Abstract
Small ubiquitin-related modifier (SUMO) is a highly conserved protein that is covalently attached to target proteins. This posttranslational modification, designated SUMOylation, is a major protein-conjugation-driven strategy designed to regulate structure and function of cellular proteins. SUMOylation consists of an enzymatic cascade involving the E1-activating enzyme and the E2-conjugating enzyme. The SUMO-E1 enzyme consists of two subunits, a heterodimer of activation of Smt3p 1 (Aos1) and ubiquitin activating enzyme 2 (Uba2), which resembles the N- and C-terminal halves of ubiquitin E1 (Uba1). Herein, we describe the rational design of a single polypeptide version of SUMO-E1, a chimera of mouse Aos1 and Uba2 subunits, termed mAU, in which the functional domains appear to be arranged in a fashion similar to Uba1. We also describe the construction of a mAU plasmid for expression in a baculovirus-insect cell system and present an in situ SUMOylation assay using the recombinant mAU. Our results showed that mAU has SUMO-E1 activity, thereby indicating that mAU can be expressed in baculovirus-insect cells and represents a suitable source of SUMO-E1.
Introduction
Ubiquitin is a 76 amino acid residue protein, which possesses the typical “β-grasp” or “ubiquitin-fold” structure, wherein four β-sheets pack around a central α-helix.Citation1 Ubiquitin was first described as a “heat-stable polypeptide of the ATP dependent proteolytic system”.Citation2 It was subsequently found to function as a posttranslational protein modifier, which covalently attached to target proteins via an isopeptide linkage between the carboxyl terminus of ubiquitin and the ε-amino group of lysines on target proteins, thereby regulating protein stability and function.Citation1 Posttranslational modification by ubiquitin, called ubiquitinylation, plays important roles in regulating a wide variety of cellular signals involved in cell growth, differentiation, and death.1 When the nuclear pore targeting of Ran GTPase activating enzyme 1 (RanGAP1) via specific interaction with the nuclear pore component, Ran-binding protein 2/358 kDa nuclear pore protein (RanBP2/Nup358), was first investigated nearly 15 y ago, it became clear that protein modification included a ubiquitin-like modifier, a ~100 residue polypeptide, termed SUMO (small ubiquitin-related modifier).Citation3-Citation5 SUMO possesses the typical “β-grasp” or “ubiquitin-fold” structure and covalently attaches to target proteins in a manner similar to ubiquitin, a process referred to as SUMOyaltion.Citation6-Citation9 Like ubiquitinylation, SUMOylation plays a role in regulating many cellular processes, including in genetic/epigenetic regulation, cell structural maintenance, cell signaling, and cell cycle progression.Citation10,Citation11
In this paper, we summarize the enzymatic cascade of the SUMOylation reaction and structure of the enzymes involved in SUMOylation. In particular, we focus on the SUMO-activating enzyme E1, the first enzyme required for initiating the SUMOylation cascade. To reduce the number of recombinant proteins required for the in vitro SUMOylation reaction and to eliminate formation of the heterodimer of the two subunits of the SUMO-E1 enzyme, we generated a linear fusion protein consisting of the two subunits and expressed this construct in a baculovirus-insect cell system. The genetic manipulation and heterologous expression of a functional linear-type of SUMO-E1 should aid researchers interested in characterizing the SUMO pathway, as well as help to bioengineer E1 enzymes involved in other SUMO/ubiquitin-like conjugation pathways.
