Abstract
Wet spinning was used to manufacture fibrous alginate hydrogel wound dressings. Samples manufactured using varied operating parameters (decreased air pressure and calcium concentration or increased nozzle diameter and alginate concentration) were compared with the control samples. The changes in the fiber size, Young’s modulus, swelling ratio, fetal bovine serum (BSA) release efficacy, water vapor transmission rate (WVTR) and bacterial inhibition potential due to alterations of the operating parameters were measured. The samples manufactured using altered operating parameters had larger fiber sizes (p < 0.05) and lower Young’s moduli (p < 0.05). The changes in swelling ratios, BSA release efficacies, WVTR and bacterial inhibition potential showed a significant dependence on the degree of calcium crosslinking of the hydrogel and on how tightly the fibers were bound with one another. By manipulating the operating parameters in the wet-spinning system, wound dressings with different properties were successfully made.
Introduction
Alginate is a natural polysaccharide obtained from brown algae. It forms a gel when in contact with divalent cations such as calcium and magnesium. Alginate hydrogel is biocompatible and has been widely investigated as a wound dressing.Citation1-Citation3 An important feature of alginate hydrogel is that when applied to wound beds, it does not adhere to the wounds and is easy to remove.Citation2,Citation4-Citation6 As a result, this hydrogel minimizes the pain and trauma a patient experiences during a dressing change procedure. Hydrogel dressings can provide moisture to dehydrated tissue. This process helps the removal of devitalized tissue during the destructive phase of healing.Citation5,Citation7,Citation8 Alginate dressings are also very useful for moderately to heavily exuding wounds.Citation9 When a hydrogel absorbs water and swells, debris and bacteria are trapped in the polymer interstices, helping to promote a reduced wound bioburden.Citation4 Because of its soft nature, alginate gel dressings fit the contours of wound beds and provide a moist environment that accelerates wound healing.Citation1,Citation10,Citation11 Furthermore, the hydrogel can absorb a large amount of liquid, which helps reduce the frequency of dressing changes.Citation12 Burn wounds have responded well to the application of hydrogels; the cooling effect of hydrogels is both soothing and healing because hydrogels appear to limit the extent of thermal injury.Citation13 Alginate can resist the adherence of common microorganisms and decrease the opportunity for wound infection.Citation14
Clinical results have shown that fibrous hydrogel dressings have advantages over film dressings in terms of dressing performance, patient comfort, safety and cost-effectiveness.Citation15 Hydrogel fibers have been made into wound dressings and have demonstrated good physical strength and water absorbency.Citation16 They also demonstrated good biocompatibility for tissue regeneration purposes.Citation17 The liquid is absorbed into the fibrous structure, promoting superior exudate collection and preventing the dressing from detaching from the wound.Citation18 Furthermore, the voids between the fibers provide better initial oxygen exchange to facilitate wound repair.Citation5,Citation7 Additionally, hydrogels are often used as a drug carrier to prevent the growth of undesired microorganismsCitation19,Citation20 and hence improve the function of the wound dressings.
In this study, different fibrous alginate structures were made by wet spinning, a technique that has been used to make fibrous wound dressings and tissue scaffolds.Citation16,Citation17 The aim of this study was to explore how the different manufacturing parameters of the wet-spinning technology influenced the structure of the final product and to investigate how the properties of the product, such as morphology, fiber size, porosity, degradation and swelling ratio, changed its performance as a wound dressing. The properties tested included tensile strength, swelling ratio, drug release efficacy, water vapor transmission rate and bacterial inhibition.
Results and Discussion
Morphology and fiber size
Pictures and SEM micrographs of the wetspun alginate samples manufactured using different parameters are shown in . The average fiber size of each sample is shown in . At 6 bar, alginate was quickly injected into the calcium solution and flowed to other parts of the solution. When the pressure was reduced to 3 bar, the slow-flowing alginate accumulated where the alginate entered the solution, forming a larger fiber in sample P (p < 0.05). The slow injection rate, which meant a longer manufacturing time, also allowed more time for calcium to crosslink the fiber. A loosely bound fiber structure compared with the control was formed.
Figure 1. (A) Representative of a fresh alginate hydrogel sample made by wet spinning, (B–F) are pictures (left) and SEM micrographs (right) of dry samples: (B) control (air pressure = 6 bar, needle diameter = 150 μm, calcium concentration = 0.5 w/v%, alginate concentration = 1.5 w/v%), (C) sample P (air pressure = 6 bar), (D) sample D (needle diameter = 200 μm), (E) sample Ca (calcium concentration = 5 w/v%), (F) sample A (alginate concentration = 3 w/v%).
