Abstract
Cell infiltration is a critical parameter for the successful development of 3D matrices for tissue engineering. Application of electrospun nanofibers in tissue engineering has recently attracted much attention. Notwithstanding several of their advantages, small pore size and small thickness of the electrospun layer limit their application for development of 3D scaffolds. Several methods for the pore size and/or electrospun layer thickness increase have been recently developed. Nevertheless, tissue engineering still needs emerging of either novel nanofiber-enriched composites or new techniques for 3D nanofiber fabrication. Forcespinning® seems to be a promising alternative. The potential of the Forcespinning® method is illustrated in preliminary experiment with mesenchymal stem cells.
Strategies to Increase the Pore Size of Electrospun Layers
Because of their structure, which mimics the natural extracellular matrix, nano- and microfibrous layers are promising materials for tissue engineering and for regenerative medicine.Citation1 They have some extraordinary properties, such as huge porosity, fiber diameter similar to that of fibrillar proteins, and an enormous surface-to-volume ratio. However, electrospun fibers have some disadvantages for tissue engineering applications, above all their limited thickness, their small pore size, and their planar 2D structure. Various strategies have been applied to overcome these disadvantages and to fabricate nanofibers with huge pores and a 3D structure.
Systems combining insoluble fibers and sacrificial co-fibers,Citation2,Citation3 salt leaching,Citation4 ice crystals,Citation5 and photopatterningCitation6 have been used to increase the pore size of prepared nanofibers. Ultrasonification in an aqueous solution is another physical method that has been used in the electrospinning process to extend the thickness and the porosity of the layer.Citation7 The application of ultrasound resulted in increased scaffold pore size and enhanced cellular infiltration and proliferation in vitro. In another study, PLGA nanofibers with Degrapol® (a bioresorbable polyurethane) as a sacrificial part of the system enabled deeper cell penetration to the scaffold.Citation8
In addition, systems employing patterned collectors have been used to increase the pore size. The use of classical non-patterned collectors results in homogeneous distribution of the electric field and random orientation of the nanofibers. Non-homogenous distribution of the electric field on the patterned collector results in changed deposition of the nanofibers, which correlates with the local electric field intensity and is driven by Coulombic forces.Citation9 Li et al.Citation9-Citation11 developed patterned static collectors consisting of conductive and non-conductive void spaces. Nanofibers were aligned across a non-conductive void. Another approach was introduced by Zussman et al.Citation12 They used a rotating table placed on a rotating disc moving 90° between the steps of deposition. The fibrous mesh that was produced had a square “mesh” orientation of the nanofibers, as the second layer was oriented perpendicular to the first layer. Another approach is based on parallelization of the nanofibers. Parallelizing the fibers increased the pore size of the meshes without altering the fiber diameter. For example, Zhu et al.Citation13 used a rotating frame cylinder for preparing a parallelized fibrous mesh. Vaquette and Cooper-WhiteCitation14 tested nanofibrous meshes deposited on patterned collectors. They found that increased pore size of a patterned mesh enabled deeper cell penetration to the scaffold. Recently, we introduced a studyCitation15 in which nanofibrous layers prepared using a structured collector were used as a scaffold for mesenchymal stem cell (MSC) seeding. The structure of the scaffold with increased pore size improved the cell proliferation and, moreover, the osteogenic differentiation of the cells, in comparison with a non-structured layer. A patterned surface with spots of different surface mass density caused increased pore sizes in the less dense areas. The pore sizes were huge enough to enable MSCs to penetrate through the fibrous layer. Migration of cells is very advantageous in bone tissue engineering applications.
Interestingly, the knitted-like structure of the fibrous mesh also improved the biomechanical properties of the mesh, which is of importance for tissue engineering of skin or tendon.
