Abstract
The Bacterial Ghost (BG) platform technology is an innovative system for vaccine, drug or active substance delivery and for technical applications in white biotechnology. BGs are cell envelopes derived from Gram-negative bacteria. BGs are devoid of all cytoplasmic content but have a preserved cellular morphology including all cell surface structures. Using BGs as delivery vehicles for subunit or DNA-vaccines the particle structure and surface properties of BGs are targeting the carrier itself to primary antigen-presenting cells. Furthermore, BGs exhibit intrinsic adjuvant properties and trigger an enhanced humoral and cellular immune response to the target antigen. Multiple antigens of the native BG envelope and recombinant protein or DNA antigens can be combined in a single type of BG. Antigens can be presented on the inner or outer membrane of the BG as well as in the periplasm that is sealed during BG formation. Drugs or supplements can also be loaded to the internal lumen or periplasmic space of the carrier. BGs are produced by batch fermentation with subsequent product recovery and purification via tangential flow filtration. For safety reasons all residual bacterial DNA is inactivated during the BG production process by the use of staphylococcal nuclease A and/or the treatment with b-propiolactone. After purification BGs can be stored long-term at ambient room temperature as lyophilized product. The production cycle from the inoculation of the pre-culture to the purified BG concentrate ready for lyophilization does not take longer than a day and thus meets modern criteria of rapid vaccine production rather than keeping large stocks of vaccines. The broad spectrum of possible applications in combination with the comparably low production costs make the BG platform technology a safe and sophisticated product for the targeted delivery of vaccines and active agents as well as carrier of immobilized enzymes for applications in white biotechnology.
Introduction
Bacterial ghosts (BGs) are envelopes from Gram-negative bacteria which have been produced by controlled expression of the cloned lysis gene E. The essential role of gene E in the lysis of Escherichia coli after infection with bacteriophage φX174 was discovered in 1966.Citation1 More than 16 years later, when genetic engineering had been developed, it could be shown that its sole expression after cloning is sufficient to cause subsequent lysis of E. coli.Citation2,Citation3 E was the first lethal gene for bacteria which could be silenced on plasmids. When established in non-host range bacteria of the phage expression of E converts Gram-negative bacteria into BGs whereas Gram-positive bacteria are killed without lysis.
Gene E codes for a 91-aa polypeptide,Citation4,Citation5 which, in contrast to lytic proteins from other phages, has no inherent enzymatic function.Citation6,Citation7 E represents a membrane protein with the ability to oligomerize into a transmembrane tunnel structure.Citation8,Citation9 Analysis of the primary structure of protein E revealed a hydrophobic region at its N-terminal end suggesting a cotranslational integration into the cytoplasmic membrane of E. coli.Citation10 The observations that stationary phase host cells do not respond to E-lysis induction but lyse upon provision of fresh medium and other findings such as the inhibitory effect of non-physiological pH-values on the E-lysis process, indicate that E-mediated lysis is dependent on the growth phase of the host cells and its autolytic system.Citation9,Citation11–Citation13
Analysis of the hydropathicity regions of protein E indicated an E-specific lysis tunnel spanning the inner (IM) and outer membrane (OM) which most probably is located at membrane adhesion sites within the host cell.Citation14 E-mediated lysis forms the BG by releasing all cytoplasmic content to the environment while periplasmic components remained associated with the empty cell envelope.Citation9 The collapse of the bacterial membrane potential precedes the onset of E-lysis.Citation15 When the E-lysed E. coli were viewed by high-magnification scanning and transmission electron microscopy, the E-specific lysis tunnel was observed (). In addition, electron microcopic images showed that E- lysis of E. coli was accompanied by a fusion of the inner and outer membrane () sealing the periplasmic space (PPS).Citation16 Several investigations of E-lysed E. coli cells revealed that the E-specific lysis tunnel is located either at the centre or the poles of the bacteria, both potential division zones.Citation17 Since protein E-mediated lysis is dependent on the physiological state of the host bacteriumCitation18,Citation19 and analysis of E-lysis in different E. coli cell division mutants suggest that mechanisms involved in cell division are mandatory for E-lysis.Citation17,Citation20
The observed lysis tunnel diameter varies between 40 to 200 nm and does not show any regular structure. The driving force for the rapid discharge of the cell content is the osmotic pressure difference between the cytoplasm and the surrounding medium. However, the native structure of the peptidoglycan within the envelope complex remains intact and rigid.Citation21 The observed stimulation of peptidoglycan turnover by about 10%Citation21 would agree with genetic evidence that protein E inhibits MryA translocase A.Citation22
Based on these findings and extended experiments using an E-streptavidin fusion protein (E-FXa-StrpA), Schön et al.Citation23 described the process of E-mediated tunnel formation with a three-phase model: (1) integration of protein E into the IM with the C-terminus facing the cytoplasm; (2) conformational change of protein E translocating the C-terminal domain to the PPS accompanied by oligomerization and targeting of the division initiation complex via lateral diffusion; (3) fusion of IM and OM at membrane adhesion sites induced by exposition of the C-terminus of protein E to the cell surface. This model implies that the lysis tunnel is note solely bordered by protein E oligomers but its formation requires protein E-triggered fusion of the inner and outer membrane.Citation23 A schematic drawing of this model is shown in .
Upon discovery of the remarkable features of protein E-mediated lysis in E. coli, the principle of E-lysis could be shown with other Gram-negative bacteriaCitation24,Citation25 but not for Gram-positive bacteria.Citation26 So far, BGs of numerous Gram-negative strains (different E. coli strains, Salmonella typhimurium, Salmonella enteritidis, Klebsiella pneumoniae, Bordetella bronchiseptica, Heliobacter pylori, Vibrio cholerae, Actinobacillus pleuropneumoniae, Haemophilus influenzae, Mannheimia haemolytica, Pasteurella multocida, Pseudomonas aeruginosa, Pseudomonas putida, Ralstonia eutropha, Pectobacterium cypripedii and others) have been generated successfully. This suggests that the BG platform might be extended to any Gram-negative bacterium.Citation25 The idea of utilizing BGs derived from different Gram-negative bacteria as candidate vaccines emerged due to the demand for both potent and safe new vaccines.Citation27–Citation31 The BG system offers many advantages over traditional vaccination techniques including targeting and the intrinsic adjuvant properties of the BG particles. In addition, recombinant DNA technology facilitates the development of multivalent protein or DNA vaccines. Another great feature of BGs is the fact that no denaturing effects occur during E-lysis and hence all antigenic determinants are preserved throughout BG generation. The use of BGs as candidate vaccines and advanced drug carriers can be found in several recent reviews.Citation24,Citation27,Citation29,Citation32–Citation36
BG—Production Process
Initial cloning and expression studies with gene E used the inducible lac promoter/operator system with an overexpression of the lacI repressor gene (lac PO-lacIq1).Citation2,Citation3 Later the temperature-sensitive λ-system (λpL/λpR-cI857) has proven to be more suitable for quick and efficient lysis without the need of any addition of chemical inducers.Citation18 Since the λ repressor cI857 shows incipient expression of downstream gene E at temperatures above 30°C the temperature sensitivity of the system was optimized to meet more favorable fermentation temperatures of 35°C or higher. Mutations in the OR2 operator region of the λpR promoter resulted in tight repression of downstream genes up to 36°C and 39°C, respectively.Citation37,Citation38 These temperature-inducible E expression cassettes are widely used in current BG production processes since they are robust enough to allow fermentation of the bacterial culture at 35°C and induction of protein E-mediated lysis at 42 or 44°C.
