Abstract
A central part of the core macroauto-phagy (hereafter autophagy) machinery includes the two ubiquitin-like (Ubl) conjugation systems that involve the Ubl proteins Atg8 and Atg12.1 Although the functions of these proteins have not been fully elucidated, they play critical roles in autophagosome formation. For example, Atg8 is involved in cargo recognition,2,3 and the amount of Atg8 in part determines the size of the autophagosome,4 whereas Atg12 is part of a trimer that may function as an E3 ligase to facilitate Atg8 conjugation to phosphatidylethanolamine and determine, in part, the site of the conjugation reaction.5 Thus, fully functional autophagy requires both the Atg8 and Atg12 conjugation systems. Dysfunctional autophagy is associated with various human pathophysiologies including cancer, neurodegeneration, gastrointestinal disorders and heart disease. So, if you are wondering whether autophagy is operating properly in your own body, what can you do? The problem is that there are relatively few methods for analyzing autophagy in vivo.6-11 Minimally, you might want to find out if the relevant genes are intact and have the correct sequence. Considering the rapid advances being made in DNA sequencing technology, it is likely only a matter of time before people can submit a DNA sample and obtain a rapid readout of particular genes, or their entire genome. Thus, anticipating the future, we decided to analyze a select set of autophagy-related (ATG) genes, with a focus on those encoding components of the Ubl conjugation systems, by a polymerase chain reaction (PCR)-based method that combines science with art.
Introduction
The Atg8 and Atg12 conjugation systems have been relatively well characterized with regard to the chain of events that culminate in the final products ().Citation12-Citation17 In brief, yeast Atg8 is synthesized with a C-terminal arginine that is removed by the Atg4 protease to expose the penultimate glycine residue. Atg8 is then activated by Atg7, an E1-like homolog, and subsequently conjugated to phosphatidylethanolamine through the action of Atg3, an E2-like enzyme. Atg12 is also activated by Atg7, and then conjugated to an internal lysine of Atg5 in a reaction dependent on Atg10, another E2-like component. Atg5 binds noncovalently to Atg16, which dimerizes to generate a trinary dimer referred to as Atg12–Atg5-Atg16 (the en dash between “Atg12” and “Atg5” denotes a covalent bond). A similar series of events describes the conjugation systems in higher eukaryotes.
Atg8–PE initially lines both sides of the phagophore. Much of the Atg8 is released from PE by a second Atg4-dependent cleavage reaction, although some of the Atg8–PE on the concave side of the phagophore membrane remains trapped within the completed autophagosome; in the case of selective types of autophagy, the cargo may block access to Atg8–PE that interacts with various cargo receptors or tags. It is not known what regulates the cleavage of Atg8–PE, or whether this is temporally controlled to prevent premature deconjugation. Using a yeast multiple-knockout strain that lacks the known genes required for autophagosome formation,Citation18 it was shown that Atg8–PE can be generated at multiple sites, not just the phagophore assembly site (PAS) as is seen in wild-type cells.Citation19 When Atg4 and additional components are added back, Atg8–PE (detected as a GFP chimera) is detected at the PAS. Thus, there appears to be a posttranslational aspect to Atg8 regulation whereby the access of Atg4 to Atg8–PE is controlled at the PAS, whereas any conjugation that occurs at other locations is corrected by immediate Atg4-dependent deconjugation.
At present, there are 35 Atg proteins identified in fungi. It is striking that 8 of them, almost one-quarter of the total, are involved in the conjugation of Atg8 to PE and Atg12 to Atg5. Although we do not know the precise functions of all of the proteins involved in the conjugation systems, we clearly know much about the relevant protein-protein interactions. In addition, almost all of the structural information that is available for Atg proteins has focused on the Atg8 and Atg12 systems.Citation20-Citation27 Accordingly, in planning our genomic analysis of autophagy we concentrated on the genes that correspond to the eight proteins involved in the generation of Atg8–PE and Atg12–Atg5-Atg16.
