Abstract
Calcium phosphate materials have been used increasingly in the past 40 years as bone graft substitutes in the dental and orthopedic fields. Accordingly, numerous fabrication methods have been proposed and used. However, the controlled production of spherical calcium phosphate particles remains a challenge. Since such particles are essential for the synthesis of pastes and cements delivered into the host bone by minimally-invasive approaches, the aim of the present document is to review their synthesis and applications. For that purpose, production methods were classified according to the used reagents (solutions, slurries, pastes, powders), dispersion media (gas, liquid, solid), dispersion tools (nozzle, propeller, sieve, mold), particle diameters of the end product (from 10 nm to 10 mm), and calcium phosphate phases. Low-temperature calcium phosphates such as monetite, brushite or octacalcium phosphate, as well as high-temperature calcium phosphates, such as hydroxyapatite, β-tricalcium phosphate or tetracalcium phosphate, were considered. More than a dozen production methods and over hundred scientific publications were discussed.
Every year, millions of human beings suffer from a bone loss caused by trauma and illnesses. The standard treatment of care consists in filling the bone defect with a material to support new bone formation. In most cases, autologous bone transplant is used.Citation1,Citation2 Unfortunately, extracting this material requires a second surgery and may lead to complications such as infections or long-lasting pains.Citation3-Citation5 In fact, most studies report a complication rate close to 20%. So, the scientific community has made large efforts over the past 40 years to find suitable bone graft substitutes.Citation6-Citation8 All types of materials have been considered and tested such as polymers,Citation9,Citation10 ceramic-polymer composites,Citation9 metals,Citation11,Citation12 glasses,Citation8 or ceramics.Citation2 However, since human bone consists of 65% carbonated apatite, most research efforts have been focused on calcium phosphates (CaPs), in particular hydroxyapatite (HA; Ca5(PO4)3OH), β-tricalcium phosphate (β-TCP; Ca3(PO4)2), and their composites called biphasic calcium phosphates (BCP)Citation2,Citation13 (). These materials have an excellent biocompatibility, osteoconductivity, and osteotransductivity.Citation14,Citation15 Also, recent reports have demonstrated an osteoinductive potential.Citation16-Citation19
Table 1. List of CaP phases
CaP based bone graft substitutes are available in different forms such as granules, porous blocks, cements, “putties” ( = non-setting slurries or pastes), sponges/foams, or strips/membranes.Citation2,Citation20 Granules are by far the most frequently used materials due to their relatively low cost,Citation20 broad availability, and good biological properties. Indeed, the inter-granular space is rapidly invaded by newly-formed bone and ceramic resorption can proceed fast and throughout the defect.Citation21 So, the use of non-granular materials remains fairly marginal and is often limited to very specific indications. For example, porous blocks are particularly adequate when the bone defect has a geometrically well-defined shape, such as in open-wedge tibia osteotomyCitation22 or after bone extraction with a trephine system.Citation23 Porous blocks are also extensively used in tissue engineering as scaffolds for cells.Citation24 In the 1990s, the so-called CaP cements (CPCs) raised hopes to eventually replace metals as raw materials for internal fixators.Citation25 Unfortunately, CPCs have several important drawbacks such as their high cost,Citation20 brittleness,Citation26 and slow resorption rate.Citation27 Nevertheless, they can be implanted by minimally invasive techniques,Citation25 and provide mechanical support.Citation27 Also, they have been considered for the treatment of vertebral bone fractures, especially in young patients.Citation28,Citation29 The three other forms of CaP bone substitutes mentioned herein (putties, sponge, membrane) are generally in the form of a polymer matrix filled with CaP particles. Among these three forms, putties have perhaps the best commercial potential, because they often have biological properties as good as those of CaP granules,Citation21 but a much better handling. However, putties have such a broad range of compositions, rheological properties, and biological responsesCitation30 that it is difficult to describe their properties succinctly. On one hand, putties may consist of nanoparticles dispersed in an aqueous solution.Citation31-Citation35 After implantation, the paste is seen as a dense solid by cells. Cell invasion is possible but requires material displacement or removal.Citation35 On the other hand, putties may consist of granules held together by a hydrogel, hence allowing rapid bone ingrowth into the space filled with the hydrogel and ceramic resorption throughout the defect.Citation21,Citation36 Even though the first CaP putties were proposed in the 1990sCitation37 and appeared only a decade ago in Western countries,Citation33,Citation34 numerous CaP putties have been launched in recent years.Citation30 This evolution is certainly driven by the two-digit yearly increase of the bone graft substitute market sizeCitation38 but also by a trend toward simplified pre-operative and/or operative handling properties.
All CPCs and putties have a common feature: they consist of singular solid particles that interact physically and / or chemically during handling. As such, understanding these interactions and controlling the design of CaP particles is of paramount importance to control the handling properties of putties such as cohesionCitation39 and injectability.Citation40-Citation42 Several approaches can be used to optimize both properties. For example, an increase of the viscosity of the liquid phase improves the paste cohesionCitation39 and reduces the phase separation between liquid and solid, hence resulting in a better injectability.Citation40 Another strategy is to decrease the mean particle size, as it is known that pastes made of nanoparticles combine a good injectabilityCitation40 and a good cohesion.Citation39 Unfortunately, a reduction of the mean particle size is not always possible. For instance, α-TCP and β-TCP powders which are generally obtained by high-temperature solid-state reactions and milling, do not become smaller during prolonged milling but amorphous.Citation43,Citation44 Also, most granular CaP bone graft substitutes have a diameter in the range of 0.5 to 5mm because they should allow blood vessel in-growth in the intergranular space, hence providing rapid ceramic resorption and bone in-growth. A third strategy to improve injectability is to use spherical particles. Indeed, Ishikawa et al.Citation45,Citation46 showed that the injectability of a CPC paste can be enhanced using spherical tetracalcium phosphate particles. Unfortunately, manufacturing spherical CaP particles is not easy and despite a rapid increase in papers devoted to spherical CaP particles (), it is often difficult to know which method to select. Therefore, the aim of the present document is 2-fold: (1) the main emphasis is set on the review of methods used to produce spherical CaP particles, from nano- to milliparticles, and from low-temperature to high-temperature CaP phases; (2) a minor emphasis is set on the review of studies in which spherical CaP particles have been used. The next two sections of the present article will address these two aims.
Figure 1. evolution of the documents listed in “Scopus” (www.scopus.com) fulfilling the following search criteria: (“spherical” or “sphere” or “round”) and (“calcium phosphate” or “apatite” or “calcium hydrogen phosphate”) and (“granule” or “bead” or “particle”). Date: March 14, 2013.
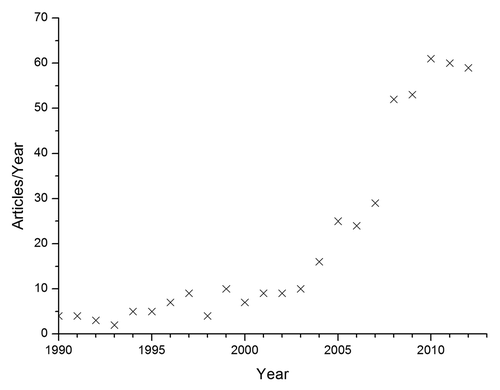
To identify the relevant literature, a search was performed in Scopus (www.scopus.com) with the following search criteria: (spherical OR sphere OR round) AND (calcium phosphate OR apatite OR calcium hydrogen phosphate) AND (granule OR bead OR particle). Over 500 documents were found (March 14, 2013). Additional articles which did not show up in the literature search but which are relevant for the present review were also considered.
Very often, several names have been given to the same or to very similar production methods. For simplicity reasons, similar production methods were grouped under one name (e.g., “drip casting” instead of “droplet extrusion,” or “plasma melting” for “combustion flame spraying” and “flame spheroidization”) ().
Table 2. Classification of the methods used to produce spherical CaP particles according to the types of reagents, the dispersion media, the dispersion tools, the consolidation methods, the resulting diameters, and the final compositions
Methods to Produce Spherical CaP Particles
More than a dozen different methods have been used to produce spherical CaP particles. Considering this large body of information, it is difficult to provide a short and clear overview. In an attempt to solve this problem, the different production methods were classified according to various criteria: (1) the types of reagents used to produce spherical particles; (2) the media in which the spherical aggregates / particles were dispersed or formed; (3) the dispersion tools; (4) the consolidation reactions that led to solid spherical particles; (5) the typical diameters of the resulting particles; and (6) the CaP phases that could be obtained (). The next lines are devoted to these various aspects.
Reagents
In principle, there are four types of reagents used in the production of spherical CaP particles: solutions, slurries ( = suspensions), pastes, and powders. Most methods are either based on solutions, slurries, and pastes because it is easier to control the dispersion of a fluid than that of a solid. In this document, it is assumed that a slurry flows under its own weight contrary to a paste.
Solution-based methods are the most adequate to produce nanoparticles (< 100 nm). For example, several authors have reported the production of spherical CaP particles with a diameter close to 15–20 nm, either by precipitation,Citation47-Citation49 precipitation-emulsification,Citation50,Citation51 spray-drying,Citation52 and flame-synthesis ( = flame pyrolysis)Citation53-Citation55 (). The solutions may be either aqueous (precipitation, spray-drying), non-aqueous (precipitation,Citation56 flame-synthesisCitation57-Citation60) or a mixture thereof (precipitation-emulsificationCitation50,Citation51,Citation61).
Slurry-based methods have many similarities with solution-based methods except that the liquid already contains dispersed particles. Therefore, the spherical particles produced from slurries generally consist of agglomerates of the primary particles dispersed in the slurry, and their diameter is often a few orders of magnitude bigger than the diameter of the primary particles (). For example, 1mm particles can be obtained by drip-casting ( = droplet extrusion),Citation62-Citation71 emulsification,Citation72-Citation82 hydro-casting,Citation83 and by the lost-wax methodCitation84,Citation85 (). The main exception to this rule is the method called suspension plasma spraying ( = atomization), in which a CaP suspension is injected into a high-energy plasma torch and then sprayed at high energy through a nozzle to form particles in the range of 10nm to 100µm.Citation86-Citation88
Figure 2. spherical CaP particles obtained using some of the methods mentioned in . (A) Precipitation, dicalcium phosphate (scale bar: 2µm), (B) spray drying; monocalcium phosphate monohydrate (50µm), (C) freeze granulation; calcium sulfate dihydrate (100µm), (D) emulsification; dicalcium phosphate dehydrate (500 µm), (E) drip casting; β-tricalcium phosphate (1000 µm), (F) extrusion-spheronization; β-tricalcium phosphate (500 µm), (G) suspension plasma spraying; mixture of high-temperature calcium phosphates (300 nm), (H) 3D printing; α-tricalcium phosphate-dicalcium phosphate mixtures (3000µm); (I) precipitation in ethylene glycol; β-tricalcium phosphate (500 nm)
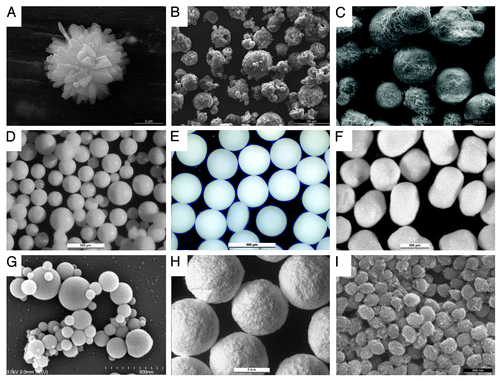
In all slurry-based methods, the consolidation stage is very important because slurries lose their shape under gravity conditions (for more information, see the section devoted to the consolidation stage). This is the main difference compared with paste-based methods. Indeed the latter methods rely on the ability of the pastes to behave like solids. For example, in the extrusion-spheronization process, pastes are extruded through a die to form rods. These rods are then spun on a horizontal plate covered by small truncated pyramids (roughly 1–2 mm in height) (). The mechanical interactions between the rods, and these truncated pyramids lead to the rupture of the rods into small segments, which are then rounded by the rotational movements and the interactions with the truncated pyramids. Since all paste-based methods (i.e., spray granulation,Citation89,Citation90 extrusion-spheronization,Citation89,Citation91 sieve-shakingCitation92) involve fairly viscous pastes, the particles obtained by these methods are typically in the millimeter range.
