Abstract
Most studies of Lactococcus lactis as delivery vehicles of pneumococcal antigens are
focused on the effectiveness of mucosal recombinant vaccines against Streptococcus
pneumoniae in animal models. At present, there are three types of pneumococcal
vaccines: capsular polysaccharide pneumococcal vaccines (PPV), protein-
polysaccharide conjugate pneumococcal vaccines (PCV) and protein-based
pneumococcal vaccines (PBPV). Only PPV and PCV have been licensed. These
vaccines, however, do not represent a definitive solution. Novel, safe and inexpensive
vaccines are necessary, especially in developing countries. Probiotic microorganisms
such as lactic acid bacteria (LAB) are an interesting alternative for their use as vehicles
in pneumococcal vaccines due to their GRAS (Generally Recognized As Safe) status.
Thus, the adjuvanticity of Lactococcus lactis by itself represents added value over the
use of other bacteria, a question dealt with in this review. In addition, the expression of
different pneumococcal antigens as well as the use of oral and nasal mucosal routes of
administration of lactococcal vaccines is considered. The advantages of nasal live
vaccines are evident; nonetheless, oral vaccines can be a good alternative when the
adequate dose is used. Another point addressed here is the use of live versus inactivated
vaccines. In this sense, few researches have focused on inactivated strains to be used as
vaccines against pneumoccoccus. The immunogenicity of live vaccines is better than the
one afforded by inactivated ones; however, the probiotic-inactivated vaccine
combination has improved this matter considerably. The progress made so far in the
protective immune response induced by recombinant vaccines, the successful trials in
animal models and the safety considerations of their application in humans suggest that
the use of recombinant vaccines represents a good short-term option in the control of
pneumococcal diseases.
Introduction
Respiratory infections represent a serious worldwide health problem that affects mainly young children, the elderly people and immunocompromised individuals. Respiratory Syncytial Virus (RSV) is the most common viral cause of lower respiratory tract infections (LRTIs), especially in young children, and Streptococcus pneumoniae (pneumococcus) and Haemophilus influenzae are the most common bacterial causes.Citation1
At present, S. pneumoniae (pneumococcus) is a leading cause of morbidity and mortality among children all over the world and a major cause of diseases such as pneumonia, meningitis and sepsis. An estimated 700,000 to one million children, most of whom live in developing countries, die of pneumococcal diseases every year.Citation2–Citation4 In these countries, pneumococcal disease is common in children under two years of age, including newborn infants, while in the elderly population incidence rates as unknown. The most common form of the disease is bacteremic pneumococcal pneumonia, whose highest incidence is associated with both extremes of age (children <2 years of age and adults >65 years of age). The next common disease is pneumococcal meningitis, especially in infants and young children, followed by blood stream infection or sepsis and otitis media. Pneumococci also colonize the nasopharynx of up to 50% of the children in the world while up to 5% of the adults are pneumococcal carriers, which increase the risk of spreading the infection.
The world trend in the control of pneumococcal diseases is towards the design of mucosal vaccines because they are practical, non invasive and effective for the induction of an adequate response at both the mucosal and the systemic levels. In this sense, lactic acid bacteria (LAB), microorganisms with GRAS (Generally Recognized As Safe) status, some of them with important immunostimulant properties and positive effects on human and animal health,Citation5–Citation7 represent an interesting alternative for the development of a mucosal vaccine. The most common lactic acid bacterium used as a vehicle of antigen presentation is Lactococcus lactis, a bacterium extensively engineered for the production of heterologous bacterial and viral proteins.Citation8–Citation10
The aim of this review is to update the most relevant findings concerning the use of the recombinant L. lactis as an adjuvant and delivery vehicle of antigens against pneumococcal infections and, from a prophylactic point of view, to gain insight into the protective immune effect induced by the oral and nasal administration of a recombinant vaccine.
Pneumococcal Vaccines
For many years pneumococcal infections were controlled by antimicrobial treatments which have contributed to the increase in the rate of antimicrobial resistance among S. pneumoniae strains. Increasing pneumococcal resistance to antibiotics indicates the urgent need for effective vaccines to prevent and control pneumococcal diseases. Thus, eliciting an adequate protective immunity through vaccination represents a major challenge.
At present, there are three established approaches in vaccine development against pneumococcus: capsular polysaccharide pneumococcal vaccines (PPV), protein-polysaccharide conjugate pneumococcal vaccines (PCV) and protein-based pneumococcal vaccines (PBPV). Only PPV and PCV have been licensed and are in current clinical use while PBPV are still under investigation.
Pneumococcal epidemiology is based on 91 serotypes,Citation11 each corresponding to the different structures of the capsular polysaccharide (CP), one of the major virulence determinants of S. pneumoniae. These polysaccharides differ in immunological properties, chemical composition and virulence potential and relatively few of S. pneumoniae serotypes are responsible for most serious pneumococcal diseases. Antibodies to the capsular polysaccharide confer protection against disease and vaccines in use are based on the induction of these antibodies. However, this protection is serotype specific. Thus, the currently available 23-valent PPV, licensed in 1983, has the potential to prevent pneumococcal disease and contains CP antigens against the 23 most prevalent serotypes in clinical isolates.Citation12 These antigens induce type-specific antibodies by a T-cell independent way that improves opsonization and promotes phagocytosis and killing of pathogens by cells of innate immunity.Citation13 The degree of protection against different serotypes and within different populations is uncertain. After PPV vaccination, the protective immune response is good in children >2 years but in younger infants, elderly people and immunocompromised individuals the specific humoral response may be poor. In addition, these individuals may respond only against some of the serotypes contained in the vaccine.Citation12,Citation13 Different meta-analyses in adults have provided inconclusive results concerning the effectiveness of PPV in preventing pneumonia in high-risk groups, especially in the elderly.Citation12,Citation14
Based on the low effectiveness of PPV in young children, a new generation of vaccines with good immunogenicity in infants was developed. These conjugate vaccines (PCV) are based on a combination of CPs with a highly immunogenic protein (i.e., a non-toxic diphtheria toxoid). These vaccines induce good B- and T-cell specific immune responses and protect against vaccine serotypes that induce invasive pneumococcal disease. The currently available PCV was licensed in 2000 and contains antigens against the seven most common pneumococcal serotypes (PCV-7) that cause disease in children. PCV-7 has already been included in the national infant immunization programme of more than 10 developed countries (http://www.who.int/nuvi/pneumococcus). In developing countries, other serotypes should be included in the formulation of PCV-7 to be effective. Thus, new conjugate vaccines including additional serotypes (9 and 11) are expected to be available in the next few years. On the other hand, although these vaccines can be a good alternative for developed countries, they are too expensive for developing countries so that support from governments and international agencies would be required. It is important to keep in mind that PCVs do no represent a definitive solution because recent researches have shown that serotypes eradicated by the vaccine are being replaced by non-vaccine pneumococcal serotypes and the impact of a pneumococcal vaccination programme would be reduced if serotype replacement were significant.Citation15–Citation17
Besides the polysaccharide capsule of S. pneumoniae, numerous pneumococcal proteins are important virulence factors and are also associated with its pathogenicity. In particular, new strategies in the fight against this pathogen involve the identification of pneumococcal surface proteins that are serotype independent. These proteins represent a valuable option for the design of a vaccine through the induction of specific antibodies that neutralize or reduce the virulence of the pathogen. Several pneumococcal proteins alone or in combination with others have been evaluated in animal models. These studies have shown that some of these PBPV formulations were able to protect against invasive infection and nasopharyngeal carriage.Citation18–Citation21 Compared with PPV and PCV, the major potential advantages of PBPV are that it could induce a serotype-independent protection and that it can be administered through the oral or nasal mucosal routes. Adjuvants are necessary for protein administration through the mucosal route and cholera toxin or heat-labile enterotoxin has been used. However, these adjuvants may not be clinically safe.Citation22 At present, none of the formulations containing proteins have been licensed for use in human health.
