Abstract
The autophagy-dependent selective degradation of mitochondria (mitophagy) plays an important role in removing excessive, damaged and dysfunctional mitochondria to maintain a proper cellular homeostasis. Relative to its significance in cell physiology, very little is known about the molecular machinery and regulatory mechanism of mitophagy in mammalian cells or yeast. We found that two mitogen-activated protein kinases (MAPKs), Slt2 and Hog1, are required for mitophagy in Saccharomyces cerevisiae. Slt2 is involved in both mitophagy and pexophagy (the selective degradation of peroxisomes through autophagy), whereas Hog1 functions specifically in mitophagy.
Keywords: :
Macroautophagy (hereafter autophagy) is a catabolic process for degrading cellular components, such as cytosol, organelles and protein aggregates. To date, two major types of autophagy have been identified in yeast: nonselective bulk autophagy and selective autophagy. Bulk autophagy mainly functions in cytoplasm recycling to allow the cell to survive starvation. Selective autophagy allows the degradation of specific cargoes or organelles, allowing the cell to adapt to changing environmental conditions, and eliminate damaged organelles. There is also a biosynthetic transport process (the cytoplasm-to-vacuole targeting pathway) that is considered a type of selective autophagy, because it shares most of the same protein machinery as nonspecific autophagy. Among the different kinds of selective autophagy, mitophagy has attracted increasing attention due to the significance of mitochondria in cell metabolism.
Physiological Roles of Mitophagy in Mammalian Cells
Mitophagy controls both the number and quality of mitochondria, which are affected by different stress and nutrient conditions. Even though mitochondria function as the cellular power plant to supply energy, excessive mitochondria might be a burden to the cell. This organelle must be maintained in a functional state, as dysfunctional or damaged mitochondria would result in the overproduction of potentially damaging reactive oxygen species (ROS). Mitophagy also plays a developmental role. For example, studies on the maturation of red blood cells indicate that all of the mitochondria are degraded by NIX (NIP3-like X)-mediated mitophagy. In addition, there are clear connections between defects in mitophagy and certain human diseases. For example, mutations in the genes encoding PINK1 and PARKIN, which are associated with some forms of Parkinson disease, cause a defect in the cell’s ability to recognize and eliminate damaged mitochondria. Similarly, Wolfram syndrome 2 and premature aging may be linked to mitochondrial dysfunction, followed by accelerated autophagy and cell death.
Mitophagy in Yeast
The budding yeast Saccharomyces cerevisiae is a good model system to study mitophagy, due to its genetic tractability. Previous studies indicate that two types of mitophagy occur in yeast. First, changing the carbon source from a nonfermentable substrate such as lactic acid or ethanol to a fermentable one, glucose, leads to the degradation of superfluous mitochondria. Second, enhanced mitophagy occurs in post-log phase grown yeast cells, in which mitochondria might be “old” and overworked. The finding of a mitophagy-specific receptor, Atg32, led to a breakthrough in the study of mitophagy at the molecular level. Atg32 is located on mitochondria, and links mitchondria destined for degradation to the autophagy machinery through interaction with Atg11 and Atg8. However, in order to clearly uncover the mystery of this catabolic process, more studies are required. Our lab recently found that two MAPK-signaling pathways (involving Slt2 and Hog1) are required for mitophagy in yeast.
Slt2 and Hog1 Signaling Pathways
Although mitochondria are degraded through an autophagic pathway, the selective engulfment of mitochondria and the exclusion of other cytoplasmic components implied the existence of some additional regulatory mechanisms other than the known upstream signaling components, such as TOR, Sch9, Ras/cAMP-dependent protein kinase A (PKA) and Pho85. We found that the Slt2 and Hog1 signaling pathways belong to this “additional” group, as bulk autophagy functions normally in either slt2∆ or hog1∆ cells, which indicated their specificity for regulating a selective type of autophagy such as mitophagy.
In our analysis, even though both Slt2 and Hog1 are activated when cells are cultured in mitophagy-inducing conditions, the timing of activation is different; Slt2 is activated before Hog1. Interestingly, Slt2, but not Hog1, is required for the recruitment of mitochondria destined for degradation to the mitophagy-specific phagophore assembly site (mitoPAS). Therefore, we propose that Slt2 might participate in an early stage of mitophagy, while Hog1 acts in a later stage ().
Figure 1. Schematic representation of the Slt2 and Hog1 signaling pathways regulating mitophagy. When lactic acid-cultured yeast cells are subjected to nitrogen starvation in the presence of glucose, Slt2 signaling is activated to regulate an early stage of mitophagy; later, Hog1 signaling is activated to control a later stage. MitoPAS, mitophagy-specific phagophore assembly site; mitophagosome, mitophagy-specific autophagosome-like double-membrane vesicle.
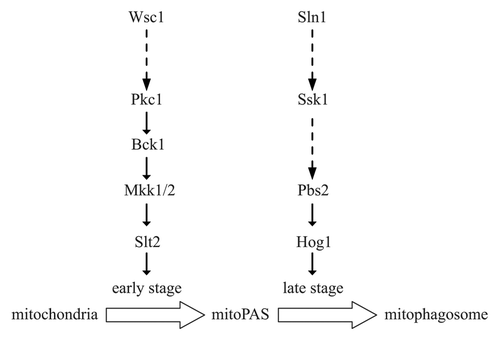
Further investigation of the upstream signaling components should help elucidate the origin of the signal transduction process, and the mechanism that links the changes in the environment to the cellular response. Of the six known cell surface sensors that activate Slt2 signaling, only Wsc1 is required for mitophagy. Wsc1 is localized on the plasma membrane, and as such is supposed to sense extracellular stress; however, mitophagy is likely to be ultimately induced by an intracellular signal, and therefore the “decision” to degrade mitochondria should be “made” within the cell. A similar issue was also found in the case of the Hog1 signaling pathway, as Sln1, a plasma membrane-localized osmosensor, seems to be in charge of the input for mitophagy control. Our results exclude the possibility that the mitophagy-inducing condition we used resulted in osmotic stress, as neither hyper- nor hypo-osmotic stress induce or affect mitophagy. One explanation is these plasma membrane sensors might also be able to respond to intracellular signals, especially those signals from mitochondria, because in yeast most of the mitochondria form tubular structures close to the cell periphery.
Due to the limitation in our current knowledge, we were unable to identify the relevant targets of either Slt2 or Hog1 for mitophagy regulation. Recently, another group determined that Hog1 plays a role in Atg32 phosphorylation, and this was necessary for mitophagy. This link further expanded our knowledge of mitophagy studies in yeast. Still, many questions remain unanswered, such as how dysfunctional mitochondria are detected in quality control mitophagy, and how the cell chooses and tags some portion of its mitochondria as “excessive” and destined for “number control” mitophagy. Similarly, the mechanism of mitophagy with regard to the protein machinery is far from being understood, as some Atg proteins are involved in mitophagy, but are not required for bulk autophagy. Determining the answers to these questions will help improve our understanding of this “new” and important cellular activity.