Enzymatic Mechanism and Structure of SUMO-E1
SUMOyaltion is an important mechanism involved in regulating protein structure and function, and therefore should be under stringent enzymatic control. SUMOyaltion involves an enzymatic cascade composed of at least two components.Citation7,Citation8 Initially, SUMOylation requires activation by the E1-activating enzyme, a heterodimer of activation of Smt3p 1 (Aos1) and the ubiquitin activating enzyme 2 (Uba2) (). The E1 enzyme catalyzes the formation of a thioester-linked complex between SUMO and the E2 enzyme. This process is initiated by activation of the carboxyl terminus of SUMO by adenylation, followed by a thioesterification reaction in which SUMO is conjugated to a cysteine residue at the active site of Uba2 in the E1 enzyme. SUMO is then transferred to the active site cysteine of the E2 enzyme, Ubc9, via a trans-thioesterification reaction. A SUMO-charged E2 enzyme and substrate are finally bound with or without the assistance of a distinct class of SUMO E3-ligases, resulting in the activated SUMO bound to the substrate through an isopeptide linkage. In many cases, SUMO-E1 and -E2 enzymes are sufficient to conjugate SUMO to substrate proteins, which is in contrast to the essential requirement of three components, E1, E2, and E3, in the ubiquitin conjugation reaction.
Figure 1. Schematic representation illustrating the activation of SUMO by an E1 enzyme. (A) Conjugation of SUMO to a substrate requires the initial activation of SUMO by the SUMO-activating enzyme (E1), a heterodimer of Aos1 and Uba2. The E1 enzyme uses ATP to activate the carboxyl terminus of SUMO as a high-energy anhydride (SUMO-AMP). This step is termed adenylation. The E1 catalytic cysteine residue then attacks the adenylated SUMO to form a thioester intermediate, referred to as thioesterification. The activated SUMO molecule is then transferred through a trans-thioesterification to a cysteine residue in the SUMO-conjugating enzyme (E2; Ubc9). Finally, the E2-bound SUMO molecule attacks a substrate either in the presence or in the absence of an SUMO-E3 ligase; this process is termed amidolysis. (B) The domain organization of mouse ubiquitin E1 (Uba1; the top bar) and SUMO-E1 (a heterodimer of Aos1 and Uba2 subunits; the middle bars). A predicted structure of the Aos1-Uba2 chimeric recombinant protein is also illustrated and designated as mAU (the bottom bar). The numbers represent residues of each polypeptide. The catalytic cysteine residues at 632, 173, and 425 amino acid residues in Uba1, Una2, and mAU, respectively, are also indicated as vertical lines.
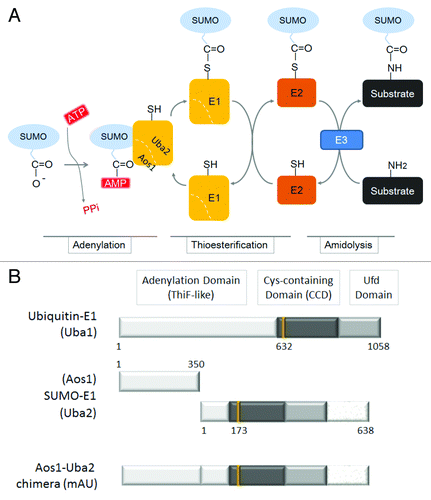
The SUMO-E1 enzyme of the Aos1-Uba2 heterodimer was originally discovered in the yeast Saccharomycess cerevisiae.Citation12 Successive studies revealed the existence of a yeast homolog of Aos1 and Uba2 in humans, called SUMO activating enzyme 1 (Sae1) and Sae2.Citation13,Citation14 By comparing the amino acid sequences of the SUMO-E1 enzyme (Aos1-Uba2 heterodimer) with the ubiquitin-E1 enzyme (a single liner polypeptide Uba1), the similarities of Aos1 to the N-terminal and Uba2 to the C-terminal halves of Uba1 were observed (). The crystal structures of human SUMO-E1 and that of the partly resolved mouse Uba1 showed that both E1 enzymes possess three modular domains.Citation7,Citation15-Citation18 The first ThiF-like domain of SUMO-E1 contributes to adenylation and is composed of Aos1 and the N-terminal portion of Uba2. ThiF is an enzyme required for the thiamine biosynthetic pathway in Escherichia coli.Citation19,Citation20 The second cysteine-containing domain (CCD) contains an enzymatic active cysteine residue that is responsible for thioesterification. The carboxyl terminal ubiquitin-fold domain (Ufd) binds to SUMO-E2, Ubc9, and recruits SUMO-E2 to facilitate the transfer of the thioesterified SUMO to the E2 enzyme. It is predicted that the spatial order of these domains must be restricted and further implies that, during adenylation, thioesterification, and trans-thioesterification processes, these three domains in the E1 enzyme undergo dynamic remodeling and conformational changes in a regulated manner.Citation15,Citation17,Citation18
Currently, six kinds of proteins that show similarity to SUMO-E1 and ubiquitin-E1 enzymes have been identified and most have been recognized as ubiquitin-like proteins, such as NEDD8,Citation21 ISG15,Citation22 FAT10,Citation23 URM-1,Citation24 UFM-1,Citation25 and ATG8/12.Citation26 Among them, the two E1 enzymes for SUMO and NEDD8 consist of two subunits, whereby each subunit resembles the N- and C-terminal halves of ubiquitin E1. Since neither of the subunits alone show E1 activity, the heterodimeric structure of the SUMO- and NEDD8-E1 enzymes lends itself to several modes of regulation that would not be applicable for a linear-type E1, such as Uba1. However, the reason for this structural complexity and the physiological significance of the heterodimer formation of SUMO- and NEDD8-E1 enzymes remains unresolved.
