directly activated MTOR.
Figure 2. 3BDO inhibited the role of rapamycin in the MTOR pathway and autophagy. (A) Western blot analysis of the phosphorylation of MTOR (Ser2448 and Ser2481) in HUVECs treated with 3BDO (60 μM), rapamycin (10 μM) or both for 6 h. Densitometry results are the ratio of p-MTOR (Ser2448 and Ser2481) to MTOR, with data for the nontreated group set to 1. (B) Western blot analysis of substrates of MTOR signaling in HUVECs, pretreated with or without 3BDO (60 μM) for 30 min, then stimulated with rapamycin (10 μM) for 6 h. (C) Images of MAP1LC3B dots in HUVEC. HUVECs were pretreated with or without 3BDO (60 μM) for 30 min, then stimulated with rapamycin (10 μM) for 6 h. Scale bar: 16 μm. (D) Western blot analysis of MAP1LC3B and SQSTM1 protein levels in HUVEC. HUVECs were pretreated with or without 3BDO (7.5, 15 or 30 μM) for 30 min, then stimulated with rapamycin (10 μM) for 6 h. (E) Densitometry results of ratio of SQSTM1 to ACTB. Ctr data were set to 1. Densitometry results of ratio of MAP1LC3B-II to ACTB. Ctr data were set to 1. *P < 0.05, **P < 0.01, n = 3.
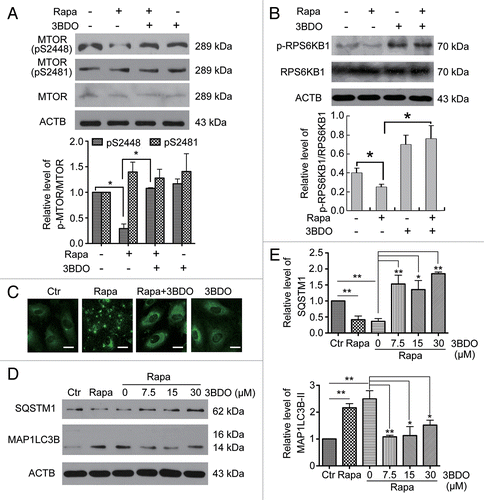
Rapamycin is a well-known pharmacological promoter of autophagy. MAP1LC3B (microtubule-associated protein 1 light chain 3 β) and SQSTM1/p62 (sequestosome 1) are protein markers of autophagy. MAP1LC3B puncta are used to monitor autophagy induction, whereas increased MAP1LC3B-II and decreased SQSTM1 levels are associated with autophagy flux.Citation30 To further confirm the role of 3BDO as an activator of the MTOR signaling pathway, we detected the effect of 3BDO on autophagy induced by rapamycin. First, MAP1LC3B puncta were monitored in HUVECs treated with rapamycin (10 μM) or both 3BDO (60 μM) and rapamycin by immunofluorescence assay. The number of MAP1LC3B puncta was increased with rapamycin, but 3BDO suppressed the increase in MAP1LC3B puncta induced with rapamycin (). Next, we detected the protein levels of SQSTM1 and MAP1LC3B in HUVECs after treatment with rapamycin (10 μM) or both 3BDO (7.5, 15 or 30 μM) and rapamycin. Rapamycin increased the protein level of MAP1LC3B-II and decreased that of SQSTM1, and 3BDO inhibited the effect of rapamycin in HUVECs ().
3BDO promoted TIA1 (TIA1 cytotoxic granule-associated RNA binding protein) phosphorylation in HUVECs
TIA1, a 3′UTR mRNA binding protein, participates in pre-mRNA splicing and inhibits mRNA translation by binding to the 3′UTR of mRNA.Citation30,Citation31 The activity of TIA1 may be more directly modulated by the serine/threonine kinase MTOR.Citation31 To study the effects of rapamycin, 3BDO or both on TIA1 phosphorylation, we analyzed the phosphorylation of Ser residues in TIA1 protein. The phosphorylation of Ser residues was decreased in HUVECs treated with 10 μM rapamycin, and 60 μΜ 3BDO reversed the phosphorylation ().
Figure 3. 3BDO promoted phosphorylation of TIA1 in HUVECs. HUVECs were treated with rapamycin (10 μΜ), 3BDO (60 μΜ) or both for 6 h; immunoprecipitation was obtained with TIA1 antibody. Western blot analysis of phosphorylation of Ser residues of TIA1. Densitometry results of the ratio of p-Ser to TIA1 with data for the nontreated group set to 1. *P < 0.05, n = 3.
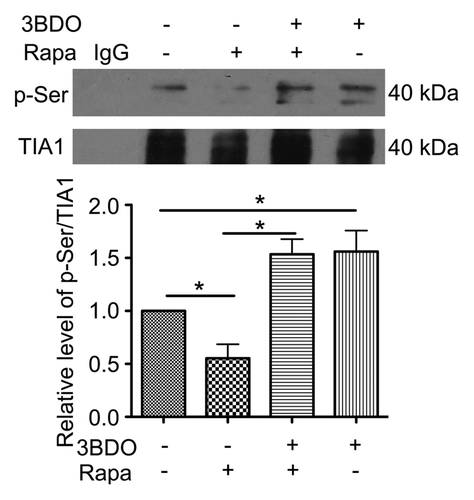
Discovery of FLJ11812 as an lncRNA by using 3BDO
Due to the ability of TIA1 to regulate the expression of mRNA and the fact that 3BDO increased TIA1 phosphorylation, we speculated that 3BDO might affect mRNA levels through TIA1. Therefore, we performed a cDNA microarray assay to determine the transcripts with changed expression with 3BDO treatment in HUVECs. In total, 278 genes showed modified expression with 3BDO treatment: 79 were downregulated and 199 upregulated by more than a 2-fold in response to 60 μM 3BDO for 24 h (). As shown in Table S1, the most significantly downregulated transcript was FLJ11812 (GenBank accession number AK021874.1). The results above showed that 3BDO suppressed the increased MAP1LC3B puncta number, MAP1LC3B-II level and decreased SQSTM1 protein level induced by rapamycin (). Because our previous studies showed that 3BDO protected against chloroquine-induced autophagy,Citation12 we supposed that FLJ11812 might be involved in autophagy.
Figure 4.FLJ11812 found on microarray analysis and was an lncRNA derived from the 3′UTR of TGFB2. (A) Differentially expressed transcripts induced by 60 μM 3BDO for 24 h. The scatter plot depicts genes with > 2-fold change in expression: upregulation (red) and downregulation (green). The arrow shows FLJ11812 with the most downregulated expression. (B) UCSC Genome Bioinformatics Browser data for FLJ11812 transcript on chromosome band 1p41. And gene view of TGFB2 indicating coding sequence (CDS, blue), 3′UTR and location of FLJ11812 (red) according to gene annotation of TGFB2. (C) (Top panel) Positions of probes for TGFB2 and FLJ11812. (Bottom panel) northern blot analysis of FLJ11812 and TGFB2 with specific probes against FLJ11812 or TGFB2. (D) Quantitative PCR (qPCR) analysis of mRNA expression of FLJ11812 and its adjacent subregion in the 3′UTR of TGFB2. (Top panel) Gene view of TGFB2 3′UTR indicating CDS (black), 3′UTR (green) and location of FLJ11812 (yellow) according to gene annotation of TGFB2. (Bottom panel) qPCR analysis of FLJ11812 with a series of corresponding primer pairs. Every span is the subregion of the TGFB2 3′UTR sequence.
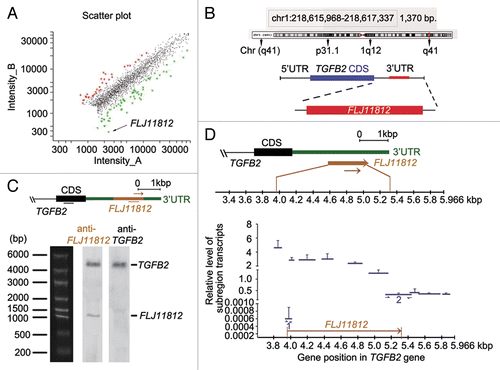
FLJ11812 is located in the 3′UTR of TGFB2 gene, contains 1,370 nt (), and is expressed in the human embryo, with highest expression in the head.Citation32 To confirm whether FLJ11812 is a distinct transcript from TGFB2, we performed northern blot analysis. FLJ11812 was a bona fide transcript (), and exhibited a different band size from TGFB2. We used the TGFB2 probe to label TGFB2 and found that the smaller band observed in the blot probed with FLJ11812 did not contain TGFB2 sequences. To determine the coding capacity of this transcript, we used GENSCAN (http://genes.mit.edu/GENSCAN.html) to assess the sequence. The cDNA sequence contained no coding information. Therefore, FLJ11812 may be a novel lncRNA.