Figure 3. Schematic description of the dispersion tools: nozzles, propellers, sieves, templates and molds. The propeller scheme shows the dispersion of a slurry into a liquid (emulsification). In the sieve-shaking method, the thick paste is pushed through a sieve with a pestle. In the extrusion-spheronization method, long rods are produced by paste extrusion through a sieve and then rounded in a spheronizer (see bottom of the figure). In the “lost-wax” method, spherical particles can be produced with a template or a mold. In the latter case, the particles are either hollow (after template removal) or biphasic.
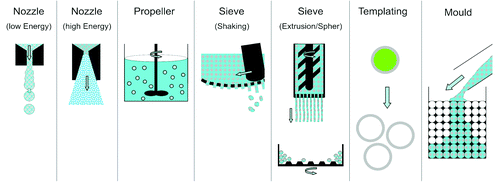
One difficulty in the use of pastes is the adjustment of their rheological properties. Generally, the liquid amount has to lie just below (spray-granulation,Citation89,Citation90 sieve shakingCitation92) or just above (extrusion-spheronizationCitation89,Citation91) the plastic limit of the powder (the plastic limit is defined as the minimum amount of water that has to be added to a powder to get a paste). However, the plastic limit may vary and small deviations may provoke drastic changes of the paste viscosity, in particular for powders with a fairly large mean particle size (> 1–10 µm). Also, the paste has to be viscous but not sticky. This is a particularly difficult task for extrusion spheronization, and explains why only very few binders can be used.Citation93
Among the different reagents, powders are solely used in the process called “plasma melting” (also called “combustion flame spraying,” and “flame spheronization”) during which particles are injected into gas plasma.Citation45,Citation46,Citation94-Citation97 The high temperature melts the particles into spherical droplets. Subsequently, these droplets are consolidated by freezing. As a result, the particle size distribution of the processed particles depends mainly on the initial particle size distribution and little can be done to control it during the process.
All the methods listed in are top-down approaches, i.e., a large volume is divided into smaller volumes. In recent years, quite a few bottom-up approaches have been proposed, the so-called solid free form fabrication (SFFF) methods, in which small solid subunits are piled up in a controlled manner to form complex 3D architectures.Citation98 One of these methods, called 3D printing, relies on the spatially-controlled reaction of a liquid with a solid.Citation99,Citation100 For example, Gbureck et al.Citation100 printed CaP porous scaffolds by jetting phosphoric acid onto an α-TCP powder bed. Even though SFFF methods are primarily used for the synthesis of complex objects, they can also be applied to the production of small particles (). However, resolution is relatively limited,Citation101 so the production of CaP spheres smaller than 1mm is possible but difficult.
Before ending this section, it is worth mentioning that spherical particles can also be obtained by the so-called double diffusion method in which a calcium-rich solution is separated from a phosphate-rich solution by a permeable membrane such as a gel.Citation102,Citation103 It can be considered as a type of precipitation reaction.
Dispersion media
With the exception of precipitation reactions, all production methods rely on the dispersion of the reagent (solution, slurry, paste, or solid) into another phase: a gas to form an aerosol, a liquid to form an emulsion, or a solid acting as a mold (lost-wax methodCitation84,Citation85). Whereas slurries have been used in all three dispersion approaches, solid reagents have only been dispersed in a gas (plasma meltingCitation45,Citation46,Citation94-Citation97). Due to their relatively low concentrations, solutions have not been used in the lost-wax method because it would only coat the walls of the template (or mold) instead of filling the template.
Interestingly, some methods combine different approaches. For example, Liu et al.Citation64 used a drip casting approach to deposit with a syringe a slurry into hemispherical molds ( = “lost-wax” method). Also, some production methods rely on two dispersion media: a gas and a liquid (e.g., for freeze-granulationCitation104 and drip castingCitation62-Citation71), or two immiscible liquids such as in hydro-casting.Citation83
Dispersion tools
Generally, three dispersion tools are used for the production of spherical CaP particles (): (1) nozzles to disperse solutions and slurries into a gas, (2) sieves to granulate pastes, and (3) propellers to produce emulsions and to granulate wet powders. Depending on the dispersion energy, strong changes of the final particle diameter can be achieved. This is particularly true for nozzle-based dispersion methods. In laminar conditions (e.g., in drip castingCitation62-Citation71), the mean diameter of the produced droplets is equal to 2–5 times the nozzle diameterCitation69 (). Also, there is a good control of the particle diameter and the particle size distribution is narrow. For example, Ribeiro et al.Citation67,Citation68 reported the synthesis of HA particles with a diameter close to 500 μm and a size dispersion lower than 10% (the size dispersion is defined by the ratio between standard deviation and mean of the particle size distribution). Even better results were obtained by Teraoka and Kato by hydro-casting, a method similar to drop-casting but with two small distinctions: the slurry is injected into a liquid instead of a gas and the injected amount is controlled with a micropipette.Citation83 Specifically, these authors produced 1.3mm α-TCP particles with a dispersity of 1.4% and a sphericity of 1.01 (ratio between long and short axis). On the negative side, it is difficult to obtain diameters below 100µm due to nozzle plugging. Interestingly, the same problem restricts the performance of sieve-based production methods such as extrusion-spheronizationCitation89,Citation91 or sieve-shakingCitation92 ().
At high dispersion energy (turbulent flow), the droplet diameters can be much smaller than the nozzle diameters. So, it is possible to obtain much smaller particles than by low-energy approaches. However, the particle size distribution is broad. For example, Andrianjatovo et al.Citation105 spray-dried β-TCP particles with a diameter in the range of 0.4 to 237µm.
Many propeller types and sizes can be used for the emulsification method but propellers play only a minor role in the achievement of a given droplet size. Other factors such as the stirring rate, the emulsifier type and concentration, or the viscosity of the two immiscible liquids are much more important.Citation73,Citation106
In the “lost-wax” method, spherical particles can be produced with a mold or a template (the mold or template has to be removed after CaP particle synthesis). In the latter case, the particles are either hollow (after template removal) or biphasic. To the best of our knowledge, there is currently no commercial application requiring such features.
Consolidation Methods
All production methods of spherical CaP particles involve fluids. This is necessary to provide the spherical shape. Immediately after their formation, the particles are still soft and their shape and size are still subject to change. The transition from a soft to a hard state ( = consolidation) is critical in order to obtain non-agglomerated, well defined spheres. Therefore, consolidation methods such as drying, freezing, gelling, and crystallization are of paramount importance ().
Figure 4. Scheme of the four consolidation methods. The particles are in gray, the aqueous solution is in blue, polymer chains are in red, and the bonds between polymer chains are in white. In the crystallization scheme, only a cement ( = dissolution-precipitation) reaction is shown. During drying, there is a size reduction. During crystallization, there is a change of the microstructure.
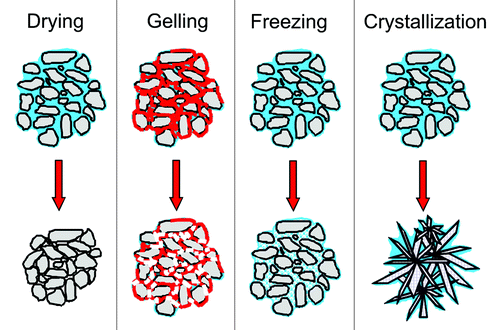
Drying is by far the most popular approach due to its simplicity. However, there is a size reduction associated with it which may cause density gradients and lead to heterogeneities (e.g., hollow particles during spray-dryingCitation107). This problem does not occur by freezing the particles in liquid nitrogen, but frozen particles have to be freeze-dried, which is a rather lengthy and expensive process. Also, the impact of the droplets into liquid nitrogen may impair the particle sphericity. Finally, the absence of shrinkage during freeze-drying is detrimental for the mechanical properties of the granules.
When a liquid is dispersed into another liquid without temperature change, it is not possible to rely on drying or freezing to consolidate the particles. In that case, two approaches can be used: (1) gelling and (2) crystallization. For example, Tuyen et al.Citation71 drop-casted slurries made of BCP-acetone-polycaprolactone (PCL) in deionized water. As PCL is insoluble in water, PCL precipitation occurred. Similarly, Paul and SharmaCitation72 emulsified slurries containing hydroxyapatite particles, chitosan, and acetic acid in paraffin oil. After five minutes of stirring, glutaraldehyde was added to crosslink chitosan and hence to harden the spheres. A slightly different approach was proposed by BohnerCitation73 using the so-called CaP emulsions: the slurry was a hydraulic cement paste that hardened over time. In such a case, polymer additives are not required, but timing becomes critical.
The present section was devoted to consolidation methods applied during the synthesis process. Consolidation methods applied after the synthesis process can also be applied (and are applied), in particular sintering. This will be discussed in a later section of the manuscript.
Particle diameters
Spherical particles can be obtained over a very broad size range, spanning from 10nm to a few millimeters. Generally, the smallest particles are obtained by solution-based methods (e.g., precipitation, flame synthesis) whereas the biggest particles are produced by paste-based methods (e.g., extrusion-spheronization, spray granulation). Even though all particle sizes can be obtained using several methods, particles in the range of 100 to 300 μm are difficult to produce with high yield and good shape control. Indeed, a diameter of 100 μm is at the upper range of what spray-drying, freeze granulation or plasma melting can successfully achieve whereas 300 μm is at the bottom range of what is feasible with drip casting, extrusion spheronization, 3D printing or the lost wax method ().
Not all methods can be used to produce spherical CaP particles with a narrow size distribution. Generally, high-energy dispersion tools lead to broad size distributions. For example, Jiao et al.Citation107 reported a diameter in the range of 0.1 to 60 μm for their spray-dried hydroxyapatite particles. Similarly, Andrianjatovo et al.Citation105 reported diameters between 0.4 and 237 µm for β-TCP particles. So, low-energy dispersion methods or SFFF methods must be used. Specifically, several methods are particularly adapted to obtain narrow size distribution: (1) precipitation methods characterized by burst nucleation and diffusion-controlled growth,Citation32 (2) drip casting,Citation62-Citation71 (3) hydro-casting,Citation83 and (4) 3D printing (). For example, Mateus et al.Citation65 who used drip casting to produce HA particles reported the synthesis of HA particles with a diameter in the range of 0.5 to 0.9mm and size dispersion lower than 10%. Nevertheless, the narrowest size distributions are obtained by machining approaches, such as hydro-casting and 3D printing, because with these techniques, the size and sometimes also the shape of each particle is controlled individually. However, these methods are currently limited to fairly large particles, typically in the millimeter range. For example, Teraoka and KatoCitation83 used hydro-casting to produce 1.3 mm α-TCP particles with a dispersity of 1.4%, and a sphericity of 1.01 (ratio between long and short axis).