Results
The standard procedure at Yonder Biology is to obtain cheek cells from the interested individual(s), isolate the DNA, and amplify short tandem repeats that produce a visually pleasing pattern determined empirically. For the analysis of the autophagy conjugation systems, however, it was necessary to design specific oligonucleotide primers to each of the relevant genes (). Primer pairs were designed based on sequences in the NCBI UniSTS database (). Autophagy primer sets were then ordered from Eurofins MWG Operon.
Table 1. Autophagy-related genes used in this analysis
Cheek cells were collected from the inside of the cheeks of two subjects using a sterile cotton swab (). Two sets of cells were collected and referred to as DK1 and DK2. DNA was isolated from cheek cells using a modified Qiagen DNeasy protocol. Autophagy DNA markers were amplified from the DNA corresponding to DK1 and DK2 using the PCR method with the autophagy-specific primer sets. The primer sets were tested using a standard PCR protocol as described in Materials and Methods (). Depending on the banding pattern, two primer sets were used for certain genes ().
Figure 3. An initial test gel was run to qualify the primer sets and visualize the banding size/pattern.
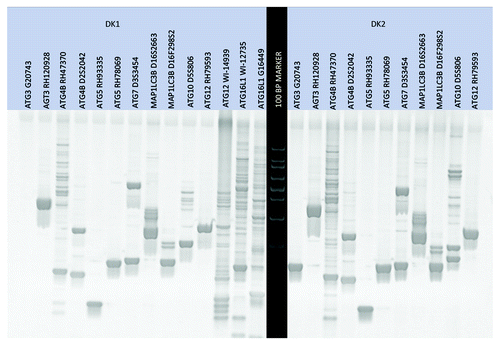
Amplified DNA markers were run on a 4% agarose gel to separate the bands and visualize the DNA. The gel was stained with ethidium bromide, and illuminated under UV light. Several images were taken with a high-quality camera, and the images were transferred to a computer. The image was cropped using Photoshop, colorized and sized for printing ().
Figure 4. The final gel-loading scenario was determined and a new gel was run to visualize the autophagy markers in a manner that was consistent with the known metabolic pathway cascade as depicted in . All DNA bands are from DK1 unless otherwise specified. Lanes correspond to the following: (1) 100 base DNA ladder; (2) Atg8 (MAP1LC3) with Atg4 (RH47370, D2S2042); (3) Atg8 (MAP1LC3B/D16S2663) with Atg7 (D3S3454); (4) Atg8 (MAP1LC3B/D16S2663 and D16F298S2) with Atg3 (RH120928); (5) Atg12 (RH79593) with Atg7 (D3S3454); (6) Atg12 (RH70503; DK2 DNA) with Atg5 (RH93335) and Atg10 (D5S806; DK2 DNA); (7) Atg12 (RH79593) with Atg5 (RH93335) and ATG16L1 (ATG16L1, WI-12735 added digitally); (8) 100 base DNA ladder. The sequence for yeast ATG8 was added to the background; this sequence was quadrupled on the final image for aesthetic reasons. The final gels are shown in the (A) zebra pattern, (B) an experimental customized pattern and (C) igloo pattern (without the background sequence).
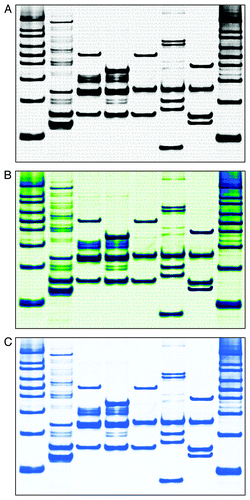
Discussion
All primer sets worked on the first attempt at amplification on both individuals, suggesting that the assay was robust and the loci highly conserved. Four markers required optimization due to multiple banding: ATG4B (RH47370), ATG12 (W1-14939), ATG12 (W1-12735) and ATG16L1 (G16449). Increasing PCR stringency by increasing the annealing temperature failed to fully resolve the multiple banding issue.
