Abstract
Therapeutic monoclonal antibodies have revolutionized the treatment of cancer and other diseases. However, several limitations of antibody-based treatments, such as the cost of therapy and the achievement of sustained plasma levels, should be still addressed for their widespread use as therapeutics. The use of cell and gene transfer methods offers additional benefits by producing a continuous release of the antibody with syngenic glycosylation patterns, which makes the antibody potentially less immunogenic. In vivo secretion of therapeutic antibodies by viral vector delivery or ex vivo gene modified long-lived autologous or allogeneic human mesenchymal stem cells may advantageously replace repeated injection of clinical-grade antibodies. Gene-modified autologous mesenchymal stem cells can be delivered subcutaneously embedded in a non-immunogenic synthetic extracellular matrix-based scaffold that guarantees the survival of the cell inoculum. The scaffold would keep cells at the implantation site, with the therapeutic protein acting at distance (immunotherapeutic organoid), and could be retrieved once the therapeutic effect is fulfilled. In the present review we highlight the practical importance of living cell factories for in vivo secretion of recombinant antibodies.
Introduction
Monoclonal antibodies (mAbs) have revolutionized the field of biology and medicine since their first description in 1975.Citation1 However, the development of therapeutic monoclonal antibodies has been complicated by a number of technical challenges including the appearance of immunogenic responses against murine antibody domains, and their inability to trigger human effector functions.Citation2 These drawbacks were overcome initially by the generation of chimeric and humanized antibodies and now can be completely avoided by using fully human antibodies.Citation2
However, several limitations hamper native mAb-based treatments, such as low tumor-to-blood ratio, due to long serum half-life and limited tissue penetration, and the need for high doses over a long period of time. There is a wide range of different recombinant antibodies fragments with differences in molecular weight, valence, specificity and format. Thus, half-life and tumor penetration can be fine-tuned by adjusting these parameters.Citation3 There remain, however, at least two major concerns: the extremely high cost of therapy and the achievement of sustained plasma levels, since the recommended dosage and administration involve repeated bolus injections, and fluctuating plasma concentrations ranging from subtoxic to subtherapeutic.
In Vivo Secretion of Therapeutic Antibodies
Gene therapy has the potential to overcome some of the shortcomings associated with conventional bolus protein therapy by producing a sustained release of the antibody with syngenic glycosylation patterns, that makes the antibody potentially less immunogenic and better tolerated.Citation4 Two main approaches to gene therapy include in vivo and ex vivo gene transfer methods (). In vivo gene therapy implies direct injection of genetic material into the human body, usually by using viral vectors. Ex vivo gene therapy involves modifying target cells, prior to implanting these into the tissues of the living body.
In Vivo Secretion of Full-Length mAbs
Pioneering work by Noel et al.Citation5 demonstrated that several types of non-lymphoid cells have the ability to secrete full-length IgG antibodies in vitro after retroviral gene transfer. Furthermore, implantation of ex vivo retrovirally-modified myoblasts resulted in detectable mAb serum levels (~1–3 μg/ml) for long periods of time. Four years later, the same group demonstrated that in vivo administration of high doses of a recombinant adenovirus encoding the same antibody gene resulted in a 100- to 200-fold increase in mAb serum levels (~200 μg/ml). However, adenoviral vectors are highly immunogenic and trigger an innate immune response that reduces therapeutic effect and causes inflammation-related side effectsCitation6,Citation7 On the other hand, adeno-associated virus (rAAV) is a weak innate immunogen and it does not elicit the immune response observed for adenoviral vectors, although both type of viral vectors share the drawback of prevalence of neutralizing antibodies in the human population.Citation8 Using this expression system, Fang et al.Citation9 generated a rAAV serotype 8 encoding a full-length VEGFR-2 neutralizing mAb (DC101). The mAb is expressed from a single open reading frame by linking the heavy and light chains with a self-processing peptide 2A derived from the foot-and-mouth disease virus. A furin cleavage site was introduced to remove 2A-derived residues. A single dose of rAAV8-DC101 resulted in long-term expression of high-levels (> 1,000 μg/ml) of mAb, demonstrating significant anti-tumor efficacy. Watanabe et al.Citation10 reported that adenoviral vectors and rAAV encoding a full-length anti-VEGF mAb equivalent to bevacizumab (Avastin®) effectively suppresses the growth of human tumors.
Sustained high serum levels of a full-length anti-HER2 (also referred to as HER2/neu or ErbB-2) mAb have also been reported after intramuscular administration of a rAAV vector incorporating the furin/2A technology for monocistronic expression of both heavy and light chains. This strategy achieved significant tumor growth inhibition when rAAV was administered prior to tumor challenge, and demonstrated antitumor efficacy against pre-established tumors when AAV was administered up to 20 d after tumor challenge.Citation11 Also, long-term therapeutic levels of an anti-HER2 mAb have been documented after a single intravenous injection of an AAV vector based on the non-human primate AAV serotype rh.10 containing the furin/2A expression system, which reduced the growth of HER2 positive tumors and increased the survival of tumor-bearing mice.Citation12
A different strategy for cancer therapy used a systemically administered bidirectional lentiviral vector for the in vivo secretion of a full-length anti-Met mAb. This approach resulted in substantial inhibition of tumor growth.Citation13 Recently, Balazs et al.Citation14 showed that a single intramuscular injection in mice of a specialized AAV vector containing a self-processing 2A sequence induces lifelong expression of high concentrations of a HIV neutralizing full-length mAb (b12), and it is possible to reach sustained protection against HIV infection.
In Vivo Secretion of Novel Recombinant Antibody Formats
In an attempt to improve tumor penetration, recombinant antibodies with modified properties have been generated. Novel antibody formats, such as the single-chain antibody (scFv), exhibit better pharmacokinetics than intact IgG.Citation3 However, scFv antibodies exhibit rapid blood clearance and poor retention times on the target owing to small sizes and monovalent binding properties, which results in the necessity of frequent delivery of such fragments.Citation3 To circumvent these limitations, several gene therapy approaches have been developed to express antibody fragments in vivo.