Even though certain production methods provide particle populations with a narrow size distribution, such populations are generally classified, for example by sieving.Citation90 This is not only a way to remove too small or too large particles, but also to control their sizes.
In this section, the diameter of the particles formed by various production processes was discussed. However, there is always a risk of agglomeration that could potentially ruin the efforts to produce a narrow particle size distribution. In general, small particles (< 1–10 µm) are much more prone to agglomeration due to van der Waals forces than larger particles. Also, drying processes, particularly in the presence of water, are very critical because they can promote compaction and agglomeration of previously loose particles.
Composition
Not all production methods can be used to produce one particular CaP phase (). As a result, the choice of a particular production method has to be related to the phase that has to be produced. Generally, high-temperature processes, e.g., plasma melting and flame-synthesis, cannot be used to produce hydrated phases such as DCPD and OCP. Similarly, low-temperature processes, such as precipitation, are not adequate for the production of α-TCP and TetCP which are only thermodynamically stable above 1100–1200°C. However, there are exceptions. For example, Mohn et al.Citation59 showed recently that MCPM, DCP and DCPD were present in flame-synthesized CaP nanoparticles. The authors assumed that hydration occurred during cooling. Also Tao et al.Citation31,Citation56 synthesized nanosized β-TCP octahedral and hexagonal crystals by precipitation in a non-aqueous medium. β-TCP is generally considered to be a high-temperature phase that can only be obtained by thermal treatments above 700–800°C. Another approach to obtain low-temperature CaP phases from high-temperature CaP phases is to apply post-treatments. Indeed, Gonda et al.Citation77 demonstrated that α-TCP beads placed into an autoclave at 160°C converted to CDHA. Similarly, Nomura et al.Citation108 converted calcium sulfate dihydrate particles into HA particles. Finally, DCP particles can be obtained by incubating α-TCP particles in phosphoric acid.Citation109
Obviously, high-temperature phases can be obtained from low-temperature phases by a simple thermal treatment. In fact, this strategy is widely used because the application of a thermal treatment on e.g., spray-dried or drip-casted particles cannot only remove organic additives used during the manufacturing process, but also consolidate the particles by sintering. Nevertheless, post-treatments may lead to aggregation or even deterioration of the particles. Thus, the use of post-treatments has to be considered carefully.
As hinted in the previous paragraph, many production methods listed in require the use of additives. The additives can have different functions, such as growth inhibitors for precipitations (e.g., proteinsCitation47), gelling agents for drip casting (e.g., alginatesCitation62,Citation65-Citation69), dispersants for the lost wax method, emulsions, and freeze casting (e.g., ammonium polyacrylatesCitation73,Citation84,Citation104), binders for spray-granulation and extrusion spheronization (e.g., microcrystalline celluloseCitation89,Citation91), and emulsifiers for emulsions (e.g., polyethoxylated castor oilCitation73). In some cases, the use of these additives is essential (e.g., microcrystalline cellulose for extrusion-spheronizationCitation89,Citation91), but in most cases, additives are only used to improve the properties of the final product. Since additives should be removed prior to the clinical use of the CaP particles, the advantages related to their use should be balanced with the troubles generated by their removal. Normally, organic remnants are pyrolyzed.Citation72,Citation84,Citation91 The latter strategy is obviously not applicable for hydrated CaP phases such as DCPD and OCP, because these phases decompose well below 500°C. Another possibility is to dissolve them in an appropriate solvent.Citation50,Citation79 However, it is often difficult to get rid of all additives, in particular when dealing with HA. Indeed, precipitated HA crystals are generally very small and hence present very large specific surface areas (typically above 50m2/gCitation110-Citation115). Moreover, HA has excellent adsorption properties, which explains its use in chromatography.Citation116-Citation118
So far, it has been assumed that spherical particles have a homogeneous composition. However, CaP particles obtained at high-temperature are prone to heterogeneities.Citation58 For example, Carayon et al.Citation119 postulated that plasma-sprayed particles are made of three layers: (1) an apatite core, (2) an intermediate layer of calcium oxide, TetCP and tricalcium phosphate, and (3) an outer layer consisting of amorphous calcium phosphate. CaP particles obtained at low temperatures can also present local heterogeneities: Jäger et al.Citation120 showed that precipitated HA nanocrystals consisted of a crystalline core with a calcium to phosphate ratio of 1.67 and an amorphous layer with a calcium to phosphate ratio of 1.00.
Other aspects
Beside the aspects described in , many other aspects might be relevant for a particular clinical indication, such as the mechanical stability or the specific surface area of the spherical particles. A few of these aspects are discussed in the next few lines.
Since this review focused on spherical CaP particles for clinical use, it is relevant to look at their mechanical stability. Unfortunately, the determination of the mechanical properties of spherical particles, particularly of such small diameters, is very difficult and hence hardly performed.Citation90,Citation121 The few attempts of mechanical characterization found in the literature hence still leave room for speculation. It is likely that high-temperature synthesis methods, such as flame-synthesis and plasma melting, provide the mechanically most stable CaP spherical particles because sintering and/or crystallization occurs. Contrarily, consolidation by freeze-drying is expected to produce the most unstable particles.
If the mechanical stability of the spherical particles is too low, three main approaches can be used to reinforce them. First, a binder can be added into the raw materials. Generally, a few weight percents of a polymer are able to give enough stability for handling purposes.Citation84 Second, the particles can be sintered. For example, Paul and SharmaCitation72 sintered chitosan-bonded HA particles at 1100°C for 1h to obtain organic-free phase pure HA particles. Depending on the conditions of sintering, linear shrinkage can easily reach 20–25% and the particle composition may change. The third consolidation method involves the hydraulic or hydrothermal conversion of a CaP phase into another phase. For example, TasCitation92 converted α-TCP spherical agglomerates into apatite particles by incubation in distilled water for 48h at room temperature. Similarly Gonda et al.Citation77 autoclaved α-TCP spherical particles for 20h at 160°C to convert α-TCP grains into interlocked CDHA rods. Very often, several approaches are combined: Takahashai et al.Citation122 produced α-TCP spherical beads with an emulsion route. They used gelatin as a binder, removed it by sintering at 1200°C, and then transformed hydrothermally the α-TCP phase into HA. Here, it is also worth mentioning the work of Day et al.Citation123,Citation124 who first produced spherical glass microspheres and then converted them into hollow apatite spheres by incubation in a phosphate solution.
A large number of studies have been devoted to the synthesis of spherical particles for drug delivery.Citation67,Citation70,Citation74,Citation80,Citation104,Citation125-Citation127 As a result, the internal structure of the particles, such as the porosity, the pore size distribution, or the size distribution of the pore interconnections, is of particular relevance. Various authors have attempted to control it: Descamps et al.Citation84 showed that macroporous CaP spheres could be produced with a controlled fenestration size between the macropores (). Unfortunately, this approach can only work with fairly large spheres (several millimeters in diameter) and CaP phases stable at high temperature (e.g., β-TCP, HA). Similar granule sizes and macropore dimensions were obtained by Lee and ParkCitation76 using double emulsions and by Yang et al.Citation78 using drip casting. At a much lower scale, ≈100nm hollow apatite particles can be obtained by precipitation either withoutCitation112-Citation114,Citation125 or with templates.Citation118,Citation128 Finally, hollow particles can also be produced in an intermediate size range (100–1000µm) using emulsions,Citation81,Citation82 and precipitations.Citation129-Citation131
Figure 5. hierarchical structures obtained using different methods: (A) emulsification (Scale bar: 300µm),Citation81 (B) drip-casting (50µm)Citation70; (C) lost-wax method (3mm)Citation84; (D) precipitation (50µm)Citation129; and (E) emulsification (sphere diameter ≈1.5mm).Citation78 With the exception of (D) (OCP), all particles consist of an apatite (images reproduced with the permission of the authors).
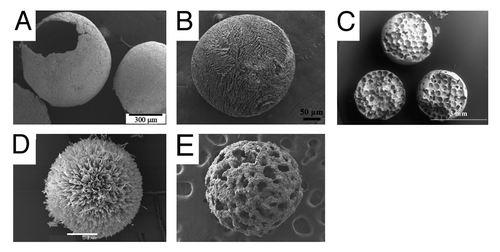
Drug loading is often achieved duringCitation67,Citation80,Citation125,Citation132 or after particle productionCitation70,Citation74,Citation104,Citation127 depending on the drug and the production method. When drug loading is performed during particle production, it is more difficult to control the production and purity of the particles because the system contains more components. When drug loading is performed after particle production, drug absorption into the particle core might be difficult, in particular if the particles are designed for prolonged drug release (small pores).
Drug release is generally controlled by dissolution, diffusion, or surface interactions. In the latter two cases, HA is the most interesting CaP phase because it has often very high specific surface areas, typically above 50m2/g.Citation110-Citation115 However, this implies that the spherical particles must be produced at or close to room temperature, e.g., by precipitation.Citation110-Citation115 Indeed, high temperature processes, e.g., plasma melting or sintering, would strongly reduce the specific surface area of the HA.
As described in the previous paragraphs, the pore size distribution of spherical particles is of prime importance for drug delivery. For orthopedic and dental applications, the focus is set on the space between the particles. Indeed, blood vessels and cells should be able to invade the inter-particular network to promote ceramic resorption and bone formation throughout the particle-filled defect. So, particles should be big enough to promote an easy blood vessel / bone ingrowth but not too large to keep an acceptable resorption time.Citation133 Zhang et al.Citation90 tried to estimate the size of the intergranular space based on the size of the spherical particles. They came to the conclusion that values close to 1 to 2mm are ideal for orthopedic and dental applications.
Use of Spherical CaP Particles
Beside orthopedics and dentistry (), spherical CaP particles are used in very diverse fields of application such as food industry, pharmaceutics, or agriculture (). For the latter applications, very large volumes are produced and sold. So, in terms of the production volume, the use of CaPs in the medical industry can be considered as a niche application. However, the sale prices are much higher than in other applications and the market is rapidly growing.Citation20 Also, the types of CaPs used in orthopedics and dentistry are much more diverse than those used in other fields. Indeed, not only low-temperature but also high temperature phases such as β-TCP, α-TCP or TetCP are used, either as raw materials for hydraulic cements or as bone substitutes.Citation134
Table 3. Applications of spherical calcium phosphate particles in orthopedic and dental surgery
Table 4. Some selected applications of spherical calcium phosphate particles in other fields than orthopedic and dental applications
So far, the number of commercial products based on spherical CaP particles and used in orthopedics and dentistry is still limited (). However, this number is increasing. Moreover, the rapid increase in scientific publications in this field of research () suggests that new commercial products will be launched soon. From the list of medical products given in , only three products are applied in an injectable form. For example, “Radiance,” a product used in aesthetic surgery, contains 30% volume HA in a CMC gel.Citation135,Citation136 Similarly, the orthopedic cement called “chronOS Inject” contains roughly 20% volume β-TCP beads in its paste. Finally, no information about the ceramic volume fraction could be found for another injectable bone substitute called “MBCP Gel.”