In standard fermentations of various bacteria the quality criterion for a successful E-lysis process is a BG formation of at least 99.9% of the bacterial culture within a time window of 2 h. Depending on the host organism E-lysis efficiencies of more than 99.99% and higher can be achieved in this time frame. In the time-point of E-lysis induction is defined as time-point zero (0 min) with the preceding growth phase denoted in negative minutes.
BG production has been established in fermentation volumes up to 20 l using Labfors-3 and Techfors-S fermenters (Infors HT, Bottmingen, CH). Starting with a pre-culture that is growing exponentially, the production fermenter is inoculated with the starter culture at a volume ratio of 1:10. The standard fermentation process can be divided into three major stages: growth phase (90 min), E-lysis phase (120 min) and downstream processing.
The overall timeline for the production process is designed in such a way as the time from the automatic inoculation of the starter culture to the final concentration of the product the whole process takes 18 h and can be performed in one working cycle. The key events of the BG production process () are discussed in more detail below.
Growth phase.
The growth phase in an example fermentation with E. coli harboring plasmids for temperature-inducible E-lysis is conducted at 35°C, pH 7.20 and aeration parameters sufficient for exponential growth. To maintain a level of dissolved oxygen (dO2) of approximately 20% saturation both stirring and aeration rate are adjusted gradually over the course of the growth phase. After 90 min E-lysis of E. coli is induced at cell densities of approximately 1–2 × 109 cells/ml.
When recombinant proteins are expressed to become incorporated into the envelope complex (before E-mediated lysis) expression of the corresponding genes is induced chemically 30 min after inoculation (e.g., lac-, arabinose induction system). If synthesis of the foreign proteins slows down the growth rate so that lower cell densities are reached, this growth phase may be prolonged up to 120 min to compensate and increase the BG yield.
E-lysis phase.
E-lysis of the culture is induced by temperature up-shift from 35 to 42°C (). Currently, it takes roughly 10 min to reach the new temperature in the fermenter. During this time, stirring and aeration control is locked to prevent foaming during BG formation. The dO2-level subsequently drops below 5% and so remains for about 30 min. In the fermentation log visual evidence for E-lysis onset is a sudden signature increase of dO2 (). The E-lysis phase continues for a total of 120 min with its end being characterized by the dO2 reaching a stationary value of >95% saturation ().
Downstream processing.
The BG product is harvested from the fermenter via tangential flow filtration (TFF) in a 0.2 µm hollow fiber module at a temperature of 15°C. Firstly, the fermentation broth (20 l) is concentrated to 2.0 l () and transferred to a stirred reservoir. Then the concentrate is inactivated with β-propiolactone (BPL). Secondly, the BPL-treated broth is washed with sterile, de-ionized water (dH2O) by diafiltration in a smaller 0.2 µm hollow fiber module. A total of 5.0 l dH2O displaces the remaining medium and all residual cytoplasmic content. During the non-steady-state diafiltration, the product suspension is further concentrated to 400 ml (). The overall concentration factor is 50-fold while virtually all medium (>99%) is withdrawn. The final BG concentrate is divided into aliquots and lyophilized. As freeze-dried product, BGs are stable at room temperature for many years.
The TFF procedure as described above is an alternative to harvesting and washing BG via centrifugation. In contrast to the filtration process, this centrifugation is more laborious, time-consuming and might lead to BG aggregation because of difficulties with a proper re-suspension of the BG pellet. Another advantage of the implementation of TFF for harvesting and washing of BGs keeps all processes in a closed system and reduces the risk of cross-contamination during the handling procedure.
Process and quality control.
During fermentation all relevant process parameters (T, pH, dO2, aeration, stirring) are monitored and controlled. Starting from the time-point of inoculation (, −90 min) samples are taken every 30 min over the course of the fermentation () and analyzed for optical density (OD600) and colony forming units (cfu). All samples are also examined by light-microscopy and flow cytometry. Optionally, the biomass is also investigated for DNA content by real-time PCR and the level of protein E expression.
In standard fermentations with E. coli, the onset of E-lysis is linked to a sudden drop in OD600 of the culture broth and this simple determination is an important indicator of successful E-lysis induction. BG formation can also be observed as the appearance of translucent bacterial bodies in light microscopy. Both methods are good indicators for the quality of E-mediated lysis of E. coli but contain no quantitative information. The actual E-lysis efficiency is determined by cfu counting one day after sample collection. Flow cytometry has been established as a reliable real-time tool for the assessment of E-lysis onset and the progress of BG formation.Citation39 For flow cytometry diluted samples of the culture broth are stained with two fluorescent dyes and run through a CyFlow analyzer (Partec, Münster, Germany). The first dye (RH414) stains phospholipid membranes and its fluorescence signal defines a gate for the exclusion of all non-cellular background. The discrimination of living cells, dead but non-lysed cells and BGs is achieved by a combination of the forward scatter signal (FSC) and the fluorescence signal (FL1) of the second dye [DiBAC4(3)] which stains only cells that have lost their membrane potential. DiBAC-negative cells with a high scatter signal represent living cells, DiBAC-positive cells with a similar scatter signal represent the dead cell fraction. DiBAC-positive cells with a diminished scatter signal are identified as BGs. The general procedure for online monitoring E-lysis of E. coli by flow cytometry has been developed by Haidinger et al.Citation39,Citation40 and was adapted recently. The flow cytometry result for a given sample is available in less than 10 min after sampling. Representative dot-plots of an E. coli culture growth during the E-lysis process is shown in for the time-points induction (a), course (b) and end of the E-lysis phase (c).
After lyophilization the dry BG product is investigated with respect to sterility and re-suspensibility. For sterility investigations, 10 mg of BGs are re-suspended in rich medium and aliquoted for both nutrient agar plating and enrichment cultures. All sterility tests are performed in triplets to ensure that the final product does not contain any viable cells. The re-suspensibility is evaluated via flow cytometry with a lyophilized sample after re-suspension in dH2O. Since lyophilized BGs generally are easily rehydrated, the sample should give a similar picture and corresponding particle counts as the original sample.
BG—Inactivation
For the last 2 years, a new quality criterion stipulated that the harvested BG product should be free of any living cells before lyophilization. Although the efficiency of BG formation reaches three to five orders of magnitude during the time window of E- lysis ( and ), any remaining live cells must be inactivated subsequently. The presence of protein E in the envelope complex of bacteria does not necessarily kill all bacteria by E-lysis. However, protein E in the membrane renders all bacteria more acutely sensitive to killing by lyophilization and in the past no living cell counts could be detected in the lyophilized BG samples. In applications where nucleic acid-free BGs are produced, inactivation can be accomplished by the expression of an additional “kill gene” in the host cells in combination with E-lysis.Citation41 For this, the staphylococcal nuclease A (SNUC) is used, which reduces the DNA content below the detection limit of real-time PCR. SNUC activity is also responsible for cleaning up residual DNA in BGs and can lead to complete inactivation of the culture as it degrades the host DNA into fragments no longer than 100 base pairs.Citation41 Activation of the positive effect of SNUC expression, minimizing both cell viability and residual DNA-content in the BG product, is dependent on the addition of Mg2+ and Ca2+ as well as a shift in pH to 8.0.41 shows a Shigella flexneri 2a culture harboring plasmid pGLNic for co-expression of temperature-inducible protein E and IPTG-inducible SNUC.