In 2002, Arafat et al.Citation15 demonstrated for the first time the therapeutic effect of a scFv secreted by eukaryotic cells Effective concentrations of scFv were achieved following in vivo administration of an adenoviral vector expressing an anti-erbB2 scFv. Furthermore, in vivo gene transfer via the anti-erbB2 scFv encoding adenovirus resulted in substantial inhibition of tumor growth. A few months later Sanz et al. demonstrated that in vivo secretion of the L36 scFv,Citation16 that recognizes an angiogenesis-associated laminin epitope,Citation17 inhibited tumor growth in vivo.Citation18 In 2006, the same group reported that genetically modified human cells efficiently secreted trivalent and hexavalent antibodies, based on fusion of L36 scFv to different portions of the C-terminal noncollagenous domain of collagen XVIII.Citation19 In vivo secretion of the multivalent L36 antibodies was more effective in preventing tumor growth than the monomeric scFv counterpart.Citation19
Afanasieva et al.Citation20 demonstrated that a single systemic administration of recombinant adenovirus encoding an anti-VEGF scFv (V65 scFv) or bivalent derivatives (minibody and scFv-Fc) resulted in tumor inhibition and had a therapeutic effect equivalent to that of multiple injections of high amounts of purified V65 scFv. Systemic administration of an adenoviral vector has also been used to deliver in vivo an immunotoxin comprising an anti-HER2 scFv as targeting moiety.Citation21
Bispecific antibodies (bsAbs) represent promising approaches to more efficacious antitumor therapy.Citation22 BsAbs targeting tumor-associated antigens (TAA) and effector cell trigger molecules have been generated and shown to redirect cellular cytotoxicity toward target cells.Citation2,Citation23,Citation24 The potential of T-cell activating anti-TAA x anti-CD3 bsAbs in cancer therapy has been demonstrated in a variety of in vitro and in vivo models and several bsAbs have been tested in clinical trials.Citation22 In fact, an anti-EpCAM x anti-CD3 full-length IgG (catumaxomab) has been approved for intraperitoneal treatment of malignant ascites.Citation25,Citation26 However, maximum tolerated dose is low due to the toxicity caused by induction of ‘cytokine storm’, a consequence of cross-linking of T cells with accessory cells bearing Fc receptors, followed by cytokine release-related symptoms.
For these reasons, it is highly recommended using the use of recombinant Fc-lacking bsAbs such as tandem scFv [(scFv)2] and diabodies. Numerous studies have demonstrated the potency of these formats in preclinical and clinical studies.Citation27,Citation28 However, these recombinant bsAbs present a very short serum half-life and must be administered by continuous infusion using portable minipumps.
In 2003, Blanco et al.Citation29demonstrated for the first time therapeutic effect of a recombinant Fc-lacking anti-carcinoembryonic antigen (CEA) x anti-CD3 two-chain diabody secreted by mammalian cells. They generated a bicistronic vector that enable the secretion of functionally active diabody by gene-modified human cells, and promoted unstimulated human primary T cells to proliferate and kill CEA-expressing cancer cells. Importantly, locally produced diabody showed significant cytotoxic activity in vivo against established tumors.Citation29 Four years later, the same group generated a bicistronic lentiviral vector and demonstrated that primary human lymphocytes can be efficiently transduced to secrete high levels of functional anti-CEA × anti-CD3 diabody. Importantly gene-modified lymphocytes significantly reduced in vivo tumor growth rates in xenograft studies.Citation30
Ex Vivo Gene-Modified Cells as Factories for Long-Term In Vivo Secretion of Therapeutic Proteins
The application of ex vivo gene-modified human cells for in vivo secretion of therapeutic proteins offers several advantages over viral vector-mediated in vivo gene therapy. Viral vectors are highly efficient as gene delivery vehicles, but raise concerns about safety and limitation of the effect due to immune response. The use of ex vivo gene-modified cells as “factories or biological pumps” eliminates the risk of non-specific diffusion, allows in vitro quantification of protein secretion, and offers the possibility of selection for high-level expression clones prior to administration. Furthermore, cells can be retrieved if administered in certain formats, and/or armed with a “suicide” gene (e.g., HSV thymidine kinase), which can be activated to ensure the destruction of the cell inoculum.
Terminally differentiated mature cells possess a short lifespan, and this implies an obvious limitation to their application in cell-based gene therapy strategies for cancer immunotherapy. In contrast, stem/progenitor cells have greater expansion capacity and constitute a more appropriate cellular source. Since the advent of gene therapy, hematopoietic stem cells (HSC) have been a delivery cell of choice. However, the ex vivo expansion of adult HSC is expensive and time-consuming, and they are difficult to transduce. On the contrary, mesenchymal stem cells (MSC) can be found in virtually all postnatal tissues, are easily transduced and exhibit a unique in vitro expansion capacity using a simple medium formulation.
Local or Systemic Injection of Ex Vivo Gene-Modified MSC for Cancer Immunotherapy
MSC suspended in PBS or solution saline, have been administered in preclinical models of cancer through a wide variety of routes (), in the proximity or not of the tumor. Although MSC are supposed to be endowed with tumor-targeting properties, most studies showing therapeutic effect of gene-modified MSC use one of these two strategies: either coinjection or intratumoral/peritumoral injection for localized tumors, or intravenous administration for lung metastasis, taking advantage of the fact that most of the intravenous-inoculated MSC (especially human MSC) are physically retained in the mouse pulmonary filter as a consequence of their size.Citation31,Citation32
Table 1. Ex vivo gene-modified mesenchymal stem cells for cancer immunotherapy
In fact, Bexell et al.Citation33 found no evidence of MSC homing to intracranial gliomas following intravenous injections and suggested that MSC should be administered by intratumoral implantation to achieve a therapeutic effect. In a work by a different group, MSC genetically modified for the expression of TNFSF10/TRAIL (MSCTRAIL) inhibited colon carcinoma tumor growth after subcutaneous coinjection, but systemic application of MSCTRAIL had no effect, which appeared to be due to lung entrapment and low rate of tumor grafting.Citation34
Intratumoral/peritumoral MSC have been validated for the delivery of CX3CL1, IFN-β, IL-2, IL-7, IL-12, IL-18, TNF α and TRAIL to subcutaneous or intracranial tumors. Using a different experimental approach, Studeny et al.Citation35 reported that intravenous-injected, IFN-β expressing MSC (MSCIFNβ) significantly extended animal survival in mice with human melanoma lung metastasis. These results were supported by Ren et al.,Citation36 who demonstrated the therapeutic effect of systemically administered murine MSCIFNβ in a mouse model for prostate cancer metastasis.
