Abstract
X-ray irradiation influences metastatic properties of tumor cells and, moreover, metastasis and cellular motility can be modified by members of the Eph receptor/ephrin family of receptor tyrosine kinases. We hypothesized that irradiation-induced changes in cellular properties relevant for metastasis in melanoma cells could be mediated by Eph receptor/ephrin signaling. In this pilot study, we analyzed one pre-metastatic (Mel-Juso) and three metastatic human melanoma (Mel-Juso-L3, A375, and A2058) cells lines and predominantly found anti-metastatic effects of X-ray irradiation with impaired cell growth, clonal growth and motility. Additionally, we observed an irradiation-induced increase in adhesion paralleled by a decrease in migration in Mel-Juso and Mel-Juso-L3 cells and, in part, also in A375 cells. We further demonstrate a decrease of EphA2 both in expression and activity at 7 d after irradiation paralleled by an upregulation of EphA3. Analyzing downstream signaling after irradiation, we detected decreased Src kinase phosphorylation, but unchanged focal adhesion kinase (FAK) phosphorylation, indicating, in part, irradiation-induced downregulation of signaling via the EphA2-Src-FAK axis in melanoma cells. However, to which extent this finding contributes to the modification of metastasis-relevant cellular properties remains to be elucidated.
Introduction
Radiotherapy is one of the most common treatments of human cancer. Although there is evidence that irradiation can lead to radioresistant tumor cell subpopulations with enhanced proliferation and invasion, and that irradiation-induced cytotoxic effects impair also surrounding healthy tissue,Citation1 it is broadly accepted that ionizing radiation like X-ray lead to biochemical changes in the cellular metabolism resulting in death of tumor cells. The irradiation-induced impairment of cellular viability, proliferation and clonal survival was analyzed in many different tumor entities, among them were glioblastoma, non-small-cell lung cancer, colon carcinoma and melanoma.Citation2-Citation4 The influence of irradiation on human melanoma cells was analyzed by Ristic-Fira and colleagues who found a dose-dependent decrease of cell proliferation 7 d after irradiation with gamma rays.Citation5 In a previous study we analyzed the impact of irradiation on mouse melanoma cells and also found decreased viability and proliferation, respectively, which persisted for at least two weeks.Citation6 Further we observed a recurrence of proliferation and of clonal growth properties at two and three weeks after treatment. Concerning irradiation-induced modulation of metastatic properties inconsistent data exist. On the one hand, recent findings suggest that irradiation may cause an increase in metastatic properties of surviving cells. This could explain tumor recurrence and metastasis after radiation therapy and could be mediated by modulation of adhesion molecules (reviewed in refs. Citation1 and Citation7). In this regard, an irradiation-induced increase in substrate adhesion was demonstrated for mouse melanoma, fibrosarcoma cells and lung cancer.Citation2,Citation8-Citation11 Furthermore, irradiation was found to increase motility, migration and invasion in glioma, pancreatic cancer, non-small-cell lung cancerCitation8,Citation11-Citation13 and also to increase metastasis in vivo.Citation10,Citation14,Citation15 Further, increasing migration at low doses has been described, followed by a decrease at higher doses.Citation2,Citation9 On the other hand, irradiation-induced reduction of migration and invasion was shown for colon carcinoma, glioblastoma and lung carcinomaCitation3,Citation4,Citation16 as well as reduction of plating efficiency in irradiated hepatocarcinoma and mouse mammary carcinoma.Citation17,Citation18
Eph receptors constitute the largest familiy of receptor tyrosine kinases with at least 14 members in mammals.Citation19 In combination with their membrane-bound ephrin ligands they form a unique cellular communication system mediating cell repellent effects, cell-cell and cell-matrix attachment, cell shape and motility. Therefore, they play a crucial role in embryonal development, development and plasticity of the central nervous system and angiogenesis (see refs. Citation20, Citation21 and references therein), but also in pathological conditions like cellular transformation, tumorangiogenesis and metastasis (see refs. Citation20, Citation24 and references therein). One of the best known Eph receptors is EphA2, which was found to be overexpressed in many tumor entities, whereby highest levels are mostly found in aggressive cells.Citation25 In this regard, EphA2 was detected in aggressive melanoma cell lines, but not in melanocytes and benign nevi.Citation26,Citation27 High levels of EphA2 were also found in breast cancer,Citation28,Citation29 prostate cancer,Citation30 lung cancer,Citation31 malignant gliomaCitation32 and gastric cancer.Citation33 Although the EphA2/ephrinA1 pathway can be linked to tumor neovascularizationCitation34 and the formation of tubular structures in metastatic melanoma,Citation35 most data reveal its role in cell proliferation and modulation of the cytoskeleton. Activation of EphA2 receptor negatively regulates the growth and survival of different tumor cell models.Citation25 For instance, stimulation of EphA2 by ephrinA1 inhibited proliferation and clonal growth in prostate epithelium and endothelial cells by inhibiting the Ras/MAPK cascade.Citation36 EphA2 activation also decreased proliferation in breast cancer cells and in prostate carcinoma cells by inhibiting phosphorylation of serine/threonine protein kinase Akt.Citation37,Citation38 On the other hand, it was demonstrated that the expression of EphA2 is associated with increased tumor cell proliferation in cutaneous melanoma.Citation39 The influence of EphA2 activation on the cytoskeleton and, hence, on adhesion, migration and metastasis is under intensive investigation and several involved signaling pathways have been identified so far. As important downstream targets of EphA2 for instance phosphatidylinositol-3 kinase, integrins, focal adhesion kinase (FAK), Src kinase, Akt and Rho GTPases have been identified, leading to decreased tumor cell adhesion and increased migration and invasion, thus finally resulting in a prometastatic phenotype.Citation40-Citation43 Another member of the Eph receptor family, EphA3Citation44 was also found to be present or overexpressed in a panel of tumors such as leukemias and lymphomas, neural cancers, lung cancer, rhabdomyosarcoma cell lines, breast cancer cell lines, colorectal carcinoma and melanoma (see refs. Citation45, Citation48 and references therein). Its main functions seem to be closely connected to the rearrangement of the cytoskeleton, because activation of EphA3 by its main ligand ephrinA5 results in reduction of cell adhesion, inhibition of migration and reduction of neurite outgrowth.Citation49,Citation50 As potential downstream signaling pathways and binding partners Rho GTPases, Src family kinases and the adapterprotein Nck1 have been identified.Citation45,Citation51,Citation52 Very recently, Lisabeth and coworkers suggested that both EphA2 and EphA3, on the one hand, have tumor suppressor activities that depend on ephrin and kinase activity and, on the other hand, tumor promoting activity depending on crosstalk with other signaling molecules.Citation53 The latter does not require ephrin binding or kinase activity.Citation42,Citation53
Although in recent years the importance and the complex signaling of Eph receptors and ephrins in cancer were considerably illuminated, there is rare knowledge about the influence of irradiation on Eph receptors. In squamous cell carcinoma Noriji and colleagues found upregulation of ephrinA1 in radioresistant but not in radiosensitive cell lines after irradiation with carbon ions and gamma rays.Citation54 It remains unclear, if irradiation could modify cellular properties by regulation of Eph receptors or ephrins. In this pilot study we characterized effects of X-ray irradiation on proliferation, metastatic properties and, additionally, on Eph receptor/ephrin expression and downstream signaling moleclues in various human melanoma cell lines. We focused on both EphA2 and EphA3, which, with their corresponding main ligands ephrinA1 and ephrinA5, respectively, have been described to play a crucial role also in melanoma progression. For the first time we demonstrated that X-ray irradiation leads to dysregulation of these selected EphA receptors and their main ephrin ligands in melanoma cells. This is assumed to be associated with the observed irradiation-induced cellular changes in a predominantly anti-metastatic manner in the same cells.
Results
The aim of the present study was the characterization of effects of external X-ray irradiation on melanoma cells. Thereby, we focused on cellular properties, such as proliferation and metastatic properties and analyzed a panel of receptor tyrosin kinases of the Eph family and their ephrin ligands. We used four human melanoma cells lines, one originating of a primary tumor (“pre-metastatic”), one self-generated subline originating from this cell line (designated as Mel-Juso-L3) and two malignant melanoma cell lines. Different doses of external X-ray irradiation were applied and cells were analyzed 1 d and 7 d after irradiation to distinguish between short-term and long-term effects.
External irradiation alters cellular properties in a predominantly anti-metastatic manner
As metastasis is a multi-step process, we analyzed a broad range of cellular properties, strongly suggested to be involved in the development of metastases. Initially, we analyzed proliferation (). In all four cell lines we found a significant dose-dependent decrease in cell proliferation 1 d as well as 7 d after irradiation.