Addition of the alkylating agent β-propiolactone (BPL) after harvesting is effective in fully inactivating all viable cells either in combination with or as an alternative to SNUC. BPL is known to react with nucleic acids, mainly guanine. BPL is widely used for the inactivation of viruses and further to sterilize vaccines, human tissue implants and plasma.Citation42 The presence of BPL causes alterations (transition mutations, cross-linking, nicks) in nucleic acids. The presence of water fully hydrolyses BPL at room-temperature into non-toxic β-hydroxypropionix acid.Citation42 In BG production, the amount of BPL needed for complete inactivation of the BG product suspension depends on three parameters: the amount of DNA present in the suspension, time and temperature. Most DNA in the BG suspension is present in the liquid phase due to the expulsion of the cytoplasmic content, which makes it reasonable to apply BPL in the BG concentrate after harvesting but before diafiltration. At this point approximately 97% of the original fermentation liquid—and therefore 97% of the free DNA—has been removed from the product. Two equal dozes of BPL given at 30 min intervals are sufficient for total inactivation of all surviving cells at 42°C within 60 min. The final BG product is washed with another 5.0 l dH2O by diafiltration before dispensing into aliquots for lyophilization.
BGs—Applications
BGs solo.
Immunization against pathogenic Gram-negative bacteria using BGs has been studied in various animal models.Citation24,Citation36
BGs have been used in model investigations for human lung pathogens and for the development of veterinary vaccine candidates vaccination of swine with A. pleuropneumoniae (App). AppBGs resulted in protection against aerogenic infection with the potentially lethal pathogen. They also prevented colonization of the lungs and tonsils which indicated that immunization with BGs is superior to treatment with bacterins.Citation43 More importantly, no clinical side-effects have been reported.
The application of BGs via mucosal inoculation is superior to parental inoculation. The mucosal application of App BGs as oral immunizationCitation44 or as aerosolsCitation43,Citation45 induced sterile immunity and cross-protection against other serotypes in pigsCitation45 whereas intramuscular immunizationCitation46 fully prevented the vaccinated pigs against the disease after lethal challenge but did not confer sterile immunity because the challenge bacteria could be re-isolated from the tonsils from the vaccinated pigs.
BG produced from P. multocida and M. haemolytica (formerly: P. haemolytica) were used in rabbit and mice models. The antibodies produced were cross-protective; effective not only against the strain used for immunization but also against other Pasteurella strains.Citation47 M. haemolytica BG immunization of cattle offered protective immunity comparable to commercially available vaccines.Citation48
For V. cholera pre-clinical studies have been completed. The ilea loop challenge model revealed full protection of rabbits. Interestingly, partial cross-protection between the classical O1 strain and the new upcoming O139 strain was observed.Citation49 In most models mucosal application has proven to be a favorable route for administration of BG candidate vaccines inducing both humoral and cellular immune response.Citation29,Citation36
BGs as adjuvants.
The BG morphology is not subject to denaturation during the lysis process. Thus all major immune stimulating elements are preserved. Those elements are referred to as pathogen-associated molecular patterns (PAMPs) and include lipopolysaccharides (LPS), monophosphoryl lipid A (MPL), peptidoglycan or flagella. As PAMPs are recognized by toll-like receptors (TLR) they trigger also the innate immune response. Consequently, all bacterial strains from which the BGs are derived induce innate immune reactions (Abtin, Koller, Lubitz, personal communication) as first response. They also carry intrinsic adjuvant properties which makes them extremely versatile to induce specific humoral and cellular immune responses in experimental animals.Citation29
BGs as carriers of foreign protein antigens.
Using recombinant DNA technology, foreign antigens (AGs) can be incorporated into or become associated with the envelope complex of the bacteria before lysis and become elements of the BGs (). AGs may be presented on the cell surface via fusion with outer membrane proteins (e.g., ompA)Citation50 or on the IM as membrane anchor fusions with N-, C- or N/C-terminal targeting.Citation30 Fusion with these membrane anchors did not affect proper folding and assembly or diminish the functionality of enzymes supporting the assumption that AGs are in their correct conformation. In addition to directly fusing the target AG to the membrane anchor, a system for subsequent loading of BGs with AGs was developed. In this approach the BGs are equipped with membrane-anchored streptavidin. After lyophilization such streptavidin BGs can be loaded with a desired biotinylated compound.Citation51
Another method of incorporating foreign proteins into BGs is the directed export to the PPS via MalE fusion proteins or PPS signal sequences. The PPS is sealed during lysis and the vast majority of all periplasmic components are retained within the envelope complex.Citation9,Citation16 The membrane-derived oligosaccharidesCitation52 of the PPS provide a protective environment against inactivation during lyophilisation.Citation34
Fusions of target antigen DNA sequences with the bacterial surface layer (S-layer) genes sbsA or sbsB of Bacillus stearothermophilus, when expressed heterologous in Gram-negative bacteria, form sheet-like self-assembling superstructures within the cytoplasmic space.Citation53,Citation54 Since S-layers are made up of several 100,000 subunits, they are not expelled with the cytoplasm during E-lysis. Both S-layer genes accept insertion of foreign sequences coding for large foreign proteins.Citation54,Citation55 Linking MalE to SbsA the protein subunits can also be exported to the PPS prior to S-layer formation.Citation56 All different options of AG presentation in BG envelopes are summarized in as a schematic drawing.
BGs as carrier of biologically active substances.
The BG system provides a new promising platform for the delivery of drugs and other biologically active substances. BGs are devoid of any cytoplasmic content so the carrier capacity of the inner cytoplasmic lumen provides an intracellular space of approximately 250 femtoliter per BG. This lumen can be filled with drugs of interest as liquid or absorbed to the lipid compartment (independent of the inner volume) or specifically attached to receptors presented in the BGs.
BGs produced from M. haemolytica were used for the in vitro delivery of the moderate hydrophilic cytostatic drug doxorubicin (DOX) to human colorectal adenocarcinoma (Caco-2) cells. Endogenous drug release was confirmed. Enhanced cytotoxic and antiproliferative activities in the Caco-2 cells were observed. DOX-loaded BGs were 2–3 orders of magnitude more effective compared to the substance alone.Citation57 The water soluble substance calcein was used in another delivery model, whereby the former lysis holes were plugged with bacterial membrane vesicles.Citation58
BGs from P. cypripedii were used as pesticide delivery systems with the lipophilic fungizide tebuconazole. The investigations demonstrated that this formulation conferred a higher resistance to rainfalls due to adherence of the BGs to the plant. This BG application showed protective and curative effects against agricultural plant pathogens.Citation59
It is assumed that organic ring structures bind unspecifically to the membrane compartments of BGs. Recent investigations of loading BGs with polyphenolic compounds, like resveratrol, agree with this assumption. Currently, the modulating ability of such substances bound to BGs to induce the innate immune system, i.e., iNOS, was examined (Koller and Lubitz, personal communication). Furthermore, increased drug cytotoxicity was demonstrated. We predict that this effect was due to stabilization and protection of the UV-labile resveratrol derivates, (Digalloylresveratrol and M8) by adsorption to the BG-interior (Koller and Lubitz, personal communication).