Northern blot analysis can directly detect the presence of RNAs.Citation33 Our northern blot results showed that TGFB2 and FLJ11812 were differentially expressed (). Moreover, sequence alignment (Fig. S1) as well as genome annotations (GenBank accession number AK021874.1) indicated that FLJ11812 was an lncRNA. In addition, for greater understanding of these 2 differentially expressed transcripts, we performed qPCR experiments.Citation33 We designed a series of specific primers as shown in Table S3, the positions of which were before, after, and along the FLJ11812 sequence. We determined the expression of 11 divided subregions spanning about 2100 bp in TGFB2 in HUVECs (). Primers designed to span the 2 putative cleavage sites of FLJ11812 sequence were used for qPCR, and the levels of both transcripts (1 and 2) decreased sharply (). In addition, we applied divided subregions in the FLJ11812 sequence to qPCR and found that the levels of each group were relatively stable and did not significantly differ. These results were consonant with our conclusion of the existence of an lncRNA of about 1300 bp processed from the TGFB2 3′UTR. Therefore, the qPCR experiments could detect a long transcript and estimate its possible size.Citation33
3BDO selectively decreased the level of FLJ11812 but did not affect TGFB2 expression
3BDO could dose- and time-dependently decrease FLJ11812 level in HUVECs (). Because FLJ11812 is derived from the 3′UTR of TGFB2, we detected whether 3BDO affected the expression of TGFB2 but found that it affected neither the mRNA or protein levels (). Therefore, 3BDO may specifically modulate the level of FLJ11812 but not TGFB2.
Figure 5. 3BDO could downregulate FLJ11812 and did not affect TGFB2 expression. (A) Positions of the 2 primers used in qPCR. (B) RT-PCR analysis of the level of FLJ11812 in HUVECs treated with different concentrations of 3BDO for 24 h and with 120 μM 3BDO for various times. (C) qPCR analysis of the mRNA level of TGFB2 in HUVECs treated with concentrations of 3BDO for 24 h and 120 μM 3BDO for various times. (D) Western blot analysis of TGFB2 protein level and quantification. (E and F) qPCR analysis of levels of FLJ11812 and TGFB2 in HUVECs treated with scrambled siRNA or FLJ11812 siRNA for 36 h. *P < 0.05. **P < 0.01, n = 3.
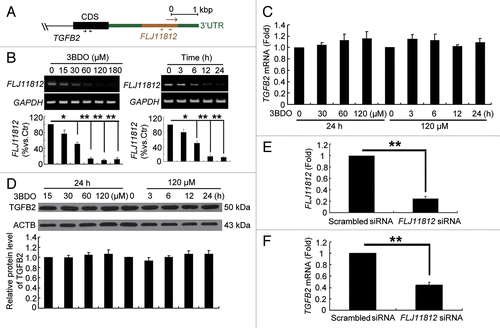
To further confirm the role of FLJ11812 in HUVECs, we used siRNA interference. Because FLJ11812 is derived from the 3′UTR of TGFB2, both FLJ11812 and TGFB2 were knocked down at the same time (). Therefore, 3BDO may be better than siRNA interference for specifically downregulating FLJ11812.
TIA1 was responsible for the processing of FLJ11812
Previous studies have shown that TIA1 acts downstream of MTOR,Citation31 and our results demonstrated that 3BDO increased TIA1 phosphorylation. To determine whether TIA1 participates in the processing of FLJ11812, we used an siRNA interference assay to knock down TIA1 expression (). Knockdown of TIA1 inhibited the level of FLJ11812, with no effect on TGFB2 (). Thus, TIA1 may be involved in the processing of FLJ11812. To validate this suggestion, we performed RNA-chromatin immunoprecipitation (RNA-ChIP) assay. TIA1 interacted directly with the position 1 sequence of the 3′UTR of TGFB2 and 3BDO decreased the interaction between TIA1 and this 3′UTR position of TGFB2 (). In contrast, TIA1 could not bind to position 2. Positions of the 2 primer sequences used are in . Position 1 is the TIA1 binding sequence, and position 2 is the negative control binding sequence.
Figure 6. TIA1 regulated the processing of FLJ11812. (A) Western blot analysis of siRNA knockdown of TIA1. (B and C) qPCR analysis of the mRNA levels of FLJ11812 and TGFB2 in HUVECs with siRNA knockdown of TIA1 for 36 h. **P < 0.01, #P > 0.05, n = 3. (D) HUVECs were treated with DMSO or 120 μM 3BDO for 10 h, then total RNA-protein complexes purification were performed using these cells and underwent ChIP assay. ChIP assays with TIA1 antibody against TIA1 and the fragment that contains part of the FLJ11812 sequence amplified by RT-PCR with an adjusted PCR cycle with specific primer pairs. (E) Positions of the 2 primers used in RNA-ChIP experiments. Position 1 is the TIA1 binding sequence, and position 2 is the negative control sequence.
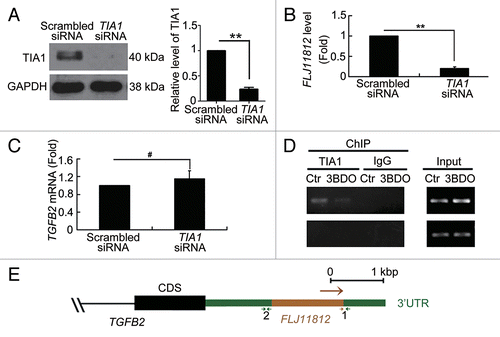
FLJ11812 promoted autophagy in HUVECs
According to the finding that 3BDO selectively depressed the level of FLJ11812 and 3BDO could inhibit autophagy in HUVECs,Citation13 we deduced that FLJ11812 might participate in autophagy regulation. To understand the relationship between FLJ11812 and autophagy, we first investigated the protein level of MAP1LC3B-II after transfection of HUVECs with concentrations of pCMV6-FLJ11812 and pCMV6-XL5 control vectors. The protein level of MAP1LC3B-II was greatly increased in HUVECs with FLJ11812 overexpression (). MAP1LC3B puncta were increased in number in HUVECs treated with pCMV6-FLJ11812 vector, and the proportion of cells containing MAP1LC3B puncta (> 5) was significantly increased with pCMV6-FLJ11812 vector treatment (). These data suggest that FLJ11812 promoted autophagy in HUVECs.
Figure 7.FLJ11812 induced HUVEC autophagy. (A) Western blot analysis of MAP1LC3B protein level in HUVECs transfected with pCMV6 or pCMV6-FLJ11812 for 48 h. (B and C) Immunostaining of MAP1LC3B in HUVECs transfected with pCMV6 or pCMV6-FLJ11812 (0.2 μg/ml) for 48 h, and the percentage of cells containing MAP1LC3B puncta (> 5). Scale bar: 16 μm. Western blot analysis of protein level of (D) MAP1LC3B in HUVECs transfected with pCMV6 or pCMV6-FLJ11812 (0.2 μg/ml) for 42 h, then treated with or without bafilomycin for 6 h; (E) MAP1LC3B in HUVECs transfected with pCMV6 or pCMV6-FLJ11812 (0.2 μg/ml) for 24 h, then treated with or without 3BDO for 24 h; and (F) TGFB2 in HUVECs transfected with pCMV6 and pCMV6-FLJ11812 for 48 h. *P < 0.05, **P < 0.01, #P > 0.05, n = 3.