Some of the autophagy DNA marker primer sets worked on yeast and dog DNA, suggesting that these human autophagy genes are highly conserved. This work was not confirmed, however, and was only attempted for use as a control for another project that Yonder Biology was working on in the lab.
Determining the autophagic response in vivo following treatments aimed at modulating autophagy is a major goal of researchers and clinicians who are attempting to evaluate the effect of anticancer drugs, or regimens designed to ameliorate neurodegenerative or other diseases. Although the method of analysis described here does not provide a practical method for analyzing macroautophagy in vivo, it highlights the need for additional research on this topic.
Materials and Methods
Table 2. Autophagy primer sequences
Collecting cells
Cheek cells were collected from the inside of the cheek using a sterile cotton swab (DNA Genotek, ORAcollect OC-100).
Isolating the DNA
(1) Opening the cells: The cotton end of the collection swab was dispensed into a microcentrifuge tube and reagents to lyse the cells were added. Lysis was performed with the QIAamp DNA mini Kit (Qiagen, 51304). (2) Capturing the DNA: The DNA-cell lysis solution was then passed through a column using vacuum pressure; the DNA binds to the silica membrane in the column and the rest of the cell matter passes though the column. (3) Washing and eluting the DNA: To wash contaminants from the DNA, ethanol in varying concentrations (57% and 70%) was passed through the column using vacuum pressure. Water or Tris-EDTA (10 mM Tris, pH 8.0, 1 mM EDTA) was used to elute the DNA from the column.
Yeast DNA was isolated from California Ale Yeast (White Labs; WLP001).
Amplifying specific DNA regions by PCR
The DNA was transferred into a microtiter plate along with DNA Taq Polymerase, 1 μl 40 mM DNTPs (Monserate Biotech, 2001 and 8001) and 1 mM MgCl2 in 20 μl reactions. The PCR was performed using an ABI GeneAmp PCR System 9700. One cycle of PCR consisted of raising the temperature to 90°C, 30 min to denature the DNA, followed by an annealing temperature of 54–64°C, 30 min and a 72°C extension reaction, 45 min. This cycle was repeated 25 to 30 times.
Separating the amplified segments of DNA by gel electrophoresis.
Nine microliters of the amplified DNA segments were removed from the PCR microtiter plate. A gel loading dye (GenScript, M00120) was added to each amplified DNA solution, and this solution was loaded into the wells of a 4% UltraPure agarose gel (Invitrogen, 16500500). The gel was run at 120 V for 90 min.
Imaging the DNA pattern.
The gel was submerged in an ethidium bromide solution for 20 min, rinsed (destained) and imaged on a Bio-Rad Gel DocTM XR+ System and the image was transferred to a computer.
Style and colorize DNA image.
Computer-aided design was used to crop, adjust, colorize and size the DNA portrait.
Acknowledgements
The authors thank Lisa Klionsky for “volunteering” to provide cheek cells.
Disclosure of Potential Conflicts of Interest
Andy Bass and Dean Sauer are the co-founders of Yonder Biology.
