Abstract
SDF-1 is ubiquitously expressed in vertebrate tissues in a constitutive manner. It performs an essential role in cell migration and proliferation as well as participates in tissue-specific physiological processes such as neuromodulation. It is also involved in many pathological processes including: HIV infection, metastatic malignancy, chronic inflammatory disorders and benign proliferative diseases. SDF-1 is mostly regulated at the splicing, and not transcriptional level. Different splicing variants share agonist potency to their cognate receptor, CXCR4, but are characterized by distinct properties. SDF-1α is the predominant isoform found in all organs, but undergoes rapid proteolysis in blood. SDF-1β is more resistant to blood-dependent degradation, stimulates angiogenesis and is present in highly vascularized organs such as: the liver, spleen and kidneys. In contrast, SDF-1γ is located in very active, less vascularized organs susceptible to infarction such as the heart and the brain. The understanding of the functional diversity of the different splicing variants will help in developing therapeutic strategies.
Introduction
Stromal-derived factor 1 (SDF-1) was defined in a murine bone marrow (BM) stromal cell line supporting hematopoietic cell culture. The development of a new signal sequence trapping method contributed to the identification of the SDF-1 gene. Then the two splicing variants of the SDF-1 gene product were described: SDF-1α and SDF-1β, consisting of 89 and 93 amino acids (AA) respectively. They were assigned to the intercrine cytokine family (chemokines) which is characterized by four conserved cysteines that form two disulfide bonds. Furthermore their expression was found in all organs except in blood cells.Citation1 Independently, they were shown to be a sufficient factor capable of supporting rodent B-cell lymphopoiesis.Citation2 Shortly thereafter human equivalents were reported and the SDF-1 gene was located on chromosome 10q, unlike the remaining chemokines.Citation3 The striking similarity of the SDF-1 protein sequence between species and the gene's unusual chromosomal location allowed its essential role in the functioning of vertebrates to be anticipated. This was confirmed by the perinatal death of animals lacking SDF-1.Citation4 The identification of its cognate receptor (CXCR4) has brought us closer to understanding the functional complexity of SDF-1.Citation5,Citation6 CXCR4 has previously been known as a fusin due to its role in HIV entry into cells. Strong chemotactic activity in relation to monocytes/macrophages and lymphocytes but not neutrophils was found.Citation7 What is more important is that SDF-1 was also shown to be capable of controlling the migration of cells in the early stages of development, such as progenitor and stem cells.Citation7 Its effect on the biology of stem cells as well as HIV infection attracted many researchers to investigate in detail all aspects of SDF-1 function. Further data showed that the SDF-1/CXCR4 axis is also involved in the process of metastasis, chronic inflammatory disorders, benign proliferative diseasesCitation8 as well as the physiological function of bone marrow and the nervous system. Four more splicing variants were identified.Citation9 The structure of SDF-1 is a key factor in the understanding of the biological role of particular splicing variants.
SDF-1 Structure
SDF-1 proteins are found in living organisms as monomers. SDF-1α consists of three exons, and the remaining splicing variants of SDF-1 share the same three exons and have a different fourth exon attached to a C-terminal residue. Between the disordered N- and C-terminal ends there is a well-ordered structure consisting of three parallel β-strands and an overlying α-helix. The N-terminus (residues 1–8) is responsible for SDF-1's interaction with CXCR4. The first two AAs (amino acids) (Lys-1 and Pro-2) take part in receptor activation while a further six AAs are involved in the binding of the chemokine to the receptor.Citation10 The structured part together with the disordered C-terminus stabilizes receptor binding via its interaction with glycoseaminoglycans (GAGs) on the cell surface. The positive charge of SDF-1 proteins, resulting from numerous basic AAs is worthy of note. In this way, negatively charged GAGs attract and hold SDF-1 down. Each fourth exon is disordered and carries an additional strong positive charge. Splicing variants differ in the length of the fourth exon and consequently in the strength of GAG binding.
Splicing Variant-Based Regulatory Mechanisms of SDF-1 Activity
Degradation process.