Model investigations for enhanced binding of drugs to a substituted matrix on the inner membrane of BGs have used membrane anchored streptavidin which bound the biotinylated coupling partner to the inside of the cytoplasmic membrane. Biotinylated alkaline phosphatase or biotinylated fluorescence-labelled dextrans displayed successful binding within the inner lumen of BGs.Citation51
BGs as carriers of DNA vaccines.
Conventional viral and bacterial vaccine delivery systems with high transfection efficiencies bear a risk of reversion to their original pathogenic forms. “Safer” non-viral systems such as attenuated bacteria, polycation/DNA complexes, nucleoporation have reduced transfection efficiencies.Citation60–Citation67 The BG system represents an alternative to current viral and bacterial methods in vaccine development with a new highly efficient gene delivery platform. One of the biggest advantages of the new DNA-carrier system is the safety of BGs. Recent in vitro investigations proved that BGs have no cytotoxic or genotoxic impact on different types of human cells after mutual co-incubation. This observation was independant of the BG species used (Koller and Lubitz, personal communication).
Recently, DNA vaccines were approved for use in veterinary practice.Citation68 DNA vaccines still require intensive research and improvements before they are considered safe for use in human medicine. One reason for this slow pace in development and licensing approval of DNA vaccines is the requirement of high plasmid dosages and low immunogenicity, most commonly attributed to the absence of efficient delivery system.Citation69,Citation70 Many experiments have been carried out in order to deliver DNA vaccines using BGs as carriers, and a simple procedure for loading BGs with plasmid DNA has been standardized. Lyophilized BGs are re-suspended in DNA solutions followed by a couple of washing steps to remove unbound plasmid DNA from inside the BGs. The amount of DNA loaded inside the BGs is directly related to the concentration of DNA solution used. This loading procedure is very efficient and up to 6,000 midsize plasmid copies per BG can be loaded.Citation71
One of the main advantages of BGs is that they are non-living. They retain all of the surface morphological, structural and antigenic components of their living counterparts. BGs also have an outstanding loading capacity.Citation72 The inner space of BGs empty envelope can be loaded with a combination of peptides, drugs or foreign DNA which gives us an opportunity to design new types of polyvalent vaccines.Citation57,Citation71,Citation73,Citation74 We have shown that BGs loaded with plasmid DNA encoding green fluorescent protein (GFP) are efficiently internalized and phagocytized by both professional antigen presenting cells (APCs) and tumor cells. BGs were able to deliver the heterologous genes to both non-dividing cells (monocyte-derived dendritic cells) and dividing cells (macrophages and melanoma). Study results showed that up to 82% of cells expressing the plasmid encoded reporter gene delivered by BGs. Importantly, no cytotoxic impact was observed on target cells.Citation32,Citation71,Citation74,Citation75 Intradermal and intramuscular immunization of Balb/c mice with BGs loaded with pCMV encoding beta-galactosidase stimulated more efficient humoral and cellular AG-specific immune responses than naked DNA. Beta-galactosidase-specific immune response was detected after intravenous immunization of mice with autologous dendritic cells (DCs) transfected ex vivo with pCMVbeta-loaded BGs.Citation32 An increase of IFN-gamma producing AG-specific CD8+ T cells was observed in animals vaccinated with DNA loaded BGs in response to restimulation by APCs pulsed with peptide containing the immunodominant MHC class I epitope. BGs enhanced expression of MHC class I molecules and costimulatory molecules on DCs.Citation32 Cross-presentation of AGs delivered to DCs by BGs could activate both CD4+ and CD8+ T cells and stimulates the immune system to enhance immune response against AGs expressed by target cells. Bacterial LPS enhances maturation of DCs, affects endosomal acidification of DCs and also improves cross-presentation of AGs.Citation76,Citation77 Inner and outer membrane structures of BGs including LPS remain intact in BGs and the surface LPS effectively stimulate the AG-cross-presentation by DCs.Citation17,Citation72
In general the production and loading of BGs with plasmid DNA are two separate tasks. With the introduction of our new self immobilizing plasmids (pSIP) this multistep procedure was simplified into one step in-vivo, cost effective procedure. During this process the plasmid DNA carrying an operator sequence is bound to a specific DNA binding protein present on the IM of the bacteria.Citation78 The bacterial backbone sequences and antibiotic resistant genes are considered to be a biological safety risk for DNA vaccination and plasmid DNA used in gene therapy. To overcome this hurdle, new more sophisticated versions of pSIP BG-DNA-vaccines, based on minicircle (mc) DNA devoid of such biologically risky remnants were developed. This improved version of pSIP is based on the ParA resolvase system to produce mcDNA which is bound to the IM receptor. The corresponding sister pair miniplasmid produced during this process is expelled to the culture media during the gene-E mediated lysis.Citation79 A modified system for minicircle production, digesting the miniplasmid has been reported, based on endonuclease activity of I-SceI gene encoded from parent plasmid.Citation80 A new modified pSIP generation is currently under construction with encoded endonuclease activity to digest non-recombinant mother plasmids and the ParA produced miniplasmids.
The main benefit of DNA vaccines is the induction of both cellular and humoral immune responses. Processing of AG through both endogenous and exogenous pathways followed by AG epitopes present both MHC class I and class II molecules.Citation81–Citation83 Well designed and applied gene therapy should provide successful delivery of desired AG DNA to the APCs. This is followed by its expression, naturally processing and presentation of AG-derived epitopes. T cells raised against delivered, naturally processed and presented AGs by APCs might be more effective in recognition of the same epitopes presented by cells expressing identical AGs. The expression of a delivered gene should induce strong immune responses or change the behavior of targeted cells.
BGs with their intact envelope structures include peptidoglycan and LPS. These elements are not only “waking up” professional phagocytic APCs but are also providing stimulatory impulses to tumor cells. It is known that e.g., melanoma cells have the capacity to behave as non-professional APCs and can phagocyte both apoptotic and live cellsCitation84–Citation87 and as recently shown respond to challenge by BGs.Citation73 Despite the high DNA loading capacity of BGs, relatively low concentrations of DNA are sufficient for effective gene delivery and expression by melanoma cells. High transfection efficiencies were obtained after incubation of BGs with melanoma cells. Similar results were seen with monocyte-derived DCs encouraging us to design BGs carrying selected immunogenic and immunodominant AGs. These DNA loaded BGs would be used simultaneously for gene transfer to both professional APCs and to tumor cells to induce or amplify AG-specific immune responses.