References
- Geng J, Klionsky DJ. The Atg8 and Atg12 ubiquitin-like conjugation systems in macroautophagy. EMBO Rep 2008; 9:859 - 64; http://dx.doi.org/10.1038/embor.2008.163; PMID: 18704115
- Okamoto K, Kondo-Okamoto N, Ohsumi Y. Mitochondria-anchored receptor Atg32 mediates degradation of mitochondria via selective autophagy. Dev Cell 2009; 17:87 - 97; http://dx.doi.org/10.1016/j.devcel.2009.06.013; PMID: 19619494
- Shintani T, Huang W-P, Stromhaug PE, Klionsky DJ. Mechanism of cargo selection in the cytoplasm to vacuole targeting pathway. Dev Cell 2002; 3:825 - 37; http://dx.doi.org/10.1016/S1534-5807(02)00373-8; PMID: 12479808
- Xie Z, Nair U, Klionsky DJ. Atg8 controls phagophore expansion during autophagosome formation. Mol Biol Cell 2008; 19:3290 - 8; http://dx.doi.org/10.1091/mbc.E07-12-1292; PMID: 18508918
- Fujita N, Itoh T, Omori H, Fukuda M, Noda T, Yoshimori T. The Atg16L complex specifies the site of LC3 lipidation for membrane biogenesis in autophagy. Mol Biol Cell 2008; 19:2092 - 100; http://dx.doi.org/10.1091/mbc.E07-12-1257; PMID: 18321988
- Barth S, Glick D, Macleod KF. Autophagy: assays and artifacts. J Pathol 2010; 221:117 - 24; http://dx.doi.org/10.1002/path.2694; PMID: 20225337
- Haspel J, Shaik RS, Ifedigbo E, Nakahira K, Dolinay T, Englert JA, et al. Characterization of macroautophagic flux in vivo using a leupeptin-based assay. Autophagy 2011; 7:629 - 42; http://dx.doi.org/10.4161/auto.7.6.15100; PMID: 21460622
- Iwai-Kanai E, Yuan H, Huang C, Sayen MR, Perry-Garza CN, Kim L, et al. A method to measure cardiac autophagic flux in vivo. Autophagy 2008; 4:322 - 9; PMID: 18216495
- Ju JS, Varadhachary AS, Miller SE, Weihl CC. Quantitation of “autophagic flux” in mature skeletal muscle. Autophagy 2010; 6:929 - 35; http://dx.doi.org/10.4161/auto.6.7.12785; PMID: 20657169
- Kim KW, Moretti L, Mitchell LR, Jung DK, Lu B. Combined Bcl-2/mammalian target of rapamycin inhibition leads to enhanced radiosensitization via induction of apoptosis and autophagy in non-small cell lung tumor xenograft model. Clin Cancer Res 2009; 15:6096 - 105; http://dx.doi.org/10.1158/1078-0432.CCR-09-0589; PMID: 19773376
- Martinet W, De Meyer GR, Andries L, Herman AG, Kockx MM. In situ detection of starvation-induced autophagy. J Histochem Cytochem 2006; 54:85 - 96; http://dx.doi.org/10.1369/jhc.5A6743.2005; PMID: 16148314
- George MD, Baba M, Scott SV, Mizushima N, Garrison BS, Ohsumi Y, et al. Apg5p functions in the sequestration step in the cytoplasm-to-vacuole targeting and macroautophagy pathways. Mol Biol Cell 2000; 11:969 - 82; PMID: 10712513
- Mizushima N, Noda T, Yoshimori T, Tanaka Y, Ishii T, George MD, et al. A protein conjugation system essential for autophagy. Nature 1998; 395:395 - 8; http://dx.doi.org/10.1038/26506; PMID: 9759731
- Nemoto T, Tanida I, Tanida-Miyake E, Minematsu-Ikeguchi N, Yokota M, Ohsumi M, et al. The mouse APG10 homologue, an E2-like enzyme for Apg12p conjugation, facilitates MAP-LC3 modification. J Biol Chem 2003; 278:39517 - 26; http://dx.doi.org/10.1074/jbc.M300550200; PMID: 12890687