Strategies to Increase the Thickness of Electrospun Layers
In addition, various strategies were used to increase the thickness of the fibrous layers. One of the easiest ways to produce 3D fibrous structures is with the use of sequential or multilayering electrospinning. Using these methods, electrospun layers hundreds of microns in thickness can be produced. Han et al.Citation16 prepared a multilayered electrospun layer mimicking the ECM of the urinary bladder in a single-step process. Another way is to modify simple electrospun layer by bending, folding, or stacking. Chen et al.Citation17 prepared nanofiber structures with aligned or randomly oriented nanofibers. They overlaid five cell-seeded layers, and prepared two types of 3D scaffold, with aligned or random orientation of the nanofibers. An interesting method for preparing thicker nanofibrous layer was introduced by Shabani et al.Citation18 They used focused infrared irradiation during the electrospinning process. The lamps were arrayed in a manner such that their light was focused on the last third of the top-to-collector distance. As a result, a nanofibrous layer was prepared, which was 10 times thicker than the layer from conventional electrospinning and showed a less dense structure than when conventional electrospinning was used. In addition, special collectors were used to prepare 3D nanofibers. A liquid bath was used as a collector in an electrospinning technique known as “wet electrospinning.” The pore size and the thickness of the nanofibers that were produced was dramatically increased.Citation19 Blakeney et al.Citation20 demonstrated the use of a grounded spherical dish collector with an array of needle-like probes. The nanofibers that were prepared exhibited a loosely packed 3D structure with a ball-like morphology. The loose ball-like morphology enabled cell penetration into the scaffold.
Forcespinning as an Alternative Method for Producing 3D Nanofibers
Recently, Forcespinning® was introduced as a novel method for producing polymeric nanofibers. While electrospinning is based on the electrostatic forces between an electrically charged solution and a collector with the opposite charge, Forcespinning® uses a centrifugal force to produce ultra-thin fibers. As a consequence, Forcespinning® can prepare layers both from solutions and from melts. This eliminates some limitations of electrospinning, e.g., high electric voltage, and the need to use dielectric solvents. The Forcespinning® process has already been described in detail elsewhere.Citation21-Citation23 The device for Forcespinning® is composed of a spinneret loaded with a polymer solution or a melt. While the fiber is being produced, the polymer solution is drawn from the orifice by rotating forces. Subsequently, the solution is evaporated from the fibers and they are deposited on a collector. The main parameters influencing the Forcespinning® process are the centrifugal force that is used, the viscosity of the solution or melt, the diameter of the collector, the radius of the orifice, and solvent evaporation rate.Citation22 A well-balanced force must be used. It must be high enough to overcome the surface tension of the solution or melt, but not too high to form polymeric beads. Similarly, the viscosity of the polymer solution has to be chosen appropriately. If the viscosity is high, the forces being used to draw the fiber may not be strong enough to create a jet. However, beads can be produced when a solution with low viscosity is used. The diameter of the orifice is also an important factor in the process. A decrease in the diameter of the orifice reduced the overall diameter of the fibers. The orientation and the geometry of the orifice also have effect on fiber formation.Citation21 Another important parameter is the evaporation rate of the solvents from the polymer solution. If the evaporation rate of the solvent is too low, the fibers may be converted into a thin film as the layers build up on the collector and the fibers that are still wet merge. If the evaporation rate is relatively high, the elongation process of the polymer jet is disturbed, and fibers of large diameter are produced. The distance between the spinneret and the collector, which is determined by the diameter of the collector, is not as crucial as in electrospinning. The critical distance is too short to enable the fibers to be stretched. However, when this critical distance is exceeded, the reduction in fiber diameter with increasing distance becomes minimal. The Forcespinning® process enables relatively thick, fluffy fibrous layers to be produced. Scaffolds of this kind, with a highly porous 3D structure, are desired for tissue engineering applications.
