Abstract
Amyloid precursor protein (APP), commonly associated with Alzheimer disease, is upregulated and distributes evenly along the injured axons, and therefore, also known as a marker of demyelinating axonal injury and axonal degeneration. However, the physiological distribution and function of APP along myelinated axons was unknown. We report that APP aggregates at nodes of Ranvier (NOR) in the myelinated central nervous system (CNS) axons but not in the peripheral nervous system (PNS). At CNS NORs, APP expression co-localizes with tenascin-R and is flanked by juxtaparanodal potassium channel expression demonstrating that APP localized to NOR. In APP-knockout (KO) mice, nodal length is significantly increased, while sodium channels are still clustered at NORs. Moreover, APP KO and APP-overexpressing transgenic (APP TG) mice exhibited a decreased and an increased thickness of myelin in spinal cords, respectively, although the changes are limited in comparison to their littermate WT mice. The thickness of myelin in APP KO sciatic nerve also increased in comparison to that in WT mice. Our observations indicate that APP acts as a novel component at CNS NORs, modulating nodal formation and has minor effects in promoting myelination.
Introduction
Amyloid precursor protein (APP) is best known for its involvement in the pathogenesis of Alzheimer disease (AD). Increasing evidence suggests that damage to myelin may be an important component of AD.Citation1-3 It has been suggested the measures of white matter tract integrity may be a viable neuroimaging biomarkers of the earliest stages of Alzheimer disease.Citation4 In a mouse transgenic model of Alzheimer disease expressing mutant APP and presenilin-1, extensive myelin pathology and disruption of myelinated fibers was observed in association with amyloid plaques.Citation5 Moreover, APP is upregulated and distributes evenly along the injured axons, and therefore, also known as a marker of demyelinating axonal injury and axonal degeneration. APP expression is upregulated in axons on injury and has long been utilized as a marker for axonal degeneration.Citation6,7 Demyelinating injury, such as in multiple sclerosis, is also associated with increased axonal APP expression.Citation8,9 However, the exact distribution and function of APP along myelinated axons has yet to be elucidated. An understanding of how APP is associated with myelinated axons is important, as myelination is a vital biological process disrupted in a wide variety of congenital and acquired neurological diseases.
Myelinated axon is characterized by their segmental structures: axonal initial segment (AIS), node of Ranvier (NOR), paranode, juxtaparanode, and internode.Citation10 The establishment and maintenance of the elaborate architecture of axonal domains and the congregation of specific ion channels and recognition molecules, such as Nav1.6 sodium channels, F3/contactin, tenascin-R, and Oligodendrocyte myelin glycoprotein (OMgp), at NORs are critical to ensuring rapid saltatory conduction of action potentials along myelinated fibers.Citation11 In the present study, we have shown that APP localizes at NORs in the central nervous system (CNS) and modulates nodal formation.
Results
APP clusters at NORs in the CNS, but not PNS
To investigate APP distribution along myelinated axons in the CNS, the longitudinal sections of rat spinal cord were stained for APP and Kvβ2 (Potassium channel β2 subunit; a marker to label juxtaparanodeCitation12) () or Tenascin-R (TnR; a marker to label NORCitation13) () by immunofluorescence (IF). APP immunoreactivity exhibited a clustering pattern, was flanked by juxtaparanodal Kvβ2 (), and colocalized with Tenascin-R (). These results indicate that APP localizes at NORs in myelinated axons of spinal cords. Similar distribution of APP at NORs was also found in optic nerve and cerebellum (). APP is also expressed in the peripheral nervous system (PNS).Citation14 However, in contrast to APP's nodal location in the CNS, APP immunoreactivity was evenly distributed along myelinated fibers in the adult rat sciatic nerve. This was shown by double immunofluorescence labeling with APP and juxtaparanodal potassium channels Kv1.2 (). The specificity of APP antibody was tested previously and confirmed by lack of immunoreactivity in APP KO mice.Citation15-18 These observations demonstrate that axonal APP is a novel component of the NORs and its clustering occurs specifically in the CNS, but not in the PNS.
Figure 1. APP localizes at nodes of Ranvier in the CNS, but not in the PNS. (A and B) The longitudinal sections of spinal cord of 2-mo-old rat were stained for APP (A and B) and Kvβ2 (A) or tenascin-R (TnR; B). The Z-stack confocal images were showed in the right panels. Scale bars: 10 μm. (C) The longitudinal sections of optic nerves and cerebellum of 2-mo-old rat were stained for APP and tenascin-R (TnR). Scale bar: 20 μm. (D) The teased sciatic nerve were stained for APP and neurofilament200 (NF200) or Kv1.2. Scale bars: 10 μm.