White biotechnology—BGs as micro-bioreactors for enzymatic reactions. Another possible application for the BG platform is the use of BGs as enzyme carriers. The lack of cytoplasm and of a membrane potential due to E-mediated lysis of the bacteria does not lead to a total loss of enzymatic activities. The enzymatic activities of BG membrane-bound β-galactosidase and chloramphenicol acetyl transferase have been described.Citation88,Citation89 Membrane associated enzymes like ATPases are still functionally active in BGs. Moreover, even though the cytoplasmic content is expelled during lysis, the inside of the cytoplasmic membrane and its associated products are retained. As the IM and OM are fused at the border of the E-specific lysis tunnel enzymes from the PPS like alkaline phosphatase and β-lactamase are largely retained and active.Citation9,Citation17 ATPase and β-lactamase sustained relative activities in suspended BGs even after one week storage at 4°C. Enzyme activities were also detectable in lyophilized BG-batches stored long-term at ambient temperatures. The enzyme activities were similar to those of recently produced freeze-dried samples, e.g., ATPase activity no significant differences in enzyme activity were observed after five years of storage (Koller, Lubitz—personal communication). This data confirms that BGs enzymes stay functionally preserved during long storage, which indicates the potential of BGs as reservoirs for biological functions e.g., as dietary enzyme substitution or for other use.
BGs can act as micro-reactors which follows the idea of Pfründer et al. of producing enantiopure fine chemicals such as e.g., asymmetric synthesis of a 3,5-dicarboxyhydroxylate in biphasic ionic liquid/water systems.Citation90,Citation91 Potent enzymes [e.g., specialized alcohol dehydrogenases (ADHs)] are anchored to the IM while the internal lumen of the BG becomes the reaction space. Re-suspension of the BGs in an aqueous solution with a suitable reduction equivalent allows for proper function of the desired enzyme. Both product and educts in these kinds of reactions are often poorly water-soluble. For this reason, the use of a non-polar solvents such as a suitable ionic liquid (IL)—is essential. IL are organic salts which are liquid at ambient temperatures; due to their low vapor pressure they are considered as safe (“green solvents”). They feature good in-situ extraction properties for product recovery.Citation92 BGs loaded with the reduction equivalent solution are dispersed in the ionic liquid. Thus this BG system provides the substrate and receives the product. It was shown that the enzyme activity of β-galactosidase could be vastly increased in an IL environment.Citation93 Preliminary studies of our lab have demonstrated that β-galactosidase was active when BGs were re-suspended in the IL [Bmim]PF6.
Qualitative determinations showed successful hydrolysis reactions of the substrates which were delivered in the ionic liquid. These findings give a first indication of the feasibility and attainable enzymatic activity of such approaches. When a multi-step enzyme system is introduced a limited series of reactions could be performed within one BG. Therefore the BG system could become a versatile vehicle in white biotechnology.
Abbreviations
BG | = | bacterial ghost |
IM | = | inner membrane |
OM | = | outer membrane |
PPS | = | periplasmic space |
cfu | = | colony forming units |
dO2 | = | dissolved oxygen |
TFF | = | tangential flow filtration |
BPL | = | β-propiolactone |
dH2O | = | de-ionized water |
OD600 | = | optical density at 600 nm |
FSC | = | forward scatter |
FL1 | = | fluorescence signal 1 |
IPTG | = | isopropyl β-D-thiogalactopyranoside |
App | = | A. pleuropneumoniae |
PAMP | = | pathogen-associated molecular pattern |
LPS | = | lipopolysaccharide |
MPL | = | monophosphoryl lipid A |
TLR | = | toll-like receptor |
AG | = | antigen |
CPS | = | cytoplasmic space |
DOX | = | doxorubicin |
Caco-2 | = | colorectal adenocarcinoma |
DC | = | dendritic cell |
pSIP | = | self-immobilizing plasmid |
GFP | = | green fluorescent protein |
mc | = | minicircle |
APC | = | antigen-presenting cell |
ADH | = | alcohol dehydrogenase |
IL | = | ionic liquid |
Figures and Tables
Figure 1 (A) Lysis tunnel formation and expulsion of the cytoplasmic contents—reproduced from Ebensen et al.Citation32 (B) Lysis tunnel formation, accompanied by the fusion of IM and OM (arrow)—reproduced from Witte et al.Citation16
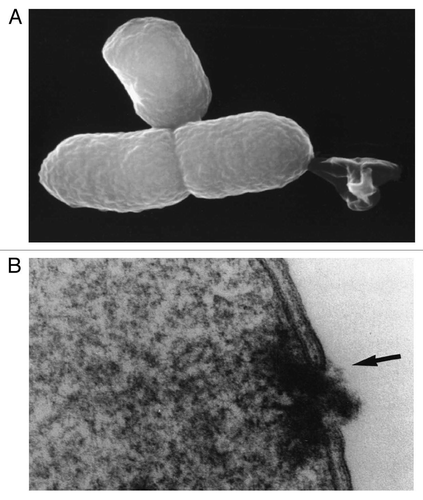
Figure 2 (A) Different methods for AG presentation in the BG envelope complex—BG themselves carry native AG (LPS, OMP, IMP, TCP, flagella, pili)—TA may be presented on the cell surface via fusion with OmpA—the PPS can be loaded with TA via MBP-SbsA-fusion proteins (1), by fusion of the TA with MBP (2) or as sole TA using the gene III signal sequence (3) Protein TA may be incorporated into the IM via E′, L′ or E′/L′-anchoring, biotinylated AG can be attached to E′-FXa-StrpA membrane anchors, DNA carrying the lac operator site can be attached to L′-anchored lacI repressor molecules—TA fused with SbsA-/SbsB proteins form S-layers in the PPS. (B) Model of lysis tunnel formation according to Schön et al.Citation23
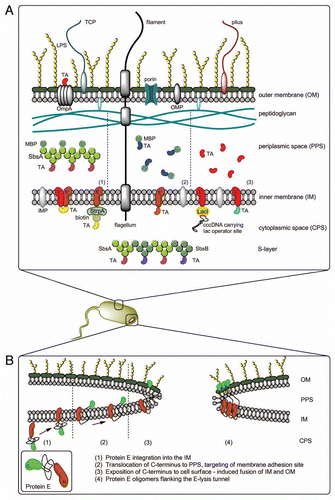
Figure 3 Fermentation protocol (growth/lysis phase) monitoring all relevant process parameters; (a) lysis induction, (b) lysis onset as indicated by dO2 up-shift, (c) stationary dO2 plateau indicating end of lysis phase.
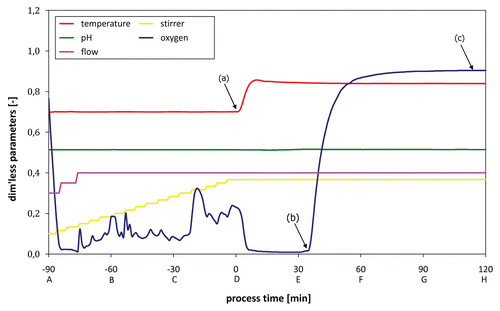
Figure 4 Process timeline for the production of BG including the pre-culture (ON) and downstream processing.
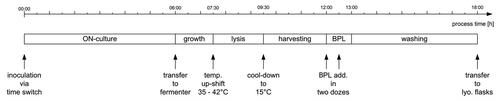
Figure 5 (a) Harvesting of the BG product via TFF; concentration from 20 to 2 l in the fermenter. (b) Washing of the BG product with 5.0 l dH2O via diafiltration; concentration from 2.0 l to 400 ml in a stirred reservoir.