Table 5. Non exhaustive list of commercial products containing spherical calcium phosphate particles
The type of application in which CaPs are used depends on the CaPs properties, mainly solubility, acidity, and nanostructure.Citation134 MCP and MCPM are the most soluble and most acidic CaPs. So, MCP/MCPM are used for example as fertilizers in agriculture,Citation137,Citation138 as food additives (e.g., in combination with sodium bicarbonate to make a leavening agentCitation139), and as raw material for hydraulic cements in orthopedics.Citation140,Citation141 DCP and DCPD are neutral and have an intermediate solubility (the human serum is in equilibrium with DCPCitation142). Accordingly, DCP and DCPD are used as excipients for tablets,Citation58,Citation93 as food additives (for example as phosphorus or calcium sourceCitation143), and as bone substitutes.Citation44,Citation109,Citation144,Citation145 CaP materials with slightly larger Ca/P molar ratios (1.33 to 1.67) have been the most extensively used CaPs due to two features: (1) first, their composition and properties are very close to those of bone mineral; (2) second, these materials easily precipitate in the form of nanocrystals. As a result, their specific surface area is very large (typically above 50 m2/g), which is of great interest for chromatography,Citation52,Citation110,Citation113,Citation114,Citation116,Citation117,Citation146,Citation147 drug delivery,Citation79,Citation104,Citation126,Citation127 or heavy ion capture.Citation110,Citation148 Also, nanocrystals have strong van der Waals interactions, hence leading to the formation of very cohesive and viscous aqueous pastes.Citation39 This can be used to thicken soups and yoghurts. TetCP is the most basic and also one of the most soluble CaP (behind MCP and MCPM). Therefore, it is a very good raw material for hydraulic CaP cements.Citation149 To the best of our knowledge, it is not used in other applications than orthopedics and dentistry.
So far, the manuscript has been focused on the synthesis and use of spherical CaP particles. The next paragraphs will address the delivery of the particles. Indeed, the commercial success of a product is often related to its ease of handling. In orthopedics and dentistry, spherical particles are generally mixed with a liquid and then injected. It is therefore crucial to understand the rheological properties of such mixtures, and in particular prevent phase separation. The injectability of CaP-based pastes has been addressed in many articles.Citation40 However, most studies have been devoted to CPCs which are characterized by a mean particle size in the low micrometer range (typically below 10 μm), whereas most CaP particles used for bone graft substitution are much bigger, typically above 100 μm (“milliparticles”). Since many putties and some CPCs consists of CaP milliparticles embedded in a paste, it is essential to better understand the role of milliparticles on the injectability of such pastes. Questions that can be raised are: (1) Beyond what volume fraction of milliparticles is injectability impaired? (2) What is the importance of milliparticle size on injectability? (3) Are the lessons drawn from CPCs valid for pastes made of milliparticles? Tadier et al.Citation42 tried to address some of these questions in a recent study using a model paste consisting of β-TCP microparticles (mean size ≈10 μm), water and glass milliparticles (mean size in the range of 100 to 400 μm). The results confirmed most previous findings made with microparticles. For example, injectability dropped beyond 35–40% milliparticle volume fraction.Citation40 Also, the latter volume fraction threshold decreased with an increase in particle size. Furthermore, the β-TCP and water phases separated at a constant rate during injection.Citation150 Moreover, phase separation was reduced with the replacement of water with a viscous aqueous gel.Citation40 However, a surprising result was observed: the glass milliparticles were extruded faster than the β-TCP microparticles. In other words, not all lessons learned from CPC pastes can be translated to pastes containing milliparticles.
Conclusion
The aim of this article was to review the methods used to produce spherical CaP particles and to look at the use of such particles in the orthopedic and dental fields. Over a dozen of production methods were identified and described. The various methods were classified according to the starting materials (solutions, slurries, pastes, and solids), the dispersion phases (gas, solution, solid), the dispersion tools (nozzles, propellers, sieves, molds), and the consolidation methods (drying, precipitation, gelling, freezing). As a result of this diversity, a broad range of properties can be obtained, for example in terms of particle diameters (10nm to 10millimeters), particle size distributions (very narrow to broad), porosities (low to high), or compositions (all known CaP phases). This review also revealed that there has been a very rapid increase in the number of research articles since 2003. This has resulted in new approaches to better control the production of spherical CaP particles, for example with hierarchical structures or with very narrow particle size distributions.
Acknowledgments
The authors would like to thank L. Galea and J. Thüring for Figure 2a and 2i, and the authors of Figure 5a-5e for the permission to use their photos.
Disclosure of Potential Conflicts of Interest
No potential conflicts of interest were disclosed.
References
- Chau AMT, Mobbs RJ. Bone graft substitutes in anterior cervical discectomy and fusion. Eur Spine J 2009; 18:449 - 64; http://dx.doi.org/10.1007/s00586-008-0878-4; PMID: 19152011
- Bohner M. Resorbable biomaterials as bone graft substitutes. Mater Today 2010; 13:24 - 30; http://dx.doi.org/10.1016/S1369-7021(10)70014-6
- Younger EM, Chapman MW. Morbidity at bone graft donor sites. J Orthop Trauma 1989; 3:192 - 5; http://dx.doi.org/10.1097/00005131-198909000-00002; PMID: 2809818
- Banwart JC, Asher MA, Hassanein RS. Iliac crest bone graft harvest donor site morbidity. A statistical evaluation. Spine (Phila Pa 1976) 1995; 20:1055 - 60; http://dx.doi.org/10.1097/00007632-199505000-00012; PMID: 7631235
- Arrington ED, Smith WJ, Chambers HG, Bucknell AL, Davino NA. Complications of iliac crest bone graft harvesting. Clin Orthop Relat Res 1996; 300 - 9; http://dx.doi.org/10.1097/00003086-199608000-00037; PMID: 8769465
- Hulbert SF, Young FA, Mathews RS, Klawitter JJ, Talbert CD, Stelling FH. Potential of ceramic materials as permanently implantable skeletal prostheses. J Biomed Mater Res 1970; 4:433 - 56; http://dx.doi.org/10.1002/jbm.820040309; PMID: 5469185
- Klawitter JJ, Hulbert SF. Application of porous ceramics for the attachment of load bearing internal orthopedic applications. J Biomed Mater Res Symp 1971; 2:161 - 229; http://dx.doi.org/10.1002/jbm.820050613
- Hench LL. The story of Bioglass. J Mater Sci Mater Med 2006; 17:967 - 78; http://dx.doi.org/10.1007/s10856-006-0432-z; PMID: 17122907
- Hutmacher DW, Schantz JT, Lam CX, Tan KC, Lim TC. State of the art and future directions of scaffold-based bone engineering from a biomaterials perspective. J Tissue Eng Regen Med 2007; 1:245 - 60; http://dx.doi.org/10.1002/term.24; PMID: 18038415
- Coombes AG, Meikle MC. Resorbable synthetic polymers as replacements for bone graft. Clin Mater 1994; 17:35 - 67; http://dx.doi.org/10.1016/0267-6605(94)90046-9; PMID: 10150176
- Bansiddhi A, Sargeant TD, Stupp SI, Dunand DC. Porous NiTi for bone implants: a review. Acta Biomater 2008; 4:773 - 82; http://dx.doi.org/10.1016/j.actbio.2008.02.009; PMID: 18348912
- Witte F. The history of biodegradable magnesium implants: a review. Acta Biomater 2010; 6:1680 - 92; http://dx.doi.org/10.1016/j.actbio.2010.02.028; PMID: 20172057
- Daculsi G, LeGeros RZ, Nery E, Lynch K, Kerebel B. Transformation of biphasic calcium phosphate ceramics in vivo: ultrastructural and physicochemical characterization. J Biomed Mater Res 1989; 23:883 - 94; http://dx.doi.org/10.1002/jbm.820230806; PMID: 2777831
- Driessens FC, Planell JA, Boltong MG, Khairoun I, Ginebra MP. Osteotransductive bone cements. Proc Inst Mech Eng H 1998; 212:427 - 35; http://dx.doi.org/10.1243/0954411981534196; PMID: 9852738
- LeGeros RZ. Properties of osteoconductive biomaterials: calcium phosphates. Clin Orthop Relat Res 2002; 81 - 98; http://dx.doi.org/10.1097/00003086-200202000-00009; PMID: 11937868
- Barradas AM, Yuan H, van Blitterswijk CA, Habibovic P. Osteoinductive biomaterials: current knowledge of properties, experimental models and biological mechanisms. Eur Cell Mater 2011; 21:407 - 29, discussion 429; PMID: 21604242
- Le Nihouannen D, Daculsi G, Saffarzadeh A, Gauthier O, Delplace S, Pilet P, et al. Ectopic bone formation by microporous calcium phosphate ceramic particles in sheep muscles. Bone 2005; 36:1086 - 93; http://dx.doi.org/10.1016/j.bone.2005.02.017; PMID: 15869915
- Ripamonti U. The morphogenesis of bone in replicas of porous hydroxyapatite obtained from conversion of calcium carbonate exoskeletons of coral. J Bone Joint Surg Am 1991; 73:692 - 703; PMID: 1675218
- Habibovic P, de Groot K. Osteoinductive biomaterials--properties and relevance in bone repair. J Tissue Eng Regen Med 2007; 1:25 - 32; http://dx.doi.org/10.1002/term.5; PMID: 18038389
- Mendenhall Associates I. Bone grafts and bone substitutes. Orthopedic Network News 2008; 19:18 - 21
- Dupraz A, Delécrin J, Moreau A, Pilet P, Passuti N. Long-term bone response to particulate injectable ceramic. J Biomed Mater Res 1998; 42:368 - 75; http://dx.doi.org/10.1002/(SICI)1097-4636(19981205)42:3<368::AID-JBM4>3.0.CO;2-H; PMID: 9788498
- van Hemert WL, Willems K, Anderson PG, van Heerwaarden RJ, Wymenga AB. Tricalcium phosphate granules or rigid wedge preforms in open wedge high tibial osteotomy: a radiological study with a new evaluation system. Knee 2004; 11:451 - 6; http://dx.doi.org/10.1016/j.knee.2004.08.004; PMID: 15581763
- Steffen T, Stoll T, Arvinte T, Schenk RK. Porous tricalcium phosphate and transforming growth factor used for anterior spine surgery. Eur Spine J 2001; 10:Suppl 2 S132 - 40; http://dx.doi.org/10.1007/s005860100325; PMID: 11716010
- Mastrogiacomo M, Muraglia A, Komlev V, Peyrin F, Rustichelli F, Crovace A, et al. Tissue engineering of bone: search for a better scaffold. Orthod Craniofac Res 2005; 8:277 - 84; http://dx.doi.org/10.1111/j.1601-6343.2005.00350.x; PMID: 16238608
- Constantz BR, Ison IC, Fulmer MT, Poser RD, Smith ST, VanWagoner M, et al. Skeletal repair by in situ formation of the mineral phase of bone. Science 1995; 267:1796 - 9; http://dx.doi.org/10.1126/science.7892603; PMID: 7892603
- Morgan JP, Dauskardt RH. Notch strength insensitivity of self-setting hydroxyapatite bone cements. J Mater Sci Mater Med 2003; 14:647 - 53; http://dx.doi.org/10.1023/A:1024035426820; PMID: 15348429
- Bohner M, Gbureck U, Barralet JE. Technological issues for the development of more efficient calcium phosphate bone cements: a critical assessment. Biomaterials 2005; 26:6423 - 9; http://dx.doi.org/10.1016/j.biomaterials.2005.03.049; PMID: 15964620
- Grafe IA, Baier M, Nöldge G, Weiss C, Da Fonseca K, Hillmeier J, et al. Calcium-phosphate and polymethylmethacrylate cement in long-term outcome after kyphoplasty of painful osteoporotic vertebral fractures. Spine (Phila Pa 1976) 2008; 33:1284 - 90; http://dx.doi.org/10.1097/BRS.0b013e3181714a84; PMID: 18469705
- Maestretti G, Cremer C, Otten P, Jakob RP. Prospective study of standalone balloon kyphoplasty with calcium phosphate cement augmentation in traumatic fractures. Eur Spine J 2007; 16:601 - 10; http://dx.doi.org/10.1007/s00586-006-0258-x; PMID: 17120071
- Bohner M. Design of ceramic-based cements and putties for bone graft substitution. Eur Cell Mater 2010; 20:1 - 12; PMID: 20574942
- Tao J, Jiang W, Zhai H, Pan H, Xu R, Tang R. Structural components and anisotropic dissolution behaviors in one hexagonal single crystal of β-tricalcium phosphate. Cryst Growth Des 2008; 8:2227 - 34; http://dx.doi.org/10.1021/cg700808h
- Kwon SG, Hyeon T. Formation mechanisms of uniform nanocrystals via hot-injection and heat-up methods. Small 2011; 7:2685 - 702; http://dx.doi.org/10.1002/smll.201002022; PMID: 21805623
- Kilian O, Wenisch S, Heiss C, Horas U, Dingeldein E, Schnettler R. OSTIM-Einfluss kombiniert mit autologem thrombozytären Wachstumfaktoren auf die Knochendefektheilung in vivo. Biomaterialien 2002; 3:126 - 32
- Kilian O, Wenisch S, Heiss C, Horas U, Dingeldein E, Schnettler R. Einfluss von OSTIM kombiniert mit autologem thrombozytären Wachstumsfaktoren. Biomaterialien 2002; 3:70 - 3
- Lamer VK, Dinegar RH. Theory, production and mechanism of formation of monodispersed hydrosols. J Am Chem Soc 1950; 72:4847 - 54; http://dx.doi.org/10.1021/ja01167a001
- Gauthier O, Goyenvalle E, Bouler JM, Guicheux J, Pilet P, Weiss P, et al. Macroporous biphasic calcium phosphate ceramics versus injectable bone substitute: a comparative study 3 and 8 weeks after implantation in rabbit bone. J Mater Sci Mater Med 2001; 12:385 - 90; http://dx.doi.org/10.1023/A:1011284517429; PMID: 15348276
- Klein CP, van der Lubbe HB, de Groot K. A plastic composite of alginate with calcium phosphate granulate as implant material: an in vivo study. Biomaterials 1987; 8:308 - 10; PMID: 3663809
- Sullivan F.. European Markets for bone grafts and bone. 2008.