SDF-1 is ubiquitously expressed in vertebrate tissues in a constitutive manner. Vertebrates have developed a method of maintaining the SDF-1 balance via the regulation of the degradation process.Citation11 SDF-1 is proteolytically degraded at both ends. Proteolysis of the N-terminus takes place slowly, is splicing variant-independent and occurs in both: the blood and the tissues. It switches off chemokine activity and decreases SDF-1's binding affinity to the receptor. Conversely, the degradation of the C-terminus is rapid, splicing variant-dependent and occurs only in the blood. It is mediated by carboxypeptidase-N (CPN), an enzyme also involved in the degradation of other proteins such as bradykinin and kallidin II.Citation12 C-terminus proteolysis does not inactivate SDF-1, but decreases its activity by half, attenuating GAG-dependent stabilization of chemokines on the cell surface. However only SDF-1α, which is the predominant and also the smallest isoform, is susceptible to this process. It is likely that vertebrates have developed an additional, C-terminus-dependent regulation mechanism of the most prevalent isoform—SDF-1α. It may prevent homeostatic disequilibrium resulting from a sudden release of SDF-1α from the tissues into the blood. The attachment of the fourth exon during the splicing process prevents proteolytic degradation of chemokines at the C-terminus in the blood (). Thus SDF-1β which is virtually the same as SDF-1α, except in that the fourth exon consists of only four residues attached to a C-terminus, shows very similar activity in vitro and in tissues, but is twice as potent in the blood.
Receptor binding.
Apart from susceptibility to C-terminus proteolysis, another regulatory mechanism of SDF-1 activity was observed. It was shown that GAG binding is necessary for leukocyte accumulation and prevents proteolytic attack, extending the lifespan of SDF-1 molecules.Citation13 Therefore, GAG binding affinity, which varies among SDF-1 splicing variants, provides another regulatory mechanism specific to the SDF-1/CXCR4 axis. The fourth exon of the SDF-1γ isoform is long and strongly basic, giving it the highest affinity for GAGs ever observed for any chemokine.Citation14 Thus, SDF-1γ binds to the cell surface immediately upon secretion and is almost never found in a disengaged state (). Although this splicing variant has less agonist potency, it is more efficient especially over a longer timeframe, thanks to the persistency of its interaction, which is mediated by the GAG-dependent stability of receptor binding. Intraperitoneal administration of SDF-1α and SDF-1γ produces virtually the same local inflammatory response after 3 hours, but after 16 hours, inflammatory reaction was present only in animals injected with SDF-1γ. SDF-1δ, SDF-1ε and SDF-1φ splicing variants have only been reported recently and no specific regulatory mechanism has been attributed to them to date.Citation9
Tissue Distribution of SDF-1 Splicing Variants
SDF-1α is the most widespread splicing variant.Citation12 It participates in many local tissue-specific physiological processes such as: the management of the HSC population in bone marrow,Citation15 the guidance of primordial germ cells during development,Citation16 neuromodulation in the CNS,Citation17 etc.
SDF-1β is expressed less abundantly and seems to be related to the vascular system. Its greater resistance to proteolysis within the blood predispose it to this role.Citation12 Endothelial cells of cerebral microvessels in mice express SDF-1β selectively. Its upregulation was found following focal cerebral ischemia and was associated with the infiltration of CXCR4-expressing peripheral blood cells, such as macrophages. At the same time neuronal SDF-1α was transiently downregulated.Citation18 SDF-1β also has a greater effect on angiogenesis in human microvascular endothelial cells (HMEC). In patients with systemic sclerosis, polymorphism within the C-terminal residues of SDF-1β predisposes to microvascular disease.Citation19 An estimate of the distribution of SDF-1β transcripts in human organs revealed their presence mainly in highly vascularized organs such as the liver, spleen, bone marrow and kidneys, and their absence in the brain.Citation9,Citation20
SDF-1γ is the predominant isoform in the adult rat brain, replacing SDF-1β which is abundant in embryonic life.Citation21 In humansCitation9 and mice,Citation22 SDF-1γ was found mainly in the heart. However, following an experimental heart infarct in mice, no change in the quantity of SDF-1γ was found, but there was an increase in SDF-1α expression.Citation22 The modest data on SDF-1γ distribution indicates an association with less vascularized organs susceptible to infarction such as the brain and the heart. Thus the expression of SDF-1γ may not support vascularization, but due to the persistency of its activity, it may ensure a strong and long-term inflammatory response to an infarct necessary for the removal of large areas of necrotic tissue.