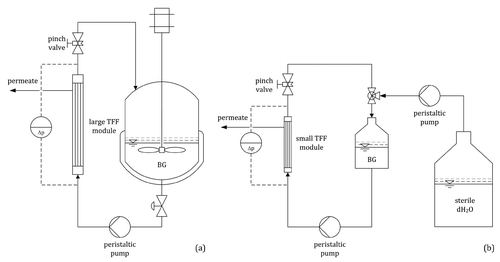
Figure 6 Flow cytometry pictures following the progress of lysis in an E. coli NM522 culture (pGLysivb); R1: living cells, R2: dead but intact cells, R3: lysed cells (BG); RN6: exclusion of non-cellular background with RH414 (not shown); FSC - forward scatter, FL1 - fluorescence intensity by DiBAC4(3); (a) sample D (0 minutes, lysis induction), (b) sample E (30 minutes), (c) sample H (120 minutes, end of lysis phase).
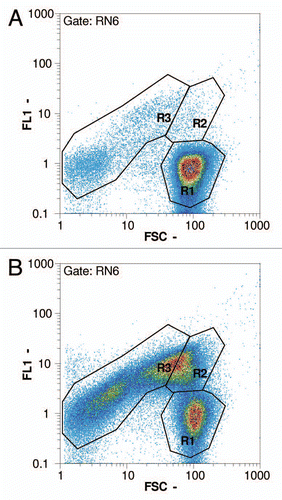
Figure 7 Standard fermentation for a S. flexneri 2a culture harboring plasmid pGLNic: (a) IPTG-addition at −45 min to induce biosynthesis of SNUC, (b) temperature up-shift to 42°C at 0 min to induce lysis, (c) pH up-shift to 8.0 and addition of Mg2+ and Ca2+ at +90 min to activate the enzymatic function of SNUC.
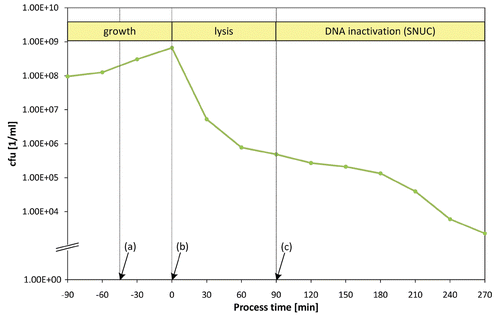
Acknowledgements
We would like to thank Jacqueline Montanaro for critical reading of the manuscript. This work was supported by BIRD-C.
References
- Hutchinson CA III, Sinsheimer RL. The process of infection with bacteriophage phiX174. X. Mutations in a phiX174 lysis gene. J Mol Biol 1966; 18:429 - 447
- Henrich B, Lubitz W, Plapp R. Lysis of Escherichia coli by induction of cloned phiX174 genes. Mol Gen Genet 1982; 185:493 - 497
- Young KD, Young R. Lytic action of cloned phiX174 gene E. J Virol 1982; 44:993 - 1002
- Barrell BG, Air GM, Hutchison CA 3rd. Overlapping genes in bacteriophage phiX174. Nature 1976; 264:34 - 41
- Pollock TJ, Tessman ES, Tessman I. Identification of lysis protein E of bacteriophage phiX174. J Virol 1978; 28:408 - 410
- Denhardt DT, Sinsheimer RL. The process of infection with bacteriophage phi-X174. 3. Phage maturation and lysis after synchronized infection. J Mol Biol 1965; 12:641 - 646
- Markert A, Zillig W. Studies on the lysis of Escherichia coli C by bacteriophage PhiX174. Virology 1965; 25:88 - 97
- Bläsi U, Linke RP, Lubitz W. Evidence for membrane-bound oligomerization of bacteriophage phiX174 lysis protein-E. J Biol Chem 1989; 264:4552 - 4558
- Witte A, Lubitz W. Biochemical characterization of phiX174-protein-E-mediated lysis of Escherichia coli. Eur J Biochem 1989; 180:393 - 398
- Bläsi U, Geisen R, Lubitz W, Henrich B, Plapp R. Localisation of the bacteriophage phiX174 lysis gene product in the cell envelope of Escherichia coli 1983; Berlin & New York de Gruyter
- Halfmann G, Leduc M, Lubitz W. Different sensitivity of autolytic deficient Escherichia coli mutants to the mode of induction. FEMS Microbiology Letters 1984; 24:205 - 208
- Halfmann G, Lubitz W. Differential induction of Escherichia coli autolysis by penicillin and the bacteriophage phi X174 gene E product. J Bacteriol 1986; 166:683 - 685
- Lubitz W, Pugsley AP. Changes in host cell phospholipid composition of phiX174 gene E product. FEMS Microbiology Letters 1985; 30:171 - 175
- Bayer ME. Areas of adhesion between wall and membrane of Escherichia coli. J Gen Microbiol 1968; 53:395 - 404
- Witte A, Lubitz W, Bakker EP. Proton-motive-force-dependent step in the pathway to lysis of Escherichia coli induced by bacteriophage phi X174 gene E product. J Bacteriol 1987; 169:1750 - 1752
- Witte A, Wanner G, Blasi U, Halfmann G, Szostak M, Lubitz W. Endogenous transmembrane tunnel formation mediated by phiX174 lysis protein E. J Bacteriol 1990; 172:4109 - 4114
- Witte A, Wanner G, Sulzner M, Lubitz W. Dynamics of phiX174 protein E-mediated lysis of Escherichia coli. Arch Microbiol 1992; 157:381 - 388
- Bläsi U, Henrich B, Lubitz W. Lysis of Escherichia coli by cloned phiX174 gene E depends on its expression. J Gen Microbiol 1985; 131:1107 - 1114
- Lubitz W, Halfmann G, Plapp R. Lysis of Escherichia coli after infection with phiX174 depends on the regulation of the cellular autolytic system. J Gen Microbiol 1984; 130:1079 - 1087
- Witte A, Brand E, Mayrhofer P, Narendja F, Lubitz W. Mutations in cell division proteins FtsZ and FtsA inhibit phiX174 protein-E-mediated lysis of Escherichia coli. Arch Microbiol 1998; 170:259 - 268
- Witte A, Wanner G, Lubitz W, Holtje JV. Effect of phi X174 protein E-mediated lysis on murein composition of Escherichia coli. FEMS Microbiol Lett 1998; 164:149 - 157
- Bernhardt TG, Roof WD, Young R. Genetic evidence that the bacteriophage phi X174 lysis protein inhibits cell wall synthesis. Proc Natl Acad Sci USA 2000; 97:4297 - 4302
- Schön P, Schrot G, Wanner G, Lubitz W, Witte A. Two-stage model for integration of the lysis protein E of phiX174 into the cell envelope of Escherichia coli. FEMS Microbiol Rev 1995; 17:207 - 212
- Jalava K, Hensel A, Szostak M, Resch S, Lubitz W. Bacterial ghosts as vaccine candidates for veterinary applications. J Control Release 2002; 85:17 - 25
- Lubitz W, Witte A, Eko FO, Kamal M, Jechlinger W, Brand E, et al. Extended recombinant bacterial ghost system. J Biotechnol 1999; 73:261 - 273
- Halfmann G, Gotz F, Lubitz W. Expression of bacteriophage PhiX174 lysis gene E in Staphylococcus carnosus TM300. FEMS Microbiol Lett 1993; 108:139 - 143
- Jalava K, Eko FO, Riedmann E, Lubitz W. Bacterial ghosts as carrier and targeting systems for mucosal antigen delivery. Expert Rev Vaccines 2003; 2:45 - 51
- Lubitz P, Mayr UB, Lubitz W. Applications of bacterial ghosts in biomedicine 2009; Austin Landes Bioscience
- Riedmann EM, Kyd JM, Cripps AW, Lubitz W. Bacterial ghosts as adjuvant particles. Expert Rev Vaccines 2007; 6:241 - 253
- Szostak M, Lubitz W. Recombinant bacterial ghosts as multivaccine vehicles 1991; New York Cold Spring Harbor Laboratory Press
- Szostak M, Wanner G, Lubitz W. Recombinant bacterial ghosts as vaccines. Res Microbiol 1990; 141:1005 - 1007