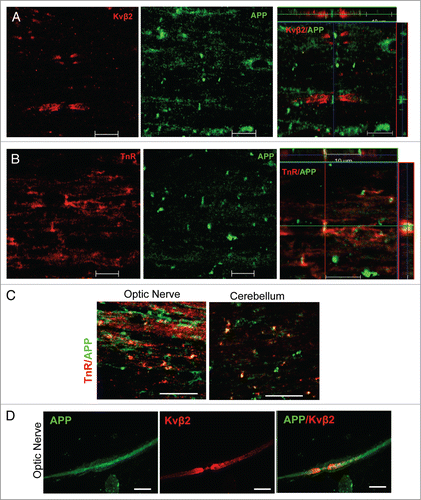
Myelination is a dynamic process mediated by bidirectional axoglial interactions that are involved in the clustering of axonal molecules, such as sodium channels and F3/contactin, at the NORs.Citation19 Next, we studied the aggregation of APP in correlation with sodium and potassium channel clustering during nodal maturation. At P6, before sodium channels start clustering at the NORs, APP was diffusely distributed along axons (). APP appeared to start clustering in the NORs around P10 () and was prevalent after P15 when the nodal structure has been formed (). These observations indicate that APP clustering lags behind sodium channel clustering in the CNS. The intensity of APP nodal clustering was quantified in relation to Kv1.2 clustering during development. The ratio of APP clustering to Kv1.2 clustering, reflecting the developmental progress of APP clustering in the population of axons, was around 50% at P10, 60% at P15 and P21, and 90% at 2 mo (). These results support the notion that nodal accumulation of APP requires the integrity of distinct axonal domains, as is the case for other compartmentalized axonal proteins.Citation43
Figure 2. Location of APP in the spinal cord during development. (A) The longitudinal sections of spinal cord of rats at postnatal day 6 (P6), P10, P15, and P21 were stained for APP and sodium channel (Pan; P6) or Kv1.2 (Kch; P10, 15, and P21). Scale bars: 10 μm. (B) The numbers of APP+ nodes were quantified and expressed as the percentage of APP+ nodes and Kch+-defined gaps. Data are presented as mean ± SEM.
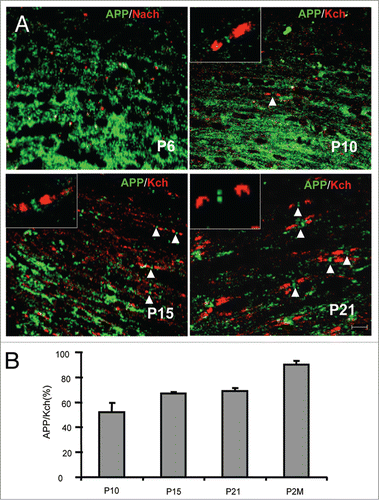
APP deficiency increases the length of NORs
Structural maturation of NORs is essential for saltatory conduction, which is completed during the late phase of myelination.Citation21-23 To define whether APP may play a regulatory role in nodal formation, we analyzed nodal structures in the spinal cord of APP KO as well as their WT mice. As reflected by double-staining using antibodies against Nav1.6 and Caspr (Contatin associated protein; a paranode marker), nodal length increased in axons of the spinal cord of APP KO vs. WT mice (). Meanwhile, neither Nav1.6 () nor Tenascin-R (not shown) clustering showed any discernible alteration in the APP KO vs. their WT littermates. Quantification showed that nodal length was significantly increased in the APP KO vs. WT mice ().
Figure 3. Increased nodal length in APP KO spinal cord. (A) The longitudinal sections of spinal cord from APP- KO and WT mice at 2 mo old were stained for Caspr and sodium chanels (Nach). Scale bars: 10 μm. Scale bars in higher magnification: 5 μm. (B) Nodal lengths in APP KO vs. WT mice were analyzed. The nodal length in APP WT spinal cord was normalized to 1.0. The relative nodal length in APP KO spinal cord was quantified and compared. Data are presented as mean ± SEM. *P < 0.05. (C and D) EM analyses of longitudinal spinal cord sections of APP KO vs. WT mice (all aged 3 mo). The red dotted lines and bars mark the spans of nodal gaps. Scale bars: 500 nm. The nodal length in APP WT spinal cord was normalized to 100. The relative nodal length in APP KO spinal cord was quantified and compared. Data are presented as mean ± SEM. *P < 0.05.
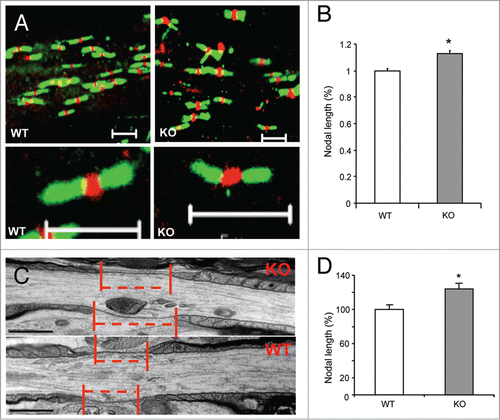
Moreover, we analyzed nodal structures in the spinal cord of APP KO mice as well as their WT mice using electromicroscopical (EM) approach. Ultra-structural observations revealed that the lateral oligodendrocytic loops remained compacted in APP KO mice, as they are in WT mice (). Ultra-structural quantification showed that the nodal length was significantly reduced in the APP KO mice vs. WT littermates (). Together, these observations suggest that APP plays a role in modulating the length of NORs.
APP has minor effect on myelination
Nodal molecules, which usually interact with glial molecules, taken an instance, Omgp, modulate myelination through axon-glia interaction.Citation24 Considering that APP localizes at NORs, we therefore investigated whether APP might modulate myelination using electromicroscope (EM) analysis. The degree of myelination in spinal cord from 3-mo-old APP WT and KO mice as well as transgenic (APP TG) mice which overexpress human APP driven by the prion promoterCitation25 was analyzed. EM showed no axonal swelling in KO and transgenic mice at this age (, , and ). Interestingly, the g ratio, the numeric ratio between the diameter of the axon and the outer diameter of the myelinated fiber,Citation26 which affects the thickness of myelin (Smaller g ratio indicates thicker myelin), was increased in the spinal cord of APP KO mice (0.829 ± 0.002) in comparison to WT mice (0.817 ± 0.002) (). Although statistic significances in the g ratio were observed, the g ratio in APP KO spinal cord showed only 2% increase compared with that in APP WT mice. To confirm these results, we analyzed the spinal cord from 3-mo-old APP TG mice. As predicted, the g ratio was decreased in APP TG spinal cord compared with WT spinal cord (). The g ratio in APP TG spinal cord showed a 1.3% decrease compared with that in the littermate WT mice. However, the axonal distribution showed no differences in between the spinal cord of APP KO, APP TG, and their littermates WT mice (Fig. S1A and B). Thus, APP only has a minor effect on promoting myelination in the spinal cord. Moreover, the average g ratio in APP KO sciatic nerve increased (), and showed a 5% increase in comparison to that in APP WT sciatic nerve, while the axonal distribution in APP KO sciatic nerve showed no differences from WT sciatic nerve (Fig. S1C). These results indicate that APP promotes myelination in sciatic nerve.
Figure 4. The effects of APP on myelination. (A) EM studies of cross-sections revealed the ultrastructure of myelin sheaths in spinal cords (SC) of WT vs. APP KO mice (all aged 3 mo). Scale bars: 2 μm. (B) The g ratio in APP WT and KO spinal cord was analyzed. Data are presented as mean ± SEM. **P < 0.01. (C) The g ratio in APP WT spinal cord was normalized as 100%. The relative g ratio in APP KO spinal cord was shown. (D) EM studies of cross-sections revealed the ultrastructure of myelin sheaths in the spinal cord (SC) of WT littermates vs. APP TG mice (all aged 3 mo). Scale bars: 2 μm. (E) The g ratio in APP WT and TG spinal cord was analyzed. Data are presented as mean ± SEM. **P < 0.01. (F) The g ratio in APP WT spinal cord was normalized as 100%. The relative g ratio in APP TG spinal cord was shown. (G) EM studies of cross-sections revealed the ultrastructure of myelin sheaths in sciatic nerves (SN) of APP WT and KO mice (all aged 3 mo). Scale bars: 5 μm. (H) The g ratio in the sciatic nerve of APP WT and KO at 3 mo old was analyzed. Data are presented as mean ± SEM. **P < 0.01. (I) The g ratio in APP WT sciatic nerve was normalized as 100%. The relative g ratio in APP KO sciatic nerve was shown.
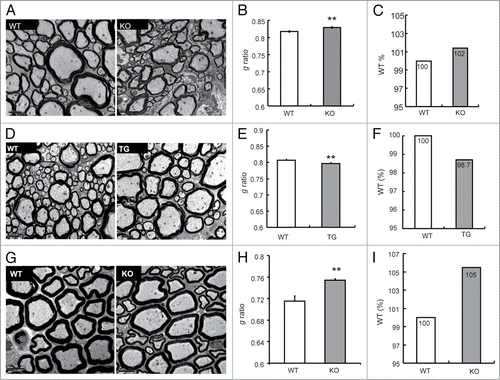
Discussion
We have found that APP is clustered at the NORs of myelinated axons in the CNS but not in the PNS. APP, as a novel component at CNS NORs, is involved in negatively modulating nodal length in the CNS.
During myelination, various axonal and glial molecules redistribute themselves to take up defined domains through dynamic axoglial interaction.Citation10 NORs are the naked regions of the axolemma and intermittently distribute along myelinated axons, implying potential interactions between exposed axonal molecules and the extracellular partners. Over the past decades, the molecular composition corresponding to the morphological domains of the myelinated axon has been extensively studied, with a major focus on the cell adhesion molecules. For instance, F3/contactin, a member of the immunoglobulin superfamily, has been implicated in molecular communication in both nodal and paranodal sites of the CNS, via interacting with sodium channels and Caspr/paranodin, respectively. TAG-1/TAX, from the same L1 cell adhesion molecule subfamily as F3/contactin, has been suggested to be a constituent of juxtaparanodal formation.Citation27-29 The sodium channel β subunits, which associate with α subunits of voltage-gated sodium channels at the NORs, have an extracellular immunoglobulin-like loop and act as cell adhesion molecules.Citation30 APP also plays a role in cell adhesion and interacts with various cell adhesion molecules,Citation31-33 including TAG-1/TAX.Citation17 Previously, it has been noted that APP expression in axons is upregulated on neural injury, and hence, APP expression has been utilized as a marker for axonal degeneration.Citation7 Moreover, following demyelinating injury, APP expression was also increased.Citation8,9 We found that in the absence of injury, APP was largely aggregated at NORs along axons in the CNS. The diffusely distribution of APP along the injured axons may be due to the fact that the clustering of nodal proteins such as sodium channels at NORs requires the integrity of myelinated axons.Citation34,35
We further investigated the pattern of APP clustering at NORs during development. APP clustering occurred later than the clustering of both sodium and potassium channels. Consistent with this fact, APP is not required for clustering of other nodal molecules such as tenascin-R, Caspr, and sodium channels, as clustering of these molecules still occurs in APP KO mice. Thus, it is clear that APP is not an initiator for the formation of NORs. However, the nodal gap is abnormally widened in APP KO mice, indicating that APP may function in generating normal nodal architecture and in maintaining the physiological functions of NORs. Various proteins have been identified to maintain the normal architecture and functions of NORs, which includes axonal and glial molecules.Citation24 It is supposed that the axon–glial interaction play essential roles in nodule formation.Citation24 APP is expressed in both axonsCitation35 and glial cells, including astrocytesCitation36 and oligodendrocytes.Citation37 The nodal APP we have observed in this study was detected by an antibody recognizing the intracellular domain of APP.Citation15-17 Therefore, it is unlikely that the nodal APP is secreted from glial cells, like TnR.Citation13 However, how axonal APP modulates nodal length remains unclear. One possibility is that APP interacts with other nodal proteins such as ankyrin G, Neurofascin 155, and TnR, modulating the formation of NORs. APP also possibly interacts with paranodal protein, through which, anchoring the paranodal loops to the boundary of NORs. Identification of the binding partners of APP at NORs will help to address such possibilities.
Myelination is a multi-step coordinated process involving cellular interactions between axons and oligodendrocytes in the CNS and Schwann cells in the PNS. Interestingly, reduced myelination thickness, as reflected by increased g ratios, occurs in both CNS and PNS of APP KO mice, implying that APP promotes myelination in both CNS and PNS. However, the alterations of g ratio in both APP KO and APP TG spinal cords are about 2% in comparison to that in their WT littermates. The g ratio in the sciatic nerve of APP KO mice has 5% increase compared with that in WT mice. These results indicate that APP only has minor effects on myelination. Considering the different location of APP in the PNS and CNS, it is unlikely that the effects of APP on myelination in both the PNS and CNS are related to the nodal location of APP.
APP plays essential roles in Alzheimer disease as it generates amyloid-β by proteolytic cleavage.Citation38 Impaired myelinated axons have been detected in the brains of Alzheimer disease patients and been supposed as an early component of Alzheimer disease.Citation1-3,Citation39,40 Abnormalities in CNS myelination affect the molecular organization at and around nodal axons leading to disturbances in CNS functions. For example, the heterozygous proteolipid protein transgenic mice exhibited profound reduction in Nav1.6 clusters, loss of the paranodal axo-glial apparatus, and a marked increase in Nav1.2.Citation41 Dysfunction of nodes of Ranvier are now recognized as key contributors to the pathophysiology of various neurological diseases.Citation42 It requires more investigation on the functions of the nodal APP, especially how the nodal APP contributes to Alzheimer disease.
Materials and Methods
Antibodies, peptides, inhibitors, and activators
Rabbit polyclonal antibodies against CasprCitation43 and monoclonal antibodies against Tenascin-R (596, 619)Citation44 and Rabbit polyclonal antibodies against APPCitation15-17 were generously from Dr Dennis Selkoe (Harvard University; C7 and C9). Monoclonal antibodies against pan-sodium channel (Sigma), potassium channels Kv1.2 (K14/16), Kvβ2 (Upstate), and NF200 (Sigma) were obtained from the respective commercial sources
Mutant mice
The APP KOCitation45 and APP overexpressing TG miceCitation25 were as previously described. All experiments involving mice were approved by the Institutional Animal Care and Use Committee of Soochow University.
Immunofluorescence labeling
Animals were perfused sequentially with 0.1 M Ringer's solution and 4% paraformaldehyde in 0.1 M phosphate buffer, pH 7.4 (PB). Spinal cords were removed immediately. For cryosectioning, tissues were immersed, first in 15% sucrose and then in 30% sucrose in PB. Ten μm-thick sections were mounted onto gelatin or poly-l-lysine-coated glass slides. After being air-dried, slides were kept at −20 °C for later use. Sciatic nerve was teased as previously described.Citation46
For immunofluorescence staining, tissue slices were first immersed in absolute acetone at −20 °C for 20 min. Slices were then thoroughly washed in 0.1 M phosphate buffered saline (PBS, pH 7.6) containing 0.3% Triton X-100. After blocking with 10% normal goat serum (NGS) diluted in PB for 1 h, tissue slices were incubated with primary antibodies at their optimal dilutions, either overnight or for 2 h at room temperature. Cy2- or Cy3-conjugated secondary antibodies (Amersham) corresponding to the primary antibodies were used for further incubation at room temperature for 1 h. For double labeling, one more cycle of labeling was similarly conducted for the second primary antibodies. All labeled slices were visualized and photographed under a Leica RXA2 upright fluorescence microscope or an LSM5 Carl Zeiss confocal microscope system.
Electron microscopy
Animals were transcardially perfusion-fixed with 2% paraformaldehyde/3% glutaraldehyde in 0.1 M cacodylate buffer (pH 7.4) for electron microscopy. The dorsal part of cervical spinal cords and sciatic nerves were removed and post-fixed in the same fixative solution for 2 h. After being finely trimmed, the samples were immersion-washed overnight. Tissue blocks were further post-fixed in 1% osmium tetroxide (OsO4) containing 1.5% potassium ferrocyanide for 2 h, and thereafter, subjected to dehydration in an ascending series of alcohol and acetone. After undergoing gradual infiltration with Araldite 502 (EMS), the blocks were embedded and polymerized overnight at 60 °C. Ultrathin sections (∼90 nm in thickness) were placed on 200-mesh copper grids and counterstained with uranyl acetate and lead citrate. All samples were examined and photographed under a Jeol 1220 electron microscope.
Quantification
The numbers of APP+ NORs, the nodal length was counted from more than 3 different sections each mouse. More than 3 mice were analyzed each group. The length of node in the IF and EM analysis was counted using Image J software. The diameters of axons and fibers in more than 10 pictures taken under 6000× magnification from each mouse were counted using Image J software. At least 3 mice were analyzed in each group. The g ratios were calculated as axonal diameter/fiber diameter.
Data analysis
All values were expressed as mean ± SEM, where n indicated the number of mice. Student t test was performed to determine whether there were significant differences followed by Bonferroni test as post hoc analysis; *P < 0.05; **P < 0.01.
Disclosure of Potential Conflicts of Interest
No potential conflicts of interest were disclosed.
2014CAM0015R-Sup.pdf
Download PDF (100.4 KB)Acknowledgments
This work was supported by grants from The Talent Program of Yunnan Province, China, and The Professorial Fellowship of Monash University, Australia, to ZC Xiao from the National Basic Research Program of China (973 program) (2013CB945602), A Project Funded by the Priority Academic Program Development of Jiangsu Higher Education Institutions, Suzhou Science and Technology Development Program (SZS201205), and the National Natural Science Foundation of China (31171313 and 81271424), to QH Ma from MERLION, a Singapore-France joint scientific program, to both ZC Xiao and D Bagnard; and from the National Medical Research Council of Singapore (NMRC/1059/2006) to both GS Dawe and ZC Xiao. We are grateful to Prof Dennis Selkoe (Harvard University) for generously providing APP antibodies and to Dr Hasan Mohajeri (University of Zurich) for preparing the samples of APP transgenic mice.
Supplemental Material
Supplemental material may be found here: www.landesbioscience.com/journals/celladhesion/article/28802.
References
- Noble M. The possible role of myelin destruction as a precipitating event in Alzheimer's disease. Neurobiol Aging 2004; 25:25-31; PMID:14675726; http://dx.doi.org/10.1016/j.neurobiolaging.2003.07.001
- Bartzokis G. Age-related myelin breakdown: a developmental model of cognitive decline and Alzheimer's disease. Neurobiol Aging 2004; 25:5-18, author reply 49-62; PMID:14675724; http://dx.doi.org/10.1016/j.neurobiolaging.2003.03.001
- Bartzokis G. Alzheimer's disease as homeostatic responses to age-related myelin breakdown. Neurobiol Aging 2011; 32:1341-71; PMID:19775776; http://dx.doi.org/10.1016/j.neurobiolaging.2009.08.007
- Fieremans E, Benitez A, Jensen JH, Falangola MF, Tabesh A, Deardorff RL, Spampinato MV, Babb JS, Novikov DS, Ferris SH, et al. Novel white matter tract integrity metrics sensitive to Alzheimer disease progression. AJNR Am J Neuroradiol 2013; 34:2105-12; PMID:23764722; http://dx.doi.org/10.3174/ajnr.A3553
- Schmued LC, Raymick J, Paule MG, Dumas M, Sarkar S. Characterization of myelin pathology in the hippocampal complex of a transgenic mouse model of Alzheimer's disease. Curr Alzheimer Res 2013; 10:30-7; PMID:23157338
- Selkoe DJ. American College of Physicians; American Physiological Society. Alzheimer disease: mechanistic understanding predicts novel therapies. Ann Intern Med 2004; 140:627-38; PMID:15096334; http://dx.doi.org/10.7326/0003-4819-140-8-200404200-00010
- Coleman M. Axon degeneration mechanisms: commonality amid diversity. Nat Rev Neurosci 2005; 6:889-98; PMID:16224497; http://dx.doi.org/10.1038/nrn1788
- Dutta R, Chang A, Doud MK, Kidd GJ, Ribaudo MV, Young EA, Fox RJ, Staugaitis SM, Trapp BD. Demyelination causes synaptic alterations in hippocampi from multiple sclerosis patients. Ann Neurol 2011; 69:445-54; PMID:21446020; http://dx.doi.org/10.1002/ana.22337
- Ferguson B, Matyszak MK, Esiri MM, Perry VH. Axonal damage in acute multiple sclerosis lesions. Brain 1997; 120:393-9; PMID:9126051; http://dx.doi.org/10.1093/brain/120.3.393
- Salzer JL. Polarized domains of myelinated axons. Neuron 2003; 40:297-318; PMID:14556710; http://dx.doi.org/10.1016/S0896-6273(03)00628-7
- Ma QH, Yang WL, Nie DY, Dawe GS, Xiao ZC. Physiological roles of neurite outgrowth inhibitors in myelinated axons of the central nervous system–implications for the therapeutic neutralization of neurite outgrowth inhibitors. Curr Pharm Des 2007; 13:2529-37; PMID:17692021; http://dx.doi.org/10.2174/138161207781368602
- Rasband MN, Trimmer JS, Schwarz TL, Levinson SR, Ellisman MH, Schachner M, Shrager P. Potassium channel distribution, clustering, and function in remyelinating rat axons. J Neurosci 1998; 18:36-47; PMID:9412484
- Weber P, Bartsch U, Rasband MN, Czaniera R, Lang Y, Bluethmann H, Margolis RU, Levinson SR, Shrager P, Montag D, et al. Mice deficient for tenascin-R display alterations of the extracellular matrix and decreased axonal conduction velocities in the CNS. J Neurosci 1999; 19:4245-62; PMID:10341229
- Alvarez J, Moreno RD, Llanos O, Inestrosa NC, Brandan E, Colby T, Esch FS. Axonal sprouting induced in the sciatic nerve by the amyloid precursor protein (APP) and other antiproteases. Neurosci Lett. 1992; 144(1-2):130-4.
- Puglielli L, Konopka G, Pack-Chung E, Ingano LA, Berezovska O, Hyman BT, Chang TY, Tanzi RE, Kovacs DM. Acyl-coenzyme A: cholesterol acyltransferase modulates the generation of the amyloid beta-peptide. Nat Cell Biol 2001; 3:905-12; PMID:11584272; http://dx.doi.org/10.1038/ncb1001-905
- Saura CA, Chen G, Malkani S, Choi SY, Takahashi RH, Zhang D, Gouras GK, Kirkwood A, Morris RG, Shen J. Conditional inactivation of presenilin 1 prevents amyloid accumulation and temporarily rescues contextual and spatial working memory impairments in amyloid precursor protein transgenic mice. J Neurosci 2005; 25:6755-64; PMID:16033885; http://dx.doi.org/10.1523/JNEUROSCI.1247-05.2005
- Ma QH, Futagawa T, Yang WL, Jiang XD, Zeng L, Takeda Y, Xu RX, Bagnard D, Schachner M, Furley AJ, et al. A TAG1-APP signalling pathway through Fe65 negatively modulates neurogenesis. Nat Cell Biol 2008; 10:283-94; PMID:18278038; http://dx.doi.org/10.1038/ncb1690
- Young-Pearse TL, Bai J, Chang R, Zheng JB, LoTurco JJ, Selkoe DJ. A critical function for beta-amyloid precursor protein in neuronal migration revealed by in utero RNA interference. J Neurosci 2007; 27:14459-69; PMID:18160654; http://dx.doi.org/10.1523/JNEUROSCI.4701-07.2007
- Kazarinova-Noyes K, Malhotra JD, McEwen DP, Mattei LN, Berglund EO, Ranscht B, Levinson SR, Schachner M, Shrager P, Isom LL, et al. Contactin associates with Na+ channels and increases their functional expression. J Neurosci 2001; 21:7517-25; PMID:11567041
- Huber AB, Weinmann O, Brösamle C, Oertle T, Schwab ME. Patterns of Nogo mRNA and protein expression in the developing and adult rat and after CNS lesions. J Neurosci 2002; 22:3553-67; PMID:11978832
- Hodgkin AL, Huxley AF. A quantitative description of membrane current and its application to conduction and excitation in nerve. J Physiol 1952; 117:500-44; PMID:12991237
- Pedraza L, Huang JK, Colman DR. Organizing principles of the axoglial apparatus. Neuron 2001; 30:335-44; PMID:11394997; http://dx.doi.org/10.1016/S0896-6273(01)00306-3
- Hille B. Ion channels of excitable membranes, 3d ed. Scitech Book News. Portland: Book News, Inc., 2001.
- Susuki K, Rasband MN. Molecular mechanisms of node of Ranvier formation. Curr Opin Cell Biol 2008; 20:616-23; PMID:18929652; http://dx.doi.org/10.1016/j.ceb.2008.09.007
- Hsiao KK, Borchelt DR, Olson K, Johannsdottir R, Kitt C, Yunis W, Xu S, Eckman C, Younkin S, Price D, et al. Age-related CNS disorder and early death in transgenic FVB/N mice overexpressing Alzheimer amyloid precursor proteins. Neuron 1995; 15:1203-18; PMID:7576662; http://dx.doi.org/10.1016/0896-6273(95)90107-8
- Michailov GV, Sereda MW, Brinkmann BG, Fischer TM, Haug B, Birchmeier C, Role L, Lai C, Schwab MH, Nave KA. Axonal neuregulin-1 regulates myelin sheath thickness. Science 2004; 304:700-3; PMID:15044753; http://dx.doi.org/10.1126/science.1095862
- Traka M, Dupree JL, Popko B, Karagogeos D. The neuronal adhesion protein TAG-1 is expressed by Schwann cells and oligodendrocytes and is localized to the juxtaparanodal region of myelinated fibers. J Neurosci 2002; 22:3016-24; PMID:11943804
- Poliak S, Salomon D, Elhanany H, Sabanay H, Kiernan B, Pevny L, Stewart CL, Xu X, Chiu SY, Shrager P, et al. Juxtaparanodal clustering of Shaker-like K+ channels in myelinated axons depends on Caspr2 and TAG-1. J Cell Biol 2003; 162:1149-60; PMID:12963709; http://dx.doi.org/10.1083/jcb.200305018
- Poliak S, Peles E. The local differentiation of myelinated axons at nodes of Ranvier. Nat Rev Neurosci 2003; 4:968-80; PMID:14682359; http://dx.doi.org/10.1038/nrn1253
- McEwen DP, Isom LL. Heterophilic interactions of sodium channel beta1 subunits with axonal and glial cell adhesion molecules. J Biol Chem 2004; 279:52744-52; PMID:15466474; http://dx.doi.org/10.1074/jbc.M405990200
- Breen KC, Bruce M, Anderton BH. Beta amyloid precursor protein mediates neuronal cell-cell and cell-surface adhesion. J Neurosci Res 1991; 28:90-100; PMID:1645774; http://dx.doi.org/10.1002/jnr.490280109
- Yamazaki T, Koo EH, Selkoe DJ. Cell surface amyloid beta-protein precursor colocalizes with beta 1 integrins at substrate contact sites in neural cells. J Neurosci 1997; 17:1004-10; PMID:8994055
- Annaert WG, Esselens C, Baert V, Boeve C, Snellings G, Cupers P, Craessaerts K, De Strooper B. Interaction with telencephalin and the amyloid precursor protein predicts a ring structure for presenilins. Neuron 2001; 32:579-89; PMID:11719200; http://dx.doi.org/10.1016/S0896-6273(01)00512-8
- Craner MJ, Newcombe J, Black JA, Hartle C, Cuzner ML, Waxman SG. Molecular changes in neurons in multiple sclerosis: altered axonal expression of Nav1.2 and Nav1.6 sodium channels and Na+/Ca2 +exchanger. Proc Natl Acad Sci U S A 2004; 101:8168-73; PMID:15148385; http://dx.doi.org/10.1073/pnas.0402765101
- Craner MJ, Hains BC, Lo AC, Black JA, Waxman SG. Co-localization of sodium channel Nav1.6 and the sodium-calcium exchanger at sites of axonal injury in the spinal cord in EAE. Brain 2004; 127:294-303; PMID:14662515; http://dx.doi.org/10.1093/brain/awh032
- Siman R, Card JP, Nelson RB, Davis LG. Expression of beta-amyloid precursor protein in reactive astrocytes following neuronal damage. Neuron 1989; 3:275-85; PMID:2518369; http://dx.doi.org/10.1016/0896-6273(89)90252-3
- Skaper SD, Evans NA, Evans NA, Rosin C, Facci L, Richardson JC. Oligodendrocytes are a novel source of amyloid peptide generation. Neurochem Res 2009; 34:2243-50; PMID:19557514; http://dx.doi.org/10.1007/s11064-009-0022-9
- De Strooper B, Annaert W. Proteolytic processing and cell biological functions of the amyloid precursor protein. J Cell Sci 2000; 113:1857-70; PMID:10806097
- Desai MK, Mastrangelo MA, Ryan DA, Sudol KL, Narrow WC, Bowers WJ. Early oligodendrocyte/myelin pathology in Alzheimer's disease mice constitutes a novel therapeutic target. Am J Pathol 2010; 177:1422-35; PMID:20696774; http://dx.doi.org/10.2353/ajpath.2010.100087
- Connor JR. Myelin breakdown in Alzheimer's disease: a commentary. Neurobiol Aging 2004; 25:45-7; PMID:14675730; http://dx.doi.org/10.1016/j.neurobiolaging.2003.06.004
- Rasband MN, Kagawa T, Park EW, Ikenaka K, Trimmer JS. Dysregulation of axonal sodium channel isoforms after adult-onset chronic demyelination. J Neurosci Res 2003; 73:465-70; PMID:12898531; http://dx.doi.org/10.1002/jnr.10675
- Susuki K. Node of Ranvier disruption as a cause of neurological diseases. ASN Neuro 2013; 5:209-19; PMID:23834220; http://dx.doi.org/10.1042/AN20130025
- Nie DY, Zhou ZH, Ang BT, Teng FY, Xu G, Xiang T, Wang CY, Zeng L, Takeda Y, Xu TL, et al. Nogo-A at CNS paranodes is a ligand of Caspr: possible regulation of K(+) channel localization. EMBO J 2003; 22:5666-78; PMID:14592966; http://dx.doi.org/10.1093/emboj/cdg570
- Xiao ZC, Revest JM, Laeng P, Rougon G, Schachner M, Montag D. Defasciculation of neurites is mediated by tenascin-R and its neuronal receptor F3/11. J Neurosci Res 1998; 52:390-404; PMID:9589384; http://dx.doi.org/10.1002/(SICI)1097-4547(19980515)52:4≤390::AID-JNR3≥3.0.CO;2-4
- Zheng H, Jiang M, Trumbauer ME, Sirinathsinghji DJ, Hopkins R, Smith DW, Heavens RP, Dawson GR, Boyce S, Conner MW, et al. beta-Amyloid precursor protein-deficient mice show reactive gliosis and decreased locomotor activity. Cell 1995; 81:525-31; PMID:7758106; http://dx.doi.org/10.1016/0092-8674(95)90073-X
- Melendez-Vasquez CV, Rios JC, Zanazzi G, Lambert S, Bretscher A, Salzer JL. Nodes of Ranvier form in association with ezrin-radixin-moesin (ERM)-positive Schwann cell processes. Proc Natl Acad Sci U S A 2001; 98:1235-40; PMID:11158623; http://dx.doi.org/10.1073/pnas.98.3.1235