A therapeutic effect was also reported by Chen et al.,Citation37 who injected different tumor cells into the footpad of syngenic mice and after intravenous administration of MSCIL-12 observed inhibition of tumor growth and spontaneous metastasis. MSC could be detected into the tumors five weeks after administration but interestingly they were absent from normal tissues such as lung and liver. As an alternative route of systemic delivery, MSCIL-12 were administered intraperitoneally before intravenous inoculation of melanoma cell.Citation33 Treatment led to a considerable decrease in the number of lung metastasis, but unfortunately no data about potential MSC homing or increased IL-12 serum levels were reported. Recently, it was shown that adipose-derived MSC expressing TRAIL, intratumoral- or intravenous-administered in mice bearing subcutaneous tumors, caused a reduction in tumor burden.Citation38 Presence of MSCTRAIL in tumors after intravenous administration was demonstrated, but no data on potential localization of MSC in normal tissues were provided. In a different setting, MSCLIGHT exhibited a notable prophylactic and therapeutic effect when administered subcutaneous in the flank contralateral to tumors. However, migration of MSC from the left flank to the right one where tumor cells were inoculated is not addressed in this work.Citation39
Considering that it is difficult to estimate the percentage of MSC effectively homing to tumors in these models (probably very low), and given the antitumoral effect observed in most of them, these results could be attributed, at least in part, to therapeutic protein production in locations other than tumors. Therefore, perhaps MSC as cell factories for soluble proteins can be “outsourced” from the tumor while preserving their therapeutic effect.
If MSC tumor homing is not a requirement, perhaps we could implant them in a determined location, within a controlled environment providing clues that could enhance their engraftment and survival. Moreover, some MSC properties (e.g., immunosupresion, metastasis and angiogenesis promotion) would strongly recommend avoiding the direct contact between MSC and tumor cells.Citation40,Citation41
Confined Administration of Ex Vivo Gene-Modified MSC for Cancer Immunotherapy
In fact, for strategies where MSC are used as cell factories for therapeutic antibodies, ex vivo gene-modified producer cells can be confined within a controlled environment providing clues that could enhance their engraftment and survival. The scaffold would keep cells at the implantation site, with the therapeutic protein acting at distance (immunotherapeutic organoid), and could be retrieved once the therapeutic effect is fulfilled (). A seminal work by Eliopoulos et al. reported that transduced MSC secreting EPO (MSCEPO), when delivered freely in the subcutaneous or intraperitoneal spaces led to a temporary hematocrit increase.Citation42 In contrast, subcutaneous implantation of MSCEPO embedded in Matrigel led to a sustained pharmacological effect. This systemic effect of locally produced proteins (IL-2, IL-12, PEX, sIGF-IR and TRAIL) has also been reported in the context of cancer therapy (). For example, Wang et al.Citation43 reported that Matrigel-embedded sIGF-IR-secreting MSC subcutaneous implanted, provided sustained delivery of this decoy receptor in vivo. The protein achieved therapeutically effective concentrations, resulting in marked reductions in the ability of three different highly metastatic tumor cell types to colonize the liver.
Figure 2. Ex vivo generation of genetically modified mesenchymal stem cells (MSC) as factories for long-term in vivo secretion of immunotherapeutic proteins. (A) Ex vivo gene therapy of autologous or allogeneic MSC (isolation, expansion and lentiviral transduction) to generate an immunotherapeutic cell vehicle (ICV). (B) The autologous ICV is embedded in a non-immunogenic synthetic extracellular matrix and implanted by subcutaneous injection (immunotherapeutic organoids). The allogenic ICV is microencapsulated and implanted by subcutaneous injection (immunotherapeutic microcapsules).
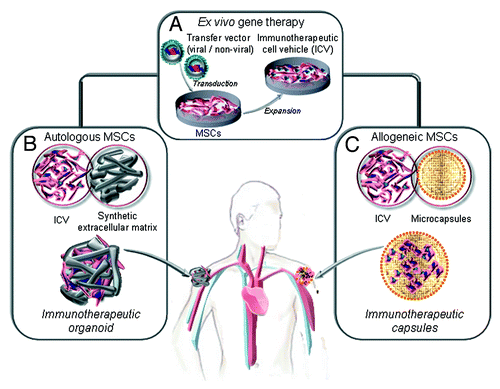
Matrigel is a reconstituted extracellular matrix (ECM) preparation extracted from a murine sarcoma cell line, not suitable for MSC immobilization in a clinical setting. For this reason we used a chemically defined and non-immunogenic synthetic ECM (sECM) for in vivo MSC engraftment in a bsAb-based cancer immunotherapy approach. MSC were transduced ex vivo with a lentiviral vector expressing a recombinant anti-CEA x anti-CD3 diabody, embedded in a sECM crosslinkable in situ (Extracel-X) and inoculated in the ventral subcutaneous space of nude mice. The antibody was released into the bloodstream at detectable levels for at least 7 weeks. MSC-secreted diabody activated tumor-specific T cells and reduced the growth of CEA-expressing human colon carcinoma cells.Citation40
In previous works, two immunotherapeutic MSC-based organoids had been reported for the expression of IL-2 and IL-12.Citation74,Citation75 Matrix-embedded IL-2-producing MSC inoculated in the vicinity of B16 melanoma led to inhibition of tumor growth.Citation74 Similarly, gene-modified MSC to express IL-12, embedded in a matrix, and implanted peritumorally in a model of breast cancer caused a significant decrease of tumor growth.Citation75 Although MSCIL-12 scaffolds determined increased IL-12 plasma levels, the observed therapeutic benefit was not due to a systemic effect, since MSCIL-12 implanted contralaterally did not inhibit significantly tumor growth. In contrast, MSC-secreted diabody demonstrated the systemic effect of a locally produced protein.Citation40
In summary, the necessary components for generating a long-lasting immunotherapeutic organoid () are: (1) gene/s encoding a soluble protein/s with immune-modulating activity, (2) an efficient and safe transfer vector, (3) a suitable an easily transduced long-lived cell and (4) a non-immunogenic synthetic matrix scaffold that guarantees the survival of the cell vehicle at the point of implantation.