- Ebensen T, Paukner S, Link C, Kudela P, de Domenico C, Lubitz W, et al. Bacterial ghosts are an efficient delivery system for DNA vaccines. J Immunol 2004; 172:6858 - 6865
- Mayr UB, Koller VJ, Lubitz P, Lubitz W. Sleator R, Hill C. Bacterial ghosts as vaccine and drug delivery platforms. Patho-Biotechnology 2008; Austin, TX Landes Bioscience 50 - 59
- Mayr UB, Walcher P, Azimpour C, Riedmann E, Haller C, Lubitz W. Bacterial ghosts as antigen delivery vehicles. Adv Drug Deliv Rev 2005; 57:1381 - 1391
- Paukner S, Stiedl T, Kudela P, Bizik J, Al Laham F, Lubitz W. Bacterial ghosts as a novel advanced targeting system for drug and DNA delivery. Expert Opin Drug Deliv 2006; 3:11 - 22
- Walcher P, Mayr UB, Azimpour-Tabrizi C, Eko FO, Jechlinger W, Mayrhofer P, et al. Antigen discovery and delivery of subunit vaccines by nonliving bacterial ghost vectors. Expert Rev Vaccines 2004; 3:681 - 691
- Jechlinger W, Glocker J, Haidinger W, Matis A, Szostak MP, Lubitz W. Modulation of gene expression by promoter mutants of the lambdacI857/pRM/pR system. J Biotechnol 2005; 116:11 - 20
- Jechlinger W, Szostak MP, Witte A, Lubitz W. Altered temperature induction sensitivity of the lambda pR/cI857 system for controlled gene E expression in Escherichia coli. FEMS Microbiol Lett 1999; 173:347 - 352
- Haidinger W, Szostak MP, Jechlinger W, Lubitz W. Online monitoring of Escherichia coli ghost production. Appl Environ Microbiol 2003; 69:468 - 474
- Haidinger W, Szostak MP, Beisker W, Lubitz W. Green fluorescent protein (GFP)-dependent separation of bacterial ghosts from intact cells by FACS. Cytometry 2001; 44:106 - 112
- Haidinger W, Mayr UB, Szostak MP, Resch S, Lubitz W. Escherichia coli ghost production by expression of lysis gene E and Staphylococcal nuclease. Appl Environ Microbiol 2003; 69:6106 - 6113
- Perrin P, Morgeaux S. Inactivation of DNA by beta-propiolactone. Biologicals 1995; 23:207 - 211
- Hensel A, van Leengoed LA, Szostak M, Windt H, Weissenbock H, Stockhofe-Zurwieden N, et al. Induction of protective immunity by aerosol or oral application of candidate vaccines in a dose-controlled pig aerosol infection model. J Biotechnol 1996; 44:171 - 181
- Hensel A, Stockhofe-Zurwieden N, Petzoldt K, Lubitz W. Oral immunization of pigs with viable or inactivated Actinobacillus pleuropneumoniae serotype 9 induces pulmonary and systemic antibodies and protects against homologous aerosol challenge. Infect Immun 1995; 63:3048 - 3053
- Huter V, Hensel A, Brand E, Lubitz W. Improved protection against lung colonization by Actinobacillus pleuropneumoniae ghosts: characterization of a genetically inactivated vaccine. J Biotechnol 2000; 83:161 - 172
- Hensel A, Huter V, Katinger A, Raza P, Strnistschie C, Roesler U, et al. Intramuscular immunization with genetically inactivated (ghosts) Actinobacillus pleuropneumoniae serotype 9 protects pigs against homologous aerosol challenge and prevents carrier state. Vaccine 2000; 18:2945 - 2955
- Marchart J, Dropmann G, Lechleitner S, Schlapp T, Wanner G, Szostak MP, et al. Pasteurella multocida and Pasteurella haemolytica-ghosts: new vaccine candidates. Vaccine 2003; 21:3988 - 3997
- Marchart J, Rehagen M, Dropmann G, Szostak MP, Alldinger S, Lechleitner S, et al. Protective immunity against pasteurellosis in cattle, induced by Pasteurella haemolytica ghosts. Vaccine 2003; 21:1415 - 1422
- Eko FO, Schukovskaya T, Lotzmanova EY, Firstova VV, Emalyanova NV, Klueva SN, et al. Evaluation of the protective efficacy of Vibrio cholerae ghost (VCG) candidate vaccines in rabbits. Vaccine 2003; 21:3663 - 3674
- Hobom G, Arnold N, Ruppert A. OmpA fusion proteins for presentation of foreign antigens on the bacterial outer membrane. Dev Biol Stand 1995; 84:255 - 262
- Huter V, Szostak MP, Gampfer J, Prethaler S, Wanner G, Gabor F, et al. Bacterial ghosts as drug carrier and targeting vehicles. J Control Release 1999; 61:51 - 63
- Kennedy EP. Osmotic regulation and the biosynthesis of membrane-derived oligosaccharides in Escherichia coli. Proc Natl Acad Sci USA 1982; 79:1092 - 1095
- Kuen B, Sara M, Lubitz W. Heterologous expression and self-assembly of the S-layer protein SbsA of Bacillus stearothermophilus in Escherichia coli. Mol Microbiol 1996; 19:495 - 503
- Truppe M, Howorka S, Schroll G, Lechleitner S, Kuen B, Resch S, et al. Biotechnological applications of recombinant S-layer proteins rSbsA and rSbsB from Bacillus stearothermophilus PV72. FEMS Microbiol Rev 1997; 20:88 - 98
- Howorka S, Sara M, Wang Y, Kuen B, Sleytr UB, Lubitz W, et al. Surface-accessible residues in the monomeric and assembled forms of a bacterial surface layer protein. J Biol Chem 2000; 275:37876 - 37886
- Riedmann EM, Kyd JM, Smith AM, Gomez-Gallego S, Jalava K, Cripps AW, et al. Construction of recombinant S-layer proteins (rSbsA) and their expression in bacterial ghosts—a delivery system for the nontypeable Haemophilus influenzae antigen Omp26. FEMS Immunol Med Microbiol 2003; 37:185 - 192
- Paukner S, Kohl G, Lubitz W. Bacterial ghosts as novel advanced drug delivery systems: antiproliferative activity of loaded doxorubicin in human Caco-2 cells. J Control Release 2004; 94:63 - 74
- Paukner S, Kohl G, Jalava K, Lubitz W. Sealed bacterial ghosts—novel targeting vehicles for advanced drug delivery of water-soluble substances. J Drug Target 2003; 11:151 - 161
- Hatfaludi T, Liska M, Zellinger D, Ousman JP, Szostak M, Ambrus A, et al. Bacterial ghost technology for pesticide delivery. J Agric Food Chem 2004; 52:5627 - 5634