Figure 1. Decrease in proliferation after irradiation. Cells were seeded with 1 × 105 cells per well in 6-well plates and cultured overnight. Cells were irradiated with 5 or 10 Gy or remained untreated, followed by cell counting every 24 h, up to 3 d. For growth assay 7 d after X-ray, cells were irradiated with 5 or 10 Gy and cultured for another 6 d. Then, cells were plated with 1 × 105 cells per well in 6-well plates and counted every 24 h, up to 3 d. Y axis displays cell number per well at the 3rd day of cell counting. Three independent experiments, each in duplicate were performed. Symbols represent means ± SEM, (p ≤ 0.01, two-tailed), *, vs. 0 Gy; Mann-Whitney U test.
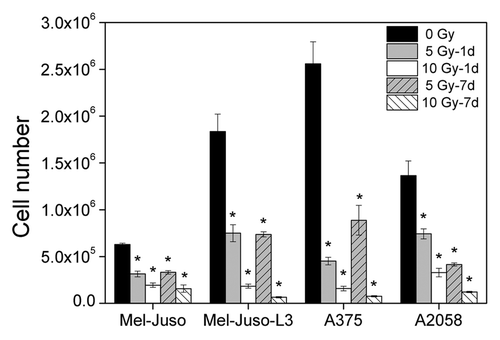
Because clonal growth assays reflect metastasis better than simple growth curves, we analyzed clonal regrowth capability of cells by determining the plating efficiency (). In all cell lines we found a decreased plating efficiency in irradiated cells with exception of cells at 7 d after irradiation with 5 Gy. However, we cannot exclude that decreased plating efficiency in part could result from reduced cell growth after irradiation. Independent of treatment, melanoma cells showed different general plating efficiencies with highest observed in A375, followed by Mel-Juso, Mel-Juso-L3 and A2058.
Figure 2. Influence of irradiation on plating efficiency. Untreated or treated cells were plated with 1,000 or 2,000 cells/well in 6-well plates, cultured for 5 to 11 d. Colonies greater 50 cells were counted, and plating efficiency was calculated as ratio of the number of colonies to the number of seeded cells × 100%. Three independent experiments, each in duplicate were performed. Upper panel: representative wells after staining. Lower panel: data after counting the cell clones. Symbols represent means ± SEM, (p ≤ 0.05, two-tailed), *, vs. 0 Gy; # vs. A2058-0 Gy; Mann-Whitney U test.
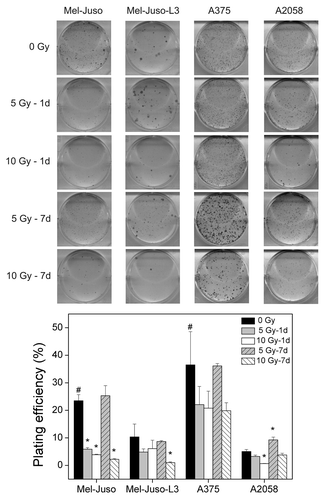
As further metastasis-relevant cellular properties we analyzed motility, adhesion and migration. As shown in , 1 d after irradiation motility is significantly decreased in cell lines Mel-Juso, Mel-Juso-L3 and A375 and slightly decreased in A2058 cells irradiated with 10 Gy. Seven days after irradiation A375 and A2058 cells showed no difference in motility of irradiated or untreated cells, whereas motility in irradiated Mel-Juso and Mel-Juso-L3 cells significantly increased 7 d after treatment. Motility assay of A375, 7 d after irradiation with 10 Gy was hindered as these cells do not constitute a confluent cell monolayer.
Figure 3. Influence of irradiation on motiliy, measured by scratch assay. (A) Representative images of cells directly (0 h) or 10 h after scratch in controls (con) or cells irradiated with 10 Gy (X-ray). Upper panel: cells 1 d after irradiation, lower panel: cells 7 d after irradiation. (B) Measured scratch widths. Upper panel: cells were seeded, irradiated with 5 or 10 Gy or remained untreated, cultured overnight and the scratch was generated. Scratch width at five sites on a particular position was measured after 4, 6, 8, 10 and 24 h. Lower panel: cells were irradiated with 5 or 10 Gy and cultured for another 6 d. Treated and untreated cells were seeded and cultured overnight before starting with the assay as described above. Three independent experiments were performed. Symbols represent means ± SEM, (p ≤ 0.05), *, vs. 5 and 10 Gy; #, vs. 5 Gy; §, vs. 10 Gy. ANOVA, Bonferroni post hoc method.
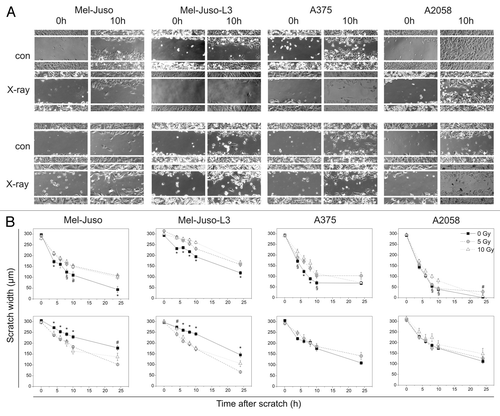
displays adhesion to fibronectin and migration through pores of a membrane. Mel-Juso and Mel-Juso-L3 cells show same behavior with irradiation-induced increase in adhesion and simultaneous decrease in migration. In A375 cells adhesion is also increased after irradiation, whereby significant decrease in migration is only seen 7 d after irradiation with 10 Gy. A2058 cells show increased adhesion 1 d but not 7 d after irradiation. In contrast to the other cell lines, migration is increased in A2058 cells with significant changes 7 d after irradiation with 5 Gy.
Figure 4. Influence of irradiation on adhesion and migration. Left panel: adhesion assay: untreated or treated cells were seeded with 1 × 105 cells per well in fibronectin-coated 24-well plates and allowed to adhere. Non-adherent cells were washed away and the remaining cells were stained with crystal violet. Cells were lysed and absorbance of cell lysates was measured. Four independent experiments were performed each in quadruplicate. Symbols represent means ± SEM, (p ≤ 0.05, two-tailed), *, vs. 0 Gy; Mann-Whitney U test. Right panel: untreated or treated cells were seeded with 2 × 105 cells in the upper compartment of a Boyden chamber. Cells were allowed to migrate through the 8 µm pores of a membrane toward chemoattractant and stained with calcein. Fluorescence signal of the cells was measured at 485/528 nm and relative migration was calculated as fluorescence units of samples with chemoattractant (10% FCS) vs. control samples (0% FCS). Three independent experiments were performed, each in triplicate. Symbols represent means ± SEM, (p ≤ 0.05), *, vs. 0 Gy; ANOVA, Bonferroni post hoc method.
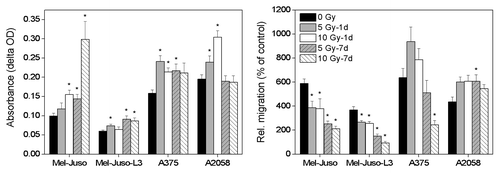
Taken together, we found irradiation-induced decrease in proliferation, clonal growth, motility and migration but similar increase in adhesion, which indicates that external X-ray irradiation changes properties of melanoma cells in a merely anti-metastatic manner.
External irradiation alters Eph receptors and ephrin ligands in melanoma cells
We analyzed the mRNA expression of selected Eph receptors and ephrin ligands in human melanoma cells (). In all cell lines we found expression of the receptors EphA2, A3 A4, B2, B3 and B4. Several Eph receptors were expressed in a subset of the melanoma cells investigated, such as EphA1 in A375 cells, EphA5 in Mel-Juso, Mel-Juso-L3 and A2058 cells, EphA7 in Mel-Juso and A375 cells, and EphB1 in Mel-Juso, Mel-Juso-L3 and A375 cells. Receptor EphB6 was only found to be expressed in control cells. Ephrins A2, A5, B1 and B2 were detected in all investigated cell lines. In contrast, ephrinA1 was detected only in A375 and A2058 cells and ephrinA3 in Mel-Juso-L3, A375 and A2058 cells.
Table 1. Expression of selected Eph receptors and ephrin ligands as determined by RT-PCR
As EphA2 and EphA3 receptors are known to play an important role in melanoma progression and metastasis we decided to further focus on these receptors and their main ligands ephrinA1 and ephrinA5. First, we quantified mRNA content of irradiated melanoma cells in relation to untreated cells using quantitative real-time RT-PCR (). We detected an irradiation-induced decrease in EphA2 mRNA with the exception of Mel-Juso-L3 and A375 cells 1 d after treatment. EphA3 receptor mRNA showed a significant decrease in Mel-Juso (1 d after X-ray) and A2058 cells (1 d and 7 d after X-ray), but significant increase in A375 cells 7 d after irradiation. Mel-Juso-L3 cells showed a slight but not significant increase in EphA3 mRNA after treatment. EphrinA1 mRNA was detected in A375 and A2058 but not in untreated Mel-Juso and Mel-Juso-L3 cells. Interestingly, ephrinA1 mRNA was detected in irradiated Mel-Juso-L3 cells (7 d after X-ray). In A375 cells we found a decrease 1 d after treatment and in A2058 cells an increase 7 d after treatment. EphrinA5 mRNA showed a decrease in all melanoma cells after treatment.
