Abstract
Therapeutic properties of recombinant proteins are very often affected by the composition and heterogeneity of their glycans. Conventional expression systems for recombinant pharmaceutical proteins typically do not address this problem and produce a mixture of glycoforms that are neither identical to human glycans nor optimized for enhanced efficacy. In terms of glycosylation, plants offer certain advantages over mammalian cells as the N-glycosylation pathway of plants is comparably simple and a typical mammalian O-glycosylation pathway is not present at all. During the last ten years we have developed a plant-based expression platform for the generation of recombinant glycoproteins with defined N-glycans. Now we have extended our tool-box for glyco-engineering in the tobacco related species Nicotiana benthamiana toward the production of tailored mucin-type O-glycans on recombinant proteins.
Introduction
In recent years plants have emerged as interesting new hosts for the production of pharmaceutical proteins as they provide several advantages compared with conventional mammalian cell-based expression systems. Cultivation of plants is comparably simple and inexpensive. Plants offer virtually unrestricted scalability and plants are considered safe as they lack human pathogens. In addition, major advances in expression technologies, downstream processing and the implementation of GMP manufacturingCitation1 make plants attractive alternatives for the production of recombinant biopharmaceuticals. This development is also reflected by the recent marketing approval of a plant-produced recombinant α-glucocerebrosidase for enzyme-replacement therapy in humans.Citation2 Within the different plant-based expression systems transient production using viral-based vectors is very promising as it is very flexible and allows high-yield protein production within a few days.Citation3,Citation4 This approach is, for example, optimal suited for the production of recombinant therapeutics to treat infectious diseases. A monoclonal antibody against Ebola virus has recently been produced by transient expression in N. benthamiana.Citation5
Another advantage of plants is their ability to assemble proteins and perform posttranslational modifications similar to mammalian cells. Glycosylation is the most important posttranslational modification of recombinant biopharmaceuticals and many different classes of recombinant drugs like monoclonal antibodies, growth factors or hormones are glycosylated. In mammalian cells two major types of protein glycosylation exist: N-glycosylation, which is initiated by transfer of a preassembled oligosaccharide to an asparagine residue present in unfolded or partially folded polypeptides and O-glycosylation, which involves the transfer of a single sugar residue to a serine or threonine of a fully folded protein. Both types of glycosylation are subjected to extensive processing reactions resulting in the generation of a large variety of different glycans on proteins. It has been clearly demonstrated that certain glycoforms drastically affect the efficacy of recombinant proteins. Yet, the conventional expression hosts like CHO cells produce only sub-optimal glycan structures and frequently heterogeneity of N- and O-glycans is observed on recombinant therapeutics. For example, the presence of a core α1,6-fucose residue on the N-glycan of the IgG heavy chain affects the binding to the Fc receptors and subsequently alters the effector functions of IgG molecules.Citation6 In addition, there are raising concerns regarding the presence of non-human glycosylation like the incorporation of N-glycolylneuraminic (Neu5Gc) into glycosylated biopharmaceuticals produced in some mammalian cell lines.Citation7
In contrast to N-glycosylation, the biological function of distinct O-glycan structures on recombinant glycoproteins is still poorly understood. Given the fact that certain biopharmaceutical proteins, like for example IgAs or erythropoietin (EPO), are O-glycosylated, a production platform for tailored O-glycans might not only improve product quality by reducing glycan heterogeneity but could also enhance the biological activities of recombinant therapeutics. Similar to mammalian N-glycosylation, O-glycan initiation and processing is rather a complex process. In humans, for example, initiation of mucin-type O-glycosylation can be performed by up to 20 different UDP-GalNAc:polypeptide N-acetylgalactosaminyltransferases (GalNAc-Ts) which catalyze the transfer of a single N-acetylgalactosamine (GalNAc) residue to serine/threonine amino acids (GalNAcα-Ser/Thr).Citation8 These GalNAc-Ts have only partially overlapping substrate specificities and their regulation as well as their precise biological role in mammals is still not entirely clear.Citation9 Further elongation of GalNAc residues is performed by Golgi-located glycosyltransferases and results in different O-glycan structures including core 1 to core 4 structures. The uncontrolled expression of these endogenous glycosyltransferases in mammalian cell-based production hosts prevents the generation of defined structures leading to the formation of rather heterogeneous O-glycans on recombinant proteins.Citation10,Citation11 Consequently, there is a demand for novel production systems or engineered conventional expression hosts that result in the production of tailored O-glycans on recombinant proteins.