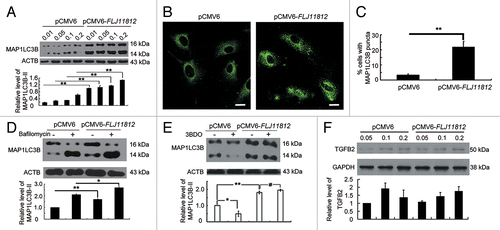
Bafilomycin A1 (BafA1), which prevents the fusion between lysosomes and autophagosomes,Citation34 is often used for measurement of autophagic flux. Overexpression of FLJ11812 and then treatment with BafA1 upregulated MAP1LC3B-II level as compared with pCMV6-FLJ11812 vector alone (). The results indicated that FLJ11812 induced autophagic flux in HUVECs. Moreover, overexpression of FLJ11812 reversed the inhibition of autophagy induced by 3BDO (), which further suggested that the effect of 3BDO on the autophagy suppression was mediated by FLJ11812. Because FLJ11812 is derived from the 3′UTR of TGFB2, we then checked the relation between FLJ11812 and TGFB2. FLJ11812 overexpression did not affect the level of TGFB2 (). Therefore, FLJ11812 promoted autophagy in HUVECs.
FLJ11812 could bind with MIR4459
To further investigate the underlying mechanism of FLJ11812 action, we used bioinformatics to predict the functioning modes of FLJ11812. First, we predicted whether FLJ11812 could produce miRNAs as a precursor (http://www.mirbase.org) but found that FLJ11812 could not generate miRNAs. Next, we analyzed whether FLJ11812 could compete to bind with miRNAs as a miRNA “sponge” and modulate the derepression of miRNA targets. Bioinformatics analysis (http://bioinfo.uni-plovdiv.bg/microinspector/) revealed 57 presumed miRNAs, the sequences of which are highly conserved, which could recognize and bind with FLJ11812. miRNAs whose targets were not expressed or functions were not clear in vascular endothelial cells were excluded. The one eligible miRNA was MIR4459, which has a predicted binding site on FLJ11812 and had the highest score to bind with the 3′UTR of ATG13 (http://www.targetscan.org), a key gene in the regulation of autophagy.Citation33
Next, we re-combined the FLJ11812 cDNA (Luc-FLJ11812-WT) and mutational cDNA with the presumed MIR4459 recognition sequences deleted (Luc-FLJ11812-Δ4459) downstream of the luciferase reporter gene. They were transfected in HEK293 cells along with MIR4459 mimics (). Luciferase activity was reduced by about 20% and 40% compared their control miRNA with 10 and 40 nM MIR4459 mimics transfection, respectively (). When adding mutant substrates for MIR4459, the decreased luciferase activity was abrogated (). Therefore, FLJ11812 could bind with MIR4459.
Figure 8.FLJ11812 is a natural decoy for MIR4459. (A) Potential sites in Luc-FLJ11812 targeted by MIR4459. FLJ11812 fragments and mutant derivatives devoid of MIR4459 binding-site fragments were cloned into the luciferase reporter vector, thus resulting in 2 constructs, Luc-FLJ11812-WT and Luc-FLJ11812-Δ4459 constructs. (B) Luc-FLJ11812-WT plasmid was cotransfected into HEK293 cells with different concentrations of MIR4459 or scrambled miRNA for 24 h and luciferase activity was measured. (C) Luc-FLJ11812-WT and Luc-FLJ11812-Δ4459 plasmids were transfected into HEK293 cells with scrambled miRNA or MIR4459 for 24 h and luciferase activity was measured. (D and E) Potential sites in the 3′UTR of ATG13 targeted by MIR4459. The 3′UTR of ATG13 fragment was cloned into the luciferase reporter vector, resulting in the Luc-ATG13-WT construct. This construct was cotransfected into HEK293 cells with different concentrations of MIR4459 or a control miRNA for 24 h, then luciferase activity was detected. *P < 0.05, **P < 0.01, #P > 0.05, n = 3.
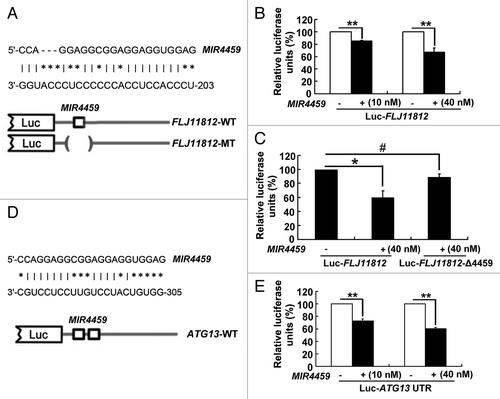
MIR4459 targeted ATG13
Among the putative targets of MIR4459, we concentrated on ATG13 because it could bind with MIR4459 with the highest score and is a key gene in the regulation of autophagy.Citation33 Comparative analysis showed conserved MIR4459-predicted binding sequences within the 3′UTR of ATG13 among mammal cells. Therefore, we fused the 3′UTR of ATG13 with the luciferase coding region (Luc-ATG13-WT, ) and transfected it into HEK293 cells together with MIR4459 in parallel with a control miRNA. Luciferase activity was about 30% and 40% downregulated with expression of the 2 concentrations of MIR4459 as compared with the control miRNA (). Therefore, ATG13 was targeted by MIR4459.
Then, we investigated the effect of FLJ11812 on ATG13 protein level as well as modulation of MIR4459 level. Downregulation of FLJ11812 by 3BDO increased and overexpression of FLJ11812 decreased the level of MIR4459; however, overexpression of the MIR4459-insensitive mutant of FLJ11812 did not affect the level of MIR4459 (). Transfection of HUVECs with MIR4459 mimics decreased and overexpression of FLJ11812 increased the level of ATG13 (). Therefore, FLJ11812, competing to bind with MIR4459, acted as a competing endogenous RNA (ceRNA) to control ATG13 level.
Figure 9.FLJ11812 decreased the level of MIR4459, and MIR4459 suppressed ATG13 protein level. (A) qPCR analysis of MIR4459 level in HUVECs treated with or without 3BDO for 24 h. (B) qPCR analysis of MIR4459 level in HUVECs transfected with pCMV6, pCMV6-FLJ11812 or MIR4459-insensitive mutant of FLJ11812 (Mut-FLJ11812) for 48 h. Western blot analysis of protein level of (C) ATG13 in MIR4459-transduced HUVECs; (D) ATG13 in HUVECs transfected with pCMV6 or pCMV6-FLJ11812 for 48 h (densitometry results are the ratio of ATG13 to ACTB or GAPDH); and (E) p-ATG13 (Ser318) and ATG13 in HUVEC stimulated with rapamycin (10 μM) for 6 h after pretreatment with or without 3BDO for 30 min. *P < 0.05, **P < 0.01, n = 3.
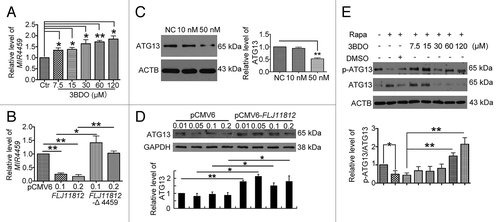
3BDO decreased ATG13 protein level and increased phosphorylation
In mammals, ATG13 is a target of TOR signaling.Citation35,Citation36 ATG13 could form a kinase complex with ULK1 (unc-51 like autophagy activating kinase 1) and RB1CC1/FIP200 (RB1-inducible coiled-coil 1), which is required to initiate autophagy, and the phosphorylation status of ATG13 was regulated by MTOR.Citation36 Therefore, we detected the phosphorylation of ATG13 (p-ATG13, Ser318) and total ATG13 in HUVECs treated with rapamycin (10 μM) or both 3BDO (7.5, 15, 30, 60 or 120 μM) and rapamycin (10 μM). Rapamycin inhibited the level of p-ATG13 as compared with normal controls (, lanes 1 and 2), but on pretreatment with various doses of 3BDO, rapamycin could not inhibit ATG13 phosphorylation (, lanes 7 and 8).
Discussion
MTOR, a Ser/Thr kinase, has an essential role in different tissues and cells. The signaling pathways mediated by MTOR regulate cell proliferation, growth, and metabolism.Citation2 Imbalance of MTOR signal pathways is found in a number of diseases, including cancers, cystic kidney disease, and cardiovascular complications of diabetes.Citation5 Rapamycin as well as newly developed pharmaceuticals targeting MTOR may increase MTOR suppression and be useful in treatment of associated diseases.Citation5 However, in some cases, inhibition of MTOR signaling by rapamycin results in the imbalance of the signals and has many side effects.Citation6 As MTOR-mediated signaling associated with many diseases, pharmacological agents that modulate MTOR signaling could be helpful in improving many diseases. So far, no chemical activators of MTOR have been found. Here, we found a small molecule 3BDO that could dock on FKBP1A in the same sites (TYR82A and ILE56A) as rapamycin. The molecule of 3BDO is smaller than that of rapamycin, so we deduced that 3BDO may occupy rapamycin binding sites easier than rapamycin. Therefore, 3BDO could interdict the role of rapamycin in MTOR pathway, and 3BDO might be a potential drug to treat diseases caused by MTOR pathway disorders.