Figure 5. Relative mRNA expression of EphA2, EphA3, ephrinA1 and ephrinA5 in irradiated (10 Gy, 1 d and 7 d after X-ray) melanoma cells analyzed by quantitative real-time RT-PCR. Total RNA was extracted, followed by DNase digestion. Fifty nanograms of the pretreated RNA was applied into each RT-PCR reaction. mRNA levels of Eph and ephrins were determined using the delta-delta CT method with 18SrRNA as reference. Relative expression is depicted in relation to untreated cells. For each cell line three independent RNA samples were analyzed, each in triplicate. Symbols represent means ± SEM, (p ≤ 0.05), *, vs. 0 Gy, ANOVA, Bonferroni post hoc method.
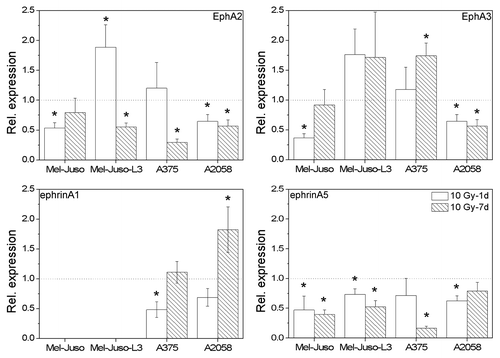
Furthermore, we analyzed the Eph receptors on protein level using SDS-PAGE and western blotting (). For the EphA2 receptor we detected highest level in A375 cells. A lower protein level was detected in the other melanoma cell lines. For the EphA3 receptor we found nearly similar protein content in all four cell lines (). At 7 d after irradiation we detected a decrease in EphA2 receptor in Mel-Juso-L3 and A375 cells (). In Mel-Juso and A2058 cells no EphA2 protein changes were observed. Interestingly, for EphA3 receptor we detected an increase 7 d after irradiation in Mel-Juso, Mel-Juso-L3 and A375 cells, while in A2058 cells a slight decrease 7 d after irradiation with 10 Gy was observed. In neither cell line we detected changes in protein level of EphA2 and EphA3 1 d after irradiation compared with untreated cells. Protein detection of ephrins A1 and A5 was not successful with SDS-PAGE and western blotting, probably due to low protein levels in melanoma cells. However, we observed a weak signal in flow cytometric analysis but found no difference in ephrin protein after irradiation (data not shown). Furthermore, we analyzed specific tyrosine phosphorylation and hence activity of the EphA2 receptor after irradiation after immunoprecipitation of EphA2 (). Analysis was performed in A375 cells due to high overall EphA2 expression. In parallel with decrease in EphA2 protein we detected a decrease in tyrosine phosphorylation at 7 d after irradiation (10 Gy), indicating irradiation-induced decrease in EphA2 activity due to reduced EphA2 expression. In part, this is consistent with lower tyrosine phosphorylation after irradiation (7 d, 5 and 10 Gy) observed in total cell lysates (data not shown in detail). Subsequent to receptor activation we analyzed downstream signaling molecules FAK and Src, both known to play a role in the promotion of malignant phenotypic characteristics and to interact with EphA2.
Figure 6. Representative western blots showing EphA2 and EphA3 receptor in untreated or irradiated melanoma cell lines. Cells were lysed, followed by protein quantification using BCA protein assay. Protein extracts were subjected to SDS-PAGE, western blotting and immunodetection using specific antibodies. A polyclonal actin antibody was used to verify equal protein amount. For each cell line at least three independent protein samples were analyzed. (A) EphA2 and EphA3 receptor in untreated melanoma cells. (B) EphA2 and EphA3 receptor in irradiated (5 and 10 Gy, 1 d and 7 d after X-ray) melanoma cells. (C) EphA2 receptor was immunoprecipitated from protein lysates from A375 cells. Immunoprecipitates were subjected to SDS-PAGE, western blotting and immunodetection using specific antibody to phosphorylated tyrosine and reprobed with EphA2 antibody.
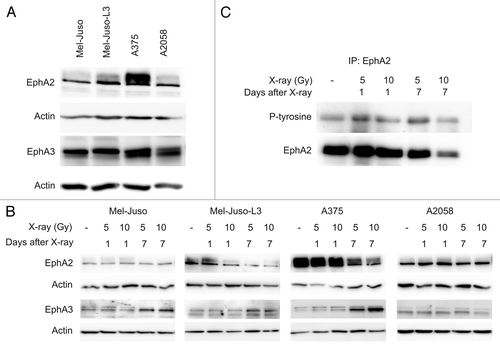
The expression of Src kinase was similar in all four melanoma cell lines investigated, but showed higher basal phosphorylation in A375 and A2058 cells than in Mel-Juso and Mel-Juso-L3, respectively (). The expression and phosphorylation of FAK was similar in Mel-Juso, A375 and A2058 cells, but showed considerably lower levels in Mel-Juso-L3 cells (). Following irradiation we detected a decrease or even a loss in phosphorylated Src in Mel-Juso, A375 and A2058 cells at 7 d and 10 Gy, while total Src protein content was unaffected (). For FAK we could not detect irradiation-induced changes in both phosphorylation and protein content ().
Figure 7. Representative western blots showing Src and FAK phosphorylation in untreated or irradiated melanoma cell lines. Cells were lysed, followed by protein quantification using BCA protein assay. Protein extracts were subjected to SDS-PAGE, western blotting and immunodetection using specific antibodies. Immundetection of the phospho-specific antibodies was followed by stripping and reprobing with anti-Src or anti-FAK. A polyclonal actin antibody was used to verify equal protein amount. For each cell line at least three independent protein samples were analyzed. (A) P-Src and Src kinase in untreated melanoma cells. (B) P-FAK and FAK in untreated melanoma cells. (C) P-Src/Src and P-FAK/FAK in irradiated (5 and 10 Gy, 1 d and 7 d after X-ray) melanoma cells.
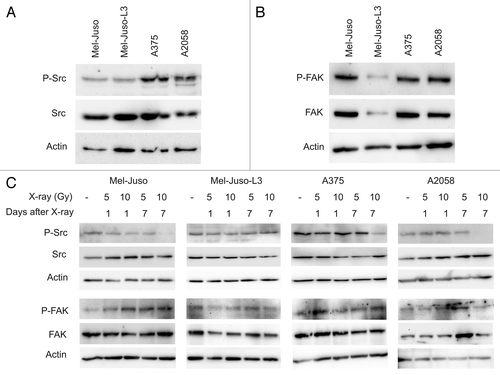
Discussion
Although many malignant tumors are well treatable by radiotherapy at the primary site, metastases are the key factor for prognosis and outcome of patients. Therefore, understanding underlying mechanisms and identification of molecules involved in metastasis is very important and hopefully will provide new possibilities for improved individualized therapeutical intervention. Beneath several adhesion molecules,Citation7 also Eph receptors and their ephrin ligands seem to be involved in metastasis. It is assumed that they are engaged via regulation of tumorangiogenesis, proliferation and metastatic properties by modifying the cytoskeleton. However, it is unknown, if irradiation (for instance previous radiotherapy of the tumor) influences metastasis by regulating Eph receptor expression. In this pilot study we initially analyzed the effects of X-ray irradiation on cellular properties of human melanoma cells as model for highly metastatic tumors, followed by analysis of irradiation-induced changes in Eph/ephrin expression and activity.