Forcespinning® produces a loose and highly porous fibrous mesh. To the best of our knowledge, no study has been published describing the interaction of mesenchymal stem cells with Forcespun nanofibers. We therefore performed a preliminary experiment, in which scaffolds from PCL layers prepared by Forcespinning® were seeded with MSCs and visualized by confocal microscopy. Forcespinning® of 40% (w/v) PCL in chloroform:ethanol (volume ratio 9:1) were processed using Cyclone 1000 L/M Forcespinning® equipment with a metallic plate with a G30 or G20 orifice with a rotation speed of 10 000 RPM (). The samples were deposited either on a stationary deposition system or with the use of vacuum-assisted deposition. The meshes that we produced had different macroscopic and microscopic morphologies. While the bulk fiber mesh deposited on the stationary deposition system exhibited a loosely-packed 3D structure (Sample 1 and Sample 2 [], Forcespinning® conditions are listed in ), the fibers deposited by vacuum-assisted deposition exhibited a packed “electrospinning-like” morphology (Sample 3 []). The SEM measurements () showed that Forcespinning® resulted in the formation of nano- and microfibers. The diameters of the fibers for all samples were similar. The thin nanofibers had mean diameter of 0.76 µm, and the mean diameter of thick microfibers was around 2.3 µm. Stereological analysis showed differences between studied groups. Sample 1 had the biggest mean pore size (64.5 µm2) and the biggest maximal pore size (1592.3 µm2).
Figure 1. Morphology of layers produced by Forcespinning® technology (A). Macroscopic view of Sample 2 deposited by static deposition (B). Macroscopic view of Sample 3 deposited by vacuum assisted deposition (C). SEM morphology of Sample 1 (D), Sample 2 (E), Sample 3 (F). Scale bar 100 μm.
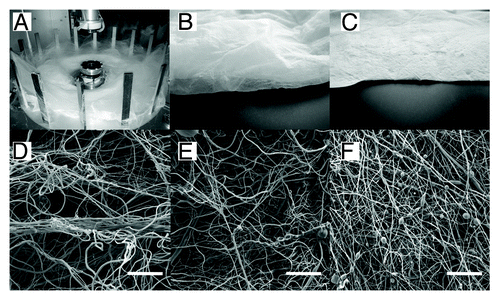
Table 1. Description of prepared micro/nano-fibers
The mean pore size of Sample 2 was smaller (42.1 µm2); however, it still was higher than Sample 3, which was deposited using vacuum-assisted deposition (29.8 µm2). All stereological data are showed in . Proportional representation of pore sizes in the samples is shown in . The samples were sterilized in 70% ethanol and seeded with porcine mesenchymal stem cells (approximately 5.4 × 105 cells/cm2). In order to visualize the penetration of the cells into the scaffolds, on day 6 after cell seeding, the samples were stained with DiOC and propidium iodide and were visualized by confocal microscopy, as was described previously.Citation15 Briefly, the samples were fixed in frozen methylalcohol (-20 ° C) and incubated with the fluorescent probe 3,3′-diethyloxacarbocyanine iodide (DiOC6; 0.1–1 µg/mL in PBS; pH 7.4) and subsequently with propidium iodide (PI; 5 µg/mL in PBS). A ZEISS LSM 5 DUO confocal microscope was used for visualizing the samples (PI: Xexc = 561 nm, Xem = 630–700 nm; DiOC6: Xexc = 488 nm, Xem = 505–550 nm). The results of the study showed that the MSCs on Sample 1 (50% PCL from G20 orifice at speed 10 000 RPM) penetrated through the scaffold to a depth greater than 250 µm (). This kind of deep cellular infiltration is sufficient for the formation of 3D scaffolds. However, the cells on Sample 2 and Sample 3 did not penetrate deeper than 50 µm (). This kind of penetration is similar to the penetration in electrospun nanofibers. On non-patterned 2D PCL nanofibers, the cell infiltration depth was 50 µm (), while on patterned 3D nanofibers, the infiltration depth was 80 µm (). The Forcespinning® method is able to produce 3D constructs, but the cell penetration is highly dependent on pore size. In the case of samples with high porosity and huge pores, the penetration rate overcame the electrospun nanofibers. These preliminary results suggest that Forcespun nanofibers have huge potential for tissue engineering applications where deep cell penetration is necessary. However, the method is relatively new, and it is necessary to carry out more detailed testing and optimization in order to take full advantage of the prepared scaffolds.
