Abstract
All biological platforms for the manufacture of biopharmaceutical proteins produce an initially turbid extract that must be clarified to avoid fouling sensitive media such as chromatography resins. Clarification is more challenging if the feed stream contains large amounts of dispersed particles, because these rapidly clog the filter media typically used to remove suspended solids. Charged polymers (flocculants) can increase the apparent size of the dispersed particles by aggregation, facilitating the separation of solids and liquids, and thus reducing process costs. However, many different factors can affect the behavior of flocculants, including the pH and conductivity of the medium, the size and charge distribution of the particulates, and the charge density and molecular mass of the polymer. Importantly, these properties can also affect the recovery of the target protein and the overall safety profile of the process. We therefore used a design of experiments approach to establish reliable predictive models that characterize the impact of flocculants during the downstream processing of biopharmaceutical proteins. We highlight strategies for the selection of flocculants during process optimization. These strategies will contribute to the quality by design aspects of process development and facilitate the development of safe and efficient downstream processes for plant-derived pharmaceutical proteins.
Plants have been used to produce recombinant pharmaceutical proteins since the late 1980s, but the transition from an experimental principle to a commercially competitive platform took until 2012, when the FDA approved ELELYSO for human use.Citation1 This delay was in part due to the focus on upstream production benefits (economy, scalability, and safety) whereas downstream processing (DSP) received comparably little attention even though it can account for up to 80% of the overall production costs.Citation2 Many strategies have been developed to simplify DSP, e.g., (1) fusing target proteins to elastin-like polypeptides so they can be purified by inverse transition cycling,Citation3 (2) the heat precipitation of host cell proteins (HCPs),Citation4 and (3) aqueous two-phase separation.Citation5 However, most of these techniques require an upstream filtration step to clarify the extract. The filters are usually disposable to minimize the risk of contaminationCitation6 and can therefore account for more than 25% of the DSP costs.Citation7 Maximizing the filter capacity helps to achieve cost-effective production, and the rational selection of filter material is one way to achieve this goal, but all filters become rapidly exhausted when challenged with a heavily particulate feed stream.Citation8
To improve filter capacity beyond the nominal optimum, we have recently tested a broad range of flocculants.Citation9 These high-molecular-mass polymers may carry strong negative charges (e.g., polyacrylamides) or strong positive charges (e.g., modified polyethylenimines) that promote aggregation either by bridgingCitation10 or the charge neutralization of dispersed particles ().Citation11 This aggregation process is known as “flocculation” because it increases the average apparent size of the dispersed particles resulting in the formation of flocks. Flocculants therefore promote the removal of solids using less expensive coarse filters (e.g., bag filters), thus improving the capacity of the finer filters (e.g., depth filters) and reducing the amount of expensive filter material required to achieve a feed stream with a certain minimum turbidity threshold. The effectiveness of flocculants depends on the nature and concentration of the particulates, the concentration, charge density and length of the polymer, the shear rate, and properties of the feed stream such as pH and ionic strength.Citation10,Citation12-Citation14 However, the choice of flocculant can also affect the recovery of the target proteins because the latter may also aggregate due to interactions with the charged polymers.Citation15,Citation16
Figure 1. Effect of flocculants on turbidity and particle size distribution. (A) Charged polymers (red) initially bind to particles of opposite charge (black) in a coiled state (I). Depending on the relaxation time tR, adsorption time tA, and collision time tC, the polymer can then either interact with a second particle to form bridges (II) if the polymer extends beyond the inverse Debye length (green dashed line, κ−1) or adopt an expanded conformation (III) and neutralize the surface charges of the particle.Citation10,Citation14 (B) The selective use of different flocculants, e.g., according to the conductivity of the feed stream, facilitates a constantly low bag filtrate turbidity (area hatched in green) over a wide parameter range (data for pH 8.0, 30 min incubation, 4 g L−1 polymer concentration). Error bars indicate standard deviation of the model prediction. (C) Some polymers may induce flocculation and yield a clear supernatant, but if these flocks are fragile, they will be disrupted by filtration and the clarified extract will remain turbid (Praestol 822 BS). The initial homogenate turbidity is indicated by the dotted line. Error bars indicate standard deviation, n ≥ 3. (D) Before filtration, particles in the flocculated extract (solid blue) are generally larger (~3200 µm) than those in the untreated controls (solid green, ~2500 µm). After bag filtration, the polymer-free extract still contains large amounts of particulates in the 0.5−10 µm range (dashed green), whereas the flocculated sample (dashed blue) contains only a small fraction of particles in the 10-µm range. The majority of the dispersed particles in the filtered, flocculated samples were ~15 nm in diameter (the approximate size of antibodies and ribulose-1,5-bisphosphate carboxylase oxygenase oligomers).