Thus, new, safe and inexpensive vaccines for global application to populations at risk are necessary to control pneumococcal diseases, especially in developing countries. In this sense, probiotic microorganisms such as LAB represent an interesting alternative for their use as vehicles in vaccine formulations since they have important immunomodulatory propertiesCitation6,Citation7 that include the improvement of the immune response against respiratory pathogens.Citation23,Citation24
Pneumococcal Infection: Immunity in the Respiratory Tract
The pulmonary immune response against respiratory diseases involves both the innate and the adaptive immune responses. The former is mediated by the phagocytic defense against the pathogen, principally carried out by alveolar macrophages and neutrophils (). Alveolar macrophages have important phagocytic, microbicidal and secretory functions and play a prominent role in lung immunity by initiating inflammatory and immune responses. In the steady state, the main function of alveolar macrophages is the phagocytosis and sequestration of antigens from the immune system to shield local tissues from the development of specific immune responses.Citation25 Alveolar macrophages take up most of the particulate material delivered intranasally, but they do not migrate to regional lymph nodes, nor are they considered to have a significant role in antigen presentation.Citation26 Although resting alveolar macrophages are normally maintained in a quiescent state and produce small amounts of pro-inflammatory cytokines, in the presence of a pathogenic bacteria they are capable of producing proinflammatory chemokines and cytokines such as TNFα and IL-1, which coordinate the innate immune response.Citation27 These biological mediators induce the recruitment of neutrophils and monocytes from the lung capillaries. The recruited neutrophils provide auxiliary phagocytic capacities that are critical for the effective eradication of offending pathogens. On the other hand, it is worth noting that the functional phenotype of recently recruited monocytes shows a marked contrast with that of resident alveolar macrophages; monocytes, in particular, can function as effective antigen-presenting cells (APCs) before their maturation into immunosuppressive alveolar macrophages, which occurs over a period of days.Citation25
One of the most important APCs in lungs are dendritic cells,Citation28 which play a central role in initiating protective T-cell immune responses in the respiratory tract. Dendritic cells, with long, intertwined cytoplasmic processes at the base of the airway epithelium and distributed throughout the lung, form a network ideally positioned to sample inhaled antigens for subsequent presentation to naive T cells. In the steady state, resident lung dendritic cells are specialized for antigen uptake and processing but lack the capacity for efficient antigen presentation. However, when a dangerous antigen reaches the respiratory tissues, dendritic cells are able to take up and process these antigens and to migrate to mediastinal lymph nodes via afferent lymphatics (). The migration of respiratory dendritic cells to draining lymph nodes is accompanied by their maturation and by the increased co-stimulatory expression of CD40, CD80, CD86 and MHC-II molecules. In regional lymphoid organs dendritic cells present antigenic peptides to naive T cells and stimulate the clonal development of antigen-specific T cells.Citation29 The activated T cells proliferate and migrate through the efferent lymphatics into the bloodstream. Effector T cells exit into the bronchial mucosa through postcapillary venules in the lamina propria or disseminate from the bloodstream throughout the peripheral immune system. In the absence of bacterial stimuli, the airways environment is characterized by a weak Th2 response to inhaled harmless antigens.Citation28 Under inflammatory conditions, cytokines in the respiratory tract change dramatically. CD4+ T cells play an important role in the initiation of immune responses by providing help to other cells. Upon antigenic stimulation, naive CD4+ T cells proliferate and differentiate into different effector subsets characterized by the production of specific cytokines and effector functions. T helper cells could be divided into distinct subsets, T helper type 1 (Th1), Th2,Citation30 and Th17, characterized by distinct cytokine profiles and effector functions. Th1 cells produce large quantities of IFNγ, activate macrophages and are highly effective in clearing intracellular pathogens. In mice, Th1 cells induce B cells to produce IgG2a.Citation31 Th2 cells, on the other hand, produce IL-4, IL-5, IL-13 and IL-25. Th2 cells are especially important for humoral immunity and, in mice, they direct B cell secretion of IgE and IgG1.Citation31 The discovery of the IL-17 family of cytokines and the analysis of IL-23-mediated effector functions on T cells have suggested the existence of an additional subset of CD4+ T cells that produce IL-17, designated Th17. CD4+ Th17 cells seem to play a critical role in the enhancement of host protection against extracellular bacteria and fungi, which are not efficiently cleared by Th1 and Th2 responses.Citation32 After infection, the activated CD4+ Th2-cells contribute to stimulate B cells to proliferate and mature into polymeric IgA-producing cells and to develop specific antibodies.Citation33,Citation34 The IgA-committed B cells then migrate to mucosal effector sites where the antigen-sensitized IgM−IgA+ B cells undergo terminal differentiation to IgA-producing plasma cells. The production of specific IgA in the respiratory tract during an infectious process prevents colonization of mucosal tissues and subsequent spreading into the systemic circulation.Citation35 Additionally, specific IgA antibodies can bind antigens and minimize their entry with a consequent reduction in inflammatory reactions, which prevents potentially harmful effects on the tissue.
In the specific case of S. pneumoniae, when this pathogen reaches the alveolar space in the deep lung, resident cells secrete cytokines and chemokines to attract APCs:Citation28 macrophagesCitation35 and dendritic cells (). The induction of the specific immune response starts when immature APCs recognize and take up the antigen. Then, these APCs are induced to migrate via the afferent lymphatics into the draining lymph nodes of the lung where the primary immune response occurs. During this migration APCs mature and become efficient, losing their capacity to take up antigens and to activate the expression of the costimulatory molecules CD80 and CD86 that are necessary to stimulate naive T cells. Mature APCs present the processed antigen in association with MHC-II, which is recognized by the T-cell receptor (TCR) of naive CD4+ cells. The recognition of the antigen-MHC by the TCR and the stimulation of the costimulatory signal induce T-cell proliferation, differentiation and migration to peripheral tissues. In addition, activated T cells interact with B cells and induce stimulation of B cell clonal expansion, isotype switching and affinity maturation. Once IgM+IgG− have become IgM−IgG+ antigen-specific B cells, they traffic back to the lung where they terminally differentiate and expand into either antibody-secreting plasma cells or memory B cells.Citation35 These plasma cells are committed to the production of IgG, which has an important role in the protection against pathogens that reach the alveolar space. Opsonizing IgG antibodies are important for complement fixation and for enhanced efficiency in macrophage killing. This immune activation also induces the production at the systemic level of antibodies responsible for preventing the passage of pathogens to the blood and their subsequent dissemination. Besides, systemic antibody transudation increases during inflammatory reactions when vascular permeability is increased, which enhances pulmonary clearance of pathogenic microorganisms.Citation36
Knowledge of the mechanisms involved in the lung immune response against respiratory pathogens is necessary because it is in this context that recombinant vaccines will have to exert their preventive effect.
Expression System of Pneumococcal Antigens in Lactic Acid Bacteria
Due to their non-pathogenic status and the availability for recombinant gene expression, lactic acid bacteria are attractive candidates for use as vaccine carriers. The possibility of using LAB as vaccine vehicles has been thoroughly reviewed by Wells and Mercenier.Citation37 Most studies that evaluated LAB as vaccine vectors to elicit S. pneumoniae-antigen specific immune responses used protein antigens instead of polysaccharides (), Lactococcus lactis NZ9000 being the most common lactic acid bacterium employed as a vehicle.
Gilbert et al.Citation38 evaluated L. lactis as an attractive producer host for polysaccharides of pneumococcal origin and showed that recombinant L. lactis strains produced serotype 3 capsule polysaccharides (CPS) with a composition and structure identical to that of the native CPS produced by S. pneumoniae, while Nierop Groot et al.Citation39 did the same for serotype 14. Besides, the polysaccharide produced by recombinant L. lactis was not contaminated with C polysaccharide, a highly reactive immunogen produced by the pneumococcus. Immunization of mice with recombinant L. lactis producing type 3 CPS elicited a T-cell independent response characteristic of those elicited by unconjugated bacterial polysaccharides, showing that L. lactis is a potential host for capsule vaccine antigens.Citation38
On the other hand, formulations of LAB expressing the recombinant proteins of PspA, PsaA, PppA, PpmA, SlrA and IgA1p have been shown to be efficacious as vaccine carriers against pneumococci in animal models. Two main strategies have been applied in these studies: the use of recombinant lactic acid bacteria vectors and the use of a non-genetically modified gram-positive enhancer matrix (GEM) particle. In the first strategy, S. pneumoniae proteins were expressed in the cytoplasm or bacterial cell wall of the recombinant lactic acid bacteria using the nisin-controlled expression (NICE) system ().Citation40 The genes of interest were placed on a plasmid under the control of the inducible promoter PnisA, and their expression was induced by the addition of sub-inhibitory amounts of the lantibiotic nisin, in a strain containing the genes for the signal transduction system nisK and nisR. Depending on the presence or absence of secretion and cell wall (CW) anchoring signals, the proteins were expressed in the cytoplasm (absence of both secretion and CW signals) or in the cell wall (presence of both signals). Three LAB vaccines expressing the S. pneumoniae proteins, PsaA (pneumococcal surface antigen), PspA (pneumococcal surface protein A) and PppA (pneumococcal protective protein A) () were capable of eliciting significant protection against systemic and respiratory S. pneumoniae lethal challenges. The immune effects of each vaccine are discussed below.