In all four investigated melanoma cell lines we found a dose-dependent decrease in proliferation and plating efficiency. These findings are in line with other authors and our own former study. In this regard, proliferation of B16-F10 cells showed a partial recovery one week after irradiation with 5 GyCitation6 which could not be detected in human melanoma cells. Irradiation-induced reduction in plating efficiency was in a similar extent (reduction about one half) as observed for hepatocarcinoma cells by Liu et al.Citation18 Further we analyzed motility, adhesion and migration of the melanoma cells. The motility was found to be decreased one day after treatment in all cell lines. This effect seems to be temporary, as 7 d after treatment we found no irradiation-dependent changes in motility in A375 and A2058 cells. Nevertheless, in Mel-Juso and Mel-Juso-L3 cells we even detected an increase in motility, which resembles data from Jung and colleagues, who found increased motility 24 and 48 h after external photon beam irradiation in A549 human lung adenocarcinoma cells.Citation8 It is conspicuous that initial impairment of plating efficiency and motility is partly regressed 7 d after irradiation with the lower dose of 5 Gy. Possibly, this could reflect a kind of recurrence and it is supposed that some cells resist irradiation, recover “normal” properties and form the basis of tumor regrowth and metastasis.Citation6,Citation55 With the exception of A2058 cells, 7 d after irradiation, adhesion to fibronectin was increased after irradiation which is in accordance with other authors.Citation2,Citation9,Citation10 It is discussed that increased adhesion is mediated by irradiation-induced increase in β1 integrin expression and affinity.Citation3,Citation9 Concomitant to increased adhesion to fibronection we detected a decrease in migration. These opposite effects were most prominent in Mel-Juso and Mel-Juso-L3 cells. A375 showed decreased migration only 7 d after irradiation with 10 Gy. A2058 cells show different effects as the other cell lines with an increase in migration. The data from the literature concerning irradiation effects on migration and invasion are controversial depending on tumor entity. Some authors detected an irradiation-induced increase in migration and invasion in glioma and pancreatic cell lines,Citation12,Citation13 while other authors found an irradiation-induced decrease in lung adenocarcinoma, colorectal carcinoma or sarcoma cell lines.Citation4,Citation9,Citation16 However, the detected differences between individual melanoma cell lines in irradiation-induced changes in metastasis-relevant properties as well as in Eph/ephrin expression seem to base rather on genetic or epigenetic background than on origin of the cell lines. For instance, Mel-Juso-L3 is a “metastatic” subline of Mel-Juso and should resemble the other metastatic cell lines A375 and A2058. But, as visible, for instance, in motility and migration, Mel-Juso-L3 cells share more similarities with the parenteral Mel-Juso cell line than with A375 and A2058. Additionally, A2058 cells in several experiments show different effects compared with the other three melanoma cell lines, for example in migration or irradiation-induced changes in EphA2 and EphA3 receptors. STR DNA profiles of Mel-Juso and Mel-Juso-L3 cells are absolutely identical, but differ from the profiles of A375 and A2058 cells in 12 and 11, respectively, of 16 loci analyzed (data not shown). Disease-relevant mutations in Eph receptor genes have been described for EphA2 in congenital cataractCitation56 and for EphA3 in hepatocellular carcinoma.Citation57 To our knowledge for melanoma and, particularly, for the cell lines used, no mutations in EphA2 or EphA3 genes have been described. However, this cannot be excluded because Eph sequences were not analyzed in our study. We only detected differences in the expression of certain Eph receptors and ephrins but identified no clear expression pattern explaining the observed phenotypic differences between cell lines. Epigenetic mechanisms are suggested to play a role in tumorigenesis and metastasis. In this regard, EphA2 receptor was described to be epigenetically silenced in unfavorable neuroblastoma cellsCitation58 and EphA3 receptor transcription may be regulated by DNA methylation in hematopoietic tumors.Citation59 For melanoma cell lines A375 and Mel-Juso anti-proliferative effects mediated by epigenetic mechanisms could be demonstrated.Citation60,Citation61 This could also contribute to the observed phenotypes of the cells. However, for Mel-Juso-L3 and A2058 to our knowledge no data exist concerning epigenetic effects on cellular responses. Taken together, most of our cell culture data reveal an irradiation-induced modification of metastatic properties toward an anti-metastatic phenotype with decreased proliferation, motility, plating efficiency and migration and with increase in adhesion.
Based on the strong expression signals and on the substantial data from literature confirming the important role for EphA2 in melanoma progression, we decided to further investigate EphA2 and EphA3 receptors and their main ligands ephrinA1 and ephrinA5. Generally, ephrin expression was low compared with expression of the Eph receptors. EphrinA1 mRNA was not detected in untreated Mel-Juso and Mel-Juso-L3 cells. Interestingly, we detected a weak ephrinA1 signal in irradiated Mel-Juso-L3 cells. But, possibly due to the low expression, we could not confirm this ephrinA1 signal on protein level. In irradiated A375 and A2058 cells we detected divergent ephrinA1 expression with decrease in A375 and increase in A2058. Related results were reported by Noriji and colleagues, who detected an increase in ephrinA1 RNA in tumors from radioresistant NR-S1 murine squamous cell carcinoma after irradiation with carbon ions and gamma rays.Citation54 In contrast to ephrinA1, for ephrinA5 we consistently detected in all cell lines a decrease on mRNA level. EphA2 receptor mRNA was found to be downregulated after irradiation in most cell lines with few exceptions. This is accompanied by a decrease in EphA2 protein expression and activity as most noticable in A375 cells with the highest EphA2 level of the investigated melanoma cell lines. Comparison of our results concerning EphA2 expression and activity after X-ray with the literature is difficult, as to our knowledge we are the first investigating Eph receptors subjected to X-ray irradiation.
Important downstream signaling molecules of EphA2 are Src and focal adhesion kinases. Parri et al. described the assembly of an EphA2-associated complex involving the two kinases Src and FAK upon ephrinA1 stimulation in prostate carcinoma cells with high endogenous EphA2 level.Citation62 He also demonstrated that ephrin A1 is able to induce Src activation. Further, Buettner et al. demonstrated that inhibition of Src activity using the kinase inhibitor dasatinib inhibited migration of human melanoma cells.Citation40 In line with these data we describe an irradiation-induced reduction in both EphA2 and Src activity. This effect is assumed to influence migration of melanoma cells. Activation of EphA2 results in dephosphorylation of FAK followed by lower adhesion to fibronectin in PC3 prostate cancer cells.Citation41 In this regard, lower activity of EphA2 observed in our study could result in perpetuation of FAK phosphorylation state. In turn, this should result in unchanged adhesion to fibronectin. The observed increment in cell adhesion in this study indicates that besides FAK other molecular mechanisms should contribute to this cellular property of melanoma cells. For EphA3 we detected also a decrease in Mel-Juso and A2058 cells on mRNA level. In contrast, we detected an increase in EphA3 for Mel-Juso-L3 and A375 on mRNA level and for Mel-Juso, Mel-Juso-L3 and A375 on protein level. Conspicuously, changes in EphA2 and EphA3 protein appear mainly 7 d after treatment. Therefore, we assume that irradiation-induced regulation of Eph receptors is not a direct acute effect but rather an indirect effect resulting from slow or long-term transformation processes in cells surviving sublethal X-ray irradiation. As stated elsewhere, EphA3 and EphA2 play important roles in the progression of many tumors. Several authors reported that EphA2 acts as a promotor of melanoma metastasis.Citation27,Citation63 If EphA3 promotes metastasis is still contrary discussed. Lawrenson and colleagues induced EphA3-activation by ephrinA5-stimulation and observed retraction of cell processes, cell rounding and detachment and speculated that this may be one of the initiating events leading to melanoma metastasis.Citation64 On the other hand, EphA3 stimulation with ephrinA5 again lead to process retraction, but in the same study inhibited cell migration in transgenic HEK293A cells.Citation51 In this regard, EphA2 and EphA3 occasionally could have opposite effects, and both increase in EphA2 and decrease in EphA3 could lead to promotion of metastasis. The other way round, decrease in EphA2 and increase in EphA3 could result in decreased metastatic properties. Whether the observed decrease in EphA2 leads to the observed increase in EphA3, remains to be elucidated. Initial experiments did not demonstrate a direct counterregulation, because overexpression of EphA2 in melanoma cells showed no decrease, but also an increase in EphA3 (unpublished results).
In conclusion, the present data indicate that X-ray irradiation of melanoma cells leads to decreased EphA2 but increased EphA3 expression. We suggest that this contributes to the observed anti-metastatic effects such as decreased motility and migration as well as increased adhesion to fibronectin. Further, our data indicate that the observed effects at least partly are mediated by EphA2 signaling via FAK and Src kinase pathway. However, many important questions remain to be addressed. Further identification and clarification of involved signaling cascades is required. Possible candidates interfering with EphA2 receptor signaling are EGFR, E-cadherin, integrins or other adhesion molecules as well as metalloproteinases. As downstream mediators involved aside from FAK and Src kinase, for instance Rho GTPases are strongly suggested. Finally, it would be interesting to analyze if EphA2 regulation could influence radiosensitivity of metastatic cells. In this case, targeting of EphA receptors would represent an interesting target to enhance radiotherapy of solid tumors.