Figure 2. Proportional distribution of pore sizes determined from SEM stereological analysis. Different distributions of pore sizes were seen in the samples. While proportional representations of pores were similar in samples prepared by classic deposition (Sample 1 [A] and Sample 2 [B]), smaller pores predominated in Sample 3 [C]).
![Figure 2. Proportional distribution of pore sizes determined from SEM stereological analysis. Different distributions of pore sizes were seen in the samples. While proportional representations of pores were similar in samples prepared by classic deposition (Sample 1 [A] and Sample 2 [B]), smaller pores predominated in Sample 3 [C]).](/cms/asset/3ca8386d-14dd-4348-bea7-8260ae76c5c7/kcam_a_10927477_f0002.gif)
Figure 3. Confocal microscopy of MSCs seeded on fibers scaffolds prepared by Forcespinning®. Cells were stained with DiOC6 (green color) and propidium iodide (red color)—left column. Depth color projection was used to visualize distribution of the cells in scaffolds (right column). Cells penetrated to the depth more than 200 µm in scaffold Sample 1 (50% PCL, G20 orifice) (A and B) In the case of Sample 2—50% PCL, G30 orifice, classic deposition (C and D); and Sample 3—50% PCL, G30 orifice, CVD (E and F) the penetration was considerably smaller. The micrograph shows in figures A, C, and E mesenchymal stem cells stained with DiOC6 for membranes (green) and propidium iodide for nuclei (red). Figures B, D, and F represent color-coded depth profile of cells on scaffolds.
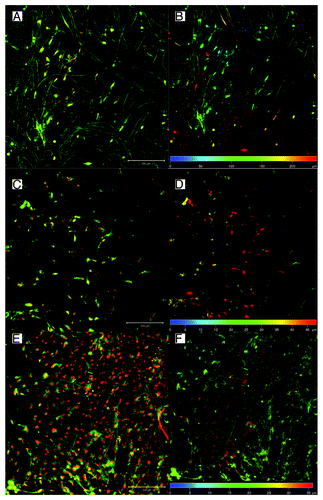
Figure 4. Penetration of mesenchymal stem cells to electrospun nanofibers on day 7. Penetration to non-patterned 2D nanofibers (A and B) and patterned 3D nanofibers (C and D). The micrograph shows in figures A and C MSCs stained with DiOC6 for membranes (green) and propidium iodide for nuclei (red). Figures B and D represents color-coded depth profile of cells on scaffolds.
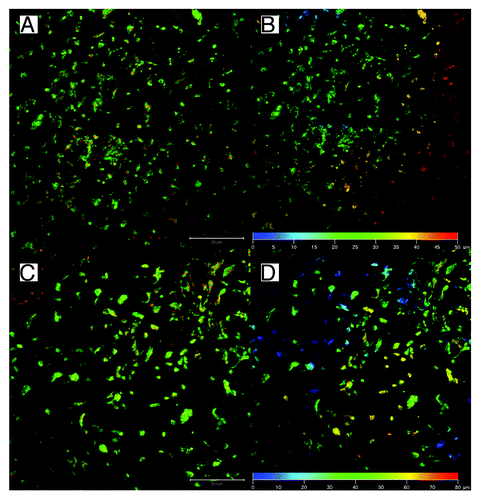
Conclusion
Electrospinning, a well-known nanofiber fabrication technique, efficiently form nanofibrous meshes from various polymers. However, the classical deposition technique often suffers from the formation of a fibrous mesh with small pore size, resulting in poor cellular infiltration. Cellular infiltration is critical for the formation of 3D tissue engineering constructs. Various strategies to increase the mean pore size and the thickness of the fibrous layer have therefore been proposed in recent years. Although the results of the studies are promising, the methods often use a complex experimental setup and their productivity is limited. However, a novel method based on centrifugal spinning may overcome these disadvantages. Forcespinning® technology enables the preparation of highly porous micro- and nanofibrous layers with considerable thickness. These properties are advantageous for tissue engineering applications, where 3D structured fibers with huge interconnected pores are necessary to enable the cells to migrate and communicate. The properties, the thickness, and the pore size of forcespun fibers are highly dependent on the device settings, which makes this method easily scalable for various applications.