In the second strategy, a non-living GEM particle was developed as an antigen delivery device.Citation41,Citation42 GEM particles were made from non-living L. lactis cells (cells were treated with a 1M hot acid solution for 30 min and washed thoroughly with PBS) free of DNA and cytoplasmic material that retained their bacterial size and surface display of antigens of the original bacterial cells. Heterologous antigens were anchored to the GEM particles by means of a lactococcal peptidoglycan binding domain called the protein anchor (PA). This binding domain is derived from the L. lactis AcmA cell-wall hydrolase and contains three repeats of a LysM-type cell-wall binding motif. Antigens, produced as antigen-PA fusions after nisin induction, were secreted into the culture growth medium and, after removal of the recombinant producer cells, were purified from the complex medium in a one-step procedure with the GEM particles. Antigen-PA molecules bind non-covalently and with affinity to GEM particles (106 PA/GEM particle) 15 times more strongly than they bind to living cells and multiple antigen-PA fusions can be bound to the same GEM particle. GEM-antigen vaccines are stable at room temperature, lack recombinant DNA, and can be used as antigen delivery particles and adjuvants capable of stimulating the mucosal and systemic responses of the immune system, a subject that will be dealt with in the next section.
Lactococcus lactis Nasally Administered as an Adjuvant and Delivery Vehicle of Pneumococcal Antigens
Lactococcus lactis as a nasal adjuvant.
The route of administration of a vaccine is crucial to the improvement in the defenses against respiratory pathogens. Classic vaccination pathways (intramuscular or subcutaneous) mainly lead to systemic immunization. However, the natural route of entry of most pathogens is the mucosa of the gastrointestinal, respiratory or urogenital tracts. Hence, in order to efficiently prevent respiratory infections, the activation of the mucosal immune system is essential.Citation43 Since numerous studies have demonstrated that the nasal administration of an antigen is the most efficient route to elicit optimal protective immunity in both the mucosal and the systemic immune compartments, nasal immunization is commonly used to induce immunity along the respiratory tract.Citation44 The nasopharyngeal associated lymphoid tissue (NALT) contains all the lymphoid cells required for the induction and regulation of the mucosal immune response to antigens delivered into the nasal cavity.Citation44 Hussell and HumphreysCitation45 suggested that NALT could fulfill an important role by reducing the pathogen burden to a level that would induce only minimal inflammation in the lower lung. Using an experimental model of S. pneumoniae infection, Cangemi de Gutierrez et al.Citation46 showed that the preventive intranasal inoculation of Lactobacillus fermentum caused a decrease in the number of pathogens throughout the respiratory tract together with an increase both in the number of activated macrophages in lung and in the lymphocyte population in the tracheal lamina propria. On the other hand, recombinant L. lactis NZ 9000 has been proven to elicit an immune response after inoculation by different mucosal routes.Citation47 In this respect, it has been reported that mice nasally immunized with a strain of L. lactis that expresses an M protein antigen were protected against pharyngeal infection following a challenge with Streptococcus pyogenes.Citation48 However, the immunological intrinsic properties of this lactococcus were not evaluated. Furthermore, the effect of nasal L. lactis administration on the local and the systemic immune response using a pneumococcal infection modelCitation49 was evaluated. Nasal administration of L. lactis cells increased the clearance rate of S. pneumoniae from lung and prevented the dissemination of pneumococci into blood. It seems probable that the L. lactis cells decreased both the adherence of S. pneumoniae to the respiratory epithelium and the number of pneumococci that reached the lung, since it has been reported that nasally administered LAB could competitively exclude pneumococcal cells.Citation50 In addition, the increased activation of macrophages observed in mice treated with L. lactis would induce a more effective neutrophil recruitment, as reported by Standford et al.Citation51 and Nelson and Summer.Citation52 The pulmonary lymphocyte population, which plays an important role in lung defense against infection,Citation53 was also significantly increased in the bronchoalveolar fluid (BAL) of mice after nasal administration of L. lactis. This fact would be related to the increase observed in BAL anti-pneumococcal IgA and IgG. Secretory IgA constitutes the main mediator of specific humoral immunity at mucosal surfaces by neutralizing bacterial toxins and viral particlesCitation54 and inhibiting adherence of bacteria to epithelial cells.Citation55 IgA may participate actively in protective phagocytosis and killing of S. pneumoniae once the inflammatory process is initiated,Citation56 Moreover, this immunoglobulin possesses intrinsic modulatory properties that stimulate the mucosal and systemic immune responses.Citation57 IgG would be involved in the protection against respiratory pathogens because of its ability to opsonise bacteria and, to a lesser extent, to mediate lysis by the complement.Citation58 Studies of lung tissue injury showed that nasal treatment significantly reduced the damage caused by the infection compared to the control. Nasal administration of L. lactis was also able to induce the activation of the systemic innate immune response, which was revealed by the increase in the microbicidal function of blood neuthophils and peritoneal cavity phagocytes.Citation49 The capacity of LAB to induce the production of cytokines is well documented,Citation59,Citation60 these mediator molecules being probably responsible for the above effect on blood and peritoneal cavity. Moreover, recent findings demonstrated in vitro that L. lactis NZ 9000 cells are able to upregulate the expression of MHC-II and CD86 co-stimulatory molecules in bone marrow derived dendritic cells.Citation61 Finally, in the bone marrow cells of mice the nasal administration of L. lactis showed: (a) a decrease in the percentage of the post-mitotic pool (metamyelocytes, band cells and neutrophils), probably related to the release of mature neutrophils and the production of granulocytes in response to inflammatory signals;Citation62 (b) an increase in bone marrow CD19+ cells, possibly related to the increase in serum anti-pneumococcal IgG; (c) a decrease in CD4+ cells, probably caused by the mobilization of cells towards the site of infection.Citation49 Then, preventive nasal administration of L. lactis at the appropriate dose was able to induce a local and systemic specific immune response.
As explained above, GEM particles have been used as a delivery system for mucosal vaccinationCitation63 with intrinsic immune stimulatory properties. Investigations have shown that these particles induced the production of the proinflammatory cytokine tumor necrosis factor-alpha (TNFα) by macrophages as well as the maturation of dendritic cells. In addition, GEM particles were able to activate in vitro the maturation of macrophages and dendritic cells, two important cell types that act as antigen presenting cells for the initiation of the innate immune system.
As shown above, the adjuvant effect of L. lactis would represent an important advantage for its use as a delivery vehicle of pneumococcal proteins. Thus, nasal immunization with recombinant L. lactis emerges as an effective route of vaccination for both systemic and mucosal immunity against pneumococcal infection.
Lactoccocus lactis as a nasal live vaccine.
Numerous studies have reported the successful stimulation of systemic and mucosal immunity in mice and humans with vaccines applied to mucosal surfaces.Citation64,Citation65 Gram positive food-grade bacteria such as lactococci have significant advantages over attenuated pathogens as vaccine delivery vehicles because of their inherently greater safety.
Lactococcus lactis NZ9000 is the main lactic acid bacterium employed as a vehicle of pneumococcal antigens and different pneumococcal proteins were expressed in this bacterium. Recombinant strains expressing the pneumococcal surface protein A (PspA), the pneumococcal surface adhesin A (PsaA) and the pneumococcal protective protein A (PppA) have been obtained and their protective effects on animal models are being evaluated.
PsaA is a surface-exposed common lipoprotein detected in all known serotypes of S. pneumoniae. This multi-functional and immunogenic protein is a lipoprotein component of an Mn2+ transporter and plays a major role in both pneumococcal attachment to the host cell and virulence. In addition, PsaA induces antibodies that would be able to reduce nasopharyngeal colonization, a major prerequisite for pneumococcal pathogenesis.Citation66 PsaA was expressed on the surface of different species of LAB,Citation67 including Lactococcus lactis, Lactobacillus casei, Lactobacillus plantarum and Lactobacillus helveticus (). Nasal immunization with recombinant L. lactis induced very low levels of IgA (in saliva, nasal and bronchial washes) and IgG (in serum) while high levels of anti-pneumococcal antibodies were induced by the other recombinant strains. Moreover, only vaccination with recombinant lactobacilli but not with lactococci led to a decrease in S. pneumoniae recovery from nasal mucosa after the colonization challenge. This was probably due to the low amount of PsaA in L. lactis and indicates the importance of the amount of the specific antigen expressed in recombinant strains to induce an adequate humoral response in the host. The selection of the LAB to be used as vaccine vectors should be addressed considering the intrinsic properties and the appropriate dose of each strain.