Materials and Methods
Cell culture and irradiation
The human metastatic melanoma cell lines A375 and A2058 were purchased from the American Type Culture Collection (LGC Standards) and the (pre-metastatic) human melanoma cell line Mel-Juso from the German Collection of Microorganisms and Cell Cultures (DSMZ). Cells were cultured in Dulbecco’s modified Eagle's medium (DMEM) supplemented with 10% fetal bovine serum and penicillin/streptomycin (all reagents from Biochrom) at 37°C in a humidified atmosphere with 5% CO2. Cells were tested to be mycoplasma negative using Venor® GeM Mycoplasma Detection Kit (Minerva Biolabs). Irradiation was applied at room temperature using single doses of 200 kV X-ray irradiation filtered with 0.5 mm copper (Maxishot, YXLON International). The absorbed dose was measured using a UNIDOS dosimeter (PTW) and dose-rate was ~1.1 Gy/min at 20 mA. The non-irradiated cells were simultaneously kept at room temperature to obtain comparable conditions. For the generation of pretreated sublines, cells were irradiated and cultured for 1 d or 7 d parallel to untreated cells and freshly plated one day before starting cell culture experiments.
In vivo generation of a metastatic subline of the Mel-Juso cell line
Seven to 10-week-old female NMRI (nu/nu) mice were purchased from the specific pathogen-free breeding facility of the Experimental Centre of the Medical Faculty Carl Gustav Carus, University of Technology, Dresden, Germany. Animal experiments were performed according to the guidelines of the German Regulations for Animal Welfare. The protocol was approved by the local Ethical Committee for Animal Experiments. For additional immunosuppression the nude mice were whole-body irradiated 3 d before tumor cell injection with 4 Gy (200 kV X-rays, 0.5 mm copper filtered, ~1.1 Gy/min at 20 mA). Subsequently, 30 mg/kg body weight ampicillin sodium salt (Roth) was added to the drinking water for 10 d. For tumor cell injection animals were anesthetized using desflurane (10% v/v in 30% oxygen/air). Tumor cells were harvested, washed in phosphate-buffered saline (PBS) and transferred to 0.9% sodium chloride. Only single-cell suspensions (1 × 106 cells/300 µl) with at least 90% viability (trypan blue exclusion) were used for injection into the tail vein. After 12–20 weeks mice were killed by CO2 inhalation, the lungs were removed and metastases were harvested under a stereo microscope. Cells were further processed with trypsin/EDTA (0.05/0.02%) and strained throught sterile 40 µm nylon mesh (Fisher Scientific). Subsequently, cells were cultured under normal conditions and medium for three to five passages. Cells from these cultures were harvested and injected intravenously into another set of mice. The procedure was repeated two times to obtain Mel-Juso cells, three times passaged through the mice lungs, designated as metastatic subline Mel-Juso-L3. The cells were tested to be mycoplasma negative. Human (and Mel-Juso) origin of Mel-Juso-L3 cells was approved by STR DNA typing (performed by DSMZ).
Growth assay
For growth assays, cells were precultured in T25-flasks (Greiner Bio-one), irradiated with 5 or 10 Gy and further cultured for another 6 d. Cells were plated with 1 × 105 cells per well in a 6-well plate. Cell number was determined using trypan blue exclusion and a Neubauer counting chamber 24, 48 and 72 h later. Concurrently, cells were seeded with 1 × 105 cells per well in a 6-well plate and cultured overnight. Cells remained untreated or were irradiated with 5 or 10 Gy. Cell number was determined 24, 48 and 72 h after irradiation. Three independent experiments, each in duplicate were performed.
Clonal growth assay
Clonal growth assay was used to analyze the sensitivity of the melanoma cell lines toward irradiation. Untreated or treated cells (5 or 10 Gy X-rays, 1 or 7 d before plating) were plated with 1,000 cells/well (A375, Mel-Juso and Mel-Juso-L3) or 2,000 cells/well (A2058) in 6-well plates using conditioned medium [DMEM 10% fetal calf serum (FCS), used for culturing the respective melanoma cell line for 2 to 3 d]. Cells were cultured for 5 to 11 d depending on the respective cell line. Then, cells were washed with PBS and fixed in 50% methanol in PBS, followed by methanol for 10 min. Methanol was removed and cells were stained with 0.5% crystal violet, 20% methanol in PBS for 15 min under agitation. Staining solution was removed; the plates were washed three times with tap water and air-dried. Colonies greater than 50 cells were counted under an inverted microscope (AxioVert 40CFL, Carl Zeiss MicroImaging). Plating efficiency was calculated as ratio of the number of colonies to the number of seeded cells × 100%.Citation65 Three independent experiments, each in duplicate, were performed.
Scratch (motility) assay
Scratch assay was performed 1 d and 7 d after treatment. In the first setup (“1 d after X-ray”), cells were seeded in 6-well plates so that they will reach 100% confluence within 48 h (0.5–1 × 106 cells/well). The next day cells were irradiated (control cells were kept at room temperature for the same time) and again cells were cultured overnight before starting with the scratch assay. In the second setup (“7 d after X-ray”), cells were irradiated and further cultured for six days. Treated and untreated cells were seeded with 1–1.5 × 106 cells per well in 6-well plates and cultured overnight to reach 100% confluence before starting the experiment. A vertical scratch was generated in the cell monolayer using a sterile micropipette tip. Cells were washed with PBS and 2 ml/well DMEM 10% FCS was added. Using an inverted phase contrast microscope each scratch was photographed at a particular position with 300 µm scratch width and the respective x-y coordinates on the microscope stage were recorded. Cells were cultured under normal conditions and photographed at the same location after 4, 6, 8, 10 and 24 h. Width of each scratch was measured at five different areas per display window using AxioVision Software package (Carl Zeiss MicroImaging). Three independent experiments were performed.
Adhesion assay
Twenty-four-well plates were coated with 5 µg/ml human fibronectin (Biochrom) in PBS overnight at 4°C. Unspecific binding sites were blocked with PBS 2% bovine serum albumin (BSA, Roth) for 1 h at 37°C. Untreated or treated cells (5 or 10 Gy X-rays, 1 or 7 d before plating) were detached with PBS containing 2 mM EDTA, washed in PBS and resuspended in DMEM 1% BSA. 1 × 105 cells per well were seeded and allowed to adhere. At the indicated timepoints (40 min for A375, 60 min for A2058 and 120 min for Mel-Juso and Mel-Juso-L3) non-adherent cells were washed away with PBS and the remaining cells were fixed in methanol and stained with crystal violet as described above. Air-dried cells were lysed using PBS 0.5% Tween-20 for 5 min under agitation and absorption at 550 nm (reference 650 nm) was measured in a microplate reader. Optical density (delta OD) was calculated by subtraction of the reference absorption from the measured value. Four independent experiments were performed each in quadruplicate.
Migration assay
Migration of melanoma cells through 8 µm pores was assessed using ThinCert™ cell culture inserts according to manufacturer’s protocol (Greiner Bio-one). As chemoattractant we used 10% FCS vs. 0% FCS in DMEM in the lower compartment of the chamber. Single cell suspensions of serum-starved untreated or treated cells (2 × 105 cells/sample, 5 or 10 Gy X-rays, 1 or 7 d before plating) were added to the upper compartment and allowed to migrate through the membrane for 24 h under normal culture conditions. Culture medium from the lower compartment was replaced by serum-free culture medium with 1 µM Calcein for 1 h (BD™ Calcein AM Fluorescent Dye, BD Biosciences). Cell culture inserts were transferred into trypsin/EDTA for 10 min to allow detachment of the migrated cells from the membrane. Fluorescence of the calcein-stained cells in trypsin/EDTA was immediately read in a fluorescence plate reader with 485 and 528 nm (Synergy HT, BioTek). Relative migration was calculated from the wells with chemoattractant (10% FCS) compared with the respective control wells with 0% FCS. Three independent experiments were performed, each in triplicate.
RNA extraction, RT-PCR and quantitative real-time RT-PCR
Total RNA was extracted from subconfluent cell cultures in T-25 flasks using RNeasy Mini kit (Qiagen) according to the manufacturer’s protocol. RNA was treated with RNase-free DNase (Fermentas). 100 ng (RT-PCR) or 50 ng (quantitative RT-PCR) of the pretreated RNA was applied into each PCR reaction. RT-PCR was performed using Masterscript™ RT-PCR system (5prime) according to the manufacturer’s protocol. All primers were purchased from Metabion and are listed in . Amplificates were separated using 2% agarose gels. In order to confirm accuracy of the RT-PCR reaction we used equally processed mRNA of endothelial cells from umbilical vein (HUVEC) or aorta (HAEC) as positive controls. For further analysis of EphA2, EphA3, ephrinA1 and ephrinA5 expression quantitative real-time RT-PCR was performed as described previously.Citation6 MRNA levels of Eph and ephrins were determined using the delta-delta CT method (2-ΔΔCT) vs. the 18S rRNA. For each cell line three independent RNA samples were analyzed, each in triplicate.