Future Prospect
AAV have demonstrated excellent safety and tolerability in human trials and two phase 1 trials currently ongoing involve the use of AAV for the expression of anti-HIV antibodies from muscle tissues.Citation79 However, the possibility of rare adverse events is still a concern. On the other hand, cell-based approaches face challenges such as loss of transgene expression over time due to limited life-span of producer cells or host immune responses against them. MSC are emerging as the best cell choice for the generation of long-lasting cell factories (). Perhaps the safest and most practical approach might be the use of scaffolds that keep genetically modified autologous MSC at the implantation site (immunotherapeutic organoids). However, in terms of cost-effectiveness, the only potential approach to apply these cell factories to the clinical setting would imply the use of “off-the-shelf” stocks of gene-modified MSC ready to be used in a series of patients. In principle, MSC would be perfect candidates for this strategy due to low immunogenicity and immunomodulatory properties, but some reports point out that allogenic MSC are not so “invisible” to immunocompetent hosts.Citation43,Citation44 Therefore, the use of encapsulation systems () to shield producer MSC from the host immune system (immunotherapeutic capsules) would be highly desirable in order to obtain long-term systemic protein delivery. In a recent work, Goren et al.Citation77 demonstrated the feasibility of this approach for the in vivo production of PEX, an inhibitor of angiogenesis, by encapsulated MSC.Citation77 The technical and biological advances may lead to the realization of the full potential of cell encapsulation.Citation80
In summary, the transfer of genes encoding antibodies, both in vivo and ex vivo, is a promising strategy that can be applied to treat clinical conditions in which continuous production of antibodies is required. Administration of ex vivo gene-modified cells embedded in appropriate scaffolds, such as hydrogels, can help to improve their therapeutic potential.
Abbreviations: | ||
AAV | = | adeno-associated virus |
bsAb | = | bispecific antibody |
CEA | = | carcinoembryonic antigen |
ECM | = | extracellular matrix |
HSC | = | hematopoietic stem cells |
mAbs | = | monoclonal antibodies |
MSC | = | mesenchymal stem cells |
scFv | = | single-chain antibody fragment |
Acknowledgments
This study was supported by grants from the Ministerio de Ciencia e Innovación (BIO2011-22738 and PSE-01000-2009-11), the Comunidad de Madrid (S2010/BMD-2312), and the European Union (SUDOE-FEDER. IMMUNONET-SOE1/P1/E014) to L.A-V; and from the Fondo de Investigación Sanitaria/ Instituto de Salud Carlos III (PI08/90856 and PS09/00227) and Fundación Investigación Biomédica Hospital Puerta de Hierro to L.S.
Disclosure of Potential Conflicts of Interest
No potential conflicts of interest were disclosed.
References
- Köhler G, Milstein C. Continuous cultures of fused cells secreting antibody of predefined specificity. Nature 1975; 256:495 - 7; http://dx.doi.org/10.1038/256495a0; PMID: 1172191
- Sanz L, Cuesta AM, Compte M, Álvarez-Vallina L. Antibody engineering: facing new challenges in cancer therapy. Acta Pharmacol Sin 2005; 26:641 - 8; http://dx.doi.org/10.1111/j.1745-7254.2005.00135.x; PMID: 15916728
- Cuesta AM, Sainz-Pastor N, Bonet J, Oliva B, Álvarez-Vallina L. Multivalent antibodies: when design surpasses evolution. Trends Biotechnol 2010; 28:355 - 62; http://dx.doi.org/10.1016/j.tibtech.2010.03.007; PMID: 20447706
- Sanz L, Blanco B, Álvarez-Vallina L. Antibodies and gene therapy: teaching old ‘magic bullets’ new tricks. Trends Immunol 2004; 25:85 - 91; http://dx.doi.org/10.1016/j.it.2003.12.001; PMID: 15102367
- Noël D, Pelegrin M, Marin M, Biard-Piechaczyk M, Ourlin JC, Mani JC, et al. In vitro and in vivo secretion of cloned antibodies by genetically modified myogenic cells. Hum Gene Ther 1997; 8:1219 - 29; http://dx.doi.org/10.1089/hum.1997.8.10-1219; PMID: 9215739
- Noël D, Pelegrin M, Kramer S, Jacquet C, Skander N, Piechaczyk M. High in vivo production of a model monoclonal antibody on adenoviral gene transfer. Hum Gene Ther 2002; 13:1483 - 93; http://dx.doi.org/10.1089/10430340260185111; PMID: 12215269
- Jooss K, Chirmule N. Immunity to adenovirus and adeno-associated viral vectors: implications for gene therapy. Gene Ther 2003; 10:955 - 63; http://dx.doi.org/10.1038/sj.gt.3302037; PMID: 12756416
- Xiao PJ, Lentz TB, Samulski RJ. Recombinant adeno-associated virus: clinical application and development as a gene-therapy vector. Ther Deliv 2012; 3:835 - 56; http://dx.doi.org/10.4155/tde.12.63; PMID: 22900466