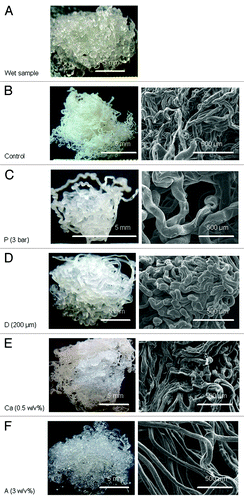
Figure 2. The fiber sizes of the dry samples. Data are presented as the mean ± SD (n = 15). *Indicates data significantly different from the control sample (p < 0.05).
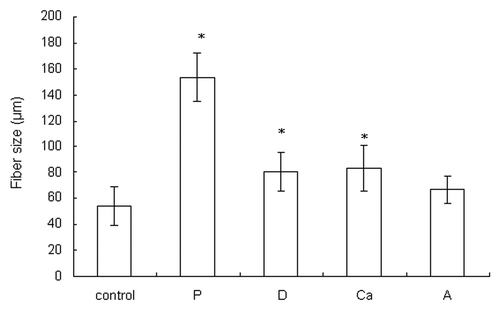
While keeping the rest of the parameters constant, the needle diameter was increased from 150 μm to 200 μm to make sample D, and it formed a larger fiber than the control (p < 0.05). An even larger needle diameter allowed the alginate solution to seep out due to gravity.
When the calcium concentration was decreased from 5% to 0.5%, the alginate molecules had more time to diffuse before they were cross-linked and formed a hydrogel in the calcium solution. The diffusion of alginate in the calcium solution resulted in the formation of a larger fiber in sample Ca (p < 0.05) compared with the control. The slow crosslinking due to the reduced calcium ions allowed more contact/adhesion between the fibers before gelation; hence, a more tightly bound fiber structure compared with the control was formed.
The 3 w/v% alginate solution was more viscous and less easily extruded compared with the control. The 3 w/v% alginate solution passed through the needle more slowly when making sample A. Similar to what happened during the making of sample P, sample A had a larger fiber size (though not significant compared with the control) and a looser structure compared with the control.
In conclusion, the control and sample Ca especially had more compact fibrous structures than the others. The control also had the smallest fiber size of all (p < 0.05, except when compared with sample A). The amount of alginate solution extruded through the needle must be coordinated with the crosslinking time. The optimum settings result in the alginate leaving the needle, forming a hydrogel and binding to the fibers with which it came in contact.
One limitation to the use of wet spinning to make the porous structure is that there were lower and upper limits for each of the parameters tested. Nonetheless, other parameters could be adjusted to accommodate a change in one parameter, such as the fiber size, to obtain the desired properties of the final product.
The samples made were reproducible as far as the size/geometry of the container where alginate fiber formation took place remained the same. The size/geometry of the container influenced how the fibers were compacted. The protocols for making the dressings described in this report produced dressings the size of 5.5 cm by 5 cm. Scaling up to 10 cm × 10 cm, which is a size common for wound dressings, should be easily achieved with a slightly larger container. The characteristics of the scaled up dressings are not expected to change significantly.
Tensile elastic modulus
It is important that a dressing be flexible and soft so that it conforms to the contour of the wound and keeps the patient comfortable. However, it is also important that the wound dressing be able to withstand manipulation in the application process without tearing. None of the samples made in this test were easily broken or torn during handling or while being examined for various properties.
All of the fibrous samples exhibited a delayed split fracture mode because of the isotropic fiber alignment.Citation21 During the tensile test, some fibers were fractured, while others were separated from one another. Hence, the tensile force (N) measured should be a combination of the strength of the fiber material and the adhesive strength between fibers. Compared with the control, the rest of the samples either had a lower degree of crosslinking (sample Ca) or a more loosely bound fiber structure (samples P, D and A). The lack of calcium crosslinking reduced Young’s modulus of the alginate hydrogel,Citation22,Citation23 and the reduced binding between the fibers reduced the tensile stress the samples. The control thus had the highest elastic modulus of all samples (p < 0.05) ().
Figure 3. Young’s modulus of the rehydrated samples. Data are presented as the mean ± SD (n = 6). *Indicates data significantly different from the control sample (p < 0.05).