Construction of mAU and its Expression in a Baculovirus and/or Insect Cell System
Although the biochemical and physiological function of heterodimer formation of the SUMO-E1 enzyme remains unresolved, we inferred that by preserving the three-domain structure commonly observed in E1 enzymes, SUMO-E1 may function without the requirement to dimerize. In other words, as long as the three domains were arranged properly in E1, two subunits of Aos1 and Uba2 could be fused into a single-linear polypeptide, as observed for ubiquitin-E1 Uba1: the domain order of chimeric Aos1 and Uba2, namely mAU, was seemingly similar to Uba1 (). In addition, if mAU possessed SUMO-E1 activity, a reduction in the number of recombinant proteins required to perform the in vitro SUMOylation reaction would be observed, thereby making the experimental procedure simpler and more efficient. We also anticipated improvement in the folding efficiency of the linear fusion of mAU compared with the protein folding of the two subunits of Aos1 and Uba2, which are independently expressed and then assembled in the test tube.
To test whether a single-polypeptide version of SUMO-E1 has enzymatic activity, we designed a chimera consisting of the Aos1 and Uba2 subunits, and expressed the Aos1-Uba2 chimeric protein, mAU, in Escherichia coli.Citation27 Briefly, cDNA fragments containing full-length mouse Aos1 and Uba2 were amplified by polymerase chain reaction (PCR), followed by ligation into the pGEX-KG vector (GE Healthcare). The resulting plasmid, pGEX-mAU, carried a Aos1-Uba2 fused fragment, in which the EcoRI site was present between the Aos1 and Uba2 fragments for purpose of ligating these two fragments. GST-mAU expressed in bacteria possessed SUMO-E1 activity and purification of the recombinant protein could be performed. However, the yield and purity of the GST-mAU expressed in bacteria was not satisfactory: less than 1 μg of GST-AU was obtained with multiple degradation products from 100 ml of bacterial culture ().Citation27
Figure 2. Construction of the mAU baculovirus/insect expression vector and the in situ SUMOylation assay using the recombinant His6-mAU. (A) Mouse AU was amplified and cloned into NotI and XhoI sites of the multi-cloning site of pFastBac-HT-C. The pFastBac-HT-C vector plasmid is designed to express inserted gene fragments in the multi-cloning site as fusion proteins with an N-terminal 6 × His-tag sequence (His6) followed by the Tobacco Etch Virus (TEV) protease cleavage site. Thus, 45 residues including the His6 and TEV recognition/cleavage regions were present in our construct. These additional residues were considered to not influence SUMO-E1 enzymatic activity of mAU. The EcoRI site inserted between the Aos1 and Uba2 fragments is indicated. ATG, start site; Six × His, hexa-histidine tag sequence; TEV, TEV-protease recognition site and cleavage site; MCS, multi-cloning sites. (B) Schematic representation of His6-mAU recombinant protein. The numbers show amino acid residues. There are two amino acid residues between Aos1 and Uba2, glutamic acid (E), and phenylalanine (F), which were derived from the linker fragment of the EcoRI site (see the plasmid map in [A]). (C) Comparison of bacteria expressed and baculovirus-insect cell-expressed mAU proteins. Total lysate from either GST-mAU-expressing E. coli or His6-mAU expressing baculovirus-insect cells was subjected to immunoblot analysis using an anti-GST or anti-His6 antibody, respectively. Positions of full-length GST-mAU and His6-mAU recombinant proteins are indicated by arrows. Protein size markers are shown on the left. (D) Application of His6-mAU recombinant protein to the in situ SUMOylation assay.Citation29,Citation30 HeLa cells were permeabilized with 0.01% digitonin followed by 4% paraformaldehyde fixation. Twenty μl of the in situ SUMOylation buffer containing Ubc9, GFP-SUMO-1, and Mg2+-ATP, supplemented with (+, left column) or without (−, right column) the His6-mAU recombinant protein. After incubation for 20 min at 18 °C, GFP (upper panel) and DAPI (bottom panel) signals were observed by fluorescence microscopy. Bars indicate 10 μm.