- Fang J, Qian JJ, Yi S, Harding TC, Tu GH, VanRoey M, et al. Stable antibody expression at therapeutic levels using the 2A peptide. Nat Biotechnol 2005; 23:584 - 90; http://dx.doi.org/10.1038/nbt1087; PMID: 15834403
- Watanabe M, Boyer JL, Crystal RG. Genetic delivery of bevacizumab to suppress vascular endothelial growth factor-induced high-permeability pulmonary edema. Hum Gene Ther 2009; 20:598 - 610; http://dx.doi.org/10.1089/hum.2008.169; PMID: 19254174
- Ho DT, Wykoff-Clary S, Gross CS, Schneider D, Jin F, Kretschmer PJ, et al. Growth inhibition of an established A431 xenograft tumor by a full-length anti-EGFR antibody following gene delivery by AAV. Cancer Gene Ther 2009; 16:184 - 94; http://dx.doi.org/10.1038/cgt.2008.68; PMID: 18758433
- Wang G, Qiu J, Wang R, Krause A, Boyer JL, Hackett NR, et al. Persistent expression of biologically active anti-HER2 antibody by AAVrh.10-mediated gene transfer. Cancer Gene Ther 2010; 17:559 - 70; http://dx.doi.org/10.1038/cgt.2010.11; PMID: 20448672
- Vigna E, Pacchiana G, Mazzone M, Chiriaco C, Fontani L, Basilico C, et al. “Active” cancer immunotherapy by anti-Met antibody gene transfer. Cancer Res 2008; 68:9176 - 83; http://dx.doi.org/10.1158/0008-5472.CAN-08-1688; PMID: 19010889
- Balazs AB, Chen J, Hong CM, Rao DS, Yang L, Baltimore D. Antibody-based protection against HIV infection by vectored immunoprophylaxis. Nature 2012; 481:81 - 4; http://dx.doi.org/10.1038/nature10660; PMID: 22139420
- Arafat WO, Gómez-Navarro J, Buchsbaum DJ, Xiang J, Wang M, Casado E, et al. Effective single chain antibody (scFv) concentrations in vivo via adenoviral vector mediated expression of secretory scFv. Gene Ther 2002; 9:256 - 62; http://dx.doi.org/10.1038/sj.gt.3301639; PMID: 11896464
- Sanz L, Kristensen P, Russell SJ, Ramirez García JR, Alvarez-Vallina L. Generation and characterization of recombinant human antibodies specific for native laminin epitopes: potential application in cancer therapy. Cancer Immunol Immunother 2001; 50:557 - 65; http://dx.doi.org/10.1007/s00262-001-0235-5; PMID: 11776378
- Sanz L, García-Bermejo L, Blanco FJ, Kristensen P, Feijóo M, Suárez E, et al. A novel cell binding site in the coiled-coil domain of laminin involved in capillary morphogenesis. EMBO J 2003; 22:1508 - 17; http://dx.doi.org/10.1093/emboj/cdg150; PMID: 12660158
- Sanz L, Kristensen P, Blanco B, Facteau S, Russell SJ, Winter G, et al. Single-chain antibody-based gene therapy: inhibition of tumor growth by in situ production of phage-derived human antibody fragments blocking functionally active sites of cell-associated matrices. Gene Ther 2002; 9:1049 - 53; http://dx.doi.org/10.1038/sj.gt.3301725; PMID: 12101437
- Sánchez-Arévalo Lobo VJ, Cuesta AM, Sanz L, Compte M, García P, Prieto J, et al. Enhanced antiangiogenic therapy with antibody-collagen XVIII NC1 domain fusion proteins engineered to exploit matrix remodeling events. Int J Cancer 2006; 119:455 - 62; http://dx.doi.org/10.1002/ijc.21851; PMID: 16477626
- Afanasieva TA, Wittmer M, Vitaliti A, Ajmo M, Neri D, Klemenz R. Single-chain antibody and its derivatives directed against vascular endothelial growth factor: application for antiangiogenic gene therapy. Gene Ther 2003; 10:1850 - 9; http://dx.doi.org/10.1038/sj.gt.3302085; PMID: 12960975
- Liu X, Wu J, Zhang S, Li C, Huang Q. Novel strategies to augment genetically delivered immunotoxin molecular therapy for cancer therapy. Cancer Gene Ther 2009; 16:861 - 72; http://dx.doi.org/10.1038/cgt.2009.30; PMID: 19461676
- Kontermann R. Dual targeting strategies with bispecific antibodies. MAbs 2012; 4:4 - 14; PMID: 22327426
- Carter P. Improving the efficacy of antibody-based cancer therapies. Nat Rev Cancer 2001; 1:118 - 29; http://dx.doi.org/10.1038/35101072; PMID: 11905803
- Müller D, Kontermann RE. Bispecific antibodies for cancer immunotherapy: Current perspectives. BioDrugs 2010; 24:89 - 98; http://dx.doi.org/10.2165/11530960-000000000-00000; PMID: 20199124
- Dhimolea E, Reichert JM. World Bispecific Antibody Summit, September 27-28, 2011, Boston, MA. MAbs 2012; 4:4 - 13; http://dx.doi.org/10.4161/mabs.4.1.18821; PMID: 22327426
- Linke R, Klein A, Seimetz D. Catumaxomab: clinical development and future directions. MAbs 2010; 2:129 - 36; http://dx.doi.org/10.4161/mabs.2.2.11221; PMID: 20190561
- Bargou R, Leo E, Zugmaier G, Klinger M, Goebeler M, Knop S, et al. Tumor regression in cancer patients by very low doses of a T cell-engaging antibody. Science 2008; 321:974 - 7; http://dx.doi.org/10.1126/science.1158545; PMID: 18703743
- Chames P, Baty D. Bispecific antibodies for cancer therapy: the light at the end of the tunnel?. MAbs 2009; 1:539 - 47; http://dx.doi.org/10.4161/mabs.1.6.10015; PMID: 20073127