- Gentschev I, Dietrich G, Spreng S, Kolb-Maurer A, Brinkmann V, Grode L, et al. Recombinant attenuated bacteria for the delivery of subunit vaccines. Vaccine 2001; 19:2621 - 2628
- Jager E, Karbach J, Gnjatic S, Neumann A, Bender A, Valmori D, et al. Recombinant vaccinia/fowipox NY-ESO-1 vaccines induce both humoral and cellular NY-ESO-1-specific immune responses in cancer patients. Proc Natl Acad Sci USA 2006; 103:14453 - 14458
- Medina E, Guzman CA. Use of live bacterial vaccine vectors for antigen delivery: potential and limitations. Vaccine 2001; 19:1573 - 1580
- Mengesha A, Dubois L, Chiu RK, Paesmans K, Wouters BG, Lambin P, et al. Potential and limitations of bacterial-mediated cancer therapy. Front Biosci 2007; 12:3880 - 3891
- Nishikawa H, Tsuji T, Jager E, Briones G, Ritter G, Old LJ, et al. Induction of regulatory T cell-resistant helper CD4+ T cells by bacterial vector. Blood 2008; 111:1404 - 1412
- Reschel T, Konak C, Oupicky D, Seymour LW, Ulbrich K. Physical properties and in vitro transfection efficiency of gene delivery vectors based on complexes of DNA with synthetic polycations. J Control Release 2002; 81:201 - 217
- Schnell S, Young JW, Houghton AN, Sadelain M. Retrovirally transduced mouse dendritic cells require CD4+ T cell help to elicit antitumor immunity: implications for the clinical use of dendritic cells. J Immunol 2000; 164:1243 - 1250
- Schumacher L, Ribas A, Dissette VB, McBride WH, Mukherji B, Economou JS, et al. Human dendritic cell maturation by adenovirus transduction enhances tumor antigen-specific T-cell responses. J Immunother 2004; 27:191 - 200
- Weiner DB. Introduction to DNA vaccines issue. Vaccine 2006; 24:4459 - 4460
- Liu MA, Wahren B, Karlsson Hedestam GB. DNA vaccines: recent developments and future possibilities. Hum Gene Ther 2006; 17:1051 - 1061
- Wiendl H, Hohlfeld R, Kieseier BC. Immunobiology of muscle: advances in understanding an immunological microenvironment. Trends Immunol 2005; 26:373 - 380
- Paukner S, Kudela P, Kohl G, Schlapp T, Friedrichs S, Lubitz W. DNA-loaded bacterial ghosts efficiently mediate reporter gene transfer and expression in macrophages. Mol Ther 2005; 11:215 - 223
- Lubitz W. Bacterial ghosts as carrier and targeting systems. Expert Opin Biol Ther 2001; 1:765 - 771
- Kudela P, Paukner S, Mayr UB, Cholujova D, Kohl G, Schwarczova Z, et al. Effective gene transfer to melanoma cells using bacterial ghosts. Cancer Lett 2007;
- Kudela P, Paukner S, Mayr UB, Cholujova D, Schwarczova Z, Sedlak J, et al. Bacterial ghosts as novel efficient targeting vehicles for DNA delivery to the human monocyte-derived dendritic cells. J Immunother 2005; 28:136 - 143
- Kudela P, Paukner S, Mayr UB, Cholujova D, Kohl G, Schwarczova Z, et al. Effective gene transfer to melanoma cells using bacterial ghosts. Cancer Lett 2008; 262:54 - 63
- Trombetta ES, Ebersold M, Garrett W, Pypaert M, Mellman I. Activation of lysosomal function during dendritic cell maturation. Science 2003; 299:1400 - 1403
- Trombetta ES, Mellman I. Cell biology of antigen processing in vitro and in vivo. Annu Rev Immunol 2005; 23:975 - 1028
- Mayrhofer P, Tabrizi CA, Walcher P, Haidinger W, Jechlinger W, Lubitz W. Immobilization of plasmid DNA in bacterial ghosts. J Control Release 2005; 102:725 - 735
- Jechlinger W, Azimpour Tabrizi C, Lubitz W, Mayrhofer P. Minicircle DNA immobilized in bacterial ghosts: in vivo production of safe non-viral DNA delivery vehicles. J Mol Microbiol Biotechnol 2004; 8:222 - 231
- Chen ZY, He CY, Kay MA. Improved production and purification of minicircle DNA vector free of plasmid bacterial sequences and capable of persistent transgene expression in vivo. Hum Gene Ther 2005; 16:126 - 131
- Wolff JA, Budker V. The mechanism of naked DNA uptake and expression. Adv Genet 2005; 54:3 - 20
- Wolff JA, Ludtke JJ, Acsadi G, Williams P, Jani A. Long-term persistence of plasmid DNA and foreign gene expression in mouse muscle. Hum Mol Genet 1992; 1:363 - 369
- Yu M, Finn OJ. DNA vaccines for cancer too. Cancer Immunol Immunother 2006; 55:119 - 130
- Brady MS, Lee F, Petrie H, Eckels DD, Lee JS. CD4(+) T cells kill HLA-class-II-antigen-positive melanoma cells presenting peptide in vitro. Cancer Immunol Immunother 2000; 48:621 - 626
- Curiel-Lewandrowski C, Demierre MF. Advances in specific immunotherapy of malignant melanoma. J Am Acad Dermatol 2000; 43:167 - 185
- Lugini L, Lozupone F, Matarrese P, Funaro C, Luciani F, Malorni W, et al. Potent phagocytic activity discriminates metastatic and primary human malignant melanomas: a key role of ezrin. Lab Invest 2003; 83:1555 - 1567
- Lugini L, Matarrese P, Tinari A, Lozupone F, Federici C, Iessi E, et al. Cannibalism of live lymphocytes by human metastatic but not primary melanoma cells. Cancer Res 2006; 66:3629 - 3638
- Buckley KJ, Hayashi M. Lytic activity localized to membrane-spanning region of phi X174 E protein. Mol Gen Genet 1986; 204:120 - 125
- Maratea D, Young K, Young R. Deletion and fusion analysis of the phage phiX174 lysis gene E. Gene 1985; 40:39 - 46
- Pfründer H, Amidjojo M, Kragl U, Weuster-Botz D. Efficient whole-cell biotransformation in a biphasic ionic liquid/water system. Angew Chem Int Ed 2004; 43:4529 - 4531
- Pfründer H, Jones R, Weuster-Botz D. Water immiscible ionic liquids as solvents for whole cell biocatalysis. J Biotechnol 2006; 124:182 - 190
- Yang Z, Pan W. Ionic liquids: Green solvents for nonaqueous biocatalysis. Enzyme and Microbial Technology 2005; 37:19 - 28
- Kaftzik N, Wasserscheid P, Kragl U. Use of ionic liquids to increase the yield and enzyme stability in the β-galactosidase catalysed cynthesis of n-acetyllactosamine. Org Process Res Dev 2002; 6:553 - 557