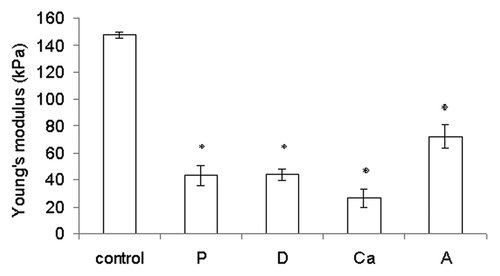
Chiu et al. reported that the commercial dressing Kaltostat® had a Young’s modulus of 1.2 MPa.Citation24 Our wetspun fibrous samples were not as durable but were more flexible (Young’s moduli between 0.026–0.148 MPa) compared with Kaltostat®. Hence, they were expected to conform better to the contours of the wound bed.
Swelling ratio
The swelling ratios measured the capacity of water (exudate) absorbance of the wound dressing. Sample Ca had a significantly lower swelling ratio than the control (p < 0.05) (). This behavior was most likely due to sample Ca’s highly compact structure, which lowered the amount of surface area that was exposed to the aqueous solution. Samples P and D had loose structures, hence higher swelling ratios, compared with the control (p < 0.05). Sample A had a higher initial swelling ratio than the control (p < 0.05) due to its loose structure, but the swelling ratio was not significantly different from the control at the end of the test (> 60 min) (p > 0.05).
Figure 4. The swelling ratios of the dry samples over 90 min. Data are presented as the mean ± SD (n = 6). *Indicates data significantly different from the control sample at 90 min (p < 0.05).
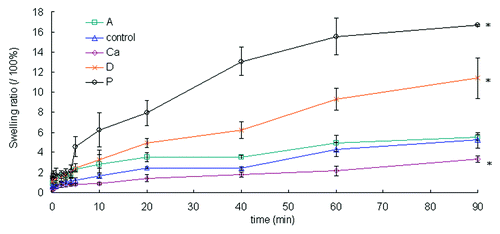
The fibrous structure of the samples quickly absorbed wound exudate, first through capillary forces and then into the fiber structure. This advantage prevents the dressings from detaching from the wound.Citation18 As a result, these fibrous dressings work better than conventional film dressings for burn patients with heavily exudating wounds because the fibrous structure removes the exudates and debris faster.
Water vapor transmission rate (WVTR)
The WVTR is a measure of how well the water in the exudate evaporates when covered with a dressing. Based on , all of the samples had significantly lower WVTRs than an open cup (p < 0.05) and were higher than average commercial wound dressings (between 2,000 to 2,500 g/m2/d).Citation25 Sample Ca had a more compact structure than the control, and it had a lower WVTR (p < 0.05). The samples with more loosely bound structures—samples P, D and A—had higher WVTRs than the control (p < 0.05 for P and D).
Figure 5. The water vapor transmission rate (WVTR) for the open cup and dry samples. Data are presented as the mean ± SD (n = 6). *Indicates data being significantly different from the control sample (p < 0.05).
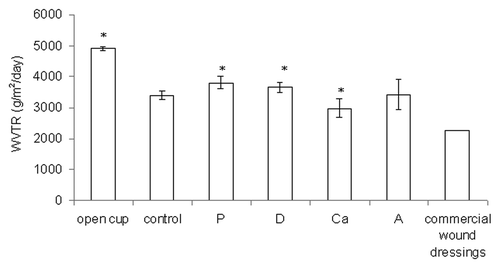
According to Ruiz-Cardona et al., the WVTR of an open cup is 4,026 g/m2/d, that of healthy skin is 200 to 500 g/m2/d, and that of commercial wound dressings is 2,000 to 2,500 g/m2/d.Citation25 An in vivo test has shown that a wound dressing with a WVTR of approximately 2,500 g/m2/d can maintain a moist environment over the wound bed in a moderately to heavily exuding wound, which enhances epithelial cell migration during the healing process.Citation1 The WVTRs of the wetspun samples fell between 2,981 and 3,797 g/m2/d. These values may work for very heavily exuding wounds, but improvements are certainly needed if they are to be used for moderately exuding wounds. Based on the results in this test, a more compact structure is needed to further reduce the WVTR, which means that a further decrease of the alginate concentration, calcium concentration, or needle size and an increase of the air pressure will be needed. These changes can create smaller fibers to increase points of contact between fibers and can enhance adhesion between contacting fibers to create a compact structure.