Disclosure of Potential Conflicts of Interest
No potential conflicts of interest were disclosed.
Acknowledgments
This study was supported by the Academy of Sciences of the Czech Republic, institutional research plans AV0Z50390703, AV0Z50390512, and the Institutional Research Concept RVO 61388971, The Ministry of Education Youth and Sports of the Czech Republic (project IPv6), The Grant Agency of the Charles University (grant No., 626012, 384311, 270513, 330611, 648112), and Internal Grant Agency of the Ministry of Health of the Czech Republic (grant No. NT12156).
References
- Sill TJ, von Recum HA. Electrospinning: applications in drug delivery and tissue engineering. Biomaterials 2008; 29:1989 - 2006; http://dx.doi.org/10.1016/j.biomaterials.2008.01.011; PMID: 18281090
- Baker BM, Gee AO, Metter RB, Nathan AS, Marklein RA, Burdick JA, Mauck RL. The potential to improve cell infiltration in composite fiber-aligned electrospun scaffolds by the selective removal of sacrificial fibers. Biomaterials 2008; 29:2348 - 58; http://dx.doi.org/10.1016/j.biomaterials.2008.01.032; PMID: 18313138
- Guimarães A, Martins A, Pinho ED, Faria S, Reis RL, Neves NM. Solving cell infiltration limitations of electrospun nanofiber meshes for tissue engineering applications. Nanomedicine (Lond) 2010; 5:539 - 54; http://dx.doi.org/10.2217/nnm.10.31; PMID: 20528450
- Nam J, Huang Y, Agarwal S, Lannutti J. Improved cellular infiltration in electrospun fiber via engineered porosity. Tissue Eng 2007; 13:2249 - 57; http://dx.doi.org/10.1089/ten.2006.0306; PMID: 17536926
- Simonet M, Schneider OD, Neuenschwander P, Stark WJ. Ultraporous 3D polymer meshes by low-temperature electrospinning: Use of ice crystals as a removable void template. Polym Eng Sci 2007; 47:2020 - 6; http://dx.doi.org/10.1002/pen.20914
- Sundararaghavan HG, Metter RB, Burdick JA. Electrospun fibrous scaffolds with multiscale and photopatterned porosity. Macromol Biosci 2010; 10:265 - 70; http://dx.doi.org/10.1002/mabi.200900363; PMID: 20014198
- Lee JB, Jeong SI, Bae MS, Yang DH, Heo DN, Kim CH, Alsberg E, Kwon IK. Highly porous electrospun nanofibers enhanced by ultrasonication for improved cellular infiltration. Tissue Eng Part A 2011; 17:2695 - 702; http://dx.doi.org/10.1089/ten.tea.2010.0709; PMID: 21682540
- Milleret V, Simona B, Neuenschwander P, Hall H. Tuning electrospinning parameters for production of 3D-fiber-fleeces with increased porosity for soft tissue engineering applications. Eur Cell Mater 2011; 21:286 - 303; PMID: 21432783
- Li D, Wang Y, Xia Y. Electrospinning of Polymeric and Ceramic Nanofibers as Uniaxially Aligned Arrays. Nano Lett 2003; 3:1167 - 71; http://dx.doi.org/10.1021/nl0344256
- Li D, Wang Y, Xia Y. Electrospinning Nanofibers as Uniaxially Aligned Arrays and Layer-by-Layer Stacked Films. Adv Mater 2004; 16:361 - 6; http://dx.doi.org/10.1002/adma.200306226