Table 2. Primer sequences, annealing temperatures, expected sizes of amplified products and number of PCR cycles used for RT-PCR. Primers for transcripts of EphA2, EphA3, ephrinA1, ephrinA5 and 18S rRNA were further used for quantitative real-time RT-PCR
Protein quantification by western blotting and immunodetection
Protein extracts were prepared from subconfluent cell cultures of untreated or treated cells (5 or 10 Gy X-rays, 1 or 7 d before lysis). Cells were washed with ice-cold PBS and incubated for 10 min on ice in modified RIPA buffer (50 mM Tris pH 8.0, 150 mM NaCl, 1% Nonidet P-40, 0.5% sodium deoxycholate 0.1% sodium dodecyl sulfate, 1 mM sodium fluoride, 1 mM sodium orthovanadate, 1 mM phenylmethylsulfonyl fluoride, 1 µg/ml leupeptin, 1 mM dithiothreitol). Cells were scraped off, cell lysate was transferred to a microcentrifuge tube and applied to ultrasound. After additional 10 min incubation on ice, cell lysates were spinned at 12,000 rpm at 4°C for 15 min and supernatant was transferred into a fresh tube. Protein content was determined using BCA protein assay (Fisher Scientific) according to the manufacturer’s protocol. Equal amounts of protein were separated in a 7.5% or 10% SDS polyacrylamide gel and then transferred to a PVDF membrane (Whatman Westran CS, Fisher Scientific) using a semi-dry transfer system (Bio-Rad Laboratories). Unspecific binding sites were blocked with 5% nonfat dried milk, 2% BSA, 0.05% Tween-20 in tris-buffered saline (TBS, for detecting EphA2, Src, phosphorylated Src, FAK and phosphorylated FAK) or with 5% nonfat dried milk in TBS (detection of EphA3). The following antibodies were used and incubated in blocking solution at 4°C overnight, rabbit polyclonal anti-EphA2 (sc-924), rabbit polyclonal anti-EphA3 (sc-920, both from Santa Cruz Biotechnology), rabbit polyclonal anti-Phospho-Src family (Tyr416) (#2101), rabbit monoclonal anti-Src (#2123), rabbit polyclonal anti-Phospho-FAK (Tyr576/577) (#3281) and rabbit polyclonal anti-FAK (#3285, all four from Cell Signaling Technology). A rabbit anti-actin antibody (A5060, Sigma-Aldrich) was used to verify equal protein amount. Membranes were washed three times in TBS, 0.05% Tween-20 and incubated with the secondary goat anti-rabbit IgG conjugated with horseradish peroxidase (A0545, Sigma-Aldrich). Finally, proteins were visualized using Super Signal West Pico and Super Signal West Dura Chemiluminescent Substrate (Fisher Scientific) and an MF-ChemiBis 3.2 imaging system (Biostep). For each cell line and treatment three independent cell lysates were analyzed.
Immunoprecipitation
Protein extracts were prepared as described above. One milligram protein extract was incubated for 1 h at 4°C with Dynabeads Protein A/G (Life Technologies) to reduce unspecific binding of proteins to the beads. Beads were removed and protein extracts were incubated with 2 µg of EphA2 antibody overnight at 4°C. Dynabeads Protein A was added and incubated for another 3 h at 4°C on an overhead shaker. Complexes of Dynabeads, bound antibody and the immunoprecipitated protein were washed four times in ice-cold modified RIPA buffer and finally subjected to SDS PAGE and western blot as described above. Membranes were blocked with 2% BSA, followed by incubation with mouse monoclonal anti-Phosphotyrosine (clone 4G10, Millipore) antibody. Membranes were washed, incubated with the secondary horseradish peroxidase-conjugated rabbit-anti-mouse antibody (A9044, Sigma-Aldrich) and processed with chemiluminescent substrate. Further, membranes were stripped (50 mM Tris, 2% SDS, 0.7% β-mercaptoethanol) and reprobed with EphA2 antibody as described above. Four independent experiments were performed.
Statistical analysis
The number of experiments and samples per data point are indicated in each figure and in the respective methods section. The data points of parallel experiments were averaged and the standard error of the mean (SEM) was calculated. For statistical analysis of proliferation, adhesion and plating efficiency, non-parametric Mann-Whitney U test was applied, whereas viability, motility, migration and quantitative RT-PCR were analyzed by one-way analysis of variance (ANOVA) with post hoc Bonferroni method using SPSS statistical software package (version 12.0 for Windows, SPSS Inc.). If not stated otherwise, p ≤ 0.05 was defined as significant.
Abbreviations: | ||
EGFR | = | epidermal growth factor receptor |
Eph | = | erythropoietin-producing hepatocellular carcinoma |
FAK | = | focal adhesion kinase |
Src | = | sarcoma tyrosine kinase |
Acknowledgments
The excellent technical assistance of Aline Morgenegg, Catharina Heinig, Regina Herrlich, Sonja Lehnert, Mareike Barth, Tobias Hoche and Nicole Kunath is greatly acknowledged. The authors also thank Dr Ralf Bergmann for his advice and many stimulating discussions. This work is part of a research initiative within the Helmholtz-Portfoliothema “Technologie und Medizin?Multimodale Bildgebung zur Aufklaerung des In-vivo-Verhaltens von polymeren Biomaterialien.”
Disclosure of Potential Conflicts of Interest
No potential conflicts of interest were disclosed.
References
- Kargiotis O, Geka A, Rao JS, Kyritsis AP. Effects of irradiation on tumor cell survival, invasion and angiogenesis. J Neurooncol 2010; 100:323 - 38; http://dx.doi.org/10.1007/s11060-010-0199-4; PMID: 20449629
- Akino Y, Teshima T, Kihara A, Kodera-Suzumoto Y, Inaoka M, Higashiyama S, et al. Carbon-ion beam irradiation effectively suppresses migration and invasion of human non-small-cell lung cancer cells. Int J Radiat Oncol Biol Phys 2009; 75:475 - 81; http://dx.doi.org/10.1016/j.ijrobp.2008.12.090; PMID: 19735871
- Cordes N, Hansmeier B, Beinke C, Meineke V, van Beuningen D. Irradiation differentially affects substratum-dependent survival, adhesion, and invasion of glioblastoma cell lines. Br J Cancer 2003; 89:2122 - 32; http://dx.doi.org/10.1038/sj.bjc.6601429; PMID: 14647148
- Goetze K, Scholz M, Taucher-Scholz G, Mueller-Klieser W. Tumor cell migration is not influenced by p21 in colon carcinoma cell lines after irradiation with X-ray or (12)C heavy ions. Radiat Environ Biophys 2010; 49:427 - 35; http://dx.doi.org/10.1007/s00411-010-0297-x; PMID: 20535615
- Ristic-Fira AM, Todorovic DV, Koricanac LB, Petrovic IM, Valastro LM, Cirrone PG, et al. Response of a human melanoma cell line to low and high ionizing radiation. Ann N Y Acad Sci 2007; 1095:165 - 74; http://dx.doi.org/10.1196/annals.1397.020; PMID: 17404029
- Mosch B, Mueller K, Steinbach J, Pietzsch J. Influence of irradiation on metabolism and metastatic potential of B16-F10 melanoma cells. Int J Radiat Biol 2009; 85:1002 - 12; http://dx.doi.org/10.3109/09553000903258871; PMID: 19895277
- Baluna RG, Eng TY, Thomas CR. Adhesion molecules in radiotherapy. Radiat Res 2006; 166:819 - 31; http://dx.doi.org/10.1667/RR0380.1; PMID: 17149971
- Jung JW, Hwang SY, Hwang JS, Oh ES, Park S, Han IO. Ionising radiation induces changes associated with epithelial-mesenchymal transdifferentiation and increased cell motility of A549 lung epithelial cells. Eur J Cancer 2007; 43:1214 - 24; http://dx.doi.org/10.1016/j.ejca.2007.01.034; PMID: 17379505