Tetracycline HCl (TCH) release and bacterial inhibition potential
Wound dressings need to be able to absorb and release antimicrobial drugs so they can prevent infection during skin repair. Sample A had the highest percentage of alginate polymer and hence the highest amount of hydrogel to absorb the TCH molecules. As a result, it could absorb and release more TCH than other samples (p < 0.05) (). Samples D, P and Ca had larger fibers than the control (). Samples with large fibers absorbed TCH less efficiently than those with small fibers because they had less specific surface area to absorb TCH. As a consequence, samples D, P and Ca absorbed and released less TCH than the control (p < 0.05). Samples D, P and Ca absorbed and released similar amounts of TCH during the course of the test (p > 0.05). It seemed that the TCH release percentages of the samples corresponded with their swelling ratios. Samples P and Ca had the highest and lowest swelling ratios of all and they also had the highest and lowest parentages of TCH release, respectively, at 6 h. Samples with more loose fibrous structures swelled up faster (section 2.3) and as a consequence released the absorbed TCH more quickly.
Figure 6. The cumulative TCH release of different samples over 6 h in PBS. Data are presented as the mean ± SD (n = 6). The total amount of TCH released within 48 h was the amount of TCH loaded on the sample. The percentages of TCH released after 6 h is shown on the figure. The cumulative amounts of TCH released from sample A at 6 h and 48 h were higher than those of the control, while those of samples P, D and Ca were lower (p < 0.05).
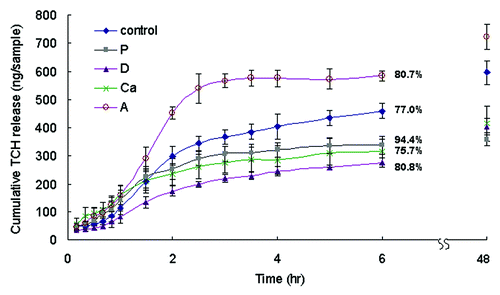
Alginate itself and what it might release did not inhibit the growth of E. coli (). Only the TCH released from the samples did inhibit the growth of E. coli and form an inhibition zone. Sample A had the largest inhibition zone of all of the samples (p < 0.05) (), most likely due to the highest amount of TCH it released. Sample P had a larger inhibition zone than the control and Ca had a smaller one (both p < 0.05). The inhibition zone of sample D was similar to that of the control (p > 0.05). There did not seem to be a strong correlation between the amount of TCH loaded, their release pattern, and the size of inhibition zone. However, the most important issue in wound care is not the delivery mechanism but rather finding medications that can be topically absorbed, which provides desirable therapeutic effects within wounds.Citation4
Materials and Methods
Sample preparation
Alginic acid monosodium salt was extracted from brown seaweed with a ratio of mannuronic acid to guluronic acid (M/G ratio) of 1.56 and a molecular weight of 100,000 Da (Sigma). The viscosity of the 2% alginate aqueous solution at 25°C was 150 cP (ARES Rheometer, Rheometric Scientific). Alginic acid monosodium salt was dissolved in deionized water, and the solution was loaded into a plastic syringe, which was immobilized onto a robotic arm and kept at room temperature. Air pressure was applied to the syringe through a pneumatic regulator (8000D, Ganbow) to expel the alginate solution through a needle into a container filled with calcium chloride solution in which the gelation process of the alginate fibers occurred. For the tensile and water vapor transmission rate tests, 35 mL of alginate solution was extruded into a plastic container (5.5 cm × 3.5 cm × 5 cm) filled with calcium chloride solution. For the rest of the tests, 1 mL of alginate solution was extruded into each well of a 24-well cell culture plate that had 2 mL of calcium chloride solution in each well.
The air pressure, the diameter of the needle, the concentration of the alginate solution and the concentration of the calcium chloride solution that were used are listed in . The choice of the parameters used to make the control sample was based on the report by Khalil and Sun.Citation26 They reported the correlations between different operating parameters in a three-dimensional plotting system with 0.1–4 w/v% high viscosity sodium alginate aqueous solution (M.W. = 150,000 Da). They utilized nozzles with sizes ranging from 100 μm to 410 μm and concluded that their model worked best with 3% alginate and 5% calcium chloride. Based on their work and by trial and error, the parameters that were chosen to make the control sample were: air pressure = 6 bar, needle diameter = 150 μm, calcium chloride concentration = 5 w/v% and alginate concentration = 1.5 w/v%. To study the influence of air pressure on the scaffold properties, sample P was manufactured by decreasing the air pressure to 3 bar and was compared with the control. Likewise, the needle diameter was increased to 200 μm to make sample D, the calcium chloride concentration was decreased to 0.5 w/v% to make sample Ca, and the alginate concentration was increased to 3 w/v% to make sample A. The samples were dehydrated using sequential 30%, 50%, 70% and 99.5% ethanol solutions and air-dried. The dry samples were then stored in a desiccator for later testing.