Although researchers have made many advances in defining the roles of the MTOR signal pathway in autophagy, the current challenge is to find downstream components involved in this signaling. 3BDO may be a useful activator of MTOR for finding new factors downstream of the MTOR signal pathway. In this study, we used 3BDO to find a novel lncRNA FLJ11812 involved in the MTOR signaling pathway. Changes in the transcript level of FLJ11812 have been observed in many microarray assays,Citation17-Citation26 but its function and mechanism were unknown. Here, we demonstrated that FLJ11812 was a bona fide transcript derived from the 3′UTR of TGFB2 but different from TGFB2. It was a key factor in regulating autophagy in HUVECs. In addition, our data indicated that the small-molecule 3BDO could downregulate rapamycin-induced autophagy and the expression of FLJ11812 but not affect the protein and mRNA levels of TGFB2. The specific siRNA against FLJ11812 knocked down FLJ11812 and also affected TGFB2 expression. So, using 3BDO is better than siRNA interference for specific knockdown of FLJ11812.
TIA1, which can bind DNA and RNA, is involved in regulating gene expression at both transcriptional and posttranscriptional levels in eukaryotic cells.Citation37 TIA1 can bind with mRNAs that are adenine/uridine-rich elements (AREs). By binding with these AREs, TIA1 is involved in the selective pre-mRNA splicing of bound mRNAs.Citation38,Citation39 In addition, by binding with the ARE in the 3′UTR of targeted mRNA transcripts, TIA1 represses translation.Citation40,Citation41 Upon amino acid starvation, TIA1 assembles with the 5′ end of 5′TOP mRNAs and represses the 5′TOP mRNA-encoded translation of protein.Citation31 Importantly, inactivation of MTOR is essential for starvation-mediated TIA1 binding with 5′TOP mRNAs,Citation31 which suggests that TIA1 acts downstream of MTOR. However, whether this Ser/Thr kinase of MTOR affects the phosphorylation and activity of TIA1 was unclear. Our data suggest that MTOR activation by 3BDO promoted TIA1 phosphorylation and inhibited the activity of TIA1 for binding with the mRNA.
Furthermore, we first investigated the possible pattern of FLJ11812 processing in this study in light of several considerations. First, TIA1 was regulated by MTOR.Citation31 As well, TIA1 could bind with AREs of mRNA.Citation42 In addition, the sequence of FLJ11812 contains several AREs. Therefore, we speculated that TIA1 regulated the processing of FLJ11812. We found that knockdown of TIA1 greatly suppressed the level of FLJ11812 with no effect on the level of TGFB2 and TIA1 could bind to the 3′UTR of TGFB2 directly, 3BDO inhibited the binding, which suggested that TIA1 regulated the processing of FLJ11812. Many studies show that TIA1 could affect cell proliferation, apoptosis, angiogenesis, invasion, and metastasis, and evasion of immune recognition.Citation43 Our data suggest that TIA1 could regulate autophagy by controlling FLJ11812 processing in HUVECs for the first time.
A recent report supports the conclusion that 3′UTR sequences can function not only to regulate protein expression in cis, but also independently to act as noncoding RNAs (also called uaRNA) in trans. The data suggest that large numbers of uaRNA in human, mouse, and fly undergo post-transcriptional processing.Citation44 FLJ11812 is derived from 3′UTR of TGFB2, and using RNAi and RNA-ChIP analysis, we provide the evidence that FLJ11812 is cleaved by TIA1. In addition, the results of prediction by 3 websites (Neural Network Promoter Prediction; Promoter 2.0 Prediction Server; Promoter and gene expression regulatory motifs search) showed that there was no credible promoter in internal or vicinity of FLJ11812 to control its expression. Therefore, we draw a tentative conclusion that FLJ11812 is processed by post-transcriptional cleavage.
However, qPCR and bioinformatics analyses do not discard the possibility that a promoter is present in the internal or vicinity of FLJ11812 and TIA1 would have an indirect effect linked by post-transcriptional cleavage of 3′UTR of TGFB2 to process FLJ11812. Thus, in our next study, we will conduct the luciferase activity assay to detect whether any promoters exist in the internal or vicinity of FLJ11812 and the capped analysis of gene expression (CAGE) assayCitation44 to further demonstrate the post-transcriptional processing of FLJ11812.
Studies showed that ceRNA, including coding and noncoding ceRNAs, regulate each other by competing to bind with their coincident miRNA.Citation45 ceRNAs can bind and seize miRNAs, which protects their corresponding mRNAs against inhibition. In this paper, we identified an autophagy-related lncRNA, FLJ11812, that showed decoy activity for MIR4459.
At present, finding new noncoding transcripts associated with miRNA and its targeted gene functions is advantageous, the importance of which has been revealed recently.Citation46 This significance was demonstrated initially by the finding that lncMD1 “sponges” MIR133 and MIR135 to regulate the mRNA level of the 2 transcription factors in activating muscle-specific gene expression, which indicates that lncMD1 has an important role in muscle differentiation.Citation47 With these studies, the lncRNA derived from the 3′UTR was found to regulate tumorigenesis and progression.Citation46,Citation48
Among the various predicted targets of MIR4459, we concentrated on ATG13, because it encodes for the protein with an essential role in autophagy. Consistent with FLJ11812 being a decoy for MIR4459, its downregulation by 3BDO increased the level of MIR4459 and reduced the level of ATG13, whereas its overexpression downregulated MIR4459 and increased ATG13 protein level (). Our results are consistent with the previous conclusion that decoy lncRNAs modulate gene expression by binding with miRNAs. ATG13, which associates with ULK1 and RB1CC1 to form a stable protein complex, localizes on the phagophore membrane and is essential for autophagosome formation and then autophagy initiation.Citation33 The identification of the target ATG13 indirectly regulated by FLJ11812 contributes to explaining the autophagy alterations observed with changes in FLJ11812 level.
Many studies show that MTORC1 directly interacts with ULK1 and phosphorylated ULK1 and ATG13 in a nutrient-dependent manner.Citation36 When treated with rapamycin or starvation, ULK1 and ATG13 are dephosphorylated. Consistent with these data, ATG13 phosphorylation was decreased in HUVECs with rapamycin treatment, and 3BDO increased the phosphorylation of ATG13 (), which further demonstrated that 3BDO could antagonize the role of rapamycin during autophagy.
In summary, as shown in , 3BDO could occupy the rapamycin-binding site and blocked the interaction between rapamycin and FKBP1A and activated the MTOR signaling pathway and subsequent TIA1 phosphorylation. TIA1 phosphorylation and the reduced interaction between the 3′UTR of TGFB2 and TIA1 by 3BDO might affect the processing of an lncRNA, FLJ11812. LncRNA FLJ11812, with decoy activity for MIR4459 and by sequestering MIR4459, regulates the level of the MIR4459 target, ATG13. This process is involved in autophagy regulation. LncRNA FLJ11812 is a novel promoter of autophagy, and 3BDO, an activator of MTOR, may be a useful tool against autophagy and to study the downstream MTOR signal pathway. Our findings highlight the role and action mechanism of this lncRNA in autophagy, and 3BDO might be a potential drug to treat diseases caused by disorders of autophagy.
Figure 10. Conceptual schematic of 3BDO and FLJ11812 mechanism of action. 3BDO can occupy the rapamycin-binding site, blocks the interaction of rapamycin and FKBP1A, and activates the MTOR signaling pathway and subsequent TIA1 phosphorylation. TIA1 phosphorylation and the reduced interaction between the 3′ UTR of TGFB2 and TIA1 by 3BDO might affect the production of an lncRNA, FLJ11812. FLJ11812, with decoy activity for MIR4459 and by sequestering MIR4459, regulates the level of MIR4459 target, ATG13, and autophagy.