- Bohner M, Doebelin N, Baroud G. Theoretical and experimental approach to test the cohesion of calcium phosphate pastes. Eur Cell Mater 2006; 12:26 - 35; PMID: 16941384
- Bohner M, Baroud G. Injectability of calcium phosphate pastes. Biomaterials 2005; 26:1553 - 63; http://dx.doi.org/10.1016/j.biomaterials.2004.05.010; PMID: 15522757
- Fatimi A, Tassin JF, Bosco J, Deterre R, Axelos MAV, Weiss P. Injection of calcium phosphate pastes: prediction of injection force and comparison with experiments. J Mater Sci Mater Med 2012; 23:1593 - 603; http://dx.doi.org/10.1007/s10856-012-4640-4; PMID: 22528073
- Tadier S, Galea L, Gruenenfelder S, Bohner M. Investigation of the phase separation occurring during the injection of β-tricalcium phosphate -Water -Glass beads pastes. Key Eng Mater 2012; 493-494:693 - 7; http://dx.doi.org/10.4028/www.scientific.net/KEM.493-494.693
- Gbureck U, Barralet JE, Radu L, Klinger HG, Thull R. Amorphous alpha-tricalcium phosphate: Preparation and aqueous setting reaction. J Am Ceram Soc 2004; 87:1126 - 32; http://dx.doi.org/10.1111/j.1551-2916.2004.01126.x
- Gbureck U, Hölzel T, Klammert U, Würzler K, Müller FA, Barralet JE. Resorbable Dicalcium Phosphate Bone Substitutes Prepared by 3D Powder Printing. Adv Funct Mater 2007; 17:3940 - 5; http://dx.doi.org/10.1002/adfm.200700019
- Ishikawa K. Effects of spherical tetracalcium phosphate on injectability and basic properties of apatitic cement. Key Eng Mater 2003; 240-2:369 - 72; http://dx.doi.org/10.4028/www.scientific.net/KEM.240-242.369
- Ishikawa K, Matsuya S, Nakagawa M, Udoh K, Suzuki K. Basic properties of apatite cement containing spherical tetracalcium phosphate made with plasma melting method. J Mater Sci Mater Med 2004; 15:13 - 7; http://dx.doi.org/10.1023/B:JMSM.0000010092.01661.a8; PMID: 15338586
- Han Y, Li S, Wang X. A novel thermolysis method of colloidal protein precursors to prepare hydroxyapatite nanocrystals. Cryst Res Technol 2009; 44:336 - 40; http://dx.doi.org/10.1002/crat.200800404
- Qiu CF, Xiao XF, Liu RF, She HD. Biomimetic synthesis of spherical nano-hydroxyapatite with polyvinylpyrrolidone as template. Mater Sci Technol 2008; 24:612 - 7; http://dx.doi.org/10.1179/174328407X176974
- Yamane S, Sugawara A, Watanabe A, Akiyoshi K. Hybrid nanoapatite by polysaccharide nanogel-templated mineralization. J Bioact Compat Polym 2009; 24:151 - 68; http://dx.doi.org/10.1177/0883911508101554
- Singh S, Singh V, Aggarwal S, Mandal UK. Synthesis of brushite nanoparticles at different temperatures. Chem Papers 2010; 64:491 - 8; http://dx.doi.org/10.2478/s11696-010-0032-8
- Zhou WY, Wang M, Cheung WL, Guo BC, Jia DM. Synthesis of carbonated hydroxyapatite nanospheres through nanoemulsion. J Mater Sci Mater Med 2008; 19:103 - 10; http://dx.doi.org/10.1007/s10856-007-3156-9; PMID: 17577636
- Luo P, Nieh TG. Synthesis of ultrafine hydroxyapatite particles by a spray dry method. Mater Sci Eng C 1995; 3:75 - 8; http://dx.doi.org/10.1016/0928-4931(95)00089-5
- Brunner TJ, Bohner M, Dora C, Gerber C, Stark WJ. Comparison of amorphous TCP nanoparticles to micron-sized alpha-TCP as starting materials for calcium phosphate cements. J Biomed Mater Res B Appl Biomater 2007; 83:400 - 7; http://dx.doi.org/10.1002/jbm.b.30809; PMID: 17410573
- Brunner TJ, Bohner M, Grass RN, Stark WJ. Effect of particle size, crystal phase and crystallinity on the reactivity of tricalcium phosphate cements for bone reconstitution. J Mater Chem 2007; 17:4072 - 8; http://dx.doi.org/10.1039/b707171j
- Loher S, Stark WJ, Maciejewski M, Baiker A, Pratsinis SE, Reichardt D, et al. Fluoro-apatite and calcium phosphate nanoparticles by flame synthesis. Chem Mater 2005; 17:36 - 42; http://dx.doi.org/10.1021/cm048776c
- Tao J, Pan H, Zhai H, Wang J, Li L, Wu J, et al. Controls of tricalcium phosphate single-crystal formation from its amorphous precursor by interfacial energy. Cryst Growth Des 2009; 9:3154 - 60; http://dx.doi.org/10.1021/cg801130w
- Cho JS, Ko YN, Koo HY, Kang YC. Synthesis of nano-sized biphasic calcium phosphate ceramics with spherical shape by flame spray pyrolysis. J Mater Sci Mater Med 2010; 21:1143 - 9; http://dx.doi.org/10.1007/s10856-009-3980-1; PMID: 20052521
- Cho JS, Rhee SH. Preparation of submicron-sized hydroxyapatite powders by spray pyrolysis. Key Eng Mater 2012; 493-494:215 - 8; http://dx.doi.org/10.4028/www.scientific.net/KEM.493-494.215
- Mohn D, Doebelin N, Tadier S, Bernabei RE, Luechinger NA, Stark WJ, et al. Reactivity of calcium phosphate nanoparticles prepared by flame spray synthesis as precursors for calcium phosphate cements. J Mater Chem 2011; 21:13963 - 72; http://dx.doi.org/10.1039/c1jm11977j
- Trommer RM, Santos LA, Bergmann CP. Nanostructured hydroxyapatite powders produced by a flame-based technique. Mater Sci Eng C 2009; 29:1770 - 5; http://dx.doi.org/10.1016/j.msec.2009.02.006
- Hirai T, Hodono M, Komasawa I. Preparation of spherical calcium phosphate fine particles using an emulsion liquid membrane system. Langmuir 2000; 16:955 - 60; http://dx.doi.org/10.1021/la990590i
- Barrias CC, Ribeiro CC, Barbosa MA. Adhesion and Proliferation of Human Osteoblastic Cells Seeded on Injectable Hydroxyapatite Microspheres. In: Barbosa MA, Monteiro FJ, Correia R, Leon B, eds. Porto, 2004:877-80.