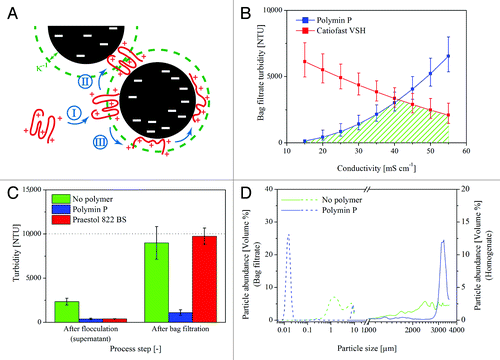
The full potential of flocculation for cost reduction in DSP can be exploited by identifying the polymer which is most effective under the anticipated working conditions (e.g., a specific pH and conductivity). A design of experiments (DoE) approach is appropriate under these circumstances, because many different factors and settings can be tested with only moderate effort, and refined models for the flocculant can be established to investigate the robustness of the process.Citation17 The latter is important because a mechanistic model of flocculation may be available for well-defined model systemsCitation14 but not for complex feedstock. Nevertheless, insight into the most important mechanisms can be obtained if a flocculant is incorporated in a DoE approach as a defined combination of continuous parameters (e.g., a parameter set consisting of charge density and molecular mass) rather than a categorical factor, because the former will yield additional information about the flocculant properties that affect particle aggregation. This information can then be used to select additional flocculants with relevant properties that were not included in the initial screen because time and/or resources were limited. We have used this approach to screen a total of 23 different polymers under varying pH, conductivity, incubation time, and temperature conditions and modeled their impact on turbidity and filter capacity.Citation9 Among the initial set of 18 flocculants (), only one (the modified polyethylenimine “Polymin P”, BASF) achieved a significant reduction in turbidity after bag filtration and an increase in the capacity of the subsequent depth filter.
Table 1. Flocculants used to precipitate particulates from crude tobacco extracts prior to depth filtration. The best performers are shown in bold
The DoE-based models also revealed that the effectiveness of a polymer changes under varying process conditions (e.g., pH and conductivity) or when applied in a set of processes conducted under different conditions (e.g., different products or expression platforms). The simplest way to adapt to these situations is to adjust the flocculant concentration but this may not be sufficient in every case, as we observed when testing Polymin P at high conductivity (). Hence, the selection of a set of polymers with complementary flocculation properties can be more expedient and complies with the principles of quality by design.Citation18 We established such a flocculant set by selecting five new candidates () based on the properties of Polymin P, which we anticipated would have a positive effect on flock formation based on our predictive models. The three polymers with the greatest similarity to Polymin P in terms of charge density and size accordingly achieved the anticipated increase in depth filter capacity. The resulting set of polymers can now be used to (1) induce flocculation in various feed streams with different properties (e.g., pH and conductivity) because they have complementary high performance windows in terms of particle flocculation (e.g., Polymin P and Catiofast VSH, ) and (2) to replace one another if necessary because of technical or regulatory issues (e.g., Polymin P and Lupasol PS, data not shown).