SDF-1δ, SDF-1ε and SDF-1φ splicing variants have been described only recently in human tissues. They are all abundant in the pancreas. Additionally, SDF-1φ and SDF-1ε were found in the heart and liver, as well as in fetal and adult kidneys, but the expression of the former is more pronounced than that of the latter. SDF-1δ, on the other hand, was also detected in the spleen, fetal liver and lungs.
Thus different splicing variants display a similar effect in cells but are active in different tissues and physiological or pathological conditions.
Pathology-Specific Role
HIV infection.
The discovery of a natural ligand for the HIV co-receptor, CXCR4, opened new avenues in antiviral therapy. SDF-1 has been shown to interfere with HIV entry. Initial experiments suggested that SDF-1's antiviral activity is by way of downregulation and internalization of CXCR4.Citation23 However, other studies have suggested distinct mechanisms for the activation of CXCR4 and the inhibition of HIV infection.Citation10,Citation24 It seems that SDF-1's binding affinity to CXCR4 plays a pivotal role. In fact, SDF-1γ revealed enormous binding affinity to the cell surface and proved to be the most potent blocker of HIV entry among all the SDF-1 splicing variants.Citation24 Knowledge of SDF-1's antiviral effect was used for the synthesis of a small molecule (AMD3100) antagonizing the function of CXCR4. However, despite its potency in vitro,Citation25 it failed to be efficient in vivo.Citation26 The diversity of HIV phenotypes as well as a lack of GAG-dependent stabilization of AMD3100 on the cell surface could be the reason for its failure in clinical trials.
Metastatic malignancy.
The mechanism of metastatic malignancy was elusive for long time. Recent research on the SDF-1/CXCR4 axis has shed some light on this process. The cells of many investigated tumors do not produce SDF-1 but do express CXCR4.Citation27 In this way, a positive gradient develops between the tumor and the rest of the body. A high concentration of SDF-1 in lymph nodes and the resulting gradient inside lymphatic vessels strongly attracts tumor cells.Citation28–Citation30 This, in turn, may be responsible for the high frequency of metastases to the lymph nodes. SDF-1 activity in the blood seems to be less pronounced than in lymphatic vessels, but following entry into the blood, tumor cells progress further to the organs in accordance with the SDF-1 gradient. In this way, metastases are predominantly located in organs of higher SDF-1 concentration such as the lungs, bones, adrenal glands, and not in the heart or kidneys where SDF-1 expression is lower.Citation27 The blood concentration of SDF-1 correlates positively with the presence of distant metastases in patients with gastric cancer.Citation31 The elimination of CXCR4-bearing cells from pancreatic cancer selectively suppresses metastatic activity, without affecting tumorigenesis.Citation32 The identification of the anti-metastatic substance, Zerumbone, whose activity is based on CXCR4 down-regulation has been reported recently.Citation33 To conclude, a metastasis is not a passive and random occurrence, but is the result of an active migratory process.
Chronic inflammatory disorders.
Chemokines also play a key role in the accumulation of inflammatory cells.Citation34 Their aberrant expression may be responsible for the induction of chronic inflammatory processes such as rheumatoid arthritis (RA),Citation35 multiple sclerosis (MS),Citation36 colitis ulcerosa (CU)Citation37 and allergic diseases.Citation38 SDF-1 may be a common downstream executor of inflammatory cell accumulation regardless of circumstances upregulating its expression. This gives reason to hope that low molecular weight CXCR4 antagonists could be applied in chronic inflammatory disorders too.Citation39,Citation40 The functional role of particular SDF-1 splicing variants in the context of chronic inflammation has not yet been explored. However, one can hypothesize about the involvement of the splicing process and the disequilibrium of the proportions of particular variants.