- Kuma A, Mizushima N, Ishihara N, Ohsumi Y. Formation of the approximately 350-kDa Apg12-Apg5-Apg16 multimeric complex, mediated by Apg16 oligomerization, is essential for autophagy in yeast. J Biol Chem 2002; 277:18619 - 25; http://dx.doi.org/10.1074/jbc.M111889200; PMID: 11897782
- Yamazaki-Sato H, Tanida I, Ueno T, Kominami E. The carboxyl terminal 17 amino acids within Apg7 are essential for Apg8 lipidation, but not for Apg12 conjugation. FEBS Lett 2003; 551:71 - 7; http://dx.doi.org/10.1016/S0014-5793(03)00899-8; PMID: 12965207
- Yuan W, Stromhaug PE, Dunn WA Jr. Glucose-induced autophagy of peroxisomes in Pichia pastoris requires a unique E1-like protein. Mol Biol Cell 1999; 10:1353 - 66; PMID: 10233149
- Cao Y, Cheong H, Song H, Klionsky DJ. In vivo reconstitution of autophagy in Saccharomyces cerevisiae. J Cell Biol 2008; 182:703 - 13; http://dx.doi.org/10.1083/jcb.200801035; PMID: 18725539
- Nair U, Cao Y, Xie Z, Klionsky DJ. Roles of the lipid-binding motifs of Atg18 and Atg21 in the cytoplasm to vacuole targeting pathway and autophagy. J Biol Chem 2010; 285:11476 - 88; http://dx.doi.org/10.1074/jbc.M109.080374; PMID: 20154084
- Kumeta H, Watanabe M, Nakatogawa H, Yamaguchi M, Ogura K, Adachi W, et al. The NMR structure of the autophagy-related protein Atg8. J Biomol NMR 2010; 47:237 - 41; http://dx.doi.org/10.1007/s10858-010-9420-1; PMID: 20428927
- Fujioka Y, Noda NN, Nakatogawa H, Ohsumi Y, Inagaki F. Dimeric coiled-coil structure of Saccharomyces cerevisiae Atg16 and its functional significance in autophagy. J Biol Chem 2010; 285:1508 - 15; http://dx.doi.org/10.1074/jbc.M109.053520; PMID: 19889643
- Satoo K, Noda NN, Kumeta H, Fujioka Y, Mizushima N, Ohsumi Y, et al. The structure of Atg4B-LC3 complex reveals the mechanism of LC3 processing and delipidation during autophagy. EMBO J 2009; 28:1341 - 50; http://dx.doi.org/10.1038/emboj.2009.80; PMID: 19322194
- Yamada Y, Suzuki NN, Hanada T, Ichimura Y, Kumeta H, Fujioka Y, et al. The crystal structure of Atg3, an autophagy-related ubiquitin carrier protein (E2) enzyme that mediates Atg8 lipidation. J Biol Chem 2007; 282:8036 - 43; http://dx.doi.org/10.1074/jbc.M611473200; PMID: 17227760
- Matsushita M, Suzuki NN, Obara K, Fujioka Y, Ohsumi Y, Inagaki F. Structure of Atg5·Atg16, a complex essential for autophagy. J Biol Chem 2007; 282:6763 - 72; http://dx.doi.org/10.1074/jbc.M609876200; PMID: 17192262
- Suzuki NN, Yoshimoto K, Fujioka Y, Ohsumi Y, Inagaki F. The crystal structure of plant ATG12 and its biological implication in autophagy. Autophagy 2005; 1:119 - 26; http://dx.doi.org/10.4161/auto.1.2.1859; PMID: 16874047
- Sugawara K, Suzuki NN, Fujioka Y, Mizushima N, Ohsumi Y, Inagaki F. The crystal structure of microtubule-associated protein light chain 3, a mammalian homologue of Saccharomyces cerevisiae Atg8. Genes Cells 2004; 9:611 - 8; http://dx.doi.org/10.1111/j.1356-9597.2004.00750.x; PMID: 15265004
- Sugawara K, Suzuki NN, Fujioka Y, Mizushima N, Ohsumi Y, Inagaki F. Structural basis for the specificity and catalysis of human Atg4B responsible for mammalian autophagy. J Biol Chem 2005; 280:40058 - 65; http://dx.doi.org/10.1074/jbc.M509158200; PMID: 16183633