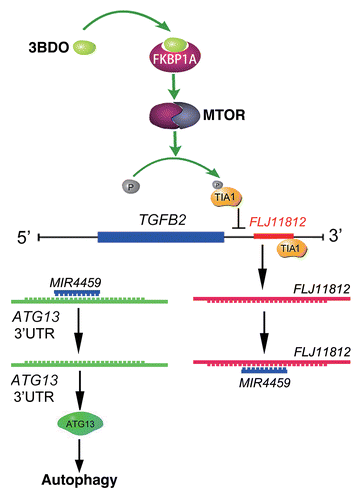
Materials and Methods
Cell culture and treatment
HUVECs were isolated from umbilical cords as described,Citation49 then cultured in M199 medium (Gibco, 31100-035) with 20% (v/v) fetal bovine serum (Hyclone, SV30087.02) and 10 IU/mL fibroblast growth factor 2 (FGF2) in a humidified incubator at 37 °C with 5% CO2. Cells up to passage 10 were used for experiments. When HUVECs were grown to 80% confluency, cells were treated with or without 3BDO.
Molecular docking
Three-dimensional coordinates of the FKBP1A structure (PBD ID: 2PPN; 1FAP) were obtained from the Protein Data Bank (PDB). SYBYL software (Sybyl-X 1.1.083; Tripos, St. Louis, MO) was used to dock 3BDO to the pocket site of FKBP1A to examine possible binding modes of 3BDO. All docking conformations were ranked according to the CScore, and the best-ranked results (CScore 5) was checked visually.
TIA1 phosphorylation detection
Total protein was obtained from HUVECs by use of IP lysis buffer (Beyotime, P0013) after treatment with rapamycin (10 μM), 3BDO (60 μM) or both for 6 h. After centrifuging at 4 °C, the supernatant was collected and incubated with protein A/G agarose beads (Beyotime, P2012) and TIA1 antibody (sc-365349) or normal mouse IgG as a control (both Santa Cruz Biotechnology, sc-2025) at 4 °C overnight. The beads were washed 3 times with IP lysis buffer and then eluted with 4 × SDS loading buffer. Ser phosphorylation was detected by western blot assay with Ser phosphorylation antibody (Santa Cruz Biotechnology, sc-81514).
Microarray analysis
HUVECs were treated with DMSO or 60 µM 3BDO for 24 h, then total RNA was extracted with use of Trizol reagent (Invitrogen, 15596018). The cDNA microarray assay in response to 3BDO treatment was performed by CapitalBio Corp. (Beijing). The microarray process was as described.Citation50
Northern blot analysis
Northern blot analysis involved use of the NorthernMax Kit (Ambion, AM1940). Total RNA was extracted as described previously. RNA was fractionated by formaldehyde agarose electrophoresis, then transferred to Hybond-N+ nylon membranes (Amersham, 137N). FLJ11812 and TGFB2 expression was detected by use of digoxin (DIG)-labeled probes described below that corresponded to the sequence of FLJ11812. Hybridization was visualized by autoradiography with Kodak BIOMax-XR film.
Generation of RNA probes
The probes against FLJ11812 and TGFB2 used in northern blot analysis were RNA probes and strand-specific. We designed specific primer pairs for RT-PCR with the pCMV6-FLJ11812 and pCMV6-TGFB2 template. Some of the PCR products were cloned into the polylinker site of a pEASY-T3 cloning vector, the sequences of which contain promoters for T7 and SP6. Then vectors were transformed into Trans1-T1-competent cells. Blue white spot screening was used to detect recombinant clones. Clones were cultured in LB liquid medium overnight and plasmids were extracted. After identification of the orientation of the goal sequence, we used these plasmids for the transcription template and with T7/SP6 RNA polymerase, the DIG-labeled RNA probe was transcribed. The primer pair sequences for TGFB2, forward, 5′-CTACAACAGC ACCAGGGACT-3′, and reverse, 5′-GCCATCAATA CCTGCAAATC-3′; and FLJ11812, forward 5′-GCAGTTTCAC CTAAAGAGCA GC-3′, and reverse, 5′-TTCCTTCCCA CCTCCACCC-3′. The positions of probes of TGFB2 and FLJ11812 are shown in .
Immunofluorescence assay
Immunofluorescence assay was performed as described.Citation51 In brief, treated HUVECs were fixed with 4% paraformaldehyde for 15 min and blocked with 3% normal goat serum or rabbit serum for 20 min at room temperature, incubated with MAP1LC3B primary antibody at 4 °C overnight, then a corresponding FITC-conjugated secondary antibody (1:200; Sangon Biotech, AB10051) at 37 °C for 1 h. Cells were rinsed 3 times with 0.1 mol/L phosphate-buffered saline (PBS; 137 mM NaCl, 2.7 mM KCl, 10 mM Na2HPO4, 2 mM KH2PO4) and evaluated by laser-scanning confocal microscopy (Leica, DMIRE2, Wetzlar, Germany).
Quantitative real-time PCR
Total RNA was extracted from HUVECs by the TRIzol reagent method (Invitrogen, 15596018). The reverse transcription step involved use of oligo(dT) primers, and then underwent quantitative real-time PCR (Roche, Light Cycler 2.0 system,Basel, Switzerland) with the primer pair sequences for TGFB2, forward, 5′-CGCCAAGGAG GTTTACAAAA-3′, and reverse, 5′-AATCCGTTGT TCAGGCACTC-3′; and GAPDH, forward, 5′-ACCACAGTCC ATGCCATCAC-3′ and reverse, 5′-TCCACCACCC TGTTGCTGTA-3′, as a housekeeping gene. Relative gene expression was normalized to GAPDH levels. Quantitative PCR (qPCR) reactions involved use of the QuantiTect SYBR Green PCR kit (QIAgen, 204143) and LightCycler 2.0 system (Roche). Reactions were performed in a 25-μl volume with 12.5 μl of 2× SYBR Green PCR Master Mix. The fold change in RNA level was calculated by the 2-ΔΔCt method with MxPro 4.00 (Stratagene).
One-step qPCR and RT-PCR for FLJ11812
In extracted total RNA, the level of FLJ11812 was detected by real-time PCR and RT-PCR by use of the One Step SYBR PrimeScript RT-PCR Kit II (TaKaRa, DRR086A) and PrimeScript RT reagent Kit with gDNA Eraser (TaKaRa, DRR047A), respectively. The primer pair sequences were for FLJ11812, forward 5′-GCAGTTTCAC CTAAAGAGCA GC-3′, and reverse, 5′-TTCCTTCCCA CCTCCACCC-3′; and GAPDH, forward, 5′-ACCACAGTCC ATGCCATCAC-3′, and reverse, 5′-TCCACCACCC TGTTGCTGTA-3′ as a housekeeping gene. Relative gene expression was normalized to GAPDH levels. The fold change in RNA level was calculated by the 2-ΔΔCt method with MxPro 4.00 (Stratagene). shows the positions of primers for TGFB2 and FLJ11812 used in qPCR.
Vector construction and gene overexpression
The pCMV6-FLJ11812 vector was generated by inserting the full-length FLJ11812 sequence into the pCMV6-XL5 vector at EcoRI and XbaI restriction points, with pCMV6-XL5 as a control. The GFP-tagged open reading frame clone of Homo sapiens FKBP1A was purchased from OriGene Technologies (RG201237). Transient transfection involved the LipofectamineTM 2000 method (Invitrogen, 11668-019). HUVECs were transiently transfected with the desired vectors for 4 to 6 h and cultured for 24 or 48 h, then treated with or without 3BDO (60 μM). The transfection of FKBP1A into HUVECs was confirmed by fluorescence microscopy.
Transient transfection and RNA interference
Specific siRNA against FLJ11812 was synthesized by Invitrogen. Cells at 80% confluence were transfected with siRNA against FLJ11812 (40 nM) by the RNAiFect Transfection Reagent method (QIAGEN, 301605). Scrambled siRNA was a control (Santa Cruz Biotechnology, sc-37007). After 24 h, siRNA in the medium was substituted with normal M199 medium with FGF-2. Cells were cultured for another 24 h for the desired assays.