Table 1. The different operating parameters of the wet-spinning system and a qualitative assessment of the appearances of the final products
Sample morphology
The dry samples were sputter-coated with gold (E1010, Ion Sputter) before being examined with scanning electron microscopy (SEM) (S-3000H). The fiber size was estimated from the scale bars on the SEM micrographs. The diameters of 15 fibers were averaged for each sample (n = 15).
Tensile modulus
The dry samples were rehydrated in phosphate-buffered saline (PBS, pH = 7.4) for 30 min before testing. The samples (~5 cm × 3 cm × 0.2 cm) were fixed between the two clamps (with gauge length = 2 cm) of a tensile tester (H5KS, Tinius Olsen). A 25 N load cell was used with the crosshead moving at 10 mm/min.Citation27 The elastic modulus [kPa/(mm/mm)] of the samples (n = 6) was obtained according to ASTM standard D 638–10.Citation28
Swelling
The dried samples (initial weights of W0) were placed in a beaker filled with 100 mL of PBS with Ca2+ and Mg2+ (pH = 7.4) (n = 6). At 0.1, 0.2, 0.4, 1, 2, 3, 4, 5, 10, 20, 40, 60 and 90 min, the samples were taken out of the beaker. The excess water in the samples was removed by gentle blotting with tissue paper (Kimwipes, Kimberly-Clark). The final weights of the samples (Wt) were measured, and the swelling ratios were calculated as [(Wt - W0)/ W0] × 100%.
Drug release efficacy
The model drug used in this test was tetracycline HCl (TCH) (Alfa Aesar). The wet samples were punched into small discs (diameter = 10 mm, thickness ~ 2 mm) and dehydrated using the procedures described in Materials and Methods. The dry samples were submerged in a TCH solution (10 mg/mL) for 24 h (n = 6). The excess liquid in the pores and on the surfaces of the samples was removed using gentle centrifugation before being placed in beakers filled with 100 mL of PBS with Ca2+ and Mg2+ (pH = 7.4). The six beakers were placed on a shaker moving at 50 rpm. At different time points (for 6 h), 100 μL of the PBS solution was removed from the beakers, and the same amount of fresh PBS was replenished. The absorbance of TCH in the sample solution was read at 361 nm using a spectrophotometer (Gene Quant 1200, GE Healthcare Life Science). The total TCH absorbance at each time point was converted to its concentration Ct (ng/mL) using a standard curve, and the amount of TCH released at that time (Wt) in the beakers was obtained [Wt (ng) = Ct × 100 mL]. The samples were left in the beakers for 48 h, allowing the release of all of the absorbed TCH. The total amount of TCH released after 48 h (Wf) was calculated as Wf = Cf × 100 mL, where Cf was the final concentration of TCH in the beakers at 48 h. The percentage of TCH that was released at 6 h was calculated as Wt/Wf × 100%.
Water vapor transmission rate
The moisture permeability of the hydrogel was determined by measuring the water vapor transmission rate (WVTR) across the material, as stipulated by the ASTM standard E 96/E 96 M – 05.Citation29 A glass tube (ID = 2 cm, h = 1.6 cm) was filled with 4 g of deionized water (approximately 3/4 of the tube volume). Each wet sample (~5 cm × 3 cm × 0.2 cm) was dehydrated following the procedure described in Section 2.1. The dry samples were affixed to the top of the tubes with a sealant to prevent vapor leakage from the periphery of the tube opening. The tubes (n = 6) were incubated at 37°C and 50% relative humidity in an oven. After 48 h, the weight of the water remaining in the tube was measured [W2day (g)]. WVTR was calculated as (4-W2day)/area of dressing coverage/2 (g/m2/d).