![Figure 2. Construction of the mAU baculovirus/insect expression vector and the in situ SUMOylation assay using the recombinant His6-mAU. (A) Mouse AU was amplified and cloned into NotI and XhoI sites of the multi-cloning site of pFastBac-HT-C. The pFastBac-HT-C vector plasmid is designed to express inserted gene fragments in the multi-cloning site as fusion proteins with an N-terminal 6 × His-tag sequence (His6) followed by the Tobacco Etch Virus (TEV) protease cleavage site. Thus, 45 residues including the His6 and TEV recognition/cleavage regions were present in our construct. These additional residues were considered to not influence SUMO-E1 enzymatic activity of mAU. The EcoRI site inserted between the Aos1 and Uba2 fragments is indicated. ATG, start site; Six × His, hexa-histidine tag sequence; TEV, TEV-protease recognition site and cleavage site; MCS, multi-cloning sites. (B) Schematic representation of His6-mAU recombinant protein. The numbers show amino acid residues. There are two amino acid residues between Aos1 and Uba2, glutamic acid (E), and phenylalanine (F), which were derived from the linker fragment of the EcoRI site (see the plasmid map in [A]). (C) Comparison of bacteria expressed and baculovirus-insect cell-expressed mAU proteins. Total lysate from either GST-mAU-expressing E. coli or His6-mAU expressing baculovirus-insect cells was subjected to immunoblot analysis using an anti-GST or anti-His6 antibody, respectively. Positions of full-length GST-mAU and His6-mAU recombinant proteins are indicated by arrows. Protein size markers are shown on the left. (D) Application of His6-mAU recombinant protein to the in situ SUMOylation assay.Citation29,Citation30 HeLa cells were permeabilized with 0.01% digitonin followed by 4% paraformaldehyde fixation. Twenty μl of the in situ SUMOylation buffer containing Ubc9, GFP-SUMO-1, and Mg2+-ATP, supplemented with (+, left column) or without (−, right column) the His6-mAU recombinant protein. After incubation for 20 min at 18 °C, GFP (upper panel) and DAPI (bottom panel) signals were observed by fluorescence microscopy. Bars indicate 10 μm.](/cms/asset/4d952b0e-043d-4f91-a083-581108a6f38c/kbie_a_10927544_f0002.gif)
Thus, we started to generate a bacmid DNA vector for expression of mAU in a baculovirus and/or insect cell system with the intention to dramatically improve the yields of recombinant mAU. To this end, using pGEX-mAU as a template for PCR, the mAU fragment was amplified, followed by cloning into the NotI and XbaI sites of the pFastBac-HT-C vector (; Invitrogen). The resulting plasmid was termed pFastBac-His6-mAU, and was used to express a His6-mAU recombinant protein from pFastBac-His6-mAU (). It should be noted that the EcoRI site was present as a linker sequence between the Aos1 and Uba2 fragments because of the template used in the PCR, as described above. We speculated that the linker ensured the sufficient flexibility of the ThiF-like domain to carry out the intra-molecular interactions required during activation of the SUMO molecule. However, it remains unclear as to whether there is a linker polypeptide more effective than the linker currently present in the His6-mAU construct described herein.