Organ-Specific Function
BM.
SDF-1 is critical for the maintenance of a proper BM micro-environment. It fetters progenitor and stem cells in the BM by creating a positive BM-blood gradient. The homing of hematopoietic stem cells (HSC) to BM following transplantation is also mediated by SDF-1.Citation41 The mechanism of stem cell mobilization is not based on the release of SDF-1 to the blood but, quite to the contrary, on the disruption of SDF-1's binding to CXCR4 in the BM. The traditional mobilizing factor—G-CSF increases the activity of N-terminus proteases (elastase, MMPs and catepsin G) in the BM, which degrades both: SDF-1 and to a lesser degree CXCR4, as well as the C-terminus protease of SDF-1—carboxypeptidase M.Citation42 SDF-1 proteolysis in the BM causes a reversion in the BM-blood gradient of this chemokine and a release of CXCR4-bearing cells into the blood. However, cells mobilized in this way are characterized by reduced chemotactic activity due to the partial destruction of CXCR4.Citation15 The discovery of a mechanism of stem cell mobilization from the BM resulted in the introduction of novel mobilizing factors that interact with CXCR4 directly. CXCR4 blocking by the small molecule antagonist AMD3100 proved to be very efficient even in cases of previous G-CSF failure.Citation43 Additionally, cells mobilized in this way retain their chemotactic properties in the blood.Citation44
Brain.
Like in other organs, SDF-1 also guides cells in the brain during the developmental period. Its role in the migration of embryonic cerebellar neurons from the external granular layer (EGL) to the internal granular layer (IGL) has been well defined.Citation45 It is executed by the predominant isoform, SDF-1α, produced by nearby meningeal cells.Citation46,Citation47 Recently, the crucial role of SDF-1α in the development of the cerebral cortex and the dentate gyrus has also been reported.Citation48,Citation49 The regulation of the migration of dentate gyrus cells by SDF-1 continues throughout adulthood.Citation50–Citation52 It also participates in stroke-induced neuroblast migration from SVZ towards the lesioned area.Citation53,Citation54 Apart from its migratory function, SDF-1 has also been shown to be engaged in neuromodulationCitation17,Citation55 and axonal elongation from the entorhinal cortex towards the dentate gyrus.Citation56 There is a species-specific predominance of particular splicing variants in the brain. In mice SDF-1β is the most abundant isoform, while in rats, it is SDF-1γ. In contrast, in the human brain both these isoforms are absent and only transcripts of SDF-1α have been found.Citation9 One may speculate that the difference in tissue reaction to stroke could be attributed to species-specific isoform variability. Stroke in rodents elicits enormous attraction of blood leukocytes to the ischemic area followed by cavity formation. The inflammatory response of the human brain to infarction seems to be more limited, as very often preservation of tissue in the ischemic area is visible on control MR or CT scans. Such a difference could be ascribed to the splicing variance of SDF-1. The SDF-1β and SDF-1γ isoforms are more efficient in the blood, and so they are able to produce a strong chemotaxis of leukocytes in rodents. The human brain has only got SDF-1α at its disposal which is less effective in the blood due to C-terminus proteolysis and as a consequence leukocyte infiltration and cavity formation could be less prominent.
CXCR7 - An Alternative Receptor for SDF-1
Recently, an alternative receptor for SDF-1 has been described.Citation57,Citation58 It was initially reported as RDC1 in 1994,Citation59 but only lately has the ligand for it been found. CXCR7 reveals high binding affinity to SDF-1 and CXCL11. It is a G-protein coupled receptor, but does not respond to Pertussis toxin application. Details of complementaryCitation60 and antagonisticCitation61 interactions of both receptors: CXCR4 and CXCR7 were published. Its role in the development of the cardiovascular system was established in mice by inducing CXCR7 genetic deficiency.Citation62 No developmental defects within the CNS were described.Citation63 Focal brain ischemia upregulates CXCR7 expression in intact cortical regions but no migration of CXCR7-bearing cells was visible.Citation64 CXCR7 is widely expressed in CXCR4-negative neuronal, vascular and glial cells but its function was not yet understood.Citation65 A recent report about zebrafish primordial germ cell (PGC) migration revealed a scavenging role for CXCR7. It is expressed by somatic cells surrounding PGC chains, which through the uptake and sequestration of SDF-1 create a sharper directional gradient.Citation16 A similar scavenging role for CXCR7 in the CNS cannot be ruled out. The influence of SDF-1 splicing variants on CXCR7 function has not yet been raised.