Antibacterial potential
The Kirby–Bauer disc diffusion method was used to assess the antibacterial activity of the tetracycline HCl (TCH, Sigma)-loaded samples.Citation19,Citation20 The antibacterial performance of the samples was tested against Gram-negative Escherichia coli (E. coli, DH 5-α, Bethesda Research Lab.) (n = 8). The samples without TCH were used as controls. The wet samples were punched into discs (D = 10 mm, thickness ~ 2 mm) and dehydrated using the procedure described earlier. The dry samples were placed in a TCH solution (15 mg/mL) for 12 h to absorb TCH. One-hundred microliters of E. coli (108 cells/mL) was uniformly seeded onto Lysogeny broth agar (Difco) in a Petri dish, and the samples containing TCH were then placed on the agar and incubated at 37°C for 24 h. The diameters of the clear circles were measured (Diz), and the inhibition zones were calculated as [(Diz-10)/2] (mm).
Statistical methods
The data are shown as the mean ± standard deviation (SD). Statistical analysis was performed using SigmaStat 3.5 (Systat). The differences between the wetspun samples made by different parameters were compared using one-way analysis of variance. Significance was declared at p < 0.05.
Conclusions
By manipulating the operating parameters in the wet-spinning system, wound dressings with different properties were successfully made. The results show that the operating parameters of the wet-spinning system had a significant impact on the size of the fibers and the fibrous structure. The changes in fiber size and structure significantly influenced the performance of the dressings, i.e., the tensile modulus, swelling, WVTR, drug release, and bacterial inhibition potential. This study is the first to study the effects of the manufacturing parameters of wet spinning on the structure of an alginate fiber matrix and relate the changes in the structure to the matrix’s performances as a wound dressing.
Disclosure of Potential Conflicts of Interest
No potential conflicts of interest were disclosed.
References
- Balakrishnan B, Mohanty M, Umashankar PR, Jayakrishnan A. Evaluation of an in situ forming hydrogel wound dressing based on oxidized alginate and gelatin. Biomaterials 2005; 26:6335 - 42; http://dx.doi.org/10.1016/j.biomaterials.2005.04.012; PMID: 15919113
- Rodrigues AP, Sanchez EMS, da Costa AC, Moraes AM. The influence of preparation conditions on the characteristics of chitosan-alginate dressings for skin lesions. J Appl Polym Sci 2008; 109:2703 - 10; http://dx.doi.org/10.1002/app.28203
- Thomas Adisawm S. J. Wound Care 9 (2000) 56–60. Alginate dressings in surgery and wound management. J Wound Care 2000; 9:56 - 60; PMID: 11933281
- Eisenbud D, Hunter H, Kessler L, Zulkowski K. Hydrogel Wound Dressings: Where Do We Stand in 2003?. Ostomy Wound Manage 2003; 49:52 - 7; PMID: 14652421
- S. Thomas IML. Wound dressings. Oxford: Oxford University Press, 1998.
- Thomas S. A structured approach to the selection of dressings. http://wwwworldwidewoundscom/1997/july/Thomas-Guide/Dress-Selecthtml Online 1997 July.
- Pudner R. Amorphous hydrogel dressings in wound management. J Community Nurs 2001; 15:43 - 6
- Vanscheidt W. Autolytic debridement of chronic wounds by means of alginate containing hydrogel. Vasomed 1997; 9:26 - 34
- Motta GJ. Calcium alginate topical wound dressings: a new dimension in the cost-effective treatment for exudating dermal wounds and pressure sores. Ostomy Wound Manage 1989; 25:52 - 6; PMID: 2610818
- Thomas A, Harding KG, Moore K. Alginates from wound dressings activate human macrophages to secrete tumour necrosis factor-alpha. Biomaterials 2000; 21:1797 - 802; http://dx.doi.org/10.1016/S0142-9612(00)00072-7; PMID: 10905462