- Fabbri M, Celotti GC, Ravaglioli A. Granulates based on calcium phosphate with controlled morphology and porosity for medical applications: physico-chemical parameters and production technique. Biomaterials 1994; 15:474 - 7; http://dx.doi.org/10.1016/0142-9612(94)90228-3; PMID: 8080940
- Liu DM. Fabrication and characterization of porous hydroxyapatite granules. Biomaterials 1996; 17:1955 - 7; http://dx.doi.org/10.1016/0142-9612(95)00301-0; PMID: 8894087
- Mateus AYP, Barrias CC, Ribeiro C, Ferraz MP, Monteiro FJ. Comparative study of nanohydroxyapatite microspheres for medical applications. J Biomed Mater Res A 2008; 86:483 - 93; http://dx.doi.org/10.1002/jbm.a.31634; PMID: 17975824
- Oliveira SM, Barrias CC, Ribeiro CC, Almeida IF, Bahia MF, Barbosa MA. Morphology and mechanical properties of injectable ceramic microspheres. Key Eng Mater 2009; 396-398:691 - 4; http://dx.doi.org/10.4028/www.scientific.net/KEM.396-398.691
- Ribeiro CC, Barrias CC, Barbosa MA. Calcium phosphate-alginate microspheres as enzyme delivery matrices. Biomaterials 2004; 25:4363 - 73; http://dx.doi.org/10.1016/j.biomaterials.2003.11.028; PMID: 15046927
- Ribeiro CC, Barrias CC, Barbosa MA. Preparation and characterisation of calcium-phosphate porous microspheres with a uniform size for biomedical applications. J Mater Sci Mater Med 2006; 17:455 - 63; http://dx.doi.org/10.1007/s10856-006-8473-x; PMID: 16688586
- Rodríguez-Rivero C, del Valle EMM, Galán MA. Development of a new technique to generate microcapsules from the breakup of non-Newtonian highly viscous fluid jets. AIChE J 2011; 57:3436 - 47; http://dx.doi.org/10.1002/aic.12549
- Son JS, Lee KB, Kim SG, Kwon TY, Kim KH. Porous calcium phosphate granules containing drug-loaded polymeric nanoparticles for bone regeneration. Mater Lett 2012; 76:243 - 6; http://dx.doi.org/10.1016/j.matlet.2012.02.114
- Tuyen DV, Lee BT. Formation and characterization of porous spherical biphasic calcium phosphate (BCP) granules using PCL. Ceram Int 2011; 37:2043 - 9; http://dx.doi.org/10.1016/j.ceramint.2011.02.012
- Paul W, Sharma CP. Development of porous spherical hydroxyapatite granules: application towards protein delivery. J Mater Sci Mater Med 1999; 10:383 - 8; http://dx.doi.org/10.1023/A:1008918412198; PMID: 15348122
- Bohner M. Calcium phosphate emulsions: possible applications. Key Eng Mater 2001; 192-195:765 - 8; http://dx.doi.org/10.4028/www.scientific.net/KEM.192-195.765
- Komlev VS, Barinov SM, Koplik EV. A method to fabricate porous spherical hydroxyapatite granules intended for time-controlled drug release. Biomaterials 2002; 23:3449 - 54; http://dx.doi.org/10.1016/S0142-9612(02)00049-2; PMID: 12099288
- Pradeesh TS, Sunny MD, Varma HK, Ramesh P. Preparation of microstructured hydroxyapatite microspheres using oil in water emulsions. Bull Mater Sci 2005; 28:383 - 90; http://dx.doi.org/10.1007/BF02711223
- Lee JS, Park JK. Processing of porous ceramic spheres by pseudo-double-emulsion method. Ceram Int 2003; 29:271 - 8; http://dx.doi.org/10.1016/S0272-8842(02)00115-3
- Gonda Y, Ioku K, Shibata Y, Okuda T, Kawachi G, Kamitakahara M, et al. Stimulatory effect of hydrothermally synthesized biodegradable hydroxyapatite granules on osteogenesis and direct association with osteoclasts. Biomaterials 2009; 30:4390 - 400; http://dx.doi.org/10.1016/j.biomaterials.2009.05.002; PMID: 19481798
- Yang JH, Kim KH, You CK, Rautray TR, Kwon TY. Synthesis of spherical hydroxyapatite granules with interconnected pore channels using camphene emulsion. J Biomed Mater Res B Appl Biomater 2011; 99:150 - 7; http://dx.doi.org/10.1002/jbm.b.31882; PMID: 21714080
- Chen J, Luo Y, Hong L, Ling Y, Pang J, Fang Y, et al. Synthesis, characterization and osteoconductivity properties of bone fillers based on alendronate-loaded poly(ε-caprolactone)/hydroxyapatite microspheres. J Mater Sci Mater Med 2011; 22:547 - 55; http://dx.doi.org/10.1007/s10856-011-4232-8; PMID: 21318627
- Kim CW, Yun YP, Lee HJ, Hwang YS, Kwon IK, Lee SC. In situ fabrication of alendronate-loaded calcium phosphate microspheres: controlled release for inhibition of osteoclastogenesis. J Control Release 2010; 147:45 - 53; http://dx.doi.org/10.1016/j.jconrel.2010.06.016; PMID: 20600398
- Lee HH, Hong SJ, Kim CH, Kim EC, Jang JH, Shin HI, et al. Preparation of hydroxyapatite spheres with an internal cavity as a scaffold for hard tissue regeneration. J Mater Sci Mater Med 2008; 19:3029 - 34; http://dx.doi.org/10.1007/s10856-008-3435-0; PMID: 18389344
- Park JS, Hong SJ, Kim HY, Yu HS, Lee YI, Kim CH, et al. Evacuated calcium phosphate spherical microcarriers for bone regeneration. Tissue Eng Part A 2010; 16:1681 - 91; http://dx.doi.org/10.1089/ten.tea.2009.0624; PMID: 19954329
- Teraoka E, Kato K. Production of alpha-TCP ceramic precision spheres for mosaic-like ceramics fabrication use. Bioceram Dev Appl 2011; 1:1 - 3; http://dx.doi.org/10.4303/bda/D101124
- Descamps M, Hornez JC, Leriche A. Manufacture of hydroxyapatite beads for medical applications. J Eur Ceram Soc 2009; 29:369 - 75; http://dx.doi.org/10.1016/j.jeurceramsoc.2008.06.008
- Meurice E, Leriche A, Hornez JC, Bouchart F, Rguiti E, Boilet L, et al. Functionalisation of porous hydroxyapatite for bone substitutes. J Eur Ceram Soc 2012; 32:2673 - 8; http://dx.doi.org/10.1016/j.jeurceramsoc.2012.01.014
- Bouyer E, Gitzhofer F, Boulos MI. Suspension plasma spraying for hydroxyapatite powder preparation by RF plasma. IEEE Trans Plasma Sci 1997; 25:1066 - 72; http://dx.doi.org/10.1109/27.649627
- Bouyer E, Gitzhofer F, Boulos MI. The suspension plasma spraying of bioceramics by induction plasma. JOM 1997; 49:58 - 62; http://dx.doi.org/10.1007/BF02915483
- Bouyer E, Gitzhofer F, Boulos MI. Morphological study of hydroxyapatite nanocrystal suspension. J Mater Sci Mater Med 2000; 11:523 - 31; http://dx.doi.org/10.1023/A:1008918110156; PMID: 15348004
- Jalal IM, Malinowski HJ, Smith WE. Tablet granulations composed of spherical-shaped particles. J Pharm Sci 1972; 61:1466 - 8; http://dx.doi.org/10.1002/jps.2600610926; PMID: 5068953
- Zhang X, Chem J, Zhou J, Feng J, Li C, Chen A, et al. Porous hydroxyapatite granules: Their synthesis, application and characterisation. Clin Mater 1989; 4:319 - 27; http://dx.doi.org/10.1016/0267-6605(89)90012-7
- Cortez PP, Atayde LM, Silva MA, Armada-da-Silva P, Fernandes MH, Afonso A, et al. Characterization and preliminary in vivo evaluation of a novel modified hydroxyapatite produced by extrusion and spheronization techniques. J Biomed Mater Res B Appl Biomater 2011; 99:170 - 9; http://dx.doi.org/10.1002/jbm.b.31884; PMID: 21714082
- Tas AC. Preparation of porous apatite granules from calcium phosphate cement. J Mater Sci Mater Med 2008; 19:2231 - 9; http://dx.doi.org/10.1007/s10856-007-3326-9; PMID: 18049869
- Dukić-Ott A, Thommes M, Remon JP, Kleinebudde P, Vervaet C. Production of pellets via extrusion-spheronisation without the incorporation of microcrystalline cellulose: a critical review. Eur J Pharm Biopharm 2009; 71:38 - 46; http://dx.doi.org/10.1016/j.ejpb.2008.08.005; PMID: 18771727
- Khor KA, Cheang P. Plasma sprayed hydroxyapatite(HA) coatings produced with flame spheroidised powders. J Mater Proc Tech 1997; 63:271 - 6; http://dx.doi.org/10.1016/S0924-0136(96)02634-9
- Khor KA, Cheang P, Wang Y. Plasma Spraying of Combustion Flame Spheroidized Hydroxyapatite (HA) Powders. J Therm Spray Tech 1998; 7:254 - 60; http://dx.doi.org/10.1361/105996398770351007
- Kweh SWK, Khor KA, Cheang P. Production and characterization of hydroxyapatite (HA) powders. J Mater Proc Tech 1999; 89-90:373 - 7; http://dx.doi.org/10.1016/S0924-0136(99)00061-8
- Weng J, Wang M, Chen J. Plasma-sprayed calcium phosphate particles with high bioactivity and their use in bioactive scaffolds. Biomaterials 2002; 23:2623 - 9; http://dx.doi.org/10.1016/S0142-9612(01)00393-3; PMID: 12059011
- Hollister SJ. Porous scaffold design for tissue engineering. Nat Mater 2005; 4:518 - 24; http://dx.doi.org/10.1038/nmat1421; PMID: 16003400
- Butscher A, Bohner M, Hofmann S, Gauckler L, Müller R. Structural and material approaches to bone tissue engineering in powder-based three-dimensional printing. Acta Biomater 2011; 7:907 - 20; http://dx.doi.org/10.1016/j.actbio.2010.09.039; PMID: 20920616
- Gbureck U, Hölzel T, Doillon CJ, Müller FA, Barralet JE. Direct printing of bioceramics implants with spatially localized angiogenic factors. Adv Mater 2007; http://dx.doi.org/10.1002/adma.200601370
- Butscher A, Bohner M, Roth C, Ernstberger A, Heuberger R, Doebelin N, et al. Printability of calcium phosphate powders for three-dimensional printing of tissue engineering scaffolds. Acta Biomater 2012; 8:373 - 85; http://dx.doi.org/10.1016/j.actbio.2011.08.027; PMID: 21925623
- Göbel C, Simon P, Buder J, Tlatlik H, Kniep R. Phase formation and morphology of calcium phosphate-gelatine-composites grown by double diffusion technique: The influence of fluoride. J Mater Chem 2004; 14:2225 - 30; http://dx.doi.org/10.1039/b403503h
- Teng S, Shi J, Chen L. Formation of calcium phosphates in gelatin with a novel diffusion system. Colloids Surf B Biointerfaces 2006; 49:87 - 92; http://dx.doi.org/10.1016/j.colsurfb.2006.03.005; PMID: 16621478
- Hong MH, Son JS, Kim KM, Han M, Oh DS, Lee YK. Drug-loaded porous spherical hydroxyapatite granules for bone regeneration. J Mater Sci Mater Med 2011; 22:349 - 55; http://dx.doi.org/10.1007/s10856-010-4197-z; PMID: 21222142
- Andrianjatovo H, Jose F, Lemaitre J. Effect of beta-TCPgranularity on setting time and strength of calcium phosphate hydraulic cements. J Mater Sci Mater Med 1996; 7:34 - 9; http://dx.doi.org/10.1007/BF00121187
- Moseke C, Bayer C, Vorndran E, Barralet JE, Groll J, Gbureck U. Low temperature fabrication of spherical brushite granules by cement paste emulsion. J Mater Sci Mater Med 2012; 23:2631 - 7; http://dx.doi.org/10.1007/s10856-012-4740-1; PMID: 22903599
- Jiao Y, Lu YP, Xiao GY, Xu WH, Zhu RF. Preparation and characterization of hollow hydroxyapatite microspheres by the centrifugal spray drying method. Powder Technol 2012; 217:581 - 4; http://dx.doi.org/10.1016/j.powtec.2011.11.025
- Nomura S, Tsuru K, Matsuya S, Takahashi I, Ishikawa K. Fabrication of spherical carbonate apatite using calcium sulfate as a precursor by W/O emulsion method. Key Eng Mater 2013; 529-530:78 - 81; http://dx.doi.org/10.4028/www.scientific.net/KEM.529-530.78
- Galea LG, Bohner M, Lemaître J, Kohler T, Müller R. Bone substitute: transforming β-tricalcium phosphate porous scaffolds into monetite. Biomaterials 2008; 29:3400 - 7; http://dx.doi.org/10.1016/j.biomaterials.2008.04.041; PMID: 18495242
- Aizawa M, Terado T, Howell FS, Itatani K. Preparation of spherical apatite particles by the homogeneous precipitation method in the presence of magnesium ions and their ion-exchange properties. Mater Res Bull 1999; 34:1215 - 25; http://dx.doi.org/10.1016/S0025-5408(99)00118-X
- Qiang Fu, Rahaman MN, Nai Zhou, Wenhai Huang, Deping Wang, Liying Zhang, et al. In vitro study on different cell response to spherical hydroxyapatite nanoparticles. J Biomater Appl 2008; 23:37 - 50; http://dx.doi.org/10.1177/0885328207081350; PMID: 18194997
- Kandori K, Noguchi Y, Fukusumi M, Morisada Y. Preparation of spherical and balloonlike calcium phosphate particles from forced hydrolysis of Ca(OH) 2-Triphosphate solution and their adsorption selectivity of water. J Phys Chem C 2010; 114:6440 - 5; http://dx.doi.org/10.1021/jp100829f
- Kandori K, Tada K, Fukusumi M, Morisada Y. Preparation and characterization of spherical and balloon-like calcium phosphate particles. Bull Chem Soc Jpn 2008; 81:1567 - 74; http://dx.doi.org/10.1246/bcsj.81.1567
- Kandori K, Takeguchi K, Fukusumi M, Morisada Y. Preparation and characterization of calcium hydroxyapatite and balloon-like calcium phosphate particles from forced hydrolysis of Ca(OH)2-triphosphate solution. Polyhedron 2009; 28:3036 - 42; http://dx.doi.org/10.1016/j.poly.2009.06.023
- Toyama T, Yoshida H, Kojima Y, Nishimiya N. Morphological control of hydroxyapatite precipitate from high-concentration hydroxyapatite solution by microwave irradiation. IOP Conf Series: Mater Sci Eng 2011;18:052002.