Western blot analysis
HUVECs were lysed in lysis buffer containing 25 mM TRIS-HCl (pH 6.8), 2% SDS, 6% glycerol, 1% 2-mercaptoethanol, 2 mM PMSF, 0.2‰ bromophenol blue, and a protease inhibitor cocktail (Sigma, P8340) for 10 min at room temperature and boiled for another 10 min. Equal amounts of extracted total protein (15 μg) underwent 15% SDS-PAGE and were electroblotted onto polyvinylidene difluoride membranes (Millipore, IPVH00010), which were blocked with 5% (w/v) nonfat dry milk in PBS-Tween 20 (PBST; 0.05%) for 1 h and incubated with RPS6KB1 (9202), p-RPS6KB1 (9206), EIF4EBP1 (9452), p-EIF4EBP1 (2855), MAP1LC3B (2775), p-MTOR (Ser2448; 2971), and p-MTOR (Ser2481, 2974; all Cell Signaling Technology); p-ATG13 (Rockland, 27918); ATG13 (Sigma, SAB4200100); SQSTM1/p62 (BD, 610833); MTOR (sc-8319), TGFB2 (sc-90), ACTB (sc-47778), GAPDH (sc-47724), and TIA1 (all Santa Cruz Biotechnology, sc-166247); or primary antibody (1:1000 in PBST) at 4 °C overnight. After 3 washes in PBST, membranes were incubated with appropriate horseradish peroxidase-conjugated secondary antibodies (1:5000; Santa Cruz Biotechnology, sc-2004 [goat anti-rabbit IgG-HRP] and sc-2302 [goat anti-mouse IgG-HRP]) for 1 h at room temperature. The immunoreactive bands were developed with enhanced chemiluminessence (Thermo Fisher, 34080). ACTB or GAPDH was used as loading control. The relative quantity of proteins was analyzed by use of ImageJ.
RNA/protein immunoprecipitation (IP) assay
RNA-IP involved use of the RNA ChIP-IT Kit (Motif, 53024). HUVECs were treated with or without 120 μM 3BDO for 10 h, then fixed and collected in PBS containing PMSF, complete protease inhibitor tablet and the RNase inhibitor. Cells were pelleted by centrifugation for 10 min at 3,500 rpm and resuspended with lysis buffer, then chromatin was sheared with the appropriate conditions. After spinning at 18,000 g for 15 min, supernatant was treated with DNase I and then used for precipitation of the TIA1-RNA complexes with TIA1 antibodies (sc-166247) or control mouse IgG (both Santa Cruz Biotechnology, sc-2025). Coprecipitated RNA was purified with TRIZOL reagent, treated with DNase I. RNA was reverse transcribed to cDNA, which was amplified in real-time PCR with an adjusted PCR cycle (TaKaRa, DRR086A). show positions of the 2 primers used in RNA-ChIP experiments. The position 1 is the TIA1 binding sequence, and position 2 is the negative control sequence. The primer pair for position 1 was 5′-TTTCGATGAT ATGTGGATGT CT-3′, 5′-TTGCATCACA GGTGTTTTCT-3′; position 2, 5′- GATTGACTTA AATTTGGGCT CTT-3′, 5′-GCATTTGTAG TGCAAGTCAA ACTA-3′.
Construct generation
A luciferase reporter vector (pmirGLO Dual-Luciferase miRNA Target Expression Vector; Promega) was used to generate the luciferase constructs. The FLJ11812 and ATG13 3′UTR were cloned by RT-PCR. The PCR products were digested with SacI-HF and XbaI and the fragment was inserted into a SacI-HF and XbaI-digested pmirGLO Dual-Luciferase miRNA Target Luciferase plasmid (Promega, E177A) to obtain different luciferase constructs. A mutant construct was generated by a similar approach. Similarly, the PCR products were digested with SacI-HF and XbaI, and the fragment was inserted into a SacI-HF and XbaI-digested pmirGLO Dual-Luciferase miRNA Target Luciferase plasmid to obtain different mutant luciferase constructs.
Luciferase activity assays
Luciferase activity assays involving a dual-luciferase reporter system were conducted according to the protocol provided by Promega (Promega, E2920). In brief, HEK293 cells were seeded onto 96-well tissue culture plates at 6000 cells per well in medium containing 10% fetal bovine serum and incubated overnight. Cells were cotransfected with Dual-Luciferase (containing firefly and Renilla luciferase) reporter constructs and corresponding miRNA mimics, 10 and 40 nM, by use of Lipofectamine 2000. Cells were then lysed with 75 μl Dual-Glo Luciferase Reagent (Promega, E2920) per well and placed on a shaker for 30 min; firefly luciferase activities were measured by use of a luminometer (Infinite 200 PRO, TECAN, Männedorf, Switzerland). For the internal control, 75 μl Dual-Glo Stop and Glo Reagent (Promega, E2920) was added. After a shaking for 30 min, Renilla luciferase activities were measured in the same tube. Luciferase activities after different treatments were to that of Renilla luciferase activities.
Quantitative real-time PCR for miRNA
RNA was isolated with the Trizol reagent method. RNA was quantified by use of the NanoDrop. miRNA was converted into cDNA with use of TaqMan MicroRNA Reverse Transcription Kit (Applied Biosystems, PN4366596) and specific miRNA primers. The KAPA SYBR FAST qPCR Kits (Kapa Biosystems, KK4601) was used to quantify the expression of mature MIR4459 in HUVECs. Quantitative real-time PCR reactions involved use of the LightCycler 480 Real-Time PCR System (Roche, Basel, Switzerland). Following a single cycle at 95 °C for 10 min, PCR involved 40 cycles at 95 °C for 30 s and 60 °C for 1 min. U6 was the control for small RNAs. Primers used in this study were for MIR4459, (stem-loop primer) 5′-GTCGTATCCA GTGCAGGGTC CGAGGTATTC GCACTGGATA CGACTCCACC T-3′, (pcr primer) 5′-ATTATCCAGG AGGCGGAGG-3′, 5′-TCGTATCCAG TGCAGGGTC-3′; and U6, 5′-CTCGCTTCGG CAGCACATAT ACT-3′, 5′-ACGCTTCACG AATTTGCGTG TC-3′. miRNA expression was calculated as relative to U6 expression, by independent triplicate measurements.
Transfection with MIR4459 mimics
RNA mimics for MIR4459 and negative control (NC) were designed and purchased from Invitrogen. The mimics for MIR4459 and NC were transfected into HUVECs with use of Lipofectamine 2000 (Invitrogen, 11668-019) for incubation for 48 h. The final concentrations of the mimics and NC were 10 or 50 nM.
Statistical analysis
Densitometry results of western blot and RT-PCR and numbers of MAP1LC3B puncta were acquired by using ImageJ 1.47 (National Institutes of Health, USA). All data were presented as means ± SEM and analyzed by SPSS 11.0 (SPSS Inc., Chicago, IL). Images were processed by use of Graphpad Prism 5 (GraphPad Software, Inc. La Jolla, CA, USA) and Adobe Photoshop CS3 (Adobe, San Jose, USA). Mean values were derived from at least 3 independent experiments. Differences at P < 0.05 were considered statistically significant.
Abbreviations: | ||
3′UTR | = | 3′ untranslated region |
3BDO | = | 3-benzyl-5-((2-nitrophenoxy) methyl)-dihydrofuran-2(3H)-one |
AREs | = | adenine/uridine-rich elements |
ATG13 | = | autophagy-related 13 |
App | = | amyloid beta (A4) precursor protein |
BafA1 | = | bafilomycin A1, FKBP1A, FK506-binding protein 1A, 12 kDa |
HUVECs | = | human umbilical vein endothelial cells |
lncRNA | = | long noncoding RNA |
MAP1LC3B | = | microtubule-associated protein 1 light chain 3 beta |
MTOR | = | mechanistic target of rapamycin (serine/threonine kinase) |
MTORC1 | = | MTOR complex 1 |
NUPR1 | = | nuclear protein, transcriptional regulator, 1 |
Psen1 | = | presenilin 1 |
RPS6KB1 | = | ribosomal protein S6 kinase, 70 kDa, polypeptide 1 |
SQSTM1 | = | sequestosome 1 |
TP53 | = | tumor protein p53 |
TGFB2 | = | transforming growth factor, beta 2 |
TIA1 | = | TIA1 cytotoxic granule-associated RNA binding protein |
Additional material
Download Zip (209.2 KB)Disclosure of Potential Conflicts of Interest
No potential conflicts of interest were disclosed.
Acknowledgments
This work was financially supported by the National Natural Science Foundation of China (No. 31270877, 81321061, 91313303, 20972088, and 31070735), the National 973 Research Project (No. 2011CB503906), the Science and Technology Development Project of Shandong Province (2008GG10002034) and the Independent Innovation Foundation of Shandong University (2009JC007, 2009GN033, 2009TS083).