Another pneumococcal protein considered as a potential candidate for vaccines is PspA. This protein plays an important role during systemic infection through the inhibition of complement deposition on the pneumococcal surface with which the pathogen evades the immune system.Citation68 In this sense, Hanniffy et al.Citation69 evaluated the protection exerted by Lactococcus lactis intracellularly producing PspA as a nasal mucosal vaccine in a pneumococcal infection model. The recombinant lactococcal strain was able to protect the host when sepsis and respiratory pneumococcal models were used. This finding was correlated with a shift toward a Th1-mediated immune response associated with low levels of specific antibodies. This tendency was reverted when the inactivated recombinant strain was used as a nasal vaccine and a Th2 biased response was stimulated. Humoral immunity is important in the protection against S. pneumoniae but recent reports have indicated that T CD4+ cells would also play a significant role in the host's defenses against pneumococcal infections.Citation70 Thus, these results represent important advances in the understanding of the immune mechanisms induced by nasally administered recombinant strains.
Adult mice models were used when the protection against S. pneumoniae was evaluated after immunizations with recombinant lactococcal vaccines. Considering that children are an important high risk group in pneumococcal diseases, the preventive effect of lactococcal recombinant strains in both young and adult mice was studied. Nisin-controlled gene expression (NICE) was used to develop a recombinant strain of L. lactis that efficiently expressed the pneumococcal protective protein A (PppA) on its surface. We assessed the effectiveness of L. lactis-PppA to induce local and systemic immune responses in adult (6-week old) and young (3-week old) miceCitation71 using an immunization protocol that included three sequential doses of L. lactis nasally administered. Adult and young mice induced specific anti-PppA IgM, IgA and IgG antibodies in both serum and BAL. However, the specific antibody levels were significantly higher in immunized young mice than in the adult group while no significant differences in avidity were observed. In addition, similar levels of IgG1 and IgG2a antibodies were detected in BAL and serum in the two mice groups. The two IgG subtypes would contribute to the protection against pneumococcal infection, IgG2a through optimal complement fixationCitation72 and IgG1 through Fc receptor binding or by preventing pathogen attachment and mucosal colonization. Nasal immunization with recombinant L. lactis-PppA significantly increased the survival of young and adult mice against intraperitoneal challenge with S. pneumoniae compared to the controls that received PBS and non-recombinant L. lactis. This fact would indicate that live L. lactis-PppA is able to protect against systemic pneumococcal infection. The improved resistance to systemic pneumococcal challenge in passive immunization assays of naive young mice showed the protective effect of anti-PppA antibodies induced by nasal vaccination. In addition, cross-protective immunity against four pneumococcal serotypes was demonstrated. On the other hand, the host immune response was directed against the protein expressed by L. lactis and not against the vector, as demonstrated by the lack of antibodies against L. lactis in the serum or BAL of the immunized mice. An interesting contribution of this study was the comparative assessment of the humoral immune response induced when nasal recombinant immunization was started in young or adult mice. Results suggest that vaccination at an early age would be a better vaccination strategy for improved protection of the host against S. pneumoniae infection. However, the application of a live recombinant strain by the nasal route in humans still presents aspects that need to be resolved such as the elimination of the antibiotic resistance genes used in its selection. Induction of protective antibodies by a dead recombinant lactococcus expressing PspA was evaluated in a pneumococal infection model.Citation69 Results demonstrated that a better protection was obtained with the live than with the dead recombinant bacterium. However, the immunity induced by the inactivated recombinant strains was improved by combining them with a probiotic strain. Thus, the preventive effect of the nasal administration of L. lactis-PppA live (LL), inactivated (D-LL) and both LL and D-LL vaccines in association with the probiotic strain (Lactobacillus casei CRL 431) was evaluated in young mice.Citation73 Animals that received LL and D-LL vaccines in association with Lc by the oral (O) and nasal (N) route presented the highest levels of IgA and IgG anti-PppA antibodies in bronchoalveolar lavages (BAL) and IgG in serum. However, only the groups that received LL and D-LL vaccines associated with the oral administration of the probiotic were able to prevent lung colonization by S. pneumoniae serotypes 3 and 14 in a respiratory infection model.Citation73 The oral administration of the probiotic strain induced an evident improvement in the host's defenses when it was associated with inactivated and live recombinant bacteria. Both the LL + Lc (O) and the D-LL + Lc (O) combinations favoured the induction not only of protective specific antibodies but also of specific CD4+T cells, the full protection exerted against the pneumococcal challenge being the result of a balanced humoral and cellular immune response between the protective antibodies and the CD4+ Th1, Th17 and Th2 cells specific for the PppA antigen.Citation73 Although the LL + Lc (O) combination was effective in the protection against the infectious challenge, the D-LL + Lc (O) combination would be the better strategy for potential use as a nasal vaccine in humans. The results obtained show that the association of a probiotic with an inactivated vaccine would be a valuable alternative for application to human health, especially in at-risk populations. This work represents the first report of a safe and effective immunization strategy using an inactivated recombinant strain.
The potential use of GEM particles as a vaccine was also investigated with three pneumococcal antigens: the putative proteinase maturation protein A PpmA, the streptococcal lipoprotein A SlrA and the IgA1 protease IgA1p.Citation74 Monovalent GEM antigen vaccines (GEM particles containing PpmA, SlrA or IgA1p) elicited mucosal and systemic antibody responses in CD-1 mice intranasally immunized,Citation63 while intranasal immunization with a trivalent vaccine combination without an additional adjuvant showed significant protection against fatal pneumococcal pneumonia in mice. Immunization with a combination of PpmA-PA, SrlA-PA and IgA1p-PA (trivalent vaccine) and a combination of SrlA-PA/IgA1p-PA (divalent vaccine) were found to be much more protective against fatal challenge with S. pneumoniae than immunization with either of the proteins alone.Citation74 The GEM-based trivalent vaccine is a potential pneumococcal vaccine candidate that is expected to be safe, easy to administer and affordable to produce.
Oral Administration of Lactococcus lactis: Effects as Adjuvant and Carrier of Pneumococcal Antigens
Numerous studies have demonstrated that certain probiotic LAB strains can exert their beneficial effect on the host through their immunomudulatory activity.Citation75–Citation77 Although most research concerning probiotic-mediated enhanced immune protection is focused on gastrointestinal tract pathogens, a few recent studies have centred on whether probiotics might sufficiently stimulate the common mucosal immune system to provide protection to other mucosal sites as well.Citation77,Citation78 In this sense, our laboratory studied the potential effect of probiotics on the improvement of the immune response against respiratory pathogens using an experimental model of S. pneumoniae infection in adult inmunocompetent mice. In our experimental model, mice were intranasally infected with S. pneumoniae serotype 14. After the challenge, the pathogen was detected in lung and blood samples throughout the period assayed (15 days).Citation79 In order to study tissue damage in lungs, biochemical and histological studies were performed in the respiratory tract of infected mice. Challenge with pneumococci significantly increased bronchoalveolar lavage (BAL) albumin concentration and lactate dehydrogenase (LDH) activity, which indicates that the infection increased the permeability of the alveolar-capillarity barrier and the cell damage in lungs. Moreover, lung histopathological examination revealed a gradual and intense inflammatory response with progressive parenchymal involvement, including widespread cellular infiltration, passage of blood elements from capillaries to tissues, increased fibrosis in bronchial walls and vessels, hemorrhage and reduction in the alveolar airspaces.Citation79
Since the immunostimulating properties of LAB have been proved to be strain- and dose-dependent,Citation79–Citation83 the ability of LAB to increase resistance against S. pneumoniae infection was studied using several Lactobacillus and Lactococcus strains and different doses and periods of administration.Citation79,Citation84,Citation85 Only three of the treatments assayed increased the resistance of the mice to challenge with the respiratory pathogen: administration of Lactobacillus casei CRL 431, a probiotic strain with widely documented immunomodulatory properties,Citation79–Citation83 of Lactococcus lactis NZ 9000, a strain used for the expression of heterologous proteins,Citation86 and of Lactobacillus rhamnosus CRL 1505, a new probiotic strain with optimum technological properties isolated from goat milk.Citation85 These studies demonstrated that these strains, administered by the oral route at the proper dose, were able to increase S. pneumoniae clearance rates in lung and blood, improved survival of infected mice and reduced lung injuries.