- Wang LH, Khor E, Wee A, Lim LY. Chitosan-alginate PEC membrane as a wound dressing: Assessment of incisional wound healing. J Biomed Mater Res 2002; 63:610 - 8; http://dx.doi.org/10.1002/jbm.10382; PMID: 12209908
- Gates JL, Holloway GA. A comparison of wound environments. Ostomy Wound Manage 1992; 38:34 - 7; PMID: 1482522
- V. Jandera DAH. P.M. deWet, P.M. Innes, H. Rode. Cooling the burn wound: evaluation of different modalities. Burns 2000; 26:265 - 70; http://dx.doi.org/10.1016/S0305-4179(99)00133-3; PMID: 10741593
- Al-Ahmad A, Wiedmann-Al-Ahmad M, Carvalho C, Lang M, Follo M, Braun G, et al. Bacterial and Candida albicans adhesion on rapid prototyping-produced 3D-scaffolds manufactured as bone replacement materials. J Biomed Mater Res A 2008; 87:933 - 43; http://dx.doi.org/10.1002/jbm.a.31832; PMID: 18228269
- Armstrong SH, Ruckley CV. Use of a fibrous dressing in exuding leg ulcers. J Wound Care 1997; 6:322 - 4; PMID: 9325826
- Miraftab M, Qiao Q, Kennedy JF, Anand SC, Groocock MR. Fibres for wound dressings based on mixed carbohydrate polymer fibres. Carbohydr Polym 2003; 53:225 - 31; http://dx.doi.org/10.1016/S0144-8617(03)00108-5
- Tuzlakoglu K, Alves CM, Mano JF, Reis RL. Production and characterization of chitosan fibers and 3-D fiber mesh scaffolds for tissue engineering applications. Macromol Biosci 2004; 4:811 - 9; http://dx.doi.org/10.1002/mabi.200300100; PMID: 15468275
- Qin YM. The gel swelling properties of alginate fibers and their applications in wound management. Polym Adv Technol 2008; 19:6 - 14; http://dx.doi.org/10.1002/pat.960
- Deng CM, He LZ, Zhao M, Yang D, Liu Y. Biological properties of the chitosan-gelatin sponge wound dressing. Carbohydr Polym 2007; 69:583 - 9; http://dx.doi.org/10.1016/j.carbpol.2007.01.014
- Guan M, Ren L, Wu T, Sun LP, Li LR, Zhang QQ. Potential wound dressing with improved antimicrobial property. J Appl Polym Sci 2007; 105:1679 - 86; http://dx.doi.org/10.1002/app.26291
- English A, Azeem A, Gaspar DA, Keane K, Kumar P, Keeney M, et al. Preferential cell response to anisotropic electro-spun fibrous scaffolds under tension-free conditions. J Mater Sci Mater Med 2012; 23:137 - 48; http://dx.doi.org/10.1007/s10856-011-4471-8; PMID: 22105221
- Körstgens V, Flemming HC, Wingender J, Borchard W. Influence of calcium ions on the mechanical properties of a model biofilm of mucoid Pseudomonas aeruginosa. Water Sci Technol 2001; 43:49 - 57; PMID: 11381972
- Wan LQ, Jiang J, Arnold DE, Guo XE, Lu HH, Mow VC. Calcium Concentration Effects on the Mechanical and Biochemical Properties of Chondrocyte-Alginate Constructs. Cell Mol Bioeng 2008; 1:93 - 102; http://dx.doi.org/10.1007/s12195-008-0014-x; PMID: 19890444
- Chiu CT, Lee JS, Chu CS, Chang YP, Wang YJ. Development of two alginate-based wound dressings. J Mater Sci Mater Med 2008; 19:2503 - 13; http://dx.doi.org/10.1007/s10856-008-3389-2; PMID: 18266085
- Ruiz-Cardona L, Sanzgiri YD, Benedetti LM, Stella VJ, Topp EM, RuizCardona L. Application of benzyl hyaluronate membranes as potential wound dressings: evaluation of water vapour and gas permeabilities. Biomaterials 1996; 17:1639 - 43; http://dx.doi.org/10.1016/0142-9612(95)00324-X; PMID: 8842370
- Khalil S, Sun W. Biopolyrner deposition for freefonn fabrication of hydrogel tissue constructs. Mat Sci Eng C-Bio S 2007; 27:469 - 78; http://dx.doi.org/10.1016/j.msec.2006.05.023
- Yunoki S, Mori K, Suzuki T, Nagai N, Munekata M. Novel elastic material from collagen for tissue engineering. J Mater Sci Mater Med 2007; 18:1369 - 75; http://dx.doi.org/10.1007/s10856-007-0121-6; PMID: 17277984
- ASTM Standard D 638-10. Standard Test Method for Tensile Properties of Plastics West Conshohocken, PA: ASTM International, 2010.
- ASTM E 96/E 96M–05. Standard Test Methods for Water Vapor Transmission of Materials. West Conshohocken, PA: ASTM International, 2005.