Reference
- Mizumura K, Cloonan SM, Haspel JA, Choi AM. The emerging importance of autophagy in pulmonary diseases. Chest 2012; 142:1289 - 99; http://dx.doi.org/10.1378/chest.12-0809; PMID: 23131937
- Hay N, Sonenberg N. Upstream and downstream of mTOR. Genes Dev 2004; 18:1926 - 45; http://dx.doi.org/10.1101/gad.1212704; PMID: 15314020
- Tsang CK, Qi H, Liu LF, Zheng XF. Targeting mammalian target of rapamycin (mTOR) for health and diseases. Drug Discov Today 2007; 12:112 - 24; http://dx.doi.org/10.1016/j.drudis.2006.12.008; PMID: 17275731
- Zaytseva YY, Valentino JD, Gulhati P, Evers BM. mTOR inhibitors in cancer therapy. Cancer Lett 2012; 319:1 - 7; http://dx.doi.org/10.1016/j.canlet.2012.01.005; PMID: 22261336
- Weichhart T. Mammalian target of rapamycin: a signaling kinase for every aspect of cellular life. Methods Mol Biol 2012; 821:1 - 14; http://dx.doi.org/10.1007/978-1-61779-430-8_1; PMID: 22125056
- Johnson SC, Rabinovitch PS, Kaeberlein M. mTOR is a key modulator of ageing and age-related disease. Nature 2013; 493:338 - 45; http://dx.doi.org/10.1038/nature11861; PMID: 23325216
- Alayev A, Holz MK. mTOR signaling for biological control and cancer. J Cell Physiol 2013; 228:1658 - 64; http://dx.doi.org/10.1002/jcp.24351; PMID: 23460185
- Lei FR, Li XQ, Liu H, Zhu RD, Meng QY, Rong JJ. Rapamycin and 3-methyladenine regulate apoptosis and autophagy in bone-derived endothelial progenitor cells. Chin Med J (Engl) 2012; 125:4076 - 82; PMID: 23158146
- Pende M, Kozma SC, Jaquet M, Oorschot V, Burcelin R, Le Marchand-Brustel Y, Klumperman J, Thorens B, Thomas G. Hypoinsulinaemia, glucose intolerance and diminished beta-cell size in S6K1-deficient mice. Nature 2000; 408:994 - 7; http://dx.doi.org/10.1038/35050135; PMID: 11140689
- Hamada S, Hara K, Hamada T, Yasuda H, Moriyama H, Nakayama R, Nagata M, Yokono K. Upregulation of the mammalian target of rapamycin complex 1 pathway by Ras homolog enriched in brain in pancreatic beta-cells leads to increased beta-cell mass and prevention of hyperglycemia. Diabetes 2009; 58:1321 - 32; http://dx.doi.org/10.2337/db08-0519; PMID: 19258434
- Laplante M, Sabatini DM. mTOR signaling in growth control and disease. Cell 2012; 149:274 - 93; http://dx.doi.org/10.1016/j.cell.2012.03.017; PMID: 22500797
- Huang B, Meng N, Zhao B, Zhao J, Zhang Y, Zhang S, Miao J. Protective effects of a synthesized butyrolactone derivative against chloroquine-induced autophagic vesicle accumulation and the disturbance of mitochondrial membrane potential and Na+,K+-ATPase activity in vascular endothelial cells. Chem Res Toxicol 2009; 22:471 - 5; http://dx.doi.org/10.1021/tx8002824; PMID: 19199859
- Meng N, Zhao J, Su L, Zhao B, Zhang Y, Zhang S, Miao J. A butyrolactone derivative suppressed lipopolysaccharide-induced autophagic injury through inhibiting the autoregulatory loop of p8 and p53 in vascular endothelial cells. Int J Biochem Cell Biol 2012; 44:311 - 9; http://dx.doi.org/10.1016/j.biocel.2011.11.001; PMID: 22085531
- Yang S, Wang S, Peng N, Xie Z, Wang P, Zhao C, Wei L, Yang H, Zhao B, Miao J, et al. Butyrolactone derivative 3-benzyl-5-((2-nitrophenoxy) methyl)-dihydrofuran-2(3H)-one protects against amyloid-β peptides-induced cytotoxicity in PC12 cells. J Alzheimers Dis 2012; 28:345 - 56; PMID: 21988929
- Wei L, Yang H, Xie Z, Yang S, Yang H, Zhao C, Wang P, Xu S, Miao J, Zhao B, et al. A butyrolactone derivative 3BDO alleviates memory deficits and reduces amyloid-β deposition in an AβPP/PS1 transgenic mouse model. J Alzheimers Dis 2012; 30:531 - 43; PMID: 22451314
- Spring DR. Chemical genetics to chemical genomics: small molecules offer big insights. Chem Soc Rev 2005; 34:472 - 82; http://dx.doi.org/10.1039/b312875j; PMID: 16137160
- Bossé Y, Postma DS, Sin DD, Lamontagne M, Couture C, Gaudreault N, Joubert P, Wong V, Elliott M, van den Berge M, et al. Molecular signature of smoking in human lung tissues. Cancer Res 2012; 72:3753 - 63; http://dx.doi.org/10.1158/0008-5472.CAN-12-1160; PMID: 22659451
- Busch W, Kühnel D, Schirmer K, Scholz S. Tungsten carbide cobalt nanoparticles exert hypoxia-like effects on the gene expression level in human keratinocytes. BMC Genomics 2010; 11:65; http://dx.doi.org/10.1186/1471-2164-11-65; PMID: 20105288
- Terragni J, Graham JR, Adams KW, Schaffer ME, Tullai JW, Cooper GM. Phosphatidylinositol 3-kinase signaling in proliferating cells maintains an anti-apoptotic transcriptional program mediated by inhibition of FOXO and non-canonical activation of NFkappaB transcription factors. BMC Cell Biol 2008; 9:6; http://dx.doi.org/10.1186/1471-2121-9-6; PMID: 18226221
- Pluvinet R, Olivar R, Krupinski J, Herrero-Fresneda I, Luque A, Torras J, Cruzado JM, Grinyó JM, Sumoy L, Aran JM. CD40: an upstream master switch for endothelial cell activation uncovered by RNAi-coupled transcriptional profiling. Blood 2008; 112:3624 - 37; http://dx.doi.org/10.1182/blood-2008-03-143305; PMID: 18669876
- van der Heul-Nieuwenhuijsen L, Hendriksen PJ, van der Kwast TH, Jenster G. Gene expression profiling of the human prostate zones. BJU Int 2006; 98:886 - 97; http://dx.doi.org/10.1111/j.1464-410X.2006.06427.x; PMID: 16978289
- Zhen G, Park SW, Nguyenvu LT, Rodriguez MW, Barbeau R, Paquet AC, Erle DJ. IL-13 and epidermal growth factor receptor have critical but distinct roles in epithelial cell mucin production. Am J Respir Cell Mol Biol 2007; 36:244 - 53; http://dx.doi.org/10.1165/rcmb.2006-0180OC; PMID: 16980555
- Di X, Andrews DM, Tucker CJ, Yu L, Moore AB, Zheng X, Castro L, Hermon T, Xiao H, Dixon D. A high concentration of genistein down-regulates activin A, Smad3 and other TGF-β pathway genes in human uterine leiomyoma cells. Exp Mol Med 2012; 44:281 - 92; http://dx.doi.org/10.3858/emm.2012.44.4.024; PMID: 22228119
- Ganti R, Hunt RC, Parapuram SK, Hunt DM. Vitreous modulation of gene expression in low-passage human retinal pigment epithelial cells. Invest Ophthalmol Vis Sci 2007; 48:1853 - 63; http://dx.doi.org/10.1167/iovs.06-0198; PMID: 17389521
- Kim YC, Wu Q, Chen J, Xuan Z, Jung YC, Zhang MQ, Rowley JD, Wang SM. The transcriptome of human CD34+ hematopoietic stem-progenitor cells. Proc Natl Acad Sci U S A 2009; 106:8278 - 83; http://dx.doi.org/10.1073/pnas.0903390106; PMID: 19416867
- Shields JM, Thomas NE, Cregger M, Berger AJ, Leslie M, Torrice C, Hao H, Penland S, Arbiser J, Scott G, et al. Lack of extracellular signal-regulated kinase mitogen-activated protein kinase signaling shows a new type of melanoma. Cancer Res 2007; 67:1502 - 12; http://dx.doi.org/10.1158/0008-5472.CAN-06-3311; PMID: 17308088