- Blanco B, Holliger P, Vile RG, Álvarez-Vallina L. Induction of human T lymphocyte cytotoxicity and inhibition of tumor growth by tumor-specific diabody-based molecules secreted from gene-modified bystander cells. J Immunol 2003; 171:1070 - 7; PMID: 12847281
- Compte M, Blanco B, Serrano F, Cuesta AM, Sanz L, Bernad A, et al. Inhibition of tumor growth in vivo by in situ secretion of bispecific anti-CEA x anti-CD3 diabodies from lentivirally transduced human lymphocytes. Cancer Gene Ther 2007; 14:380 - 8; http://dx.doi.org/10.1038/sj.cgt.7701021; PMID: 17218946
- Kim SM, Lim JY, Park SI, Jeong CH, Oh JH, Jeong M, et al. Gene therapy using TRAIL-secreting human umbilical cord blood-derived mesenchymal stem cells against intracranial glioma. Cancer Res 2008; 68:9614 - 23; http://dx.doi.org/10.1158/0008-5472.CAN-08-0451; PMID: 19047138
- Sasportas LS, Kasmieh R, Wakimoto H, Hingtgen S, van de Water JA, Mohapatra G, et al. Assessment of therapeutic efficacy and fate of engineered human mesenchymal stem cells for cancer therapy. Proc Natl Acad Sci U S A 2009; 106:4822 - 7; http://dx.doi.org/10.1073/pnas.0806647106; PMID: 19264968
- Bexell D, Scheding S, Bengzon J. Toward brain tumor gene therapy using multipotent mesenchymal stromal cell vectors. Mol Ther 2010; 18:1067 - 75; http://dx.doi.org/10.1038/mt.2010.58; PMID: 20407426
- Luetzkendorf J, Mueller LP, Mueller T, Caysa H, Nerger K, Schmoll HJ. Growth inhibition of colorectal carcinoma by lentiviral TRAIL-transgenic human mesenchymal stem cells requires their substantial intratumoral presence. J Cell Mol Med 2010; 14:2292 - 304; http://dx.doi.org/10.1111/j.1582-4934.2009.00794.x; PMID: 19508388
- Studeny M, Marini FC, Champlin RE, Zompetta C, Fidler IJ, Andreeff M. Bone marrow-derived mesenchymal stem cells as vehicles for interferon-beta delivery into tumors. Cancer Res 2002; 62:3603 - 8; PMID: 12097260
- Ren C, Kumar S, Chanda D, Kallman L, Chen J, Mountz JD, et al. Cancer gene therapy using mesenchymal stem cells expressing interferon-beta in a mouse prostate cancer lung metastasis model. Gene Ther 2008; 15:1446 - 53; http://dx.doi.org/10.1038/gt.2008.101; PMID: 18596829
- Chen X, Lin X, Zhao J, Shi W, Zhang H, Wang Y, et al. A tumor-selective biotherapy with prolonged impact on established metastases based on cytokine gene-engineered MSCs. Mol Ther 2008; 16:749 - 56; http://dx.doi.org/10.1038/mt.2008.3; PMID: 18362930
- Harting MT, Jimenez F, Xue H, Fischer UM, Baumgartner J, Dash PK, et al. Intravenous mesenchymal stem cell therapy for traumatic brain injury. J Neurosurg 2009; 110:1189 - 97; http://dx.doi.org/10.3171/2008.9.JNS08158; PMID: 19301973
- Zou W, Zheng H, He TC, Chang J, Fu YX, Fan W. LIGHT delivery to tumors by mesenchymal stem cells mobilizes an effective antitumor immune response. Cancer Res 2012; 72:2980 - 9; http://dx.doi.org/10.1158/0008-5472.CAN-11-4216; PMID: 22511579
- Compte M, Cuesta AM, Sánchez-Martín D, Alonso-Camino V, Vicario JL, Sanz L, et al. Tumor immunotherapy using gene-modified human mesenchymal stem cells loaded into synthetic extracellular matrix scaffolds. Stem Cells 2009; 27:753 - 60; http://dx.doi.org/10.1634/stemcells.2008-0831; PMID: 19096041
- Karnoub AE, Dash AB, Vo AP, Sullivan A, Brooks MW, Bell GW, et al. Mesenchymal stem cells within tumour stroma promote breast cancer metastasis. Nature 2007; 449:557 - 63; http://dx.doi.org/10.1038/nature06188; PMID: 17914389
- Eliopoulos N, Gagnon RF, Francois M, Galipeau J. Erythropoietin delivery by genetically engineered bone marrow stromal cells for correction of anemia in mice with chronic renal failure. J Am Soc Nephrol 2006; 17:1576 - 84; http://dx.doi.org/10.1681/ASN.2005101035; PMID: 16672321
- Wang N, Fallavollita L, Nguyen L, Burnier J, Rafei M, Galipeau J, et al. Autologous bone marrow stromal cells genetically engineered to secrete an igf-I receptor decoy prevent the growth of liver metastases. Mol Ther 2009; 17:1241 - 9; http://dx.doi.org/10.1038/mt.2009.82; PMID: 19367255
- Campeau PM, Rafei M, François M, Birman E, Forner KA, Galipeau J. Mesenchymal stromal cells engineered to express erythropoietin induce anti-erythropoietin antibodies and anemia in allorecipients. Mol Ther 2009; 17:369 - 72; http://dx.doi.org/10.1038/mt.2008.270; PMID: 19088705
- Xin H, Kanehira M, Mizuguchi H, Hayakawa T, Kikuchi T, Nukiwa T, et al. Targeted delivery of CX3CL1 to multiple lung tumors by mesenchymal stem cells. Stem Cells 2007; 25:1618 - 26; http://dx.doi.org/10.1634/stemcells.2006-0461; PMID: 17412895