Kanemaru and Saitoh reported that reasonable levels of the His6-mAU recombinant protein were expressed in baculovirus-insect cells.Citation28 The His6-mAU recombinant protein expressed in baculovirus-insect cells was reasonably soluble and stable in an aqueous environment, and therefore could be purified by conventional affinity column chromatography: 1−2 mg of the 130 kDa protein was obtained from 100 ml Sf9 cell culture and the 130-kDa His6-mAU recombinant protein can be purified efficiently by conventional nickel affinity chromatography.Citation28 When compared with GST-mAU expression in E. coli, the full-length His6-mAU recombinant proteins were induced efficiently with less degraded products in baculovirus-insect cells (). As shown in , the baculovirus-insect expressed mAU showed enzymatic activity of SUMO-E1, at least, in the in situ SUMOylation assay:Citation29,Citation30 the assay revealed enhanced nuclear rim staining, which represented an accumulation of GFP-SUMO-1 conjugated to the nuclear pore complex, when the digitonine-permeabilized and paraformaldehyde-fixed HeLa cells were treated with the SUMOylation buffer containing His6-mAU. In contrast, GFP signals in the reaction containing the SUMOylation buffer without His6-mAU exhibited negligible GFP signals. These data indicate that the His6-mAU expressed in baculovirus-insect cells possesses SUMO-E1 enzymatic activity. According to our preliminary experiment, the enzyme activity of mAU produced from baculovirus was approximately the same as that produced from E. coli. However, more detailed in vitro SUMOylation experiments remain to be performed, including comparison of the kinetic parameters, such as Km and/or Kd values, of recombinant mAU protein expressed in baculovirus-insect cells with the bacterially expressed mAU. Given that the mAU expressed in bacteria was 10-fold less active than the Aos1-Uba2 heterodimer expressed in bacteria,Citation27 it should also be investigated whether the enzymatic activity of the recombinant His6-mAU is equal to that of the baculovirus-insect cell-expressed Aos1-Uba2 heterodimer. For this purpose, we are preparing the recombinant Aos1-Uba2 heterodimer complex in a baculovirus-insect cell system.
Conclusion
We have described the rational design and construction of a recombinant chimeraconsisting of a linear fusion of Aos1 and Uba2. The resulting chimeric polypeptide, mAU, encompasses three domains functionally indispensable for E1 enzymatic activity. Since the yield of the recombinant mAU expressed in baculovirus-insect cells is reasonable, we highly recommend the baculovirus-insect cell system for expression of mAU. In addition, our strategy for bioengineering SUMO-E1 enzymes should be equally applicable to the E1 enzymes of other SUMO/ubiquitin family members.
Disclosure of Potential Conflicts of Interest
No potential conflict of interest was disclosed.
Acknowledgments
We thank all the members of the Saitoh Laboratory for helpful discussion. This work was supported by JSPS KAKENHI Grant no. 23616004 and by MEXT KAKENHI Grant no. 23131519 to H.S.