- Ogata T, Teshima T, Kagawa K, Hishikawa Y, Takahashi Y, Kawaguchi A, et al. Particle irradiation suppresses metastatic potential of cancer cells. Cancer Res 2005; 65:113 - 20; PMID: 15665286
- Onoda JM, Kantak SS, Piechocki MP, Awad W, Chea R, Liu B, et al. Inhibition of radiation-enhanced expression of integrin and metastatic potential in B16 melanoma cells by a lipoxygenase inhibitor. Radiat Res 1994; 140:410 - 8; http://dx.doi.org/10.2307/3579120; PMID: 7972695
- Tsutsumi K, Tsuda M, Yazawa N, Nakamura H, Ishihara S, Haga H, et al. Increased motility and invasiveness in tumor cells that survive 10 Gy irradiation. Cell Struct Funct 2009; 34:89 - 96; http://dx.doi.org/10.1247/csf.09006; PMID: 19724156
- Fujita M, Otsuka Y, Yamada S, Iwakawa M, Imai T. X-ray irradiation and Rho-kinase inhibitor additively induce invasiveness of the cells of the pancreatic cancer line, MIAPaCa-2, which exhibits mesenchymal and amoeboid motility. Cancer Sci 2011; 102:792 - 8; http://dx.doi.org/10.1111/j.1349-7006.2011.01852.x; PMID: 21214671
- Wild-Bode C, Weller M, Rimner A, Dichgans J, Wick W. Sublethal irradiation promotes migration and invasiveness of glioma cells: implications for radiotherapy of human glioblastoma. Cancer Res 2001; 61:2744 - 50; PMID: 11289157
- Camphausen K, Moses MA, Beecken WD, Khan MK, Folkman J, O’Reilly MS. Radiation therapy to a primary tumor accelerates metastatic growth in mice. Cancer Res 2001; 61:2207 - 11; PMID: 11280788
- Rofstad EK, Mathiesen B, Galappathi K. Increased metastatic dissemination in human melanoma xenografts after subcurative radiation treatment: radiation-induced increase in fraction of hypoxic cells and hypoxia-induced up-regulation of urokinase-type plasminogen activator receptor. Cancer Res 2004; 64:13 - 8; http://dx.doi.org/10.1158/0008-5472.CAN-03-2658; PMID: 14729600
- Akimoto T, Mitsuhashi N, Saito Y, Ebara T, Niibe H. Effect of radiation on the expression of E-cadherin and alpha-catenin and invasive capacity in human lung cancer cell line in vitro. Int J Radiat Oncol Biol Phys 1998; 41:1171 - 6; http://dx.doi.org/10.1016/S0360-3016(98)00176-X; PMID: 9719129
- Chatterjee A, Rojas A, Hodgkiss RJ. Induction of lethal mutations in experimental tumours after single and fractionated irradiations in vivo. Int J Radiat Biol 1998; 74:119 - 27; http://dx.doi.org/10.1080/095530098141780; PMID: 9687981
- Liu ZZ, Huang WY, Lin JS, Li XS, Liang KH, Huang JL. Cell cycle and radiosensitivity of progeny of irradiated primary cultured human hepatocarcinoma cells. World J Gastroenterol 2005; 11:7033 - 5; PMID: 16437612
- Wimmer-Kleikamp SH, Lackmann M. Eph-modulated cell morphology, adhesion and motility in carcinogenesis. IUBMB Life 2005; 57:421 - 31; http://dx.doi.org/10.1080/15216540500138337; PMID: 16012051
- Cheng N, Brantley DM, Chen J. The ephrins and Eph receptors in angiogenesis. Cytokine Growth Factor Rev 2002; 13:75 - 85; http://dx.doi.org/10.1016/S1359-6101(01)00031-4; PMID: 11750881
- Kullander K, Klein R. Mechanisms and functions of Eph and ephrin signalling. Nat Rev Mol Cell Biol 2002; 3:475 - 86; http://dx.doi.org/10.1038/nrm856; PMID: 12094214
- Castaño J, Davalos V, Schwartz S Jr., Arango D. EPH receptors in cancer. Histol Histopathol 2008; 23:1011 - 23; PMID: 18498077
- Mosch B, Reissenweber B, Neuber C, Pietzsch J. Eph receptors and ephrin ligands: important players in angiogenesis and tumor angiogenesis. J Oncol 2010; 2010:135285; http://dx.doi.org/10.1155/2010/135285; PMID: 20224755
- Pasquale EB. Eph receptors and ephrins in cancer: bidirectional signalling and beyond. Nat Rev Cancer 2010; 10:165 - 80; http://dx.doi.org/10.1038/nrc2806; PMID: 20179713
- Kinch MS, Carles-Kinch K. Overexpression and functional alterations of the EphA2 tyrosine kinase in cancer. Clin Exp Metastasis 2003; 20:59 - 68; http://dx.doi.org/10.1023/A:1022546620495; PMID: 12650608
- Easty DJ, Hill SP, Hsu MY, Fallowfield ME, Florenes VA, Herlyn M, et al. Up-regulation of ephrin-A1 during melanoma progression. Int J Cancer 1999; 84:494 - 501; http://dx.doi.org/10.1002/(SICI)1097-0215(19991022)84:5<494::AID-IJC8>3.0.CO;2-O; PMID: 10502726
- Margaryan NV, Strizzi L, Abbott DE, Seftor EA, Rao MS, Hendrix MJ, et al. EphA2 as a promoter of melanoma tumorigenicity. Cancer Biol Ther 2009; 8:279 - 88; http://dx.doi.org/10.4161/cbt.8.3.7485; PMID: 19223760
- Fang WB, Brantley-Sieders DM, Parker MA, Reith AD, Chen J. A kinase-dependent role for EphA2 receptor in promoting tumor growth and metastasis. Oncogene 2005; 24:7859 - 68; http://dx.doi.org/10.1038/sj.onc.1208937; PMID: 16103880
- Zelinski DP, Zantek ND, Stewart JC, Irizarry AR, Kinch MS. EphA2 overexpression causes tumorigenesis of mammary epithelial cells. Cancer Res 2001; 61:2301 - 6; PMID: 11280802
- Walker-Daniels J, Coffman K, Azimi M, Rhim JS, Bostwick DG, Snyder P, et al. Overexpression of the EphA2 tyrosine kinase in prostate cancer. Prostate 1999; 41:275 - 80; http://dx.doi.org/10.1002/(SICI)1097-0045(19991201)41:4<275::AID-PROS8>3.0.CO;2-T; PMID: 10544301
- Brannan JM, Sen B, Saigal B, Prudkin L, Behrens C, Solis L, et al. EphA2 in the early pathogenesis and progression of non-small cell lung cancer. Cancer Prev Res (Phila) 2009; 2:1039 - 49; http://dx.doi.org/10.1158/1940-6207.CAPR-09-0212; PMID: 19934338
- Li X, Wang L, Gu JW, Li B, Liu WP, Wang YG, et al. Up-regulation of EphA2 and down-regulation of EphrinA1 are associated with the aggressive phenotype and poor prognosis of malignant glioma. Tumour Biol 2010; 31:477 - 88; http://dx.doi.org/10.1007/s13277-010-0060-6; PMID: 20571968
- Yuan WJ, Ge J, Chen ZK, Wu SB, Shen H, Yang P, et al. Over-expression of EphA2 and EphrinA-1 in human gastric adenocarcinoma and its prognostic value for postoperative patients. Dig Dis Sci 2009; 54:2410 - 7; http://dx.doi.org/10.1007/s10620-008-0649-4; PMID: 19101799
- Brantley DM, Cheng N, Thompson EJ, Lin Q, Brekken RA, Thorpe PE, et al. Soluble Eph A receptors inhibit tumor angiogenesis and progression in vivo. Oncogene 2002; 21:7011 - 26; http://dx.doi.org/10.1038/sj.onc.1205679; PMID: 12370823
- Hess AR, Seftor EA, Gardner LM, Carles-Kinch K, Schneider GB, Seftor RE, et al. Molecular regulation of tumor cell vasculogenic mimicry by tyrosine phosphorylation: role of epithelial cell kinase (Eck/EphA2). Cancer Res 2001; 61:3250 - 5; PMID: 11309274
- Miao H, Wei BR, Peehl DM, Li Q, Alexandrou T, Schelling JR, et al. Activation of EphA receptor tyrosine kinase inhibits the Ras/MAPK pathway. Nat Cell Biol 2001; 3:527 - 30; http://dx.doi.org/10.1038/35074604; PMID: 11331884
- Yang NY, Fernandez C, Richter M, Xiao Z, Valencia F, Tice DA, et al. Crosstalk of the EphA2 receptor with a serine/threonine phosphatase suppresses the Akt-mTORC1 pathway in cancer cells. Cell Signal 2011; 23:201 - 12; http://dx.doi.org/10.1016/j.cellsig.2010.09.004; PMID: 20837138
- Zantek ND, Azimi M, Fedor-Chaiken M, Wang B, Brackenbury R, Kinch MS. E-cadherin regulates the function of the EphA2 receptor tyrosine kinase. Cell Growth Differ 1999; 10:629 - 38; PMID: 10511313