Settling experiments are often used to assess flocculation and the effectiveness of a polymer.Citation19 However, the results can be misleading if filters are used instead of settling tanks during the final process, e.g., to accelerate clarification. This is because certain polymers (e.g., Praestol 822 BS) can form aggregates that exhibit promising settling behavior but are sensitive to pumping and filtration, which can reduce the effective flock size due to shear stress. The resulting aggregates are smaller and may pass through the filter, thus counteracting the anticipated improvement in downstream filter capacity or reducing the ability of the corresponding filter to clarify the feed stream (). Shear stress can also be a problem during the initial mixing of the polymer and feed stream. Although some degree of mixing is essential to bring the flocculant in contact with dispersed particles, prolonged mixing can result in the irreversible disruption of flocks or a reduction in the average flock size, again affecting the performance of downstream filters.
The most effective flocculants change the particle size distribution (), which means that the filter area and retention rating must be adapted to match the new feed stream conditions to achieve optimal filter capacity and clarification. Generally, the addition of polymers will favor the use of wider filter pores because the dispersed particle size increases following the addition of flocculants ().
Figure 2. Effect of flocculants on filter capacity, HCPs and chromatography. (A) In the absence of flocculants, depth filters with a fine first layer (PDF4, 3 µm) achieved higher capacities, whereas no significant difference was achieved by adding flocculants before passing the feed stream through filters with a coarser first layer (PDH4, 8 µm). Error bars indicate standard deviation, n ≥ 3. (B) The HCP composition of a tobacco extract changes according to the pH, conductivity and presence of flocculant. The addition of 2 g L−1 Polymin P (+) results in a visible reduction in the abundance of some HCPs compared with polymer-free samples (–) specifically at pH 4.0 and 15 mS cm−1 (orange arrows). A characteristic smear < 10 kDa is present in all samples containing the polymer (red arrow). The extraction efficacy for certain HCPs changes with the buffer pH (black arrows), e.g., a 10-kDa protein is extracted at pH 6.0 but not 7.0 whereas a 50-kDa protein is extracted at pH 8.0 but not 6.0. Here, the potential target proteins (green arrows) have molecular masses of 27 kDa (DsRed) and 49.5/23 kDa (monoclonal antibody 2G12 heavy and light chain, respectively). In tobacco extracts, the antibody heavy chain band is superimposed on the of ribulose-1,5-bisphosphate carboxylase oxygenase large subunit. (C) Polymin P is present after depth filtration and bound to chromatography ligands with the opposing charge (SP and CM) in a 1-mL column, resulting in a significant pressure increase during sample loading at 1 mL min−1. Note: the pressure curves of Q FF and Capto Adhere overlap.
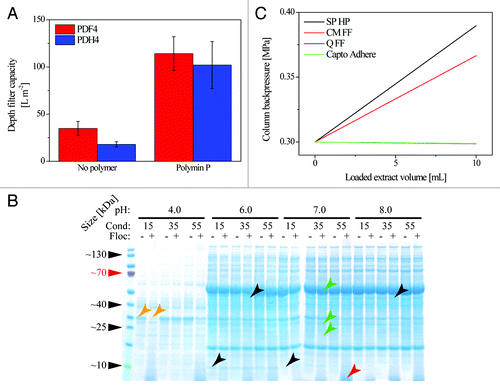
Flocculants may bind preferentially to certain other molecules including HCPs (), DNACitation20 and contaminating virus particles,Citation21,Citation22 resulting in their selective removal and thus improving the purification performance and safety profile of the process. However, the polymers themselves must also be removed during DSP to comply with regulatory guidelines.Citation23 Because of their molecular structure and degree of polymerization, it is challenging to detect flocculants using spectroscopic methods or enzymatic assays. This can make it difficult to detect residual flocculants in the product. For example, we compared a 1 g L−1 buffered solution of Polymin P with a reference buffer and found no difference in the absorbance spectra in the range 300−1000 nm, but we observed a characteristic band below 10 kDa that correlated with the polymer concentration on SDS-PAGE gels stained with Coomassie brilliant blue (). The inability to detect polymers is particularly worrying if the selected flocculant is toxic. We therefore preferentially selected flocculants used in water-treatment processes (e.g., Lupasol PS) or those approved by the FDA for contact with foods (e.g., Polymin P) to minimize the risks associated with non-detection.