- Xin H, Sun R, Kanehira M, Takahata T, Itoh J, Mizuguchi H, et al. Intratracheal delivery of CX3CL1-expressing mesenchymal stem cells to multiple lung tumors. Mol Med 2009; 15:321 - 7; http://dx.doi.org/10.2119/molmed.2009.00059; PMID: 19603106
- Ren C, Kumar S, Chanda D, Chen J, Mountz JD, Ponnazhagan S. Therapeutic potential of mesenchymal stem cells producing interferon-alpha in a mouse melanoma lung metastasis model. Stem Cells 2008; 26:2332 - 8; http://dx.doi.org/10.1634/stemcells.2008-0084; PMID: 18617688
- Nakamizo A, Marini F, Amano T, Khan A, Studeny M, Gumin J, et al. Human bone marrow-derived mesenchymal stem cells in the treatment of gliomas. Cancer Res 2005; 65:3307 - 18; PMID: 15833864
- Studeny M, Marini FC, Dembinski JL, Zompetta C, Cabreira-Hansen M, Bekele BN, et al. Mesenchymal stem cells: potential precursors for tumor stroma and targeted-delivery vehicles for anticancer agents. J Natl Cancer Inst 2004; 96:1593 - 603; http://dx.doi.org/10.1093/jnci/djh299; PMID: 15523088
- Rachakatla RS, Marini F, Weiss ML, Tamura M, Troyer D. Development of human umbilical cord matrix stem cell-based gene therapy for experimental lung tumors. Cancer Gene Ther 2007; 14:828 - 35; http://dx.doi.org/10.1038/sj.cgt.7701077; PMID: 17599089
- Matsuzuka T, Rachakatla RS, Doi C, Maurya DK, Ohta N, Kawabata A, et al. Human umbilical cord matrix-derived stem cells expressing interferon-beta gene significantly attenuate bronchioloalveolar carcinoma xenografts in SCID mice. Lung Cancer 2010; 70:28 - 36; http://dx.doi.org/10.1016/j.lungcan.2010.01.003; PMID: 20138387
- Kidd S, Caldwell L, Dietrich M, Samudio I, Spaeth EL, Watson K, et al. Mesenchymal stromal cells alone or expressing interferon-beta suppress pancreatic tumors in vivo, an effect countered by anti-inflammatory treatment. Cytotherapy 2010; 12:615 - 25; http://dx.doi.org/10.3109/14653241003631815; PMID: 20230221
- Seo KW, Lee HW, Oh YI, Ahn JO, Koh YR, Oh SH, et al. Anti-tumor effects of canine adipose tissue-derived mesenchymal stromal cell-based interferon-β gene therapy and cisplatin in a mouse melanoma model. Cytotherapy 2011; 13:944 - 55; http://dx.doi.org/10.3109/14653249.2011.584864; PMID: 21846298
- Nakamura K, Ito Y, Kawano Y, Kurozumi K, Kobune M, Tsuda H, et al. Antitumor effect of genetically engineered mesenchymal stem cells in a rat glioma model. Gene Ther 2004; 11:1155 - 64; http://dx.doi.org/10.1038/sj.gt.3302276; PMID: 15141157
- Gunnarsson S, Bexell D, Svensson A, Siesjö P, Darabi A, Bengzon J. Intratumoral IL-7 delivery by mesenchymal stromal cells potentiates IFNgamma-transduced tumor cell immunotherapy of experimental glioma. J Neuroimmunol 2010; 218:140 - 4; http://dx.doi.org/10.1016/j.jneuroim.2009.10.017; PMID: 19914721
- Elzaouk L, Moelling K, Pavlovic J. Anti-tumor activity of mesenchymal stem cells producing IL-12 in a mouse melanoma model. Exp Dermatol 2006; 15:865 - 74; http://dx.doi.org/10.1111/j.1600-0625.2006.00479.x; PMID: 17002683
- Hong X, Miller C, Savant-Bhonsale S, Kalkanis SN. Antitumor treatment using interleukin- 12-secreting marrow stromal cells in an invasive glioma model. Neurosurgery 2009; 64:1139 - 46, discussion 1146-7; http://dx.doi.org/10.1227/01.NEU.0000345646.85472.EA; PMID: 19487894
- Chen X, Lin X, Zhao J, Shi W, Zhang H, Wang Y, et al. A tumor-selective biotherapy with prolonged impact on established metastases based on cytokine gene-engineered MSCs. Mol Ther 2008; 16:749 - 56; http://dx.doi.org/10.1038/mt.2008.3; PMID: 18362930
- Duan X, Guan H, Cao Y, Kleinerman ES. Murine bone marrow-derived mesenchymal stem cells as vehicles for interleukin-12 gene delivery into Ewing sarcoma tumors. Cancer 2009; 115:13 - 22; http://dx.doi.org/10.1002/cncr.24013; PMID: 19051291
- Gao J, Dennis JE, Muzic RF, Lundberg M, Caplan AI. The dynamic in vivo distribution of bone marrow-derived mesenchymal stem cells after infusion. Cells Tissues Organs 2001; 169:12 - 20; http://dx.doi.org/10.1159/000047856; PMID: 11340257
- Ryu CH, Park SH, Park SA, Kim SM, Lim JY, Jeong CH, et al. Gene therapy of intracranial glioma using interleukin 12-secreting human umbilical cord blood-derived mesenchymal stem cells. Hum Gene Ther 2011; 22:733 - 43; http://dx.doi.org/10.1089/hum.2010.187; PMID: 21261460
- Xu G, Jiang XD, Xu Y, Zhang J, Huang FH, Chen ZZ, et al. Adenoviral-mediated interleukin-18 expression in mesenchymal stem cells effectively suppresses the growth of glioma in rats. Cell Biol Int 2009; 33:466 - 74; http://dx.doi.org/10.1016/j.cellbi.2008.07.023; PMID: 18725309
- Hu M, Yang JL, Teng H, Jia YQ, Wang R, Zhang XW, et al. Anti-angiogenesis therapy based on the bone marrow-derived stromal cells genetically engineered to express sFlt-1 in mouse tumor model. BMC Cancer 2008; 8:306.:306.