N-Glycan Engineering in Plants
Recombinant glycoproteins typically contain an N-terminal signal peptide for targeting to the secretory pathway. Upon emergence of the polypeptide in the ER-lumen the oligosaccharyltransferase complex scans the amino acid sequence for the presence of N-glycosylation sites (Asn-X-Ser/Thr) and transfers the preassembled Glc3Man9GlcNAc2 oligosaccharide to an asparagine residue within the consensus site. In the ER the terminal glucose residues are removed and the trimming of distinct mannose residues is initiated by class I α-mannosidases (MNS proteins).Citation12-Citation15 These N-glycan processing events are linked to ER-quality control processes like the calnexin/calreticulin cycle and the ER-associated degradation (ERAD) pathway. Additional mannose residues are then removed by Golgi-located MNS1/MNS2 and the Man5GlcNAc2 structure () is processed into complex N-glycans by the action of N-acetylglucosaminyltransferase I (GnTI), Golgi-α-mannosidase II (GMII) and N-acetylglucosaminyltransferase II (GnTII). Further modification of plant complex N-glycans is typically performed by β1,2-xylosyltransferase and core α1,3-fucosyltransferase, which add β1,2-xylose and core α1,3-fucose, respectively. These two glycosyltransferases are not present in mammals and the administration of glycoprotein therapeutics with xylosylated and fucosylated N-glycans might therefore lead to adverse immunogenic reactions in mammals.Citation16 As a consequence the N-glycosylation pathway of several plant species has been engineered in a way to eliminate the expression of the corresponding non-mammalian glycosyltransferases.Citation17-Citation21 The glyco-engineered N. benthamiana plants known as ΔXF plants produce predominately GlcNAc2Man3GlcNAc2 structures on recombinant proteins.Citation20 In subsequent studies this complex N-glycan has been processed by the incorporation of β1,4-linked galactose,Citation22 terminal α2,6-linked sialic acid,Citation23 core α1,6-linked fucoseCitation24 and the formation of tri- and tetra-antennary glycans.Citation25,Citation26 All these common mammalian complex N-glycan modifications are not found in plants and have to be engineered to make plants optimal hosts for the production of recombinant glycoprotein therapeutics.
Figure 1. Schematic presentation of the engineered O- and N-glycosylation pathways in N. benthamiana ΔXF plants. Only the Golgi processing steps are shown. Heterologous expressed proteins for the generation of disialylated O-glycans are shown in red (GNE: UDP-N-acetylglucosamine 2-epimerase/N-acetylmannosamine kinase; NANS: N-acetylneuraminic acid phosphate synthase; CMAS: CMP-N-acetylneuraminic acid synthetase; CST: CMP-Neu5Ac transporter). Additional expression of two mammalian glycosyltransferases (GalT: β1,4-galactosyltransferase; ST: α2,6-sialyltransferase) is required for complex N-glycan modification and expression of mammalian N-acetylglucosaminyltransferase IV (GnTIV) and N-acetylglucosaminyltransferase V (GnTV) is required for the generation of multi-antennary complex N-glycans (these mammalian enzymes are depicted in blue). Endogenous plant proteins involved in early N-glycan processing reactions (MNS1/2: Golgi-α-mannosidase I; GnTI: N-acetylglucosaminyltransferase I; GMII: Golgi-α-mannosidase II; GnTII: N-acetylglucosaminyltransferase II) or conversion and transport of nucleotide sugars for O- and N-glycan formation are shown in black (NANP: Neu5Ac-9-phosphate phosphatase).
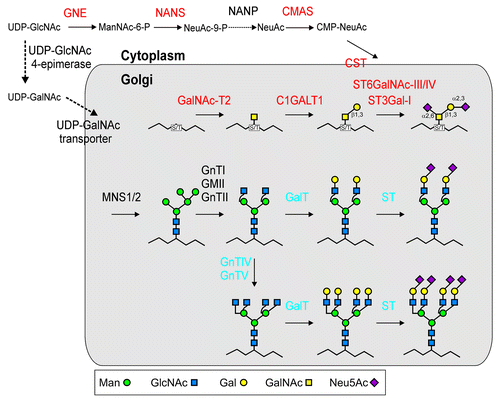
O-glycan Engineering in Plants
While the early steps of the N-glycan processing pathway are highly conserved in mammals and plants the O-glycosylation capacity is completely different. O-glycosylation in plants is frequently found on hydroxyl groups of hydroxyproline (Hyp) residues and only to a lesser extent on other amino acids like serines.Citation27 The hydroxyl groups of the amino acids can be modified by addition of a large arabinogalactan polysaccharide, a short arabinose chain, or a single galactose residue, but GalNAc is typically not present. Accordingly, mucin-type O-glycosylation has not been found on plant-produced recombinant proteins.Citation28-Citation31 Plants are therefore very well suited for de novo engineering of the mammalian O-glycosylation pathway.