Cell-Based Therapy
Many critical cell functions such as: migration, proliferation and apoptosis inhibition are regulated by SDF-1. Thus there is value in employing this knowledge in the emerging field of cell transplantation.
SDF-1 can be employed for the appropriate trafficking of grafted cells. It guides intravenously administered CXCR4-bearing cells to their destinations such as into an area of spinal cord injury,Citation66 strokeCitation67 or myocardial infarction.Citation68,Citation69 It also promotes the survival and stimulates the migration of intracerebrally injected cells towards the stroke area,Citation70 and, intraventricularly administered, towards the area of traumatic injury.Citation71 Intraparenchymal injection of SDF-1 into an intact brain additionally enhanced transplanted cell homing,Citation72 whereas such an injection to an infarcted heart failed to recruit stem cells due to protease activity. The construction of a proteolysis resistant form of SDF-1 (S4V) has overcome this limitation.Citation73
SDF-1 can mediate a cell-dependent effect as well. The secretion of SDF-1 by transplanted cells enhanced neuroplasticity within the injured brain,Citation74 preserved myocytes within the infarcted zone of the heart,Citation75 and promoted the recruitment of stem and progenitor cells which resulted in enhanced angiomyogenesis and in an improvement in the contractile function of the heart.Citation76 SDF-1 was also used for ex vivo priming of progenitors to promote conversion towards the desired phenotype. Such an approach was employed for angiogenesisCitation77 and myocardial infarction.Citation78
The abovementioned mechanisms of SDF-1 involvement in cell-based therapies are associated with the presence of grafted cells at the diseased spots. However several papers revealed a clear positive effect of cell transplantation in animal models of stroke despite the absence of grafted cells within the brain.Citation79,Citation80 This phenomenon has not yet been explained. Thus the author of this review speculates on the pivotal role of SDF-1 in the achievement of these results. The production of SDF-1 by transplanted cells homed to peripheral organs increases the blood concentration of SDF-1 and consequently decreases the positive brain-blood gradient following stroke, thus preventing excessive leukocyte migration toward the infarcted tissue and limiting secondary brain injury.
Conclusions
Unlike other chemokines, SDF-1 is chiefly regulated at the splicing, and not transcriptional level. SDF-1α is the predominant and ubiquitously expressed splicing variant. It can play different roles (motogen, mitogen, neuromodulator) in various tissues. However it is rapidly degraded after entry into the blood. It is likely that in vertebrates this isoform is reserved for intra-organ signaling via local gradients. The production of the SDF-1β isoform is more limited, but due to its activity in the blood it seems to be designed for inter-organ communication via blood-mediated gradients as well as for supporting angiogenesis. Due to rapid and strong binding to GAGs, SDF-1γ reveals paracrine activity and is a potent inductor of local long-term inflammatory response. It was found in organs characterized by high activity, low vascularity and susceptibility to ischemia (brain and heart). It seems to function as a medium ensuring the efficient removal of large areas of necrotic tissue. A better understanding of the functional diversity of SDF-1 splicing variants should make usage of the SDF-1/CXCR4 axis more specific for therapeutic purposes.