In the gut immune response induced by commensal bacteria, the antigen presentation from the luminal flora leads to the generation of large quantities of local IgA. Moreover, the increase in the number of IgA-producing cells is the most remarkable property induced by probiotic microorganisms.Citation87 It has been demonstrated that the IgA+ cells in the lamina propria of the small intestine can be increased with orally administered LAB.Citation88,Citation89 It has also been demonstrated that this effect of LAB on gut immunity can significantly increase resistance to infections due to intestinal pathogens. In summary, it is now known that certain LAB strains are capable of: (a) protecting against experimental intestinal infections in mouse; (b) enhancing the secretory IgA specific for bacterial or viral pathogens in the gut; (c) improving the efficacy of oral vaccines against intestinal infections.Citation76 Moreover, a common mucosal immune system exists whereby immune cells stimulated in one mucosal tissue spread and relocate to various mucosal sites. This concept implies that oral immune stimulation can induce immunity in distal intestinal mucosal sites.
When studying the capacity of L. lactis NZ 9000 to stimulate the IgA cycle, we found that oral treatments with this lactic acid bacterium was capable of increasing the number of IgA+ cells in intestine and bronchus.Citation84 Thus, the stimulation of the IgA cycle induced by the LAB strains could partly account for the greater resistance of the treated mice to the challenge with S. pneumoniae, since LAB treatment improved the production of anti-pneumococcal IgA in the airways.
Our experiments demonstrated that L. lactis NZ9000 treatment also improved the production of anti-pneumococcal IgG in BAL. This fact could be related to the stimulation of APCs cells in the lung that induces T-cell activation and B cell clonal expansion and differentiation into IgG+ antibody-secreting plasma cells. Bearing the above in mind, we studied the activation of the recruited macrophages and the levels of TNFa in BAL after the challenge with the pathogen. Mice treated orally with L. lactis NZ9000 showed higher values of BAL TNFα and percentages of NBT+ cells than untreated mice, which would indicate that these treatments would be capable of improving macrophage-mediated antigen presentation.Citation84 Preventive treatments with LAB were able to increase the levels of IL-6 and TNFα in the respiratory tract after challenge with S. pneumoniae.Citation79,Citation84 These results would indicate that oral treatment with LAB would be capable of improving antigen presentation mediated by pulmonary dendritic cells. At present, our laboratory is working to demonstrate this effect.
Recombinant Lactococcus lactis PppA elicited specific anti-PppA mucosal and systemic antibodies after oral immunization. Adult immunocompetent mice orally immunized with 108 recombinant L. lactis PppA cells/mouse/day for 5 consecutive days (the optimal cell dose with adjuvant propertiesCitation84) induced the production of specific anti-PppA IgM, IgG and IgA in BAL and serum.Citation90 The levels, avidity and opsonophagocytic activity of respiratory and systemic specific IgA and IgG anti-PppA antibodies, but not those of specific IgM, were significantly improved with boosting.Citation91 The efficacy of the boosting immunization scheme to afford protection against S. pneumoniae infection was evaluated in adult mice challenged with pneumococcus of serotypes 3, 6B, 9, 14, 18, 19 and 23F.Citation91 These serotypes were selected taking into account that in our country they are the ones most often associated with invasive disease, greatest prevalence being shown by serotype 14.Citation92 The four serotypes studied were capable of infecting adult mice. S. pneumoniae serotype 3 was the most virulent, followed by serotypes 14 and 6B, while serotype 23F was less virulent.Citation91 In these studies, adult mice immunized twice with L. lactis PppA showed significantly lower lung bacterial cell counts than their respective control groups. Moreover, vaccination with L. lactis PppA was able to prevent blood dissemination of serotypes 6B, 14 and 23F and enabled the elimination of serotype 3 from blood on day 5 post-infection.Citation91
Most experimental vaccines designed to prevent pneumoccocal infections have been studied in infection models with adult immunocompetent mice with a view to their future application to high risk populations (children, the elderly and immunocompromised individuals). However, pneumococcal infectious disease is a major cause of human infant mortality and, therefore, one of the major challenges in vaccinology is the development of products that are able to induce protective immunity during early life. In this sense, it was found that oral immunization protocols with L. lactis PppA that are effective in inducing protective immunity in adult mice are able to protect young mice against pneumococcal respiratory infection.Citation91 Oral administration of recombinant lactic acid bacteria L. lactis PppA induced the production of specific antibodies both in the intestinal tract and at the systemic levelCitation91 of young mice. The efficient stimulation of the gut mucosal immune system by L. lactis PppA was evidenced by the increase in the number of IgA+ cells in the intestine and by the production of specific anti-PppA IgA antibodies in the intestinal fluid, while the efficient stimulation of the systemic immune response after vaccination with L. lactis PppA was evidenced by the detection of specific anti-PppA IgG antibodies in the serum. Recent in vitro studies showed that L. lactis NZ9000 has intrinsic adjuvant properties since it is able to stimulate the maturation of dendritic cells derived from bone marrow and to induce the production of cytokines with adjuvant properties such as IL-1β.Citation93 These properties and the adjuvant activities of L. lactis NZ9000 demonstrated in vivo in our laboratory would explain the efficacy of the oral immunization with L. lactis PppA to stimulate the specific immune response against the S. pneumoniae antigen in young mice. The analysis of IgG subtypes showed that L. lactis PppA immunization stimulated a mixture of the Th1 and Th2 response, which agrees with our previous studies in adult mice where oral immunization with L. lactis PppA was able to induce the production of IL-4 and INF-γ producing spleen cells.Citation91 Recent in vitro studies also showed that L. lactis NZ9000 is able to stimulate the production of both IL-12 and IL-10 by bone marrow derived dendritic cells.Citation93
Oral immunization of young mice with L. lactis PppA was also able to induce the production of specific IgA- and IgG-type antibodies in the respiratory tract. The mucosal and systemic antibodies induced by the oral immunization with L. lactis PppA afforded protection to young mice against an S. pneumoniae respiratory infection, although their bacterial counts in lung and hemocultures after challenge with different serotypes of the pathogen were significantly higher than the ones previously found in adult mice.Citation91 Thus, oral immunization of the young mice with L. lactis PppA was able to increase resistance to infection with the four serotypes of S. pneumoniae, although the protective capacity of the experimental vaccine was different for each serotype. Immunization decreased colonization in lung, prevented bacteriemia of serotypes 6B, 14 and 23F and decreased serotype 3 counts.Citation91
The protective effect in distant mucosal sites mediated by oral vaccines could be ascribed to the existence of a common mucosal immune system. When the mucosal immune response is induced, primed T and B cells migrate through the lymphatic system and enter the peripheral blood circulation via the thoracic duct. Extravasation of the immune cells occurs not only in the gut lamina propia but also in other mucosal sites such as the respiratory tract.Citation94 Thus, this homing pathway of primed lymphoid cells from the inductive sites in Peyer's patches to distant mucosal sites after antigen stimulation could be exploited to design recombinant L. lactis-derived oral vaccines that could afford protection against respiratory pathogens.Citation77
Conclusions
Search for new vaccination strategies that are inexpensive and can afford protection against different serotypes of S. pneumoniae is a worldwide priority. The safe status and the intrinsic adjuvant properties of some LAB represent an interesting alternative in the development of mucosal vaccines to fight this important pathogen. Two pneumococcal capsular polysaccharides and different pneumococcal proteins have so far been expressed in L. lactis and the effectiveness as antigen vehicles of some of these recombinant strains has been evaluated using animal models. However, comparison of the different vaccine delivery and adjuvant systems is difficult for several reasons that include antigen nature, dose and cellular location of the recombinant (cytoplasmic versus bound to the cell membrane versus bound to the cell wall), intrinsic antigenicity and immunomodulatory properties of the bacterial carrier, mouse strain and immunization protocol. Nasal immunization of young and adult mice with recombinant LAB is able to induce an effective specific immune response at both airways and systemic sites, which suggests that the nasal route is the best alternative for protection against a pneumococcal infection using L. lactis as an adjuvant and antigen delivery vehicle. Before the potential transfer of live vaccine to human health, one important aspect that needs to be resolved with live recombinant strains is the elimination of the antibiotic resistance genes used in its selection.