Charged flocculants can also affect downstream purification steps where charge is used as the separative principle. Ion exchange chromatography is particularly susceptible to the presence of polymers carrying the opposing charge to the resin because the interaction can rapidly increase the back pressure and thus block the column, as we observed for extracts containing Polymin P applied to SP Sepharose HP and CM Sepharose FF, but not Q Sepharose FF or Capto Adhere (). Therefore, the decision to use flocculants during DSP should always take into account the anticipated chromatographic purification strategy for the target protein, especially the initial capture step. If this step does not rely on a ligand with the opposite charge to that of the polymer, flocculants can be incorporated in the process because they will be discarded with the flow through thus eliminating the risk to subsequent operations.
Flocculants therefore provide a powerful, cost-effective solution for the development of efficient clarification steps during the production of plant-derived biopharmaceutical proteins and may be applied to other production systems as well. They can help to reduce the costs of filter consumables but they must be integrated carefully with new or existing processes in order to fulfill expectations and comply with regulatory guidelines. The latter point should be addressed thoroughly by establishing reliable models that can predict the impact of a polymer on critical process parameters, ideally demonstrating the absence of negative effects on the critical quality attributes of target proteins. The DoE approach is a valuable tool for the development of such models.
Abbreviations: | ||
DoE | = | design of experiments |
DSP | = | downstream processing |
HCP | = | host cell protein |
Disclosure of Potential Conflicts of Interest
No potential conflict of interest was disclosed.
Acknowledgments
The authors would like to thank Dr Richard M Twyman for manuscript editing. This work was funded in part by the European Research Council Advanced Grant “Future-Pharma,” proposal number 269110, and the Fraunhofer-Zukunftsstiftung (Fraunhofer Future Foundation).
References
- Zimran A, Brill-Almon E, Chertkoff R, Petakov M, Blanco-Favela F, Muñoz ET, Solorio-Meza SE, Amato D, Duran G, Giona F, et al. Pivotal trial with plant cell-expressed recombinant glucocerebrosidase, taliglucerase alfa, a novel enzyme replacement therapy for Gaucher disease. Blood 2011; 118:5767 - 73; http://dx.doi.org/10.1182/blood-2011-07-366955; PMID: 21900191
- Wilken LR, Nikolov ZL. Recovery and purification of plant-made recombinant proteins. Biotechnol Adv 2012; 30:419 - 33; http://dx.doi.org/10.1016/j.biotechadv.2011.07.020; PMID: 21843625
- Phan HT, Conrad U. Membrane-based inverse transition cycling: an improved means for purifying plant-derived recombinant protein-elastin-like polypeptide fusions. Int J Mol Sci 2011; 12:2808 - 21; http://dx.doi.org/10.3390/ijms12052808; PMID: 21686152
- Buyel JF, Gruchow HM, Boes A, Fischer R. Rational design of a host cell protein heat precipitation step can simplify the subsequent purification of recombinant proteins from tobacco. Separation and Purification Technology 2014; Submitted
- Platis D, Labrou NE. Development of an aqueous two-phase partitioning system for fractionating therapeutic proteins from tobacco extract. J Chromatogr A 2006; 1128:114 - 24; http://dx.doi.org/10.1016/j.chroma.2006.06.047; PMID: 16828788
- Pegel A, Reiser S, Steurenthaler M, Klein S. Evaluating disposable depth filtration platforms for mAb harvest clarification. BioProcess Int 2011; 9:52 - 6