Abbreviations
AA | = | aminoacids |
AMD3100 | = | the company name of the substance |
BM | = | bone marrow |
CNS | = | central nervous system |
CPN | = | carboxypeptidase-N |
CT | = | computed tomography |
CU | = | colitis ulcerosa |
CXCL11 | = | C-X-C ligand 11 |
CXCR4 | = | C-X-C receptor 4 |
CXCR7 | = | C-X-C receptor 7 |
EGL | = | external granular layer |
GAGs | = | glycosaminoglycans |
G-CSF | = | granulocyte-colony stimulating factor |
HIV | = | human immunodeficiency virus |
HMEC | = | human microvascular endothelial cells |
IGL | = | internal granular layer |
MMPs | = | metaloproteinases |
MR | = | magnetic resonance |
MS | = | multiple sclerosis |
PGC | = | primordial germ cell |
RA | = | rheumatoid arthritis |
SDF-1 | = | stromal-derived factor 1 |
SVZ | = | subventricular zone |
Figures and Tables
Figure 1 Splicing variant-dependent susceptibility to the degradation process of SDF-1 in blood. Large squares correspond to the ordered structure of the protein. Disordered ends are represented by thinner shapes. The regions of the protein resistant to proteolysis are marked in blue, while parts colored in red represent endings susceptible to the degradation process. The lowest row of words describes the contribution of particular exons to the final structure of the SDF-1 protein. The scissors symbolize the sites of proteinase attack. The large gray arrow in the center of the figure points to the phenomenon of C-terminus degradation prevention by an attachment of a fourth exon resistant to proteolysis during the splicing process.
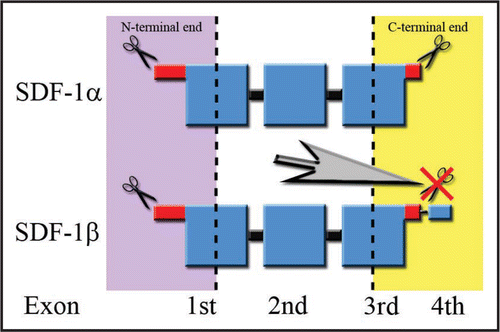
Figure 2 Splicing variant-dependent strength of SDF-1 binding to CXCR4 on the cell surface. The cell membrane is covered by negatively charged GAGs shown as blue spots. The active part of the SDF-1 molecule binding to the receptor is marked in green. Red shapes represent the positively charged part of the SDF-1 molecule stabilizing the process of receptor activation through their interaction with the negatively charged GAGs. (A) The red oval reflects the “standard” positive charge characteristic of all SDF-1 molecules. It is similar for SDF-1α and SDF-1β and is strong enough only for short term receptor binding. Thus, only a limited number of SDF-1 molecules interact with the receptor at the same time, while most of them are in an unbound state. (B) In contrast, the addition of a long, strongly positively charged product of the fourth exon in the case of the SDF-1γ isoform, which is represented in the figure by a “tail” of red spots, facilitates a very strong and long-term stabilization of SDF-1 binding to CXCR4. It results in the persistency of SDF-1 activity which is shown by widespread binding of the SDF-1 molecule to its cognate receptor.
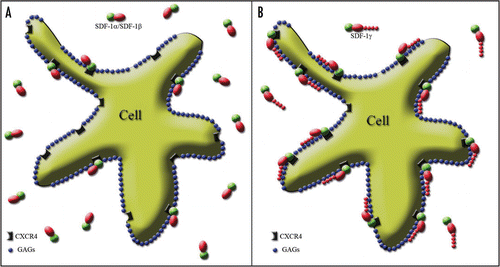
References
- Tashiro K, Tada H, Heilker R, Shirozu M, Nakano T, Honjo T. Signal sequence trap: a cloning strategy for secreted proteins and type I membrane proteins. Science 1993; 261:600 - 603
- Nagasawa T, Kikutani H, Kishimoto T. Molecular cloning and structure of a pre-B-cell growth-stimulating factor. Proc Natl Acad Sci USA 1994; 91:2305 - 2309
- Shirozu M, Nakano T, Inazawa J, Tashiro K, Tada H, Shinohara T, et al. Structure and chromosomal localization of the human stromal cell-derived factor 1 (SDF1) gene. Genomics 1995; 28:495 - 500
- Nagasawa T, Hirota S, Tachibana K, Takakura N, Nishikