Abstract
Cell migration is essential for a variety of fundamental biological processes such as embryonic development, wound healing, and immune response. Aberrant cell migration also underlies pathological conditions such as cancer metastasis, in which morphological transformation promotes spreading of cancer to new sites. Cell migration is driven by actin dynamics, which is the repeated cycling of monomeric actin (G-actin) into and out of filamentous actin (F-actin). CAP (Cyclase-associated protein, also called Srv2) is a conserved actin-regulatory protein, which is implicated in cell motility and the invasiveness of human cancers. It cooperates with another actin regulatory protein, cofilin, to accelerate actin dynamics. Hence, knockdown of CAP1 slows down actin filament turnover, which in most cells leads to reduced cell motility. However, depletion of CAP1 in HeLa cells, while causing reduction in dynamics, actually led to increased cell motility. The increases in motility are likely through activation of cell adhesion signals through an inside-out signaling. The potential to activate adhesion signaling competes with the negative effect of CAP1 depletion on actin dynamics, which would reduce cell migration. In this commentary, we provide a brief overview of the roles of mammalian CAP1 in cell migration, and highlight a likely mechanism underlying the activation of cell adhesion signaling and elevated motility caused by depletion of CAP1.
Introduction
The concentration of monomeric actin (G-actin) at which monomers are added to the lengthening end of filamentous actin (F-actin) at the same rate as subunits are released, called critical concentration, is ~0.1 μM in vitro even at physiological concentrations of salt.Citation1 In contrast, up to 50% of the total cytoplasmic actin in cells remains unpolymerized, despite being ~100-fold greater than the critical concentration.Citation2-Citation5 It is most likely that the high concentrations of G-actin are maintained by actin-binding proteins, which sequester G-actin to prevent polymerization.Citation6 One G-actin-sequestering protein is CAP (Cyclase-associated protein), which is conserved in all eukaryotes, and has been studied for the past two decades.Citation7 CAP was first identified in yeast where it regulates both Ras/cAMP signaling and the actin cytoskeleton, but the Ras function is confined to yeast, accordingly, most subsequent studies have focused on its role in the actin cytoskeleton.Citation7-Citation10 Mammalian CAP1 and yeast CAP both promote cofilin-driven actin filament turnover, which is believed to be a conserved feature among all CAP homologs based on the morphological phenotypes accompanying CAP perturbation, as well as the fact that expression of CAP homologs from other species rescues the actin phenotypes in CAP-knockout yeast.Citation7 Consistent with its role in the actin cytoskeleton, recent reports also support involvement of mammalian CAP in the invasiveness of a variety of human cancers.Citation11-Citation14 Mammals have two CAP isoforms, in CAP1 and CAP2;Citation7,Citation15 CAP1 is more widely expressed and studied. The main focus of this commentary is on the role of mammalian CAP1 in cell migration, through its role in the actin filament dynamics and potential involvement in cell adhesion signaling. However, we also include some discussion on yeast CAP, because most properties are shared between mammalian CAP1 and yeast CAP.
CAP1 as a Key Actin-Regulatory Protein that Promotes Cofilin-Driven Actin Filament Turnover
The first direct evidence that demonstrates CAP is a cytoskeletal protein is the finding that yeast CAP knockouts had two distinct phenotypes, the first was reduced cAMP signaling and the second was an aberrant cytoskeleton.Citation16 Subsequently, it was shown that both yeast and human homologs could bind and sequester G-actin.Citation17,Citation18 Most CAP homologs consist of three structural domains, namely the N terminus domain (NT), the C terminus domain (CT), and the proline-rich middle domain.Citation7,Citation15 The NT is structurally less conserved compared with the CT and the middle domain and the G-actin sequestering capability was first mapped to the CT of both mammalian CAP1 and yeast CAP, although later a second G-actin binding site consisting of a WH2 (Wasp Homology 2) domain was also found.Citation7 All three domains of mammalian CAP1 and yeast CAP contribute to promoting actin filament turnover through interactions with cofilin as well as both G- and F-actin.Citation7 While studies on yeast CAP took the lead initially, the last decade has seen substantial progress in understanding how mammalian CAP1 regulates actin filament dynamics, which has revealed some distinct aspects from the case of yeast CAP by comparison.Citation7,Citation19,Citation20 Yeast CAP preferentially binds ADP-G-actin,Citation21 whereupon the WH2 domain plays a central role to accelerate nucleotide exchange of ATP onto ADP-G-actin.Citation22 Nucleotide exchange is believed to be a rate-limiting step in actin dynamics promoted by cofilin, because the G-actin-cofilin complex resulting from severing F-actin exchanges nucleotides poorly.Citation23 Like yeast CAP, a recent report showed that mouse CAP1 also catalyzes nucleotide exchange on the ADP-G-actin bound to the CT domain. Interestingly, however, the β-sheet domain within the CT of mouse CAP1 is essential and sufficient for catalyzing nucleotide exchange on actin monomers, while the WH2 domain is not required for this function in mouse CAP1.Citation20 Finally, the NT of both yeast CAP and mammalian CAP1 promotes cofilin-mediated actin filament turnover through binding the cofilin-G-actin complex.Citation7 The NT also interacts with cofilin-bound F-actin to promote filament severing.Citation7,Citation19,Citation24 For more details on the role of CAP in actin filament dynamics, please see the recent comprehensive review by Ono.Citation7 Taken together, CAP is truly a key actin-regulatory protein that promotes actin filament turnover through multiple mechanisms mediated by its separate domains.
While most data suggesting that CAP1 interacts with cofilin are from in vitro studies, there is some evidence that they interact in cells because depletion of mammalian CAP1 causes cofilin to accumulate into cytoplasmic aggregatesCitation5,Citation25 and remain dephosphorylated.Citation5 Aggregation is likely to prevent cofilin from functioning to facilitate actin filament dynamics.
Effects of CAP1 Depletion on the Actin Cytoskeleton and Cell Migration
As noted above, rearrangement of the actin cytoskeleton is essential for cell migration. As a key actin-regulatory protein, changes in CAP1 level or its “activity” is expected to impact cell migration through effects on actin dynamics. Directional cell movement also requires cell polarization to establish the leading edge of the cell through asymmetric assembly and disassembly of F-actin-rich structures such as filopodia and lamellipodia. Available evidence from a number of species such as DictyosteliumCitation7,Citation26 suggests that CAP1 may facilitate cell polarization. In mammalian cells, CAP1 is enriched at the leading edge of migrating cells,Citation7,Citation25,Citation27 and we have also observed similar localization in multiple mammalian cell lines such as HeLa and hTERT-HPNE pancreas cells (unpublished observations). We speculate that CAP1 at the leading edge may promote local actin dynamics, which facilitates cell polarization and subsequent migration. However, it is noteworthy that the majority of CAP1 typically shows a diffuse localization across the cytosol in mammalian cells, and in Dictyostelium cells, CAP even localizes to the posterior cortex.Citation28 While localization to the leading edge suggests a possible role in cell polarization, there are no reports yet that knockdown cells have reduced polarity.
Gene silencing has been a useful approach in assessing the roles of mammalian CAP1 in the actin cytoskeleton and cell migration. In CAP1-depleted NIH3T3 fibroblasts, B16F1, and HeLa cells, common actin cytoskeletal (and morphological) alterations observed include enhanced actin stress fibers and enlarged cell size.Citation5,Citation25 The enhanced stress fibers in the CAP1 knockdowns are likely to be caused by the reduced rate of the actin filament turnover and reduced G-actin-sequestering. While stress fibers generate tension force needed to pull the trailing edge forward during cell movement, enhanced stress fibers is typically associated with reduced motility.Citation29 Thus, it was not surprising that CAP1 depletion reduced cell motility in several cell types, including cancer cells.Citation12-Citation14,Citation25
In contrast to the reduced motility in some cell types, CAP1 knockdown in HeLa cells, however, actually stimulated cell motility and invasion.Citation5 The CAP1-knockdown HeLa cells also had increased lamellipodia, which could explain the observed cell migration phenotype. Furthermore, CAP1-knockdown HeLa cells had significantly enhanced cell spreading and adhesion.Citation5 Cell adhesion, through integrin activation, plays an important role in cell migration as it provides traction force essential for cell migration.Citation30 Sufficient traction force will not be generated if there is too little adhesion for the cells to attach well; on the other hand, too much cell adhesion causes abnormally strong cell attachment, which will also hinder motility. We propose that the increased adhesion in the CAP1-knockdown HeLa cells had a positive effect on cell motility because these cells are not strongly adherent, so as a result, the positive effects of increased lamellipodia and enhanced adhesion more than offset the negative effects caused by reduced actin dynamics.
CAP1 Depletion Likely Activates Integrin Pathway Through Inside-Out Signaling
The stimulation of cell migration by CAP1 depletion may depend on cell adhesion signaling through the FAK pathway. FAK (Focal Adhesion Kinase) is a kinase that regulates cell adhesion and spreading as well as cell motility;Citation31,Citation32 CAP1 knockdown in HeLa cells stimulated FAK phosphorylation at Y397.Citation5 Y397 is an autophosphorylation site whose phosphorylation promotes FAK association with Src,Citation32 and subsequent phosphorylation of additional sites on FAK. Y397 phosphorylation is required for focal adhesion dynamics.Citation33,Citation34 Thus, the elevated FAK phosphorylation and increased cell spreading suggest that loss of CAP1 may also increase focal adhesion turnover.Citation34 Moreover, adhesion signaling can activate Rho family GTPases, including Cdc42 and Rac,Citation32,Citation35,Citation36 which then stimulate the formation of filopodia and lamellipodia. This probably underlies the phenotype of increased peripheral protrusions observed in the CAP1-knockdown HeLa cells.Citation5
The mechanism by which CAP1 depletion enhanced cell adhesion probably requires activation of integrins. Integrin activation is regulated bi-directionally; not only can information flow from extracellular stimuli (outside-in signaling), but intracellular stimuli can also cause extracellular changes in adhesion (inside-out signaling).Citation36 One possibility is that the enhanced formation of actin filaments in the CAP1-knockdown cells triggers integrin activation. To unravel possible mechanisms that link CAP1 to cell adhesion signaling, we searched for complexes between CAP1 and adhesion proteins and found that CAP1 forms complexes with two adhesion molecules FAK and Talin.Citation5 The direct partner of CAP1 is likely to be Talin because CAP1 interacts with Talin in yeast two-hybrid screens.Citation37 Talin is a cytoskeletal protein that is also a component of focal adhesion complexes,Citation38 providing a physical link between integrins and the cytoskeleton by binding to the cytoplasmic portion of integrin. During cell adhesion, it induces conformational changes in integrin extracellular domains that result in increased affinity for ligands.Citation39,Citation40 Talin binding is the final common step in integrin activation,Citation41 and abrogation of Talin expression results in impaired integrin activation.Citation36 We speculate that Talin may be the molecular link between CAP1 and the cell adhesion signaling through an “inside-out signaling” mechanism. Accordingly, we propose a model how CAP1 depletion activates integrin, as shown in . In this model, CAP1 binds and sequesters Talin molecules in normal cells. Depletion of CAP1 releases Talin, enabling more Talin molecules to bind to the cytoplasmic portion of integrin, and thus, cause integrin activation through an inside-out signaling.Citation36 Activated integrin then activates FAK, which promotes the assembly of focal adhesion platforms to recruit other signaling and structural molecules such as Src, actinin, paxilin, etc.Citation32 The activated FAK also forms complexes with Src to activate Rho family GTPases to promote cell migration.Citation32 Thus, loss of CAP1 can activate inside-out integrin signaling as well as release actin monomers to assemble into filaments.
Figure 1. A schematic model showing how depletion of CAP1 leads to Talin-mediated integrin activation through the “inside-out signaling.” (A) In normal cells, CAP1 binds and sequesters Talin and integrins are minimally activated. For simplicity, some of the detailed aspects are not shown, they include: (1) only monomeric CAP1 molecules are shown, although CAP1 has been known to exist in oligomeric form in cells when bound to actin; (2) CAP1, Talin, and FAK may also present in a large complex; (3) binding of G-actin and cofilin with CAP1. (B) In CAP1-knockdown cells, the depletion of CAP1 (shown in the cartoon as CAP1 molecules attached to scissors) releases Talin, enabling more Talin molecules to bind to the cytoplasmic tail of integrin, and thus, causing changes in the confirmation of the extracellular portion of integrin (integrin activation). FAK is subsequently activated and binds to Talin, which set up the platform for recruiting additional signaling and structural proteins to form focal adhesions. It is also possible that a subset of the Talin molecules bound to integrin may also be in complex with CAP1 (not shown). (C) The activated integrin and FAK in CAP1-knockdown cells promote formation of focal adhesions, which is connected to the actin filament network. Note that only some of the important proteins found in the focal adhesions are shown. Meanwhile, active FAK forms complex with Src, which activates downstream Rho family GTPases, including Cdc42 and Rac, to stimulate cell migration.
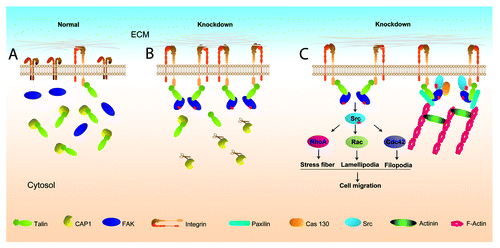
Mammalian CAP1 has been solidly established as a key regulator of actin filament dynamics. However, depletion of CAP1 can have cell type-dependent effects on cell motility, which, in the case of HeLa cells, occurs through activation of cell adhesion signaling. We recently observed that CAP1 knockdown in certain breast cancer cells also caused activation of FAK and increased peripheral protrusions, so the observation extends beyond HeLa cells (HZ and GLZ, unpublished data). Additional work is required to understand why CAP1 depletion causes activation of adhesion signaling in some cells, while it is apparently not the case in other cell types. Such studies may be relevant to cancer cell invasion and metastasis.
Disclosure of Potential Conflicts of Interest
No potential conflicts of interest were disclosed.
Acknowledgments
This work was supported by grants to GLZ from the Arkansas Biosciences Institute, the Arkansas Breast Cancer Research Program, and the University of Arkansas for Medical Sciences Translational Research Institute (CSTA Grant Award #UL1TR000039), and the Institutional Development Award (IDeA) from the National Institute of General Medical Sciences of the National Institutes of Health under grant #P20GM103429-12. JF is supported by GM048241 from National Institutes of Health.
References
- Wegner A. Treadmilling of actin at physiological salt concentrations. An analysis of the critical concentrations of actin filaments. J Mol Biol 1982; 161:607 - 15; http://dx.doi.org/10.1016/0022-2836(82)90411-9; PMID: 7154092
- Mockrin SC, Korn ED. Acanthamoeba profilin interacts with G-actin to increase the rate of exchange of actin-bound adenosine 5′-triphosphate. Biochemistry 1980; 19:5359 - 62; http://dx.doi.org/10.1021/bi00564a033; PMID: 6893804
- Bray D, Thomas C. Unpolymerized actin in fibroblasts and brain. J Mol Biol 1976; 105:527 - 44; http://dx.doi.org/10.1016/0022-2836(76)90233-3; PMID: 987247
- Heacock CS, Bamburg JR. The quantitation of G- and F-actin in cultured cells. Anal Biochem 1983; 135:22 - 36; http://dx.doi.org/10.1016/0003-2697(83)90725-X; PMID: 6670742
- Zhang H, Ghai P, Wu H, Wang C, Field J, Zhou GL. Mammalian adenylyl cyclase-associated protein 1 (CAP1) regulates cofilin function, the actin cytoskeleton, and cell adhesion. J Biol Chem 2013; 288:20966 - 77; http://dx.doi.org/10.1074/jbc.M113.484535; PMID: 23737525
- Carlsson L, Nyström LE, Sundkvist I, Markey F, Lindberg U. Actin polymerizability is influenced by profilin, a low molecular weight protein in non-muscle cells. J Mol Biol 1977; 115:465 - 83; http://dx.doi.org/10.1016/0022-2836(77)90166-8; PMID: 563468
- Ono S. The role of cyclase-associated protein in regulating actin filament dynamics - more than a monomer-sequestration factor. J Cell Sci 2013; 126:3249 - 58; http://dx.doi.org/10.1242/jcs.128231; PMID: 23908377
- Field J, Vojtek A, Ballester R, Bolger G, Colicelli J, Ferguson K, Gerst J, Kataoka T, Michaeli T, Powers S, et al. Cloning and characterization of CAP, the S. cerevisiae gene encoding the 70 kd adenylyl cyclase-associated protein. Cell 1990; 61:319 - 27; http://dx.doi.org/10.1016/0092-8674(90)90812-S; PMID: 2184942
- Fedor-Chaiken M, Deschenes RJ, Broach JR. SRV2, a gene required for RAS activation of adenylate cyclase in yeast. Cell 1990; 61:329 - 40; http://dx.doi.org/10.1016/0092-8674(90)90813-T; PMID: 2158860
- Vojtek A, Haarer B, Field J, Gerst J, Pollard TD, Brown S, Wigler M. Evidence for a functional link between profilin and CAP in the yeast S. cerevisiae. Cell 1991; 66:497 - 505; http://dx.doi.org/10.1016/0092-8674(81)90013-1; PMID: 1868547
- Shibata R, Mori T, Du W, Chuma M, Gotoh M, Shimazu M, Ueda M, Hirohashi S, Sakamoto M. Overexpression of cyclase-associated protein 2 in multistage hepatocarcinogenesis. Clin Cancer Res 2006; 12:5363 - 8; http://dx.doi.org/10.1158/1078-0432.CCR-05-2245; PMID: 17000669
- Yamazaki K, Takamura M, Masugi Y, Mori T, Du W, Hibi T, Hiraoka N, Ohta T, Ohki M, Hirohashi S, et al. Adenylate cyclase-associated protein 1 overexpressed in pancreatic cancers is involved in cancer cell motility. Lab Invest 2009; 89:425 - 32; http://dx.doi.org/10.1038/labinvest.2009.5; PMID: 19188911
- Tan M, Song X, Zhang G, Peng A, Li X, Li M, Liu Y, Wang C. Overexpression of adenylate cyclase-associated protein 1 is associated with metastasis of lung cancer. Oncol Rep 2013; 30:1639 - 44; PMID: 23842884
- Li M, Yang X, Shi H, Ren H, Chen X, Zhang S, Zhu J, Zhang J. Downregulated expression of the cyclase-associated protein 1 (CAP1) reduces migration in esophageal squamous cell carcinoma. Jpn J Clin Oncol 2013; 43:856 - 64; http://dx.doi.org/10.1093/jjco/hyt093; PMID: 23904342
- Hubberstey AV, Mottillo EP. Cyclase-associated proteins: CAPacity for linking signal transduction and actin polymerization. FASEB J 2002; 16:487 - 99; http://dx.doi.org/10.1096/fj.01-0659rev; PMID: 11919151
- Gerst JE, Ferguson K, Vojtek A, Wigler M, Field J. CAP is a bifunctional component of the Saccharomyces cerevisiae adenylyl cyclase complex. Mol Cell Biol 1991; 11:1248 - 57; PMID: 1996090
- Gieselmann R, Mann K. ASP-56, a new actin sequestering protein from pig platelets with homology to CAP, an adenylate cyclase-associated protein from yeast. FEBS Lett 1992; 298:149 - 53; http://dx.doi.org/10.1016/0014-5793(92)80043-G; PMID: 1544438
- Freeman NL, Chen Z, Horenstein J, Weber A, Field J. An actin monomer binding activity localizes to the carboxyl-terminal half of the Saccharomyces cerevisiae cyclase-associated protein. J Biol Chem 1995; 270:5680 - 5; http://dx.doi.org/10.1074/jbc.270.10.5680; PMID: 7890691
- Moriyama K, Yahara I. Human CAP1 is a key factor in the recycling of cofilin and actin for rapid actin turnover. J Cell Sci 2002; 115:1591 - 601; PMID: 11950878
- Makkonen M, Bertling E, Chebotareva NA, Baum J, Lappalainen P. Mammalian and malaria parasite cyclase-associated proteins catalyze nucleotide exchange on G-actin through a conserved mechanism. J Biol Chem 2013; 288:984 - 94; http://dx.doi.org/10.1074/jbc.M112.435719; PMID: 23184938
- Mattila PK, Quintero-Monzon O, Kugler J, Moseley JB, Almo SC, Lappalainen P, Goode BL. A high-affinity interaction with ADP-actin monomers underlies the mechanism and in vivo function of Srv2/cyclase-associated protein. Mol Biol Cell 2004; 15:5158 - 71; http://dx.doi.org/10.1091/mbc.E04-06-0444; PMID: 15356265
- Chaudhry F, Little K, Talarico L, Quintero-Monzon O, Goode BL. A central role for the WH2 domain of Srv2/CAP in recharging actin monomers to drive actin turnover in vitro and in vivo. Cytoskeleton (Hoboken) 2010; 67:120 - 33; PMID: 20169536
- Nishida E. Opposite effects of cofilin and profilin from porcine brain on rate of exchange of actin-bound adenosine 5′-triphosphate. Biochemistry 1985; 24:1160 - 4; http://dx.doi.org/10.1021/bi00326a015; PMID: 4096896
- Chaudhry F, Breitsprecher D, Little K, Sharov G, Sokolova O, Goode BL. Srv2/cyclase-associated protein forms hexameric shurikens that directly catalyze actin filament severing by cofilin. Mol Biol Cell 2013; 24:31 - 41; http://dx.doi.org/10.1091/mbc.E12-08-0589; PMID: 23135996