Inactivated vaccines have emerged as an excellent short-term alternative for clinical use. Although the immunogenicity of inactivated vaccines is reduced compared to the immunogenicity elicited by live vaccines, their administration associated with a probiotic strain has been proposed as an alternative strategy to successfully overcome this disadvantage, resulting in an even better proposal than the live vaccine by itself.
The variety of antigens evaluated, the progress made so far in the immune parameters involved in the protective effect of recombinant vaccines and the safety considerations of their application in humans suggest that the use of recombinant LAB as an antigen vehicle represents a tangible short-term option to control pneumococcal infections.
Figures and Tables
Figure 1 Innate immune response in lung. Alveolar macrophages constitute the first line of phagocytic defense against infectious agents and play a prominent role in lung immunity by initiating inflammation and immune responses. In the event that invading pathogens are too virulent or represent too large a load to be contained by macrophages alone, alveolar macrophages are capable of generating mediators that orchestrate the recruitment of large numbers of neutrophils from the pulmonary vasculature into the alveolar space. These neutrophils provide auxiliary phagocytic capacities that play a critical role in host defense against pathogens.
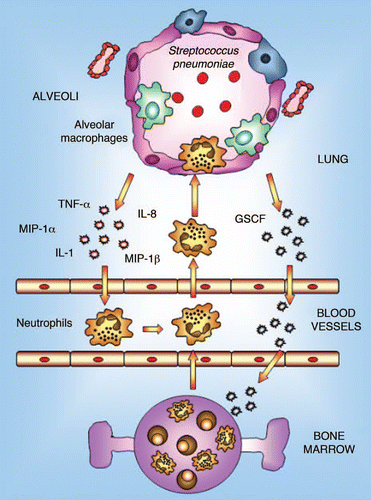
Figure 2 Antigen uptake and migratory pattern induction in the airways. The production of specific IgA in the respiratory tract during an infectious process is very important because it prevents colonization of mucosal sites and subsequent spreading into the systemic circulation.
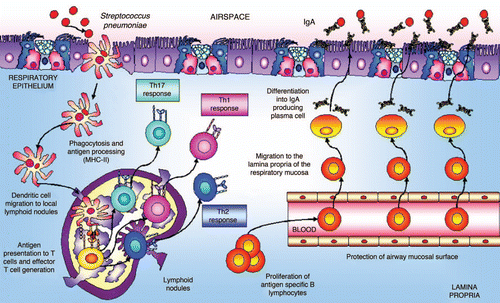
Figure 3 Antigen uptake and migratory pattern for immune induction in deep lung. IgG production plays an important role in the protection against pathogens that reach the alveolar space. Opsonizing IgG antibodies are important for complement fixation and for improvement in the efficiency of macrophages killing. At the systemic level, immune activation also induces production of antibodies responsible for preventing the passage of pathogens to the blood.
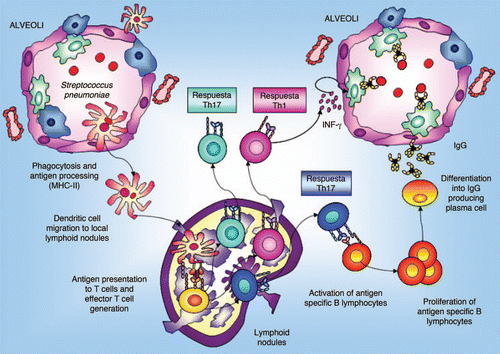
Figure 4 Schematic representation of the nisin-controlled gene expression (NICE) system (40) used to express the recombinant PppA pneumococcal protein in L. lactis. L. lactis NZ9000 cells containing the signal transduction genes nisK and nisR in their chromosome were transformed with a plasmid carrying the pppA gene under the control of the inducible promoter PnisA. Expression of the PppA recombinant protein was induced by the addition of sub-inhibitory amounts of the nisin and its insertion in the cell wall was mediated by the secretion (sec) and cell wall (CW) anchoring signals.
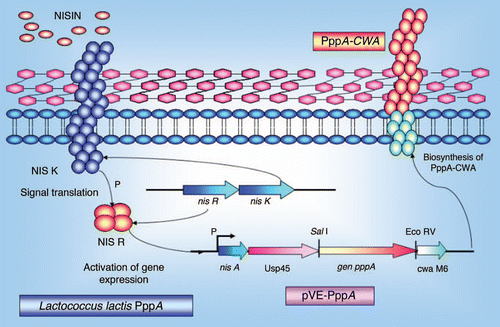
Table 1 Lactic acid bacteria as vaccine vehicles against S. pneumoniae
References
- Selwyn BJ. The epidemiology of acute respiratory tract infection in young children: comparison of findings from several developing countries. Coordinated Data Group of BOSTID Researchers. Rev Infect Dis 1990; 12:870 - 888
- Scott JA. The global epidemiology of childhood pneumonia 20 years on. Bull World Health Organ 2008; 86:494 - 496
- Mulholland K. Childhood pneumonia mortality, a permanent global emergency. Lancet 2007; 370:285 - 289
- Ruvinsky R, Gentile A, Regueira M, Corso A. Infecciones invasivas por Streptococcus pneumoniae: estudio epidemiológico e importancia del desarrollo de un sistema de vigilancia. Arch Argent Pediatr 2002; 100:31 - 43
- Michail SK, Stolfi A, Johnson T, Onady GM. Efficacy of probiotics in the treatment of pediatric atopic dermatitis: a meta-analysis of randomized controlled trials. Ann Allergy Asthma Immunol 2008; 101:508 - 516
- Sanz Y, Nadal I, Sánchez E. Probiotics as drugs against human gastrointestinal infections. Recent Pat Antiinfect Drug Discov 2007; 2:148 - 156
- Lim IS, Lee HS, Kim WY. The effect of lactic acid bacteria isolates on the urinary tract pathogens to infants in vitro. J Korean Med Sci 2009; 24:57 - 62
- Bermúdez-Humarán LG, Langella P, Cortes-Perez NG, Gruss A, Tamez-Guerra RS, Oliveira SC, et al. Intranasal immunization with recombinant Lactococcus lactis secreting murine interleukin-12 enhances antigen-specific Th1 cytokine production. Infect Immun 2003; 71:1887 - 1896
- Enouf V, Langella P, Commissaire J, Cohen J, Corthier G. Bovine rotavirus nonstructural protein 4 produced by Lactococcus lactis is antigenic and immunogenic. Appl Environ Microbiol 2001; 67:1423 - 1428
- Miyoshi A, Poquet I, Azevedo V, Commissaire J, Bermudez-Humaran L, Domakova E, et al. Controlled production of stable heterologous proteins in Lactococcus lactis. Appl Environ Microbiol 2002; 68:3141 - 3146
- Hausdorff WP, Feikin DR, Klugman KP. Epidemiological differences among pneumococcal serotypes. Lancet Infect Dis 2005; 5:83 - 93
- Vila-Córcoles A. Vaccinate your child and save its grandparents from a heart attack? Current perspectives in anti-pneumococcal vaccination. J Intern Med 21
- Fedson DS, Musher DM. Plotkin SA, Orenstein WA. Pneumococcal polysaccharide vaccine. Vaccines 2003; 4th ed Philadelphia Saunders 529 - 588
- Moberley SA, Holden J, Tatham DP, Andrews RM. Vaccines for preventing pneumococcal infection in adults. Cochrane Database Syst Rev 2008; 23:000422
- Lynch JP, Zhanel GG. Streptococcus pneumoniae: Epidemiology, risk factors and strategies for prevention. Semin Respir Crit Care Med 2009; 30:189 - 209
- Singleton RJ, Hennessy TW, Bulkow LR, Hammitt LL, Zulz T, Hurlburt DA, et al. Invasive pneumococcal disease caused by nonvaccine serotypes among Alaska native children with high levels of 7-valent pneumococcal conjugate vaccine coverage. JAMA 2007; 297:1825 - 1826
- Pletz MW, Maus U, Krug N, Welte T, Lode H. Pneumococcal vaccines: mechanism of action, impact on epidemiology and adaption of the species. Int J Antimicrob Agents 2008; 32:199 - 206
- Katsurahara T, Hotomi M, Yamauchi K, Billal DS, Yamanaka N. Protection against systemic fatal pneumococcal infection by maternal intranasal immunization with pneumococcal surface protein A (PspA). J Infect Chemother 2008; 14:393 - 398
- Ogunniyi AD, Grabowicz M, Briles DE, Cook J, Paton JC. Development of a vaccine against invasive pneumococcal disease based on combination of virulence proteins of Streptococcus pneumoniae. Infect Immun 2007; 75:350 - 357
- Cao J, Li D, Gong Y, et al. Caseinolytic protease: a protein vaccine which could elicit serotype-independent protection against invasive pneumococcal infection. Clin Exp Immunol 2009; 156:52 - 60
- Tai SS. Streptococcus pneumoniae protein vaccine candidates: properties, activities and animal studies. Crit Rev Microbiol 2006; 32:139 - 153