References
- Hershko A, Ciechanover A. The ubiquitin system. Annu Rev Biochem 1998; 67:425 - 79; http://dx.doi.org/10.1146/annurev.biochem.67.1.425; PMID: 9759494
- Ciechanover A, Hod Y, Hershko A. A heat-stable polypeptide component of an ATP-dependent proteolytic system from reticulocytes. 1978. Biochem Biophys Res Commun 2012; 425:565 - 70; http://dx.doi.org/10.1016/j.bbrc.2012.08.025; PMID: 22925675
- Mahajan R, Delphin C, Guan T, Gerace L, Melchior F. A small ubiquitin-related polypeptide involved in targeting RanGAP1 to nuclear pore complex protein RanBP2. Cell 1997; 88:97 - 107; http://dx.doi.org/10.1016/S0092-8674(00)81862-0; PMID: 9019411
- Matunis MJ, Coutavas E, Blobel G. A novel ubiquitin-like modification modulates the partitioning of the Ran-GTPase-activating protein RanGAP1 between the cytosol and the nuclear pore complex. J Cell Biol 1996; 135:1457 - 70; http://dx.doi.org/10.1083/jcb.135.6.1457; PMID: 8978815
- Saitoh H, Pu R, Cavenagh M, Dasso M. RanBP2 associates with Ubc9p and a modified form of RanGAP1. Proc Natl Acad Sci U S A 1997; 94:3736 - 41; http://dx.doi.org/10.1073/pnas.94.8.3736; PMID: 9108047
- Bayer P, Arndt A, Metzger S, Mahajan R, Melchior F, Jaenicke R, Becker J. Structure determination of the small ubiquitin-related modifier SUMO-1. J Mol Biol 1998; 280:275 - 86; http://dx.doi.org/10.1006/jmbi.1998.1839; PMID: 9654451
- Tang Z, Hecker CM, Scheschonka A, Betz H. Protein interactions in the sumoylation cascade: lessons from X-ray structures. FEBS J 2008; 275:3003 - 15; http://dx.doi.org/10.1111/j.1742-4658.2008.06459.x; PMID: 18492068
- Geiss-Friedlander R, Melchior F. Concepts in sumoylation: a decade on. Nat Rev Mol Cell Biol 2007; 8:947 - 56; http://dx.doi.org/10.1038/nrm2293; PMID: 18000527
- Kerscher O. SUMO junction-what’s your function? New insights through SUMO-interacting motifs. EMBO Rep 2007; 8:550 - 5; http://dx.doi.org/10.1038/sj.embor.7400980; PMID: 17545995
- Cubeñas-Potts C, Matunis MJ. SUMO: a multifaceted modifier of chromatin structure and function. Dev Cell 2013; 24:1 - 12; http://dx.doi.org/10.1016/j.devcel.2012.11.020; PMID: 23328396
- Sarge KD, Park-Sarge OK. SUMO and its role in human diseases. Int Rev Cell Mol Biol 2011; 288:167 - 83; http://dx.doi.org/10.1016/B978-0-12-386041-5.00004-2; PMID: 21482412
- Johnson ES, Schwienhorst I, Dohmen RJ, Blobel G. The ubiquitin-like protein Smt3p is activated for conjugation to other proteins by an Aos1p/Uba2p heterodimer. EMBO J 1997; 16:5509 - 19; http://dx.doi.org/10.1093/emboj/16.18.5509; PMID: 9312010
- Okuma T, Honda R, Ichikawa G, Tsumagari N, Yasuda H. In vitro SUMO-1 modification requires two enzymatic steps, E1 and E2. Biochem Biophys Res Commun 1999; 254:693 - 8; http://dx.doi.org/10.1006/bbrc.1998.9995; PMID: 9920803
- Gong L, Li B, Millas S, Yeh ET. Molecular cloning and characterization of human AOS1 and UBA2, components of the sentrin-activating enzyme complex. FEBS Lett 1999; 448:185 - 9; http://dx.doi.org/10.1016/S0014-5793(99)00367-1; PMID: 10217437
- Lois LM, Lima CD. Structures of the SUMO E1 provide mechanistic insights into SUMO activation and E2 recruitment to E1. EMBO J 2005; 24:439 - 51; http://dx.doi.org/10.1038/sj.emboj.7600552; PMID: 15660128