- Bertling E, Hotulainen P, Mattila PK, Matilainen T, Salminen M, Lappalainen P. Cyclase-associated protein 1 (CAP1) promotes cofilin-induced actin dynamics in mammalian nonmuscle cells. Mol Biol Cell 2004; 15:2324 - 34; http://dx.doi.org/10.1091/mbc.E04-01-0048; PMID: 15004221
- Noegel AA, Blau-Wasser R, Sultana H, Müller R, Israel L, Schleicher M, Patel H, Weijer CJ. The cyclase-associated protein CAP as regulator of cell polarity and cAMP signaling in Dictyostelium. Mol Biol Cell 2004; 15:934 - 45; http://dx.doi.org/10.1091/mbc.E03-05-0269; PMID: 14595119
- Vojtek AB, Cooper JA. Identification and characterization of a cDNA encoding mouse CAP: a homolog of the yeast adenylyl cyclase associated protein. J Cell Sci 1993; 105:777 - 85; PMID: 7691848
- Noegel AA, Rivero F, Albrecht R, Janssen KP, Köhler J, Parent CA, Schleicher M. Assessing the role of the ASP56/CAP homologue of Dictyostelium discoideum and the requirements for subcellular localization. J Cell Sci 1999; 112:3195 - 203; PMID: 10504325
- Tojkander S, Gateva G, Lappalainen P. Actin stress fibers--assembly, dynamics and biological roles. J Cell Sci 2012; 125:1855 - 64; http://dx.doi.org/10.1242/jcs.098087; PMID: 22544950
- Huttenlocher A, Horwitz AR. Integrins in cell migration. Cold Spring Harb Perspect Biol 2011; 3:a005074; http://dx.doi.org/10.1101/cshperspect.a005074; PMID: 21885598
- Michael KE, Dumbauld DW, Burns KL, Hanks SK, García AJ. Focal adhesion kinase modulates cell adhesion strengthening via integrin activation. Mol Biol Cell 2009; 20:2508 - 19; http://dx.doi.org/10.1091/mbc.E08-01-0076; PMID: 19297531
- Mitra SK, Hanson DA, Schlaepfer DD. Focal adhesion kinase: in command and control of cell motility. Nat Rev Mol Cell Biol 2005; 6:56 - 68; http://dx.doi.org/10.1038/nrm1549; PMID: 15688067
- Hamadi A, Bouali M, Dontenwill M, Stoeckel H, Takeda K, Rondé P. Regulation of focal adhesion dynamics and disassembly by phosphorylation of FAK at tyrosine 397. J Cell Sci 2005; 118:4415 - 25; http://dx.doi.org/10.1242/jcs.02565; PMID: 16159962
- Ren XD, Kiosses WB, Sieg DJ, Otey CA, Schlaepfer DD, Schwartz MA. Focal adhesion kinase suppresses Rho activity to promote focal adhesion turnover. J Cell Sci 2000; 113:3673 - 8; PMID: 11017882
- Price LS, Leng J, Schwartz MA, Bokoch GM. Activation of Rac and Cdc42 by integrins mediates cell spreading. Mol Biol Cell 1998; 9:1863 - 71; http://dx.doi.org/10.1091/mbc.9.7.1863; PMID: 9658176
- Legate KR, Wickström SA, Fässler R. Genetic and cell biological analysis of integrin outside-in signaling. Genes Dev 2009; 23:397 - 418; http://dx.doi.org/10.1101/gad.1758709; PMID: 19240129
- O'Neill KE. An analysis and functional characterization of human and yeast cyclase associated protein. Columbia University Ph.D. Thesis 1996; http://en.scientificcommons.org/repository/academic_commons_by_columbia_university_libraries.
- Wiesner S, Legate KR, Fässler R. Integrin-actin interactions. Cell Mol Life Sci 2005; 62:1081 - 99; http://dx.doi.org/10.1007/s00018-005-4522-8; PMID: 15761669
- Calderwood DA, Ginsberg MH. Talin forges the links between integrins and actin. Nat Cell Biol 2003; 5:694 - 7; http://dx.doi.org/10.1038/ncb0803-694; PMID: 12894175
- Calderwood DA. Integrin activation. J Cell Sci 2004; 117:657 - 66; http://dx.doi.org/10.1242/jcs.01014; PMID: 14754902
- Tadokoro S, Shattil SJ, Eto K, Tai V, Liddington RC, de Pereda JM, Ginsberg MH, Calderwood DA. Talin binding to integrin beta tails: a final common step in integrin activation. Science 2003; 302:103 - 6; http://dx.doi.org/10.1126/science.1086652; PMID: 14526080