- Fujihashi K, Koga T, van Ginkel FW, Hagiwara Y, McGhee JR. A dilemma for mucosal vaccination: efficacy versus toxicity using enterotoxin-based adjuvants. Vaccine 2002; 20:2431 - 2438
- Villena J, Racedo S, Agüero G, Bru E, Medina M, Alvarez S. Lactobacillus casei improves resistance to pneumococcal respiratory infection in malnourished mice. J Nutr 2005; 135:1462 - 1469
- Racedo S, Villena J, Medina M, Agüero G, Rodríguez V, Alvarez S. Lactobacillus casei administration reduces lung injuries in a Streptococcus pneumoniae infection. Microbes Infect 2006; 8:2359 - 2366
- Holt PG, Strickland DH, Wikström ME, Jahnsen FL. Regulation of immunological homeostasis in the respiratory tract. Nat Rev Immunol 2008; 8:142 - 152
- Lambrecht BN. Alveolar macrophage in the driver's seat. Immunity 2006; 24:366 - 368
- Bergeron Y, Bergeron MG. Why does pneumococcus kill?. Can J Infect Dis 1999; 10:49 - 60
- Lambretch BN, Prins JB, Hoogsteden HC. Lung dendritic cells and host immunity to infection. Eur Respir J 2001; 18:692 - 704
- Dupuis M, McDonald DM. Dendritic-cell regulation of lung immunity. Am J Respir Cell Mol Biol 1997; 17:284 - 286
- Mosmann TR, Coffman RL. TH1 and TH2 cells: different patterns of lymphokine secretion lead to different functional properties. Annu Rev Immunol 1989; 7:145 - 173 Review
- Snapper CM, Paul WE. Interferon-gamma and B cell stimulatory factor-1 reciprocally regulate Ig isotype production. Science 1987; 236:944 - 947
- Korn T, Oukka M, Kuchroo V, Bettelli E. Th17 cells: effector T cells with Inflammatory properties. Semin Immunol 2007; 19:362 - 371
- Corthésy B. Recombinant immunoglobulin A: powerful tools for fundamental and applied research. Trends Biotechnol 2002; 20:65 - 71
- Kiyono H, Fukuyama S. NALT- versus Peyer's-patch-mediated mucosal immunity. Nat Rev Immunol 2004; 4:699 - 710
- Twigg HL. Humoral immune defense (antibodies): recent advances. Proc Am Thorac Soc 2005; 2:417 - 421
- Moore BB, Moore TA, Toews GB. Role of T- and B-lymphocytes in pulmonary host defences. Eur Respir J 2001; 18:846 - 856
- Wells JM, Mercenier A. Mucosal delivery of therapeutic and prophylactic molecules using lactic acid bacteria. Nature Rev Microbiol 2008; 5:349 - 362
- Gilbert C, Robinson K, Le Page RWF, Wells JM. Heterologous expression of an immunogenic pneumococcal type 3 capsular polysaccharide in Lactococcus lactis. Infect Immun 2000; 68:3251 - 3260
- Nierop Groot Mn, Godefrooij J, Kleerebezem M. Heterologous expression of the pneumococcal serotype 14 polysaccharide in Lactococcus lactis requires lactococcal epsABC regulatory genes. Appl Environ Microbiol 2008; 74:912 - 915
- Mierau I, Kleerebezem M. 10 years of the nisin-controlled gene expression system (NICE). Appl Microbiol Biotechnol 2005; 68:705 - 717
- Boesma T, Kanninga R, Neef J, Audouy SAL, van Roosmalen ML, Steen A, et al. Novel surface display system for proteins on non-genetically modified Gram-positive bacteria. Appl Environ Microbiol 2006; 72:880 - 889
- van Roosmalen ML, Kanninga R, El Khattabi M, Neef J, Audouy S, Bosma T, et al. Mucosal vaccine delivery of antigens tightly bound to an adjuvant particle made from food-grade bacteria. Methods 2006; 38:144 - 149
- Boersma WJA, Shaw M, Claassen E. Fuller R, Perdigón G. Probiotic bacteria as live oral vaccines Lactobacillus as the versatile delivery vehicle. Probiotics 3: Immunomodulation by the Gut Microflora and Probiotics 2000; 1st ed London Kluwer Academic Publishers 234 - 270
- Kiyono H, Fukuyama S. NALT-versus Peyer's patch mediated mucosal immunity. Nature Rev Immunol 2004; 4:699 - 710
- Hussell T, Humphreys IR. Nasal vaccination induces protective immunity without immunopathology. Clin Exp Immunol 2002; 130:359 - 362
- Cangemi de Gutierrez R, Santos V, Nader-Macías ME. Protective effect of intranasally inoculated Lactobacillus fermentum against Streptococcus pneumoniae challenge on the mouse respiratory tract. FEMS Immunol Med Microbiol 2001; 31:187 - 195
- Mercenier A, Muller-Alouf H, Grangette C. Lactic acid bacteria as live vaccines. Curr Issues Mol Biol 2000; 2:17 - 25
- Mannam P, Jones KF, Geller BL. Mucosal vaccine made from live, recombinant Lactococcus lactis protects mice against pharyngeal infection with Streptococcus pyogenes. Infect Immun 2004; 72:3444 - 3450
- Medina M, Villena J, Salva S, Vintiñi E, Langella P, Alvarez S. Nasal administration of Lactococcus lactis improves local and systemic immune responses against Streptococcus pneumoniae. Microbiol Immunol 2008; 52:399 - 409
- Fowler NG. Antimicrobials and competitive exclusion. Int J Food Microbiol 1992; 15:277 - 279
- Standford TJ, Kunkel SL, Greenberger MJ, Laichalk LL, Strieter RM. Expression and regulation of chemokines in bacterial pneumonia. J Leukoc Biol 1996; 59:24 - 28
- Nelson S, Summer WR. Innate immunity, cytokines and pulmonary host defense. Infect Dis Clin North Am 1998; 12:555 - 567
- Furfaro S, Berman JS, Center DM. Kradin RL, Robinson BWS. Determinants of T cell migration in the lung. Immunopathology of Lung Disease 1996; Boston: NJ Butterworth-Heinemann 15 - 32
- Mazanec MB, Kaetzel CS, Lamm ME, Fletcher D, Nedruf JG. Intracellular neutralization of virus by immunoglobulin A antibodies. Proc Natl Acad Sci USA 1992; 89:6901 - 6905
- Brandtzaeg P. Humoral immune response patterns of human mucosae: induction and relation to bacterial respiratory tract infections. J Infect Dis 1992; 165:167 - 176
- Janoff EN, Fasching C, Oresnstein JM, Rubins JB, Osptad NL, Dalmasso AP. Killing of Streptococcus pneumoniae by capsular polysaccharide-specific polymeric IgA, complement and phagocytes. J Clin Invest 1999; 104:1139 - 1147
- Favre L, Spertini F, Corthesy B. Secretory IgA possess intrinsic modulatory properties stimulating mucosal and systemic immune responses. J Immunol 2005; 175:2793 - 2800
- García VE, Gherardi MM, Iglesias MF, Cerquetti MC, Sordelli DO. Local and systemic immunity against Streptococcus pneumoniae: Humoral responses against a non-capsulated temperature-sensitive mutant. FEMS Microbiol Lett 1993; 108:163 - 167
- Miettinen M, Vuopio-Varkila J, Varkila K. Production of human tumor necrosis factor alpha, interleukin-6 and interleukin-10 is induced by lactic acid bacteria. Infect Immun 1996; 64:5403 - 5405
- Blum S, Delneste Y, Alvarez S, Haller D, Pérez PF, Bode C, et al. Interactions between commensal bacteria and mucosal immunocompetent cells. Internat Dairy J 1999; 9:63 - 68
- Yam KK, Pouliot P, N'diaye MM, Fournier S, Olivier M, Cousineau B. Innate inflammatory responses to the Gram-positive bacterium Lactococcus lactis. Vaccine 2008; 26:2689 - 2699
- Ueda Y, Kondo M, Kelsoe G. Inflammation and the reciprocal production of granulocytes and lymphocytes in bone marrow. J ExpMed 2005; 201:1771 - 1780