- Kadoya T, Isobe T, Ebihara M, Ogawa T, Sumita M, Kuwahara H, et al. New spherical hydroxyapatite for high performance liquid chromatography of proteins. J Liquid Chrom 1986; 9:3543 - 57; http://dx.doi.org/10.1080/01483918608077803
- Aj W, Yp L, Rf Z, St L, Xl M. Effect of process parameters on the performance of spray dried hydroxyapatite microspheres. Powder Technol 2009; 191:1 - 6; http://dx.doi.org/10.1016/j.powtec.2008.10.020
- Yeo CH, Zein SHS, Ahmad AL, McPhail DS. Comparison of DOPA and DPPA liposome templates for the synthesis of calcium phosphate nanoshells. Ceram Int 2012; 38:561 - 70; http://dx.doi.org/10.1016/j.ceramint.2011.07.044
- Carayon MT, Lacout JL. Study of the Ca/P atomic ratio of the amorphous phase in plasma-sprayed hydroxyapatite coatings. J Solid State Chem 2003; 172:339 - 50; http://dx.doi.org/10.1016/S0022-4596(02)00085-3
- Jäger C, Welzel T, Meyer-Zaika W, Epple M. A solid-state NMR investigation of the structure of nanocrystalline hydroxyapatite. Magn Reson Chem 2006; 44:573 - 80; http://dx.doi.org/10.1002/mrc.1774; PMID: 16395729
- Hsu YH, Turner IG, Miles AW. Fabrication and mechanical testing of porous calcium phosphate bioceramic granules. J Mater Sci Mater Med 2007; 18:1931 - 7; http://dx.doi.org/10.1007/s10856-007-3128-0; PMID: 17554596
- Takahashai T, Kamitakahara M, Kawachi G, Ioku K. Preparation of spherical porous granules composed of rod-shaped hydroxyapatite and evaluation of their protein adsorption properties. Key Eng Mater 2008; 361-363:83 - 6; http://dx.doi.org/10.4028/www.scientific.net/KEM.361-363.83
- Fu H, Rahaman MN, Day DE. Effect of process variables on the microstructure of hollow hydroxyapatite microspheres prepared by a glass conversion method. J Am Ceram Soc 2010; 93:3116 - 23; http://dx.doi.org/10.1111/j.1551-2916.2010.03833.x; PMID: 21892226
- Fu H, Rahaman MN, Day DE, Brown RF. Hollow hydroxyapatite microspheres as a device for controlled delivery of proteins. J Mater Sci Mater Med 2011; 22:579 - 91; http://dx.doi.org/10.1007/s10856-011-4250-6; PMID: 21290170
- Cai Y, Pan H, Xu R, Hu Q, Li N, Tang R. Ultrasonic controlled morphology transformation of hollow calcium phosphate nanospheres: A smart and biocompatible drug release system. Chem Mater 2007; 19:3081 - 3; http://dx.doi.org/10.1021/cm070298t
- Santos C, Martins MA, Franke RP, Almeida MM, Costa MEV. Calcium phosphate granules for use as a 5-Fluorouracil delivery system. Ceram Int 2009; 35:1587 - 94; http://dx.doi.org/10.1016/j.ceramint.2008.08.015
- Tomoda K, Ariizumi H, Nakaji T, Makino K. Hydroxyapatite particles as drug carriers for proteins. Colloids Surf B Biointerfaces 2010; 76:226 - 35; http://dx.doi.org/10.1016/j.colsurfb.2009.10.039; PMID: 19939646
- Zhang H, Zhou K, Li Z, Huang S. Synthesis of hollow hybrid hydroxyapatite microspheres based on chitosan-poly(acrylic acid) microparticles. Biomed Mater 2009; 4:031002; http://dx.doi.org/10.1088/1748-6041/4/3/031002; PMID: 19372618
- Bigi A, Boanini E, Walsh D, Mann S. Morphosynthesis of octacalcium phosphate hollow microspheres by polyelectrolyte-mediated crystallization. Ang Chem Int Ed 2002; 41:2163 - 6
- Bigi A, Bracci B, Panzavolta S, Iliescu M, Plouet-Richard M, Werckmann J, et al. Morphological and Structural Modifications of Octacalcium Phosphate Induced by Poly-L-Aspartate. Cryst Growth Des 2004; 4:141 - 6; http://dx.doi.org/10.1021/cg034078d
- Yang X, Zhang L, Chen X, Yang G, Zhang L, Gao C, et al. Trace element-incorporating octacalcium phosphate porous beads via polypeptide-assisted nanocrystal self-assembly for potential applications in osteogenesis. Acta Biomater 2012; 8:1586 - 96; http://dx.doi.org/10.1016/j.actbio.2011.12.012; PMID: 22200612
- Santos C, Rovath CF, Franke RP, Almeida MM, Costa MEV. Spray-dried hydroxyapatite-5-Fluorouracil granules as a chemotherapeutic delivery system. Ceram Int 2009; 35:509 - 13; http://dx.doi.org/10.1016/j.ceramint.2008.01.012
- Bohner M, Baumgart F. Theoretical model to determine the effects of geometrical factors on the resorption of calcium phosphate bone substitutes. Biomaterials 2004; 25:3569 - 82; http://dx.doi.org/10.1016/j.biomaterials.2003.10.032; PMID: 15020131
- Bohner M. Calcium orthophosphates in medicine: from ceramics to calcium phosphate cements. Injury 2000; 31:Suppl 4 37 - 47; http://dx.doi.org/10.1016/S0020-1383(00)80022-4; PMID: 11270080
- Lemperle G, Morhenn V, Charrier U. Human histology and persistence of various injectable filler substances for soft tissue augmentation. Aesthetic Plast Surg 2003; 27:354 - 66, discussion 367; http://dx.doi.org/10.1007/s00266-003-3022-1; PMID: 14648064
- Busso M.. Cosmetic procedure: Soft tissue augmentation with radiance FN. 2003; 11 - 3
- Prochnow LI, Chien SH, Carmona G, Dillard EF, Henao J, Austin ER. Plant availability of phosphorus in four superphosphate fertilizers varying in water-insoluble phosphate compounds. Soil Sci Soc Am J 2008; 72:462 - 70; http://dx.doi.org/10.2136/sssaj2006.0421
- Toor GS. Enhancing phosphorus availability in low-phosphorus soils by using poultry manure and commercial fertilizer. Soil Sci 2009; 174:358 - 64; http://dx.doi.org/10.1097/SS.0b013e3181a7e716
- Cepeda M, Waniska RD, Rooney LW, Bejosano FP. Effects of leavening acids and dough temperature in wheat flour tortillas. Cereal Chem 2000; 77:489 - 94; http://dx.doi.org/10.1094/CCHEM.2000.77.4.489
- Lemaitre J, Mirtchi A, Mortier A. Calcium phosphate cements for medical use: state of the art and perspectives of development. Silicates Ind 1987; 9-10:141 - 6
- Fulmer M, Brown PW. Effects of temperature on the formation of hydroxyapatite. J Mater Res 1993; 8:1687 - 96; http://dx.doi.org/10.1557/JMR.1993.1687
- Bohner M, Lemaitre J. Can bioactivity be tested in vitro with SBF solution?. Biomaterials 2009; 30:2175 - 9; http://dx.doi.org/10.1016/j.biomaterials.2009.01.008; PMID: 19176246
- Petersen GI, Stein HH. Novel procedure for estimating endogenous losses and measurement of apparent and true digestibility of phosphorus by growing pigs. J Anim Sci 2006; 84:2126 - 32; http://dx.doi.org/10.2527/jas.2005-479; PMID: 16864873
- Habibovic P, Gbureck U, Doillon CJ, Bassett DC, van Blitterswijk CA, Barralet JE. Osteoconduction and osteoinduction of low-temperature 3D printed bioceramic implants. Biomaterials 2008; 29:944 - 53; http://dx.doi.org/10.1016/j.biomaterials.2007.10.023; PMID: 18055009
- Munting E, Mirtchi A, Lemaître J. Bone repair of defects filled with a phosphocalcic hydraulic cement: and in vivo study. J Mater Sci Mater Med 1993; 4:337 - 44; http://dx.doi.org/10.1007/BF00122290
- Yamakawa Y, Chiba J. High performance liquid chromatography of mouse monoclonal antibodies on spherical hydroxy-apatite beads. J Liquid Chrom 1988; 11:665 - 81; http://dx.doi.org/10.1080/01483918808068336
- Luo P, Nieh TG. Preparing hydroxyapatite powders with controlled morphology. Biomaterials 1996; 17:1959 - 64; http://dx.doi.org/10.1016/0142-9612(96)00019-1; PMID: 8894088
- Wang S, Wang C, Hou Z, Wang H, Hu X, Lu H, et al. Reverse microemulsion synthesis of hydroxyapatite nanoparticles and adsorption performance study. Key Eng Mater 2012; 512-515:119 - 22; http://dx.doi.org/10.4028/www.scientific.net/KEM.512-515.119
- Moseke C, Gbureck U. Tetracalcium phosphate: Synthesis, properties and biomedical applications. Acta Biomater 2010; 6:3815 - 23; http://dx.doi.org/10.1016/j.actbio.2010.04.020; PMID: 20438869
- Habib M, Baroud G, Gitzhofer F, Bohner M. Mechanisms underlying the limited injectability of hydraulic calcium phosphate paste. Acta Biomater 2008; 4:1465 - 71; http://dx.doi.org/10.1016/j.actbio.2008.03.004; PMID: 18445539
- Vereecke G, Lemaitre J. Calculation of the solubility diagrams in the system Ca(OH)2-H3PO4-KOH-HNO3-CO2-H2O. J Cryst Growth 1990; 104:820 - 32; http://dx.doi.org/10.1016/0022-0248(90)90108-W
- Antonucci JM, Fowler BO, Venz S. Filler systems based on calcium metaphosphates. Dent Mater 1991; 7:124 - 9; http://dx.doi.org/10.1016/0109-5641(91)90058-7; PMID: 1936640
- Liao CJ, Lin FH, Chen KS, Sun JS. Thermal decomposition and reconstitution of hydroxyapatite in air atmosphere. Biomaterials 1999; 20:1807 - 13; http://dx.doi.org/10.1016/S0142-9612(99)00076-9; PMID: 10509191
- Nold P, Löbe R, Müller M. Granule Production - Easy and Cost-effective, Part 1. Inter Ceram Rev 2004; 53:13 - 7
- Nold P, Löbe R, Müller M. Granule Production - Easy and Cost-effective, Part 2. Inter Ceram Rev 2004; 53:96 - 101
- Andrés-Vergés M, Fernández-González C, Martínez-Gallego M, Solier JD, Cachadiña I, Matijević E. A new route for the synthesis of calcium-deficient hydroxyapatites with low Ca/P ratio: Both spectroscopic and electric characterization. J Mater Res 2000; 15:2526 - 33; http://dx.doi.org/10.1557/JMR.2000.0362
- Jokic B, Mitric M, Popovic M, Sima L, Petrescu SM, Petrovic R, et al. The influence of silicon substitution on the properties of spherical- and whisker-like biphasic α-calcium-phosphate/hydroxyapatite particles. J Mater Sci Mater Med 2011; 22:2175 - 85; http://dx.doi.org/10.1007/s10856-011-4412-6; PMID: 21823030
- Kandori K, Yasukawa A, Ishikawa T. Preparation and characterization of spherical calcium hydroxyapatite. Chem Mater 1995; 7:26 - 32; http://dx.doi.org/10.1021/cm00049a007
- Liu Y, Shelton RM, Barralet JE. Homogeneous Octacalcium Phosphate Precipitation: Effect of Temperature and pH. In: Barbosa MA, Monteiro FJ, Correia R, Leon B, eds. Porto, 2004:79-82.