- Li D, Ouyang G, McCann JT, Xia Y. Collecting electrospun nanofibers with patterned electrodes. Nano Lett 2005; 5:913 - 6; http://dx.doi.org/10.1021/nl0504235; PMID: 15884893
- Zussman E. Formation of nanofiber crossbars in electrospinning. Appl Phys Lett 2003; 82:973; http://dx.doi.org/10.1063/1.1544060
- Zhu X, Cui W, Li X, Jin Y. Electrospun fibrous mats with high porosity as potential scaffolds for skin tissue engineering. Biomacromolecules 2008; 9:1795 - 801; http://dx.doi.org/10.1021/bm800476u; PMID: 18578495
- Vaquette C, Cooper-White JJ. Increasing electrospun scaffold pore size with tailored collectors for improved cell penetration. Acta Biomater 2011; 7:2544 - 57; http://dx.doi.org/10.1016/j.actbio.2011.02.036; PMID: 21371575
- Rampichová M, Chvojka J, Buzgo M, Prosecká E, Mikeš P, Vysloužilová L, Tvrdík D, Kochová P, Gregor T, Lukáš D, et al. Elastic three-dimensional poly (ε-caprolactone) nanofibre scaffold enhances migration, proliferation and osteogenic differentiation of mesenchymal stem cells. Cell Prolif 2013; 46:23 - 37; http://dx.doi.org/10.1111/cpr.12001; PMID: 23216517
- Han D, Gouma P-I. Electrospun bioscaffolds that mimic the topology of extracellular matrix. Nanomedicine 2006; 2:37 - 41; PMID: 17292114
- Chen X, Fu X, Shi JG, Wang H. Regulation of the osteogenesis of pre-osteoblasts by spatial arrangement of electrospun nanofibers in two- and three-dimensional environments. Nanomedicine 2013; 9:1283 - 92; PMID: 23665421
- Shabani I, Haddadi-Asl V, Seyedjafari E, Soleimani M. Cellular infiltration on nanofibrous scaffolds using a modified electrospinning technique. Biochem Biophys Res Commun 2012; 423:50 - 4; http://dx.doi.org/10.1016/j.bbrc.2012.05.069; PMID: 22618233
- Ki CS, Park SY, Kim HJ, Jung HM, Woo KM, Lee JW, Park YH. Development of 3-D nanofibrous fibroin scaffold with high porosity by electrospinning: implications for bone regeneration. Biotechnol Lett 2008; 30:405 - 10; http://dx.doi.org/10.1007/s10529-007-9581-5; PMID: 17973083
- Blakeney BA, Tambralli A, Anderson JM, Andukuri A, Lim DJ, Dean DR, Jun HW. Cell infiltration and growth in a low density, uncompressed three-dimensional electrospun nanofibrous scaffold. Biomaterials 2011; 32:1583 - 90; http://dx.doi.org/10.1016/j.biomaterials.2010.10.056; PMID: 21112625
- Padron S, Fuentes A, Caruntu D, Lozano K. Experimental study of nanofiber production through forcespinning. J Appl Phys 2013; 113:024318 - 9; http://dx.doi.org/10.1063/1.4769886
- Dabirian F, Hosseini Ravandi SA, Pishevar AR, Abuzade RA. A comparative study of jet formation and nanofiber alignment in electrospinning and electrocentrifugal spinning systems. J Electrost 2011; 69:540 - 6; http://dx.doi.org/10.1016/j.elstat.2011.07.006
- Sarkar K, Gomez C, Zambrano S, Ramirez M, de Hoyos E, Vasquez H, Lozano K. Electrospinning to Forcespinning™. Mater Today 2010; 13:12 - 4; http://dx.doi.org/10.1016/S1369-7021(10)70199-1