- Audouy SAL, van Roosmalen ML, Neef J, Kanninga R, Post R, van Deemter M, et al. Lactococcus lactis GEM particles displaying pneumococcal antigens induce local and systemic immune responses following intranasal immunization. Vaccine 2006; 24:5434 - 5441
- Tamura S, Ito Y, Asmuma H, Hirabayashi Y, Suzuki Y, Nagamine T, et al. Cross-protection against influenza virus infection afforded by trivalent inactivated vaccines inoculated intranasally with cholera toxin B subunit. J Immun 1992; 149:981 - 998
- Wilschut J, de Haan A, Geerligs HJ, Huchshorn JP, van Scharrenburg GJM, Palache AM, et al. Liposomes as a mucosal adjuvant system: an intranasal liposomal influenza subunit vaccine and the role of IgA in nasal anti-influenza immunity. J Liposome Res 1994; 4:301 - 314
- Rajam G, Anderton JM, Carlone GM, Sampson JS, Ades EW. Pneumococcal surface adhesin A (PsaA): a review. Crit Rev Microbiol 2008; 34:131 - 142
- Oliveira MLS, Areas APM, Campos IB, Monedero V, Perez-Martínez G, Miyaji EN, et al. Induction of systemic and mucosal immune response and decrease in Streptococcus pneumoniae colonization by nasal inoculation of mice with recombinant lactic acid bacteria expressing pneumococcal surface antigen A. Microbes Infect 2006; 8:1016 - 1024
- Tu AH, Fulgham RL, McCrory MA, Briles DE, Szalai AJ. Peumococcal surface protein A inhibits complement activation by Streptococcus pneumoniae 1999; 67:4720 - 4724
- Hanniffy SB, Carter AT, Hitchin E, Wells JM. Mucosal delivery of a pneumococcal vaccine using Lactococcus lactis affords protection against respiratory infection. J Infect Disease 2007; 195:185 - 193
- Cao J, Gong Y, Li D, Yin N, Chen T, Xu W, et al. CD4(+) T lymphocytes mediated protection against invasive pneumococcal infection induced by mucosal immunization with ClpP and CbpA. Vaccine 2009; 27:2838 - 2844
- Medina M, Villena J, Vintiñi E, Hebert EM, Raya R, Alvarez S. Nasal immunization with Lactococcus lactis expressing the pneumococcal protective protein A induces protective immunity in mice. Infect Immun 2008; 76:2696 - 2705
- Mawas F, Feavers IM, Corbel MJ. Serotype of Streptococcus pneumoniae capsular polysaccharide can modify the Th1:Th2 cytokine profile and IgG subclass response to pneumococcal-CRM197 conjugate vaccines in a murine model. Vaccine 2001; 19:1159 - 1166
- Vintiñi E, Villena J, Alvarez S, Medina M. Administration of a probiotic associated with nasal vaccination with inactivated Lactococcus lactis-PppA induces effective protection against pneumoccocal infection in young mice. Clin Exp Immunol 2010; 159:351 - 362
- Audouy SAL, van Selm S, van Roosmalen ML, Post E, Kanninga R, Neef J, et al. Development of lactococcal GEM-based pneumococcal vaccines. Vaccine 2007; 25:2497 - 2506
- Perdigon G, Fuller R, Raya R. Lactic acid bacteria and their effect on the immune system. Curr Issues Intest Microbiol 2001; 2:27 - 42
- Torres Vitela MR. Flora intestinal, probióticos y salud. Formas finas, eds 2002; Guadalajara, México 119 - 132
- Alvarez S, Villena J, Racedo S, Salva S, Agüero G. Malnutrition, probiotics and respiratory infections. Research Advances in Nutrition 2007; India Global Research Network, ed. 9 - 23
- Cross M. Microbes versus microbes: immune signals generated by probiotic lactobacilli and their role in protection against microbial pathogens. FEMS Immunol Med Microbiol 2002; 34:245 - 253
- Racedo S, Villena J, Medina M, Agüero G, Rodríguez V, Alvarez S. Lactobacillus casei administration reduces lung injuries in a Streptococcus pneumoniae infection. Microbes Infect 2006; 8:2359 - 2366
- Alvarez S, Herrero C, Bru E, Perdigón G. Effect of Lactobacillus casei and yogurt administration on prevention of Pseudomona aeuriginosa infection in young mice. J Food Prot 2001; 64:1768 - 1774
- Villena J, Racedo S, Aguero G, Bru E, Medina M, Alvarez S. Lactobacillus casei improves resistance to pneumococcal respiratory infection in malnourished mice. J Nutr 2005; 135:1462 - 1469
- Villena J, Racedo S, Aguero G, Alvarez S. Yogurt accelerates the recovery of defence mechanisms against Streptococcus pneumoniae in protein malnourished mice. Br J Nutr 2006; 95:591 - 602
- Salva S, Villena J, Racedo S, Alvarez S, Agüero G. Lactobacillus casei addition to a repletion diet induced early normalization of cytokine profiles during pneumococcal infection in malnourished mice. Food Agricult Immunol 2008; 19:195 - 211
- Villena J, Medina M, Vintiñi E, Alvarez S. Stimulation of respiratory immunity by oral administration of Lactococcus lactis. Can J Microbiol 2008; 54:630 - 638
- Salva S, Villena J, Alvarez S. Differential immunomodulatory activity of Lactobacillus rhamnosus strains isolated from goat milk: impact on intestinal and respiratory infections. Int J Food Microbiol 2009; In press
- Le Loir Y, Azevedo V, Oliveira SC, Freitas DA, Miyoshi A, Bermúdez-Humarán LG, et al. Protein secretion in Lactococcus lactis: an efficient way to increase the overall heterologous protein production. Microb Cell Fact 2005; 4:2 - 9
- Galdeano CM, de Moreno de LeBlanc A, Vinderola G, Bonet ME, Perdigón G. Proposed model: mechanisms of immunomodulation induced by probiotic bacteria. Clin Vaccine Immunol 2007; 14:485 - 492
- Perdigon G, Alvarez S, Medina M, Vintiñi E, Roux E. Influence of the oral administration of lactic acid bacteria on IgA producing cells associated to bronchus. Int J Immunopathol Pharmacol 1999; 12:97 - 102
- Perdigon G, Vintiñi E, Alvarez S, Medina M, Medici M. Study of the possible mechanisms involved in the mucosal immune system activation by lactic acid bacteria. J Dairy Sci 1999; 82:1108 - 1114
- Villena J, Medina M, Raya R, Alvarez S. Oral immunization with recombinant Lactococcus lactis confers protection against respiratory pneumococcal infection. Can J Microbiol 2008; 54:845 - 853
- Hausdorff WP, Bryant J, Kloek C, Paradiso PR, Siber GR. The contribution of specific pneumococcal serogroups to different disease manifestations: implications for conjugate vaccine formulation and use. Clin Infect Dis 2000; 30:122 - 140
- Villena J, Medina M, Racedo S, Alvarez S. Resistance of young mice to pneumococcal infection can be improved by oral vaccination with recombinant Lactococcus lactis. J Microbiol Immunol Infect 2010; 43:1 - 10
- Yam KK, Pouliot P, N'diaye MM, Fournier S, Olivier M, Cousineau B. Innate inflammatory responses to the Gram-positive bacterium Lactococcus lactis. Vaccine 2008; 26:2689 - 2699
- Kiyono H, Fukuyama S. NALT-versus Peyer's patch mediated mucosal immunity. Nat Rev Immunol 2004; 4:699 - 710
- Campos IB, Darrieux M, Ferreira DM, Miyaji EN, Silva DA, Áreas PAM, et al. Nasal immunization of mice with Lactobacillus casei expressing the pneumococcal surface protein A: induction of antibodies, complement deposition and partial protection against Streptococcus pneumoniae challenge. Microbes Infect 2008; 10:481 - 488