- Buyel JF, Fischer R. Predictive models for transient protein expression in tobacco (Nicotiana tabacum L.) can optimize process time, yield, and downstream costs. Biotechnol Bioeng 2012; 109:2575 - 88; http://dx.doi.org/10.1002/bit.24523; PMID: 22511291
- Buyel JF, Fischer R. Scale-down models to optimize a filter train for the downstream purification of recombinant pharmaceutical proteins produced in tobacco leaves. Biotechnol J 2013; In press http://dx.doi.org/10.1002/biot.201300369; PMID: 24323869
- Buyel JF, Fischer R. Flocculation increases the efficacy of depth filtration during the downstream processing of recombinant pharmaceutical proteins produced in tobacco. Plant Biotechnol J 2014; 12:240 - 52; http://dx.doi.org/10.1111/pbi.12132; PMID: 24165151
- Gregory J, Barany S. Adsorption and flocculation by polymers and polymer mixtures. Adv Colloid Interface Sci 2011; 169:1 - 12; http://dx.doi.org/10.1016/j.cis.2011.06.004; PMID: 21762869
- Runkana V, Somasundaran P, Kapur PC. Mathematical modeling of polymer-induced flocculation by charge neutralization. J Colloid Interface Sci 2004; 270:347 - 58; http://dx.doi.org/10.1016/j.jcis.2003.08.076; PMID: 14697700
- Barany S, Szepesszentgyörgyi A. Flocculation of cellular suspensions by polyelectrolytes. Adv Colloid Interface Sci 2004; 111:117 - 29; http://dx.doi.org/10.1016/j.cis.2004.07.003; PMID: 15571665
- Pearson CR, Heng M, Gebert M, Glatz CE. Zeta potential as a measure of polyelectrolyte flocculation and the effect of polymer dosing conditions on cell removal from fermentation broth. Biotechnol Bioeng 2004; 87:54 - 60; http://dx.doi.org/10.1002/bit.20097; PMID: 15211488
- Zhou Y, Franks GV. Flocculation mechanism induced by cationic polymers investigated by light scattering. Langmuir 2006; 22:6775 - 86; http://dx.doi.org/10.1021/la060281+; PMID: 16863222
- Holler C, Vaughan D, Zhang C. Polyethyleneimine precipitation versus anion exchange chromatography in fractionating recombinant beta-glucuronidase from transgenic tobacco extract. J Chromatogr A 2007; 1142:98 - 105; http://dx.doi.org/10.1016/j.chroma.2006.08.044; PMID: 16950325
- Fisher RR, Glatz CE. Polyelectrolyte precipitation of proteins: II. Models of the particle size distributions. Biotechnol Bioeng 1988; 32:786 - 96; http://dx.doi.org/10.1002/bit.260320610; PMID: 18587786
- Mandenius CF, Brundin A. Bioprocess optimization using design-of-experiments methodology. Biotechnol Prog 2008; 24:1191 - 203; http://dx.doi.org/10.1002/btpr.67; PMID: 19194932
- Rathore AS. Roadmap for implementation of quality by design (QbD) for biotechnology products. Trends Biotechnol 2009; 27:546 - 53; http://dx.doi.org/10.1016/j.tibtech.2009.06.006; PMID: 19647883
- Tang P, Greenwood J, Raper JA. A model to describe the settling behavior of fractal aggregates. J Colloid Interface Sci 2002; 247:210 - 9; http://dx.doi.org/10.1006/jcis.2001.8028; PMID: 16290458
- Hauser H, Wagner R. Mammalian Cell Biotechnology in Protein Production. Berlin: De Gruyter, 1997.
- Han B, Carlson JO, Powers SM, Wickramasinghe SR. Enhanced Virus Removal by Flocculation and Microfiltration. Biotechnol Bioprocess Eng 2002; 7:6 - 9; http://dx.doi.org/10.1007/BF02935872
- Akeprathumchai S, Han B, Wickramasinghe SR, Carlson JO, Czermak P, Preibeta K. Murine leukemia virus clearance by flocculation and microfiltration. Biotechnol Bioeng 2004; 88:880 - 9; http://dx.doi.org/10.1002/bit.20312; PMID: 15515166
- ICH Expert Working Group. Q6B Guideline: Specifications: Test Procedures and Acceptance Criteria for Biotechnological/Biological Products. In: ICH Harmonised Tripartite Guideline, ed. Geneva: International Conference on Harmonisation of Technical Requirements for Registration of Pharmaceuticals for Human Use, 1999:20.