- Xia W, Grandfield K, Schwenke A, Engqvist H. Synthesis and release of trace elements from hollow and porous hydroxyapatite spheres. Nanotech 2011; 22.
- Shimizu H, Zhuang Z, Aizawa M. Morphological control of hydroxyapatite particles by homogeneous precipitation method in the co-presence of various carboxylic acids. Key Eng Mater 2013; 529-530:61 - 5; http://dx.doi.org/10.4028/www.scientific.net/KEM.529-530.61
- Bohner M, Brunner TJ, Doebelin N, Tang R, Stark WJ. Effect of thermal treatments on the reactivity of nanosized tricalcium phosphate powders. J Mater Chem 2008; 18:4460 - 7; http://dx.doi.org/10.1039/b804314k
- Cho JS, Rhee SH. The size control of hydroxyapatite particles during spray pyrolysis. Key Eng Mater 2013; 529-530:66 - 9; http://dx.doi.org/10.4028/www.scientific.net/KEM.529-530.66
- Nakamura M, Zhuang Z, Aizawa M. Fabrication of boron-containing apatite ceramics via ultrasonic spray-pyrolysis route and their surface properties. Key Eng Mater 2013; 529-530:109 - 13; http://dx.doi.org/10.4028/www.scientific.net/KEM.529-530.109
- Jokanović V, Nikčević I, Dačić B, Uskoković D. Synthesis of nanostructured carbonated calcium hydroxyapatite by ultrasonic spray pyrolysis. J Ceram Proc Res 2004; 5:157 - 62
- Eltohamy M, Shin US, Won JE, Kim JJ, Kim HW. Electrosprayed tricalcium phosphate spherical microcups and antibiotic drug delivery. Mater Lett 2011; 65:2043 - 6; http://dx.doi.org/10.1016/j.matlet.2011.04.003
- Takami K, Machimura H, Takado K, Inagaki M, Kawashima Y. Novel preparation of free flowing spherically granulated dibasic calcium phosphate anhydrous for direct tabletting. Chem Pharm Bull (Tokyo) 1996; 44:868 - 70; http://dx.doi.org/10.1248/cpb.44.868
- Lemos AF, Rocha JHG, Ventura JM, Ferreira JMF. Method for tailoring and control the morphology, size and porosity of calcium phosphate granules. Key Eng Mater 2005; 284-286:309 - 12; http://dx.doi.org/10.4028/www.scientific.net/KEM.284-286.309
- Suwanprateeb J, Sanngam R, Panyathanmaporn T. Influence of raw powder preparation routes on properties of hydroxyapatite fabricated by 3D printing technique. Mater Sci Eng C 2010; 30:610 - 7; http://dx.doi.org/10.1016/j.msec.2010.02.014
- Tonegawa T, Ikoma T, Chen G, Tanaka J. Synthesis and characterization of metal ions containing hydroxyapatite microparticles with high specific surface area. J Nanosci Nanotechnol 2007; 7:839 - 43; http://dx.doi.org/10.1166/jnn.2007.526; PMID: 17450843
- van der Meulen J, Koerten HK. Inflammatory response and degradation of three types of calcium phosphate ceramic in a non-osseous environment. J Biomed Mater Res 1994; 28:1455 - 63; http://dx.doi.org/10.1002/jbm.820281211; PMID: 7876285
- Feng J, Chong M, Zhang ZY, Teoh SH, Thian ES. Apatite-based microcarriers for bone tissue engineering. Key Eng Mater 2013; 529-530:34 - 9; http://dx.doi.org/10.4028/www.scientific.net/KEM.529-530.34
- Hashimoto Y, Adachi S, Matsuno T, Omata K, Yoshitaka Y, Ozeki Y, et al. Effect of an injectable 3D scaffold for osteoblast differentiation depends on bead size. Biomed Mater Eng 2009; 19:391 - 400; PMID: 20231791
- Perez RA, Del Valle S, Altankov G, Ginebra MP. Porous hydroxyapatite and gelatin/hydroxyapatite microspheres obtained by calcium phosphate cement emulsion. J Biomed Mater Res B Appl Biomater 2011; 97:156 - 66; http://dx.doi.org/10.1002/jbm.b.31798; PMID: 21290594
- Komlev VS, Barinov SM, Girardin E, Oscarsson S, Rosengren Å, Rustichelli F, et al. Porous spherical hydroxyapatite and fluorhydroxyapatite granules: Processing and characterization. Sci Technol Adv Mater 2003; 4:503 - 8; http://dx.doi.org/10.1016/j.stam.2003.11.007
- Kamitakahara M, Imai R, Ioku K. Preparation and evaluation of spherical Ca-deficient hydroxyapatite granules with controlled surface microstructure as drug carriers. Mater Sci Eng C 2013; http://dx.doi.org/10.1016/j.msec.2013.01.017
- Apelt D, Theiss F, El-Warrak AO, Zlinszky K, Bettschart-Wolfisberger R, Bohner M, et al. In vivo behavior of three different injectable hydraulic calcium phosphate cements. Biomaterials 2004; 25:1439 - 51; http://dx.doi.org/10.1016/j.biomaterials.2003.08.073; PMID: 14643619
- Theiss F, Apelt D, Brand B, Kutter A, Zlinszky K, Bohner M, et al. Biocompatibility and resorption of a brushite calcium phosphate cement. Biomaterials 2005; 26:4383 - 94; http://dx.doi.org/10.1016/j.biomaterials.2004.11.056; PMID: 15701367
- Oh KS, Choi HW, Kim SR. Temperature rise and setting of β-TCP - MCPM bone cement containing dense β-TCP granules. Curr Appl Phys 2005; 5:489 - 92; http://dx.doi.org/10.1016/j.cap.2005.01.016
- Oh KS, Kim SR. Effect of the composition in β-TCP-MCPM bone cement containing dense β-TCP granules. Key Eng Mater 2005; 284-286:141 - 4; http://dx.doi.org/10.4028/www.scientific.net/KEM.284-286.141
- Oh KS, Kim SR, Boch P. Synthesis and Properties of Bone Cement Containing Dense β-TCP Granules. In: Barbosa MA, Monteiro FJ, Correia R, Leon B, eds. Porto, 2004:237-40.
- Matsuno T, Hashimoto Y, Adachi S, Omata K, Yoshitaka Y, Ozeki Y, et al. Preparation of injectable 3D-formed β-tricalcium phosphate bead/alginate composite for bone tissue engineering. Dent Mater J 2008; 27:827 - 34; http://dx.doi.org/10.4012/dmj.27.827; PMID: 19241692
- Hattori T, Iwadate Y. Hydrothermal preparation of calcium hydroxyapatite powders. J Am Ceram Soc 1990; 73:1803 - 5; http://dx.doi.org/10.1111/j.1151-2916.1990.tb09841.x
- Chaudhry AA, Goodall J, Vickers M, Cockcroft JK, Rehman I, Knowles JC, et al. Synthesis and characterisation of magnesium substituted calcium phosphate bioceramic nanoparticles made via continuous hydrothermal flow synthesis. J Mater Chem 2008; 18:5900 - 8; http://dx.doi.org/10.1039/b807920j
- Weiss P, Layrolle P, Clergeau LP, Enckel B, Pilet P, Amouriq Y, et al. The safety and efficacy of an injectable bone substitute in dental sockets demonstrated in a human clinical trial. Biomaterials 2007; 28:3295 - 305; http://dx.doi.org/10.1016/j.biomaterials.2007.04.006; PMID: 17466366
- Kumar R, Prakash KH, Cheang P, Gower L, Khor KA. Chitosan-mediated crystallization and assembly of hydroxyapatite nanoparticles into hybrid nanostructured films. J R Soc Interface 2008; 5:427 - 39; http://dx.doi.org/10.1098/rsif.2007.1141; PMID: 17698476
- Wang Y, Moo YX, Chen C, Gunawan P, Xu R. Fast precipitation of uniform CaCO3 nanospheres and their transformation to hollow hydroxyapatite nanospheres. J Colloid Interface Sci 2010; 352:393 - 400; http://dx.doi.org/10.1016/j.jcis.2010.08.060; PMID: 20846664
- Hulsart-Billström G, Xia W, Pankotai E, Weszl M, Carlsson E, Forster-Horváth C, et al. Osteogenic potential of Sr-doped calcium phosphate hollow spheres in vitro and in vivo. J Biomed Mater Res A 2013; http://dx.doi.org/10.1002/jbm.a.34526; PMID: 23359485
- Cheung HS, Devine TR, Hubbard W. Calcium phosphate particle induction of metalloproteinase and mitogenesis: effect of particle sizes. Osteoarthritis Cartilage 1997; 5:145 - 51; http://dx.doi.org/10.1016/S1063-4584(97)80009-X; PMID: 9219677
- Soares SE, de Freitas DG, da Silva SN. Processing and characterization of a composite of hyaluronic acid (HA) and microspheres biphasic calcium phosphate (BCP) for dermal repair. Key Eng Mater 2013; 529-530:421 - 5
- Wang W, Itoh S, Yamamoto N, Okawa A, Nagai A, Yamashita K. Enhancement of nerve regeneration along a chitosan nanofiber mesh tube on which electrically polarized β-tricalcium phosphate particles are immobilized. Acta Biomater 2010; 6:4027 - 33; http://dx.doi.org/10.1016/j.actbio.2010.04.027; PMID: 20451674
- Paul W, Sharma CP. Modified Hydroxyapatite Microspheres as Immunoadsorbent for Plasma Perfusion: Preliminary Study. J Colloid Interface Sci 1995; 174:224 - 9; http://dx.doi.org/10.1006/jcis.1995.1385
- Peters F, Reif D. Functional materials for bone regeneration from beta-tricalcium phosphate. Materialwiss Werkst 2004; 35:203 - 7; http://dx.doi.org/10.1002/mawe.200400735
- Baroth S, Bourges X, Goyenvalle E, Aguado E, Daculsi G. Injectable biphasic calcium phosphate bioceramic: The HYDROS concept. Biomed Mater Eng 2009; 19:71 - 6; PMID: 19458448