- Straume O, Akslen LA. Importance of vascular phenotype by basic fibroblast growth factor, and influence of the angiogenic factors basic fibroblast growth factor/fibroblast growth factor receptor-1 and ephrin-A1/EphA2 on melanoma progression. Am J Pathol 2002; 160:1009 - 19; http://dx.doi.org/10.1016/S0002-9440(10)64922-X; PMID: 11891198
- Buettner R, Mesa T, Vultur A, Lee F, Jove R. Inhibition of Src family kinases with dasatinib blocks migration and invasion of human melanoma cells. Mol Cancer Res 2008; 6:1766 - 74; http://dx.doi.org/10.1158/1541-7786.MCR-08-0169; PMID: 19010823
- Miao H, Burnett E, Kinch M, Simon E, Wang B. Activation of EphA2 kinase suppresses integrin function and causes focal-adhesion-kinase dephosphorylation. Nat Cell Biol 2000; 2:62 - 9; http://dx.doi.org/10.1038/35000008; PMID: 10655584
- Miao H, Li DQ, Mukherjee A, Guo H, Petty A, Cutter J, et al. EphA2 mediates ligand-dependent inhibition and ligand-independent promotion of cell migration and invasion via a reciprocal regulatory loop with Akt. Cancer Cell 2009; 16:9 - 20; http://dx.doi.org/10.1016/j.ccr.2009.04.009; PMID: 19573808
- Taddei ML, Parri M, Angelucci A, Onnis B, Bianchini F, Giannoni E, et al. Kinase-dependent and -independent roles of EphA2 in the regulation of prostate cancer invasion and metastasis. Am J Pathol 2009; 174:1492 - 503; http://dx.doi.org/10.2353/ajpath.2009.080473; PMID: 19264906
- Chiari R, Hames G, Stroobant V, Texier C, Maillère B, Boon T, et al. Identification of a tumor-specific shared antigen derived from an Eph receptor and presented to CD4 T cells on HLA class II molecules. Cancer Res 2000; 60:4855 - 63; PMID: 10987298
- Clifford N, Smith LM, Powell J, Gattenlöhner S, Marx A, O’Connor R. The EphA3 receptor is expressed in a subset of rhabdomyosarcoma cell lines and suppresses cell adhesion and migration. J Cell Biochem 2008; 105:1250 - 9; http://dx.doi.org/10.1002/jcb.21926; PMID: 18814179
- Fox BP, Kandpal RP. Invasiveness of breast carcinoma cells and transcript profile: Eph receptors and ephrin ligands as molecular markers of potential diagnostic and prognostic application. Biochem Biophys Res Commun 2004; 318:882 - 92; http://dx.doi.org/10.1016/j.bbrc.2004.04.102; PMID: 15147954
- Nakamoto M, Bergemann AD. Diverse roles for the Eph family of receptor tyrosine kinases in carcinogenesis. Microsc Res Tech 2002; 59:58 - 67; http://dx.doi.org/10.1002/jemt.10177; PMID: 12242697
- Xi HQ, Zhao P. Clinicopathological significance and prognostic value of EphA3 and CD133 expression in colorectal carcinoma. J Clin Pathol 2011; 64:498 - 503; http://dx.doi.org/10.1136/jcp.2010.087213; PMID: 21415057
- Shi G, Yue G, Zhou R. EphA3 functions are regulated by collaborating phosphotyrosine residues. Cell Res 2010; 20:1263 - 75; http://dx.doi.org/10.1038/cr.2010.115; PMID: 20697431
- Vearing C, Lee FT, Wimmer-Kleikamp S, Spirkoska V, To C, Stylianou C, et al. Concurrent binding of anti-EphA3 antibody and ephrin-A5 amplifies EphA3 signaling and downstream responses: potential as EphA3-specific tumor-targeting reagents. Cancer Res 2005; 65:6745 - 54; http://dx.doi.org/10.1158/0008-5472.CAN-05-0758; PMID: 16061656
- Hu T, Shi G, Larose L, Rivera GM, Mayer BJ, Zhou R. Regulation of process retraction and cell migration by EphA3 is mediated by the adaptor protein Nck1. Biochemistry 2009; 48:6369 - 78; http://dx.doi.org/10.1021/bi900831k; PMID: 19505147
- Knöll B, Drescher U. Src family kinases are involved in EphA receptor-mediated retinal axon guidance. J Neurosci 2004; 24:6248 - 57; http://dx.doi.org/10.1523/JNEUROSCI.0985-04.2004; PMID: 15254079
- Lisabeth EM, Fernandez C, Pasquale EB. Cancer somatic mutations disrupt functions of the EphA3 receptor tyrosine kinase through multiple mechanisms. Biochemistry 2012; 51:1464 - 75; http://dx.doi.org/10.1021/bi2014079; PMID: 22242939
- Nojiri K, Iwakawa M, Ichikawa Y, Imadome K, Sakai M, Nakawatari M, et al. The proangiogenic factor ephrin-A1 is up-regulated in radioresistant murine tumor by irradiation. Exp Biol Med (Maywood) 2009; 234:112 - 22; http://dx.doi.org/10.3181/0806-RM-189; PMID: 18997097
- von Essen CF. Radiation enhancement of metastasis: a review. Clin Exp Metastasis 1991; 9:77 - 104; http://dx.doi.org/10.1007/BF01756381; PMID: 2032423
- Zhang T, Hua R, Xiao W, Burdon KP, Bhattacharya SS, Craig JE, et al. Mutations of the EPHA2 receptor tyrosine kinase gene cause autosomal dominant congenital cataract. Hum Mutat 2009; 30:E603 - 11; http://dx.doi.org/10.1002/humu.20995; PMID: 19306328
- Bae HJ, Song JH, Noh JH, Kim JK, Jung KH, Eun JW, et al. Low frequency mutation of the Ephrin receptor A3 gene in hepatocellular carcinoma. Neoplasma 2009; 56:331 - 4; http://dx.doi.org/10.4149/neo_2009_04_331; PMID: 19469653
- Kung B, Zhao H, Hicks SL, Tang XX, Ikegaki N. Biological significance of EPHA2 expression in neuroblastoma. Int J Oncol 2009; 35:845 - 50; PMID: 19724921
- Dottori M, Down M, Hüttmann A, Fitzpatrick DR, Boyd AW. Cloning and characterization of EphA3 (Hek) gene promoter: DNA methylation regulates expression in hematopoietic tumor cells. Blood 1999; 94:2477 - 86; PMID: 10498621
- Essa S, Denzer N, Mahlknecht U, Klein R, Collnot EM, Tilgen W, et al. VDR microRNA expression and epigenetic silencing of vitamin D signaling in melanoma cells. J Steroid Biochem Mol Biol 2010; 121:110 - 3; http://dx.doi.org/10.1016/j.jsbmb.2010.02.003; PMID: 20153427
- Reu FJ, Bae SI, Cherkassky L, Leaman DW, Lindner D, Beaulieu N, et al. Overcoming resistance to interferon-induced apoptosis of renal carcinoma and melanoma cells by DNA demethylation. J Clin Oncol 2006; 24:3771 - 9; http://dx.doi.org/10.1200/JCO.2005.03.4074; PMID: 16801630
- Parri M, Buricchi F, Giannoni E, Grimaldi G, Mello T, Raugei G, et al. EphrinA1 activates a Src/focal adhesion kinase-mediated motility response leading to rho-dependent actino/myosin contractility. J Biol Chem 2007; 282:19619 - 28; http://dx.doi.org/10.1074/jbc.M701319200; PMID: 17449913
- Parri M, Taddei ML, Bianchini F, Calorini L, Chiarugi P. EphA2 reexpression prompts invasion of melanoma cells shifting from mesenchymal to amoeboid-like motility style. Cancer Res 2009; 69:2072 - 81; http://dx.doi.org/10.1158/0008-5472.CAN-08-1845; PMID: 19244130
- Lawrenson ID, Wimmer-Kleikamp SH, Lock P, Schoenwaelder SM, Down M, Boyd AW, et al. Ephrin-A5 induces rounding, blebbing and de-adhesion of EphA3-expressing 293T and melanoma cells by CrkII and Rho-mediated signalling. J Cell Sci 2002; 115:1059 - 72; PMID: 11870224
- Franken NA, Rodermond HM, Stap J, Haveman J, van Bree C. Clonogenic assay of cells in vitro. Nat Protoc 2006; 1:2315 - 9; http://dx.doi.org/10.1038/nprot.2006.339; PMID: 17406473
- Fox BP, Tabone CJ, Kandpal RP. Potential clinical relevance of Eph receptors and ephrin ligands expressed in prostate carcinoma cell lines. Biochem Biophys Res Commun 2006; 342:1263 - 72; http://dx.doi.org/10.1016/j.bbrc.2006.02.099; PMID: 16516143
- Haase C, Bergmann R, Fuechtner F, Hoepping A, Pietzsch J. L-type amino acid transporters LAT1 and LAT4 in cancer: uptake of 3-O-methyl-6-18F-fluoro-L-dopa in human adenocarcinoma and squamous cell carcinoma in vitro and in vivo. J Nucl Med 2007; 48:2063 - 71; http://dx.doi.org/10.2967/jnumed.107.043620; PMID: 18056335