- Mao W, Zhu X, Tang D, Zhao Y, Zhao B, Ma G, et al. TNF-α expression in the UCB-MSCs as stable source inhibits gastric cancers growth in nude mice. Cancer Invest 2012; 30:463 - 72; http://dx.doi.org/10.3109/07357907.2012.675385; PMID: 22536934
- Menon LG, Kelly K, Yang HW, Kim SK, Black PM, Carroll RS. Human bone marrow-derived mesenchymal stromal cells expressing S-TRAIL as a cellular delivery vehicle for human glioma therapy. Stem Cells 2009; 27:2320 - 30; http://dx.doi.org/10.1002/stem.136; PMID: 19544410
- Loebinger MR, Eddaoudi A, Davies D, Janes SM. Mesenchymal stem cell delivery of TRAIL can eliminate metastatic cancer. Cancer Res 2009; 69:4134 - 42; http://dx.doi.org/10.1158/0008-5472.CAN-08-4698; PMID: 19435900
- Grisendi G, Bussolari R, Cafarelli L, Petak I, Rasini V, Veronesi E, et al. Adipose-derived mesenchymal stem cells as stable source of tumor necrosis factor-related apoptosis-inducing ligand delivery for cancer therapy. Cancer Res 2010; 70:3718 - 29; http://dx.doi.org/10.1158/0008-5472.CAN-09-1865; PMID: 20388793
- Mohr A, Albarenque SM, Deedigan L, Yu R, Reidy M, Fulda S, et al. Targeting of XIAP combined with systemic mesenchymal stem cell-mediated delivery of sTRAIL ligand inhibits metastatic growth of pancreatic carcinoma cells. Stem Cells 2010; 28:2109 - 20; http://dx.doi.org/10.1002/stem.533; PMID: 20882532
- Kim SM, Oh JH, Park SA, Ryu CH, Lim JY, Kim DS, et al. Irradiation enhances the tumor tropism and therapeutic potential of tumor necrosis factor-related apoptosis-inducing ligand-secreting human umbilical cord blood-derived mesenchymal stem cells in glioma therapy. Stem Cells 2010; 28:2217 - 28; http://dx.doi.org/10.1002/stem.543; PMID: 20945331
- Mueller LP, Luetzkendorf J, Widder M, Nerger K, Caysa H, Mueller T. TRAIL-transduced multipotent mesenchymal stromal cells (TRAIL-MSC) overcome TRAIL resistance in selected CRC cell lines in vitro and in vivo. Cancer Gene Ther 2011; 18:229 - 39; http://dx.doi.org/10.1038/cgt.2010.68; PMID: 21037557
- Choi SA, Hwang SK, Wang KC, Cho BK, Phi JH, Lee JY, et al. Therapeutic efficacy and safety of TRAIL-producing human adipose tissue-derived mesenchymal stem cells against experimental brainstem glioma. Neuro Oncol 2011; 13:61 - 9; http://dx.doi.org/10.1093/neuonc/noq147; PMID: 21062796
- Kim SM, Woo JS, Jeong CH, Ryu CH, Lim JY, Jeun SS. Effective combination therapy for malignant glioma with TRAIL-secreting mesenchymal stem cells and lipoxygenase inhibitor MK886. Cancer Res 2012; 72:4807 - 17; http://dx.doi.org/10.1158/0008-5472.CAN-12-0123; PMID: 22962275
- Zhu X, Su D, Xuan S, Ma G, Dai Z, Liu T, et al. Gene therapy of gastric cancer using LIGHT-secreting human umbilical cord blood-derived mesenchymal stem cells. Gastric Cancer 2012 ; In press http://dx.doi.org/10.1007/s10120-012-0166-1; PMID: 22850801
- Stagg J, Lejeune L, Paquin A, Galipeau J. Marrow stromal cells for interleukin-2 delivery in cancer immunotherapy. Hum Gene Ther 2004; 15:597 - 608; http://dx.doi.org/10.1089/104303404323142042; PMID: 15212718
- Eliopoulos N, Francois M, Boivin MN, Martineau D, Galipeau J. Neo-organoid of marrow mesenchymal stromal cells secreting interleukin-12 for breast cancer therapy. Cancer Res 2008; 68:4810 - 8; http://dx.doi.org/10.1158/0008-5472.CAN-08-0160; PMID: 18559528
- Seo SH, Kim KS, Park SH, Suh YS, Kim SJ, Jeun SS, et al. The effects of mesenchymal stem cells injected via different routes on modified IL-12-mediated antitumor activity. Gene Ther 2011; 18:488 - 95; http://dx.doi.org/10.1038/gt.2010.170; PMID: 21228885
- Goren A, Dahan N, Goren E, Baruch L, Machluf M. Encapsulated human mesenchymal stem cells: a unique hypoimmunogenic platform for long-term cellular therapy. FASEB J 2010; 24:22 - 31; http://dx.doi.org/10.1096/fj.09-131888; PMID: 19726759
- Reagan MR, Seib FP, McMillin DW, Sage EK, Mitsiades CS, Janes SM, et al. Stem Cell Implants for Cancer Therapy: TRAIL-Expressing Mesenchymal Stem Cells Target Cancer Cells In Situ. J Breast Cancer 2012; 15:273 - 82; http://dx.doi.org/10.4048/jbc.2012.15.3.273; PMID: 23091539
- Balazs AB, West AP Jr.. Antibody gene transfer for HIV immunoprophylaxis. Nat Immunol 2013; 14:1 - 5; http://dx.doi.org/10.1038/ni.2480; PMID: 23238748
- Acarregui A, Murua A, Pedraz JL, Orive G, Hernández RM. A perspective on bioactive cell microencapsulation. BioDrugs 2012; 26:283 - 301; PMID: 22715813