- Huang S, Bjornsti MA, Houghton PJ. Rapamycins: mechanism of action and cellular resistance. Cancer Biol Ther 2003; 2:222 - 32; http://dx.doi.org/10.4161/cbt.2.3.360; PMID: 12878853
- Huang S, Houghton PJ. Mechanisms of resistance to rapamycins. Drug Resist Updat 2001; 4:378 - 91; http://dx.doi.org/10.1054/drup.2002.0227; PMID: 12030785
- Copp J, Manning G, Hunter T. TORC-specific phosphorylation of mammalian target of rapamycin (mTOR): phospho-Ser2481 is a marker for intact mTOR signaling complex 2. Cancer Res 2009; 69:1821 - 7; http://dx.doi.org/10.1158/0008-5472.CAN-08-3014; PMID: 19244117
- Klionsky DJ, Abdalla FC, Abeliovich H, Abraham RT, Acevedo-Arozena A, Adeli K, Agholme L, Agnello M, Agostinis P, Aguirre-Ghiso JA, et al. Guidelines for the use and interpretation of assays for monitoring autophagy. Autophagy 2012; 8:445 - 544; http://dx.doi.org/10.4161/auto.19496; PMID: 22966490
- Damgaard CK, Lykke-Andersen J. Translational coregulation of 5’TOP mRNAs by TIA-1 and TIAR. Genes Dev 2011; 25:2057 - 68; http://dx.doi.org/10.1101/gad.17355911; PMID: 21979918
- Ota T, Suzuki Y, Nishikawa T, Otsuki T, Sugiyama T, Irie R, Wakamatsu A, Hayashi K, Sato H, Nagai K, et al. Complete sequencing and characterization of 21,243 full-length human cDNAs. Nat Genet 2004; 36:40 - 5; http://dx.doi.org/10.1038/ng1285; PMID: 14702039
- Alers S, Löffler AS, Wesselborg S, Stork B. The incredible ULKs. Cell Commun Signal 2012; 10:7; http://dx.doi.org/10.1186/1478-811X-10-7; PMID: 22413737
- Yamamoto A, Tagawa Y, Yoshimori T, Moriyama Y, Masaki R, Tashiro Y. Bafilomycin A1 prevents maturation of autophagic vacuoles by inhibiting fusion between autophagosomes and lysosomes in rat hepatoma cell line, H-4-II-E cells. Cell Struct Funct 1998; 23:33 - 42; http://dx.doi.org/10.1247/csf.23.33; PMID: 9639028
- Mercer CA, Kaliappan A, Dennis PB. A novel, human Atg13 binding protein, Atg101, interacts with ULK1 and is essential for macroautophagy. Autophagy 2009; 5:649 - 62; http://dx.doi.org/10.4161/auto.5.5.8249; PMID: 19287211
- Hosokawa N, Hara T, Kaizuka T, Kishi C, Takamura A, Miura Y, Iemura S, Natsume T, Takehana K, Yamada N, et al. Nutrient-dependent mTORC1 association with the ULK1-Atg13-FIP200 complex required for autophagy. Mol Biol Cell 2009; 20:1981 - 91; http://dx.doi.org/10.1091/mbc.E08-12-1248; PMID: 19211835
- Aroca A, Díaz-Quintana A, Díaz-Moreno I. A structural insight into the C-terminal RNA recognition motifs of T-cell intracellular antigen-1 protein. FEBS Lett 2011; 585:2958 - 64; http://dx.doi.org/10.1016/j.febslet.2011.07.037; PMID: 21846467
- Förch P, Puig O, Kedersha N, Martínez C, Granneman S, Séraphin B, Anderson P, Valcárcel J. The apoptosis-promoting factor TIA-1 is a regulator of alternative pre-mRNA splicing. Mol Cell 2000; 6:1089 - 98; http://dx.doi.org/10.1016/S1097-2765(00)00107-6; PMID: 11106748
- Förch P, Valcárcel J. Molecular mechanisms of gene expression regulation by the apoptosis-promoting protein TIA-1. Apoptosis 2001; 6:463 - 8; http://dx.doi.org/10.1023/A:1012441824719; PMID: 11595836
- Dixon DA, Balch GC, Kedersha N, Anderson P, Zimmerman GA, Beauchamp RD, Prescott SM. Regulation of cyclooxygenase-2 expression by the translational silencer TIA-1. J Exp Med 2003; 198:475 - 81; http://dx.doi.org/10.1084/jem.20030616; PMID: 12885872
- Piecyk M, Wax S, Beck AR, Kedersha N, Gupta M, Maritim B, Chen S, Gueydan C, Kruys V, Streuli M, et al. TIA-1 is a translational silencer that selectively regulates the expression of TNF-alpha. EMBO J 2000; 19:4154 - 63; http://dx.doi.org/10.1093/emboj/19.15.4154; PMID: 10921895
- López de Silanes I, Galbán S, Martindale JL, Yang X, Mazan-Mamczarz K, Indig FE, Falco G, Zhan M, Gorospe M. Identification and functional outcome of mRNAs associated with RNA-binding protein TIA-1. Mol Cell Biol 2005; 25:9520 - 31; http://dx.doi.org/10.1128/MCB.25.21.9520-9531.2005; PMID: 16227602
- Izquierdo JM, Alcalde J, Carrascoso I, Reyes R, Ludeña MD. Knockdown of T-cell intracellular antigens triggers cell proliferation, invasion and tumour growth. Biochem J 2011; 435:337 - 44; http://dx.doi.org/10.1042/BJ20101030; PMID: 21284605
- Mercer TR, Wilhelm D, Dinger ME, Soldà G, Korbie DJ, Glazov EA, Truong V, Schwenke M, Simons C, Matthaei KI, et al. Expression of distinct RNAs from 3′ untranslated regions. Nucleic Acids Res 2011; 39:2393 - 403; http://dx.doi.org/10.1093/nar/gkq1158; PMID: 21075793
- Salmena L, Poliseno L, Tay Y, Kats L, Pandolfi PP. A ceRNA hypothesis: the Rosetta Stone of a hidden RNA language?. Cell 2011; 146:353 - 8; http://dx.doi.org/10.1016/j.cell.2011.07.014; PMID: 21802130
- Fang L, Du WW, Yang X, Chen K, Ghanekar A, Levy G, Yang W, Yee AJ, Lu WY, Xuan JW, et al. Versican 3′-untranslated region (3′-UTR) functions as a ceRNA in inducing the development of hepatocellular carcinoma by regulating miRNA activity. FASEB J 2013; 27:907 - 19; http://dx.doi.org/10.1096/fj.12-220905; PMID: 23180826
- Cesana M, Cacchiarelli D, Legnini I, Santini T, Sthandier O, Chinappi M, Tramontano A, Bozzoni I. A long noncoding RNA controls muscle differentiation by functioning as a competing endogenous RNA. Cell 2011; 147:358 - 69; http://dx.doi.org/10.1016/j.cell.2011.09.028; PMID: 22000014
- Rutnam ZJ, Yang BB. The non-coding 3′ UTR of CD44 induces metastasis by regulating extracellular matrix functions. J Cell Sci 2012; 125:2075 - 85; http://dx.doi.org/10.1242/jcs.100818; PMID: 22637644
- Jaffe EA, Nachman RL, Becker CG, Minick CR. Culture of human endothelial cells derived from umbilical veins. Identification by morphologic and immunologic criteria. J Clin Invest 1973; 52:2745 - 56; http://dx.doi.org/10.1172/JCI107470; PMID: 4355998
- Patterson TA, Lobenhofer EK, Fulmer-Smentek SB, Collins PJ, Chu TM, Bao W, Fang H, Kawasaki ES, Hager J, Tikhonova IR, et al. Performance comparison of one-color and two-color platforms within the MicroArray Quality Control (MAQC) project. Nat Biotechnol 2006; 24:1140 - 50; http://dx.doi.org/10.1038/nbt1242; PMID: 16964228
- Wang N, Sun C, Huo S, Zhang Y, Zhao J, Zhang S, Miao J. Cooperation of phosphatidylcholine-specific phospholipase C and basic fibroblast growth factor in the neural differentiation of mesenchymal stem cells in vitro. Int J Biochem Cell Biol 2008; 40:294 - 306; http://dx.doi.org/10.1016/j.biocel.2007.08.003; PMID: 17890138