- Szczepanowski RH, Filipek R, Bochtler M. Crystal structure of a fragment of mouse ubiquitin-activating enzyme. J Biol Chem 2005; 280:22006 - 11; http://dx.doi.org/10.1074/jbc.M502583200; PMID: 15774460
- Groettrup M, Pelzer C, Schmidtke G, Hofmann K. Activating the ubiquitin family: UBA6 challenges the field. Trends Biochem Sci 2008; 33:230 - 7; http://dx.doi.org/10.1016/j.tibs.2008.01.005; PMID: 18353650
- Lorenz S, Cantor AJ, Rape M, Kuriyan J. Macromolecular juggling by ubiquitylation enzymes. BMC Biol 2013; 11:65; http://dx.doi.org/10.1186/1741-7007-11-65; PMID: 23800009
- Duda DM, Walden H, Sfondouris J, Schulman BA. Structural analysis of Escherichia coli ThiF. J Mol Biol 2005; 349:774 - 86; http://dx.doi.org/10.1016/j.jmb.2005.04.011; PMID: 15896804
- Lehmann C, Begley TP, Ealick SE. Structure of the Escherichia coli ThiS-ThiF complex, a key component of the sulfur transfer system in thiamin biosynthesis. Biochemistry 2006; 45:11 - 9; http://dx.doi.org/10.1021/bi051502y; PMID: 16388576
- Walden H, Podgorski MS, Schulman BA. Insights into the ubiquitin transfer cascade from the structure of the activating enzyme for NEDD8. Nature 2003; 422:330 - 4; http://dx.doi.org/10.1038/nature01456; PMID: 12646924
- Yuan W, Krug RM. Influenza B virus NS1 protein inhibits conjugation of the interferon (IFN)-induced ubiquitin-like ISG15 protein. EMBO J 2001; 20:362 - 71; http://dx.doi.org/10.1093/emboj/20.3.362; PMID: 11157743
- Jin J, Li X, Gygi SP, Harper JW. Dual E1 activation systems for ubiquitin differentially regulate E2 enzyme charging. Nature 2007; 447:1135 - 8; http://dx.doi.org/10.1038/nature05902; PMID: 17597759
- Furukawa K, Mizushima N, Noda T, Ohsumi Y. A protein conjugation system in yeast with homology to biosynthetic enzyme reaction of prokaryotes. J Biol Chem 2000; 275:7462 - 5; http://dx.doi.org/10.1074/jbc.275.11.7462; PMID: 10713047
- Komatsu M, Chiba T, Tatsumi K, Iemura S, Tanida I, Okazaki N, Ueno T, Kominami E, Natsume T, Tanaka K. A novel protein-conjugating system for Ufm1, a ubiquitin-fold modifier. EMBO J 2004; 23:1977 - 86; http://dx.doi.org/10.1038/sj.emboj.7600205; PMID: 15071506
- Mizushima N, Noda T, Yoshimori T, Tanaka Y, Ishii T, George MD, Klionsky DJ, Ohsumi M, Ohsumi Y. A protein conjugation system essential for autophagy. Nature 1998; 395:395 - 8; http://dx.doi.org/10.1038/26506; PMID: 9759731
- Uchimura Y, Nakao M, Saitoh H. Generation of SUMO-1 modified proteins in E. coli: towards understanding the biochemistry/structural biology of the SUMO-1 pathway. FEBS Lett 2004; 564:85 - 90; http://dx.doi.org/10.1016/S0014-5793(04)00321-7; PMID: 15094046
- Kanemaru A, Saitoh H. High-yield expression of mouse Aos1-Uba2-fusion SUMO-activating enzyme, mAU, in a baculovirus-insect cell system. Biosci Biotechnol Biochem 2013; 77:1575 - 8; http://dx.doi.org/10.1271/bbb.130070; PMID: 23832333
- Saitoh N, Uchimura Y, Tachibana T, Sugahara S, Saitoh H, Nakao M. In situ SUMOylation analysis reveals a modulatory role of RanBP2 in the nuclear rim and PML bodies. Exp Cell Res 2006; 312:1418 - 30; http://dx.doi.org/10.1016/j.yexcr.2006.01.013; PMID: 16688858
- Muramatsu M, Uwada J, Matsumoto N, Saitoh H. A simple in situ cell-based sumoylation assay with potential application to drug screening. Biosci Biotechnol Biochem 2010; 74:1473 - 5; http://dx.doi.org/10.1271/bbb.100081; PMID: 20622442