In the first attempt to initiate O-GalNAc formation in plants a Yersinia enterocolitica UDP-GlcNAc 4-epimerase and a Caenorhabditis elegans UDP-GlcNAc/UDP-GalNAc transporter were co-expressed with human GalNAc-T2 in N. benthamiana.Citation32 Mass spectrometry revealed the attachment of a single GalNAc residue to an endogenous plant protein and lectin blotting indicated the incorporation of GalNAc residues into a co-expressed recombinant protein containing a mucin-type sequence motif with several serine/threonine residues. In a similar study, it was shown by structural analysis that transient expression of Pseudomonas aeruginosa GlcNAc C4-epimerase and human GalNAc-T2 in N. benthamiana are sufficient for O-GalNAc formation on a polypeptide containing a mucin-type tandem repeat.Citation30 This strategy was also applied to generate transgenic Arabidopsis thaliana plants and tobacco BY2 cells with the capacity for the production of O-linked GalNAc on recombinant proteins.Citation33 Very recently, we demonstrated that GalNAc can be attached to the single O-glycosylation site present in human EPO. In our effort, we transiently expressed EPO fused to the Fc domain of a human IgG heavy chain (EPO-Fc) together with the proteins for glyco-engineering in the ΔXF line.Citation31 In contrast to previous studies, we found that the first O-glycosylation reaction requires only the expression of human GalNAc-T2. The reason for the discrepancy between the studies is not clear, but might be explained by differences in the expression levels of the recombinant proteins.Citation34 In summary, these studies demonstrate that plants can be engineered to produce recombinant proteins with mucin-type O-linked GalNAc.
An important further elongation of O-GalNAc is the incorporation of galactose in β1,3-linkage. This biosynthesis step is catalyzed by β1,3-galactosyltransferase (C1GALT1 or T-synthase) and results in the formation of the Galβ1–3GalNAcα-Ser/Thr core 1 structure (also known as T antigen) (). In humans, there is a single C1GALT1 enzyme that generates this commonly found mucin-type O-glycan. Importantly, proper formation of the T antigen in mammals requires the presence of the chaperone COSMC, which prevents aggregation and subsequent degradation of C1GALT1 in the ER.Citation35 In N. benthamiana co-expression of COSMC with human C1GALT1 did not result in the incorporation of significant amounts of galactose suggesting that human COSMC is either not functional in plants or not correctly localized. By contrast, transient expression of the Drosophila C1GALT1 along with human GalNAc-T2 in N. benthamiana resulted in the formation of core 1 structures on EPO-Fc.Citation31
A common terminal modification of mucin-type O-linked glycans is the attachment of sialic acid (N-acetylneuraminic acid: Neu5Ac). As plants do not contain this sialic acid the whole biosynthetic pathway for conversion of the precursor UDP-GlcNAc to CMP-sialic acid and its transport into the Golgi has to be transferred into plants ().Citation23,Citation36 Golgi-resident sialyltransferases transfer sialic acid from CMP-sialic acid to protein-linked glycans. On urinary and recombinant EPO the most frequent core 1 extension is the incorporation of two sialic acid residues resulting in the formation of disialyl-core 1 structures. The generation of this O-linked glycan on plant-derived EPO-Fc was achieved by co-expression of the two mammalian sialyltransferases ST3Gal-I and ST6GalNAc-III/IV with human GalNAc-T2 and Drosophila C1GALT1.Citation31 In total, the implementation of this O-glycan modification required the coordinated expression of eight non-plant proteins in N. benthamiana. All of them were transiently expressed by infiltration of leaves with Agrobacterium tumefaciens containing the individual expression constructs.
Pharmaceutical proteins like EPO or IgAs contain both types of glycosylation. It is therefore essential to develop a plant-based production platform for generation of tailored O- and N-glycans. For EPO the terminal sialic acid residues present on N-linked glycans are of utmost importance for its in vivo function.Citation37 In N. benthamiana, the additional expression of human β1,4-galactosyltransferase (GalT) and rat α2,6-sialyltransferase (ST) resulted in successful manipulation of both glycosylation pathways and the formation of sialylated N- and O-glycans.Citation31 Importantly, our experimental data showed that the different mammalian glycosyltransferases for attachment of sugars to N- or O-glycans do not cross-react indicating that they retain their specificity when heterologously expressed in plants.
Future of O-glycan Engineering in Plants
Due to the absence of naturally occurring mucin-type O-glycosylation plants are very well suited for the generation of highly homogenous O-glycan structures. However, despite all the progress there are still some limitations. One obstacle that has to be overcome in plants is the formation of Hyp residues in proximity to exposed O-glycosylation sites on recombinant mammalian proteins. These hydroxylated proline residues have been detected on stable and transiently expressed mucin-derived peptidesCitation30,Citation33 and close to Ser-126 on EPO-Fc.Citation31 The Hyp residues serve as starting points for the attachment of plant-specific O-glycosylation and subsequently increase the risk of unwanted side effects like immunogenic or allergenic reactions when present on recombinant biopharmaceuticals.Citation29,Citation38 Different strategies have been proposed to prevent the formation of plant-specific Hyp glycosylation.Citation33,Citation39 In particular, knockout or knockdown of the corresponding prolyl-4 hydroxylase(s) holds great promise to achieve a robust system for expression of different types of O-glycosylated proteins without plant specific Hyp-glycosylation.
The plant-produced O-glycan structures are still not homogenous and complete conversion of all transferred GalNAc-residues to disialylated core 1 structures has not been achieved yet. Apart from appropriate expression and localization of the heterologous proteins efficient glycosylation depends on the availability of sufficient amounts of nucleotide sugars. One strategy for improvement is therefore a better control of nucleotide sugar levels. In particular, an increase in CMP-sialic acid should be beneficial for optimization of the sialylation capacity of engineered plants. CMP-sialic acid originates from endogenous UDP-GlcNAc and in mammalian cells its formation is tightly controlled and subjected to feedback regulation.Citation40 Feedback inhibition can be prevented by expression of a mutated version of UDP-N-acetylglucosamine 2-epimerase/N-acetylmannosamine kinase (GNE), the key enzyme for CMP-sialic acid formation.Citation41,Citation42 Apart from metabolic engineering of the nucleotide sugar levels it would also be highly desired to develop tools for controlling the Golgi transport and steady-state levels of the nucleotide sugars in the Golgi.
For the generation of sialylated N- and O-glycans we have simultaneously expressed ten individual constructs and the recombinant glycoprotein by infiltration of a single Agrobacterium mixture into N. benthamiana leaves.Citation31 While a further increase of constructs in the infiltration mixture is still possible, e.g., required for generation of branched sialylated N-glycans,Citation25,Citation26,Citation43 it would be very convenient to combine several of the required proteins and express them from multi-cassette expression vectors.Citation44,Citation45 For example, the three enzymes for for CMP-sialic acid biosynthesis and the corresponding Golgi transporter could all be produced from a single construct leading to a more consistent supply with the donor substrate and as a consequence to increased amounts of fully sialylated glycoproteins.
Future developments will also concentrate on O-glycan engineering steps that have not been performed so far, like for example, the formation of core 2, 3 and 4 structures. Apart from incorporation of galactose, GalNAc and sialic acid these O-glycans can contain GlcNAc and fucose in different linkages.Citation9,Citation46 The glycosyltransferases that transfer these sugars are typically not present in plants and have to be expressed similar to the glycosyltransferases for core 1 structure formation and capping with sialic acid.Citation31 Since the biosynthesis of mucin-type O-glycans occurs in a step-wise manner it is crucial to maintain the appropriate order of modification steps when performed in plants. The sub-Golgi localization of glycosyltransferases in the cis, medial and trans-Golgi plays a central role in the control of glycosylation.Citation47 Golgi-targeting signals of mammalian glycosyltransferases are functional in plants.Citation48-Citation50 However, subtle differences in sub-Golgi targeting have been observed for some mammalian glycosyltransferases resulting in altered glycan profiles on plant-produced recombinant proteins.Citation22,Citation25,Citation26,Citation43,Citation51,Citation52 Thus, fine-tuning of Golgi localization will be another target for future improvements in glyco-engineering. To this end, we need more efforts to increase our knowledge of Golgi organization in plants and we need a better understanding of the mechanisms and signals involved in sub-Golgi targeting of glycosyltransferases.
Recent major advances in glyco-engineering have demonstrated that plants can be engineered toward production of recombinant glycoproteins with fully humanized glycosylation. A variety of tools are now available to produce glycoproteins with desired N- and O-glycans in plants. The future will show whether these developments can be translated into industrial applications for the production of next-generation biopharmaceuticals.
Acknowledgments
Related work in my group is supported by a grant from the Federal Ministry of Transport, Innovation and Technology (bmvit) and Austrian Science Fund (FWF): TRP 242-B20.
References
- Fischer R, Schillberg S, Hellwig S, Twyman RM, Drossard J. GMP issues for recombinant plant-derived pharmaceutical proteins. Biotechnol Adv 2012; 30:434 - 9; http://dx.doi.org/10.1016/j.biotechadv.2011.08.007; PMID: 21856403
- Maxmen A. Drug-making plant blooms. Nature 2012; 485:160; http://dx.doi.org/10.1038/485160a; PMID: 22575938
- Bendandi M, Marillonnet S, Kandzia R, Thieme F, Nickstadt A, Herz S, et al. Rapid, high-yield production in plants of individualized idiotype vaccines for non-Hodgkin’s lymphoma. Ann Oncol 2010; 21:2420 - 7; http://dx.doi.org/10.1093/annonc/mdq256; PMID: 20494963
- Sainsbury F, Sack M, Stadlmann J, Quendler H, Fischer R, Lomonossoff GP. Rapid transient production in plants by replicating and non-replicating vectors yields high quality functional anti-HIV antibody. PLoS One 2010; 5:e13976; http://dx.doi.org/10.1371/journal.pone.0013976; PMID: 21103044
- Castilho A, Bohorova N, Grass J, Bohorov O, Zeitlin L, Whaley K, et al. Rapid high yield production of different glycoforms of Ebola virus monoclonal antibody. PLoS One 2011; 6:e26040; http://dx.doi.org/10.1371/journal.pone.0026040; PMID: 22039433
- Shields RL, Lai J, Keck R, O’Connell LY, Hong K, Meng YG, et al. Lack of fucose on human IgG1 N-linked oligosaccharide improves binding to human Fcgamma RIII and antibody-dependent cellular toxicity. J Biol Chem 2002; 277:26733 - 40; http://dx.doi.org/10.1074/jbc.M202069200; PMID: 11986321
- Ghaderi D, Zhang M, Hurtado-Ziola N, Varki A. Production platforms for biotherapeutic glycoproteins. Occurrence, impact, and challenges of non-human sialylation. Biotechnol Genet Eng Rev 2012; 28:147 - 75; http://dx.doi.org/10.5661/bger-28-147; PMID: 22616486
- Bennett EP, Mandel U, Clausen H, Gerken TA, Fritz TA, Tabak LA. Control of mucin-type O-glycosylation: a classification of the polypeptide GalNAc-transferase gene family. Glycobiology 2012; 22:736 - 56; http://dx.doi.org/10.1093/glycob/cwr182; PMID: 22183981
- Schjoldager KT, Clausen H. Site-specific protein O-glycosylation modulates proprotein processing - Deciphering specific functions of the large polypeptide GalNAc-transferase gene family. Biochim Biophys Acta 2012; 1820:2079-94..
- Schiestl M, Stangler T, Torella C, Cepeljnik T, Toll H, Grau R. Acceptable changes in quality attributes of glycosylated biopharmaceuticals. Nat Biotechnol 2011; 29:310 - 2; http://dx.doi.org/10.1038/nbt.1839; PMID: 21478841
- Taschwer M, Hackl M, Hernández Bort JA, Leitner C, Kumar N, Puc U, et al. Growth, productivity and protein glycosylation in a CHO EpoFc producer cell line adapted to glutamine-free growth. J Biotechnol 2012; 157:295 - 303; http://dx.doi.org/10.1016/j.jbiotec.2011.11.014; PMID: 22178781
- Boisson M, Gomord V, Audran C, Berger N, Dubreucq B, Granier F, et al. Arabidopsis glucosidase I mutants reveal a critical role of N-glycan trimming in seed development. EMBO J 2001; 20:1010 - 9; http://dx.doi.org/10.1093/emboj/20.5.1010; PMID: 11230125
- Gillmor CS, Poindexter P, Lorieau J, Palcic MM, Somerville C. Alpha-glucosidase I is required for cellulose biosynthesis and morphogenesis in Arabidopsis. J Cell Biol 2002; 156:1003 - 13; http://dx.doi.org/10.1083/jcb.200111093; PMID: 11901167
- Soussilane P, D’Alessio C, Paccalet T, Fitchette AC, Parodi AJ, Williamson R, et al. N-glycan trimming by glucosidase II is essential for Arabidopsis development. Glycoconj J 2009; 26:597 - 607; http://dx.doi.org/10.1007/s10719-008-9201-1; PMID: 18972207
- Liebminger E, Hüttner S, Vavra U, Fischl R, Schoberer J, Grass J, et al. Class I alpha-mannosidases are required for N-glycan processing and root development in Arabidopsis thaliana.. Plant Cell 2009; 21:3850 - 67; http://dx.doi.org/10.1105/tpc.109.072363; PMID: 20023195
- Altmann F. The role of protein glycosylation in allergy. Int Arch Allergy Immunol 2007; 142:99 - 115; http://dx.doi.org/10.1159/000096114; PMID: 17033195
- Strasser R, Altmann F, Mach L, Glössl J, Steinkellner H. Generation of Arabidopsis thaliana plants with complex N-glycans lacking beta1,2-linked xylose and core alpha1,3-linked fucose. FEBS Lett 2004; 561:132 - 6; http://dx.doi.org/10.1016/S0014-5793(04)00150-4; PMID: 15013764
- Koprivova A, Stemmer C, Altmann F, Hoffmann A, Kopriva S, Gorr G, et al. Targeted knockouts of Physcomitrella lacking plant-specific immunogenic N-glycans. Plant Biotechnol J 2004; 2:517 - 23; http://dx.doi.org/10.1111/j.1467-7652.2004.00100.x; PMID: 17147624
- Cox KM, Sterling JD, Regan JT, Gasdaska JR, Frantz KK, Peele CG, et al. Glycan optimization of a human monoclonal antibody in the aquatic plant Lemna minor.. Nat Biotechnol 2006; 24:1591 - 7; http://dx.doi.org/10.1038/nbt1260; PMID: 17128273
- Strasser R, Stadlmann J, Schähs M, Stiegler G, Quendler H, Mach L, et al. Generation of glyco-engineered Nicotiana benthamiana for the production of monoclonal antibodies with a homogeneous human-like N-glycan structure. Plant Biotechnol J 2008; 6:392 - 402; http://dx.doi.org/10.1111/j.1467-7652.2008.00330.x; PMID: 18346095
- Shin YJ, Chong YJ, Yang MS, Kwon TH. Production of recombinant human granulocyte macrophage-colony stimulating factor in rice cell suspension culture with a human-like N-glycan structure. Plant Biotechnol J 2011; 9:1109 - 19; http://dx.doi.org/10.1111/j.1467-7652.2011.00636.x; PMID: 21801300
- Strasser R, Castilho A, Stadlmann J, Kunert R, Quendler H, Gattinger P, et al. Improved virus neutralization by plant-produced anti-HIV antibodies with a homogeneous β1,4-galactosylated N-glycan profile. J Biol Chem 2009; 284:20479 - 85; http://dx.doi.org/10.1074/jbc.M109.014126; PMID: 19478090
- Castilho A, Strasser R, Stadlmann J, Grass J, Jez J, Gattinger P, et al. In planta protein sialylation through overexpression of the respective mammalian pathway. J Biol Chem 2010; 285:15923 - 30; http://dx.doi.org/10.1074/jbc.M109.088401; PMID: 20305285
- Forthal DN, Gach JS, Landucci G, Jez J, Strasser R, Kunert R, et al. Fc-glycosylation influences Fcγ receptor binding and cell-mediated anti-HIV activity of monoclonal antibody 2G12. J Immunol 2010; 185:6876 - 82; http://dx.doi.org/10.4049/jimmunol.1002600; PMID: 21041724
- Castilho A, Gattinger P, Grass J, Jez J, Pabst M, Altmann F, et al. N-glycosylation engineering of plants for the biosynthesis of glycoproteins with bisected and branched complex N-glycans. Glycobiology 2011; 21:813 - 23; http://dx.doi.org/10.1093/glycob/cwr009; PMID: 21317243
- Nagels B, Van Damme EJ, Pabst M, Callewaert N, Weterings K. Production of complex multiantennary N-glycans in Nicotiana benthamiana plants. Plant Physiol 2011; 155:1103 - 12; http://dx.doi.org/10.1104/pp.110.168773; PMID: 21233332
- Velasquez M, Salter JS, Dorosz JG, Petersen BL, Estevez JM. Recent advances on the posttranslational modifications of EXTs and their roles in plant cell walls. Front Plant Sci 2012; 3:93; http://dx.doi.org/10.3389/fpls.2012.00093; PMID: 22639676
- Karnoup AS, Turkelson V, Anderson WH. O-linked glycosylation in maize-expressed human IgA1. Glycobiology 2005; 15:965 - 81; http://dx.doi.org/10.1093/glycob/cwi077; PMID: 15901675
- Pinkhasov J, Alvarez ML, Rigano MM, Piensook K, Larios D, Pabst M, et al. Recombinant plant-expressed tumour-associated MUC1 peptide is immunogenic and capable of breaking tolerance in MUC1.Tg mice. Plant Biotechnol J 2011; 9:991 - 1001; http://dx.doi.org/10.1111/j.1467-7652.2011.00614.x; PMID: 21740504
- Yang Z, Drew DP, Jørgensen B, Mandel U, Bach SS, Ulvskov P, et al. Engineering mammalian mucin-type O-glycosylation in plants. J Biol Chem 2012; 287:11911 - 23; http://dx.doi.org/10.1074/jbc.M111.312918; PMID: 22334671
- Castilho A, Neumann L, Daskalova S, Mason HS, Steinkellner H, Altmann F, et al. Engineering of sialylated mucin-type O-glycosylation in plants. J Biol Chem 2012; 287:36518 - 26; http://dx.doi.org/10.1074/jbc.M112.402685; PMID: 22948156
- Daskalova SM, Radder JE, Cichacz ZA, Olsen SH, Tsaprailis G, Mason H, et al. Engineering of N. benthamiana L. plants for production of N-acetylgalactosamine-glycosylated proteins--towards development of a plant-based platform for production of protein therapeutics with mucin type O-glycosylation. BMC Biotechnol 2010; 10:62; http://dx.doi.org/10.1186/1472-6750-10-62; PMID: 20735851
- Yang Z, Bennett EP, Jørgensen B, Drew DP, Arigi E, Mandel U, et al. Toward stable genetic engineering of human O-glycosylation in plants. Plant Physiol 2012; 160:450 - 63; http://dx.doi.org/10.1104/pp.112.198200; PMID: 22791304
- Strasser R. Challenges in O-glycan engineering of plants. Front Plant Sci 2012; 3:218; http://dx.doi.org/10.3389/fpls.2012.00218; PMID: 23015807
- Ju T, Aryal RP, Stowell CJ, Cummings RD. Regulation of protein O-glycosylation by the endoplasmic reticulum-localized molecular chaperone Cosmc. J Cell Biol 2008; 182:531 - 42; http://dx.doi.org/10.1083/jcb.200711151; PMID: 18695044
- Castilho A, Pabst M, Leonard R, Veit C, Altmann F, Mach L, et al. Construction of a functional CMP-sialic acid biosynthesis pathway in Arabidopsis. Plant Physiol 2008; 147:331 - 9; http://dx.doi.org/10.1104/pp.108.117572; PMID: 18326787
- Elliott S, Egrie J, Browne J, Lorenzini T, Busse L, Rogers N, et al. Control of rHuEPO biological activity: the role of carbohydrate. Exp Hematol 2004; 32:1146 - 55; http://dx.doi.org/10.1016/j.exphem.2004.08.004; PMID: 15588939
- Leonard R, Petersen BO, Himly M, Kaar W, Wopfner N, Kolarich D, et al. Two novel types of O-glycans on the mugwort pollen allergen Art v 1 and their role in antibody binding. J Biol Chem 2005; 280:7932 - 40; http://dx.doi.org/10.1074/jbc.M410407200; PMID: 15591314
- Moriguchi R, Matsuoka C, Suyama A, Matsuoka K. Reduction of plant-specific arabinogalactan-type O-glycosylation by treating tobacco plants with ferrous chelator 2,2′-dipyridyl. Biosci Biotechnol Biochem 2011; 75:994 - 6; http://dx.doi.org/10.1271/bbb.100884; PMID: 21597170
- Kornfeld S, Kornfeld R, Neufeld EF, O’Brien PJ. The feedback control of sugar nucleotide biosynthesis in liver. Proc Natl Acad Sci U S A 1964; 52:371 - 9; http://dx.doi.org/10.1073/pnas.52.2.371; PMID: 14206604
- Viswanathan K, Narang S, Hinderlich S, Lee YC, Betenbaugh MJ. Engineering intracellular CMP-sialic acid metabolism into insect cells and methods to enhance its generation. Biochemistry 2005; 44:7526 - 34; http://dx.doi.org/10.1021/bi047477y; PMID: 15895995
- Bork K, Reutter W, Weidemann W, Horstkorte R. Enhanced sialylation of EPO by overexpression of UDP-GlcNAc 2-epimerase/ManAc kinase containing a sialuria mutation in CHO cells. FEBS Lett 2007; 581:4195 - 8; http://dx.doi.org/10.1016/j.febslet.2007.07.060; PMID: 17706199
- Nagels B, Van Damme EJ, Callewaert N, Zabeau L, Tavernier J, Delanghe JR, et al. Biologically active, magnICON®-expressed EPO-Fc from stably transformed Nicotiana benthamiana plants presenting tetra-antennary N-glycan structures. J Biotechnol 2012; 160:242 - 50; http://dx.doi.org/10.1016/j.jbiotec.2012.03.003; PMID: 22430811
- Engler C, Gruetzner R, Kandzia R, Marillonnet S. Golden gate shuffling: a one-pot DNA shuffling method based on type IIs restriction enzymes. PLoS One 2009; 4:e5553; http://dx.doi.org/10.1371/journal.pone.0005553; PMID: 19436741
- Sarrion-Perdigones A, Falconi EE, Zandalinas SI, Juárez P, Fernández-del-Carmen A, Granell A, et al. GoldenBraid: an iterative cloning system for standardized assembly of reusable genetic modules. PLoS One 2011; 6:e21622; http://dx.doi.org/10.1371/journal.pone.0021622; PMID: 21750718
- Ju T, Otto VI, Cummings RD. The Tn antigen-structural simplicity and biological complexity. Angew Chem Int Ed Engl 2011; 50:1770 - 91; http://dx.doi.org/10.1002/anie.201002313; PMID: 21259410
- Stanley P. Golgi glycosylation. Cold Spring Harb Perspect Biol 2011; 3:3; http://dx.doi.org/10.1101/cshperspect.a005199; PMID: 21441588
- Boevink P, Oparka K, Santa Cruz S, Martin B, Betteridge A, Hawes C. Stacks on tracks: the plant Golgi apparatus traffics on an actin/ER network. Plant J 1998; 15:441 - 7; http://dx.doi.org/10.1046/j.1365-313X.1998.00208.x; PMID: 9750355
- Palacpac NQ, Yoshida S, Sakai H, Kimura Y, Fujiyama K, Yoshida T, et al. Stable expression of human beta1,4-galactosyltransferase in plant cells modifies N-linked glycosylation patterns. Proc Natl Acad Sci U S A 1999; 96:4692 - 7; http://dx.doi.org/10.1073/pnas.96.8.4692; PMID: 10200324
- Bakker H, Bardor M, Molthoff JW, Gomord V, Elbers I, Stevens LH, et al. Galactose-extended glycans of antibodies produced by transgenic plants. Proc Natl Acad Sci U S A 2001; 98:2899 - 904; http://dx.doi.org/10.1073/pnas.031419998; PMID: 11226338
- Bakker H, Rouwendal GJ, Karnoup AS, Florack DE, Stoopen GM, Helsper JP, et al. An antibody produced in tobacco expressing a hybrid beta-1,4-galactosyltransferase is essentially devoid of plant carbohydrate epitopes. Proc Natl Acad Sci U S A 2006; 103:7577 - 82; http://dx.doi.org/10.1073/pnas.0600879103; PMID: 16675551
- Vézina LP, Faye L, Lerouge P, D’Aoust MA, Marquet-Blouin E, Burel C, et al. Transient co-expression for fast and high-yield production of antibodies with human-like N-glycans in plants. Plant Biotechnol J 2009; 7:442 - 55; http://dx.doi.org/10.1111/j.1467-7652.2009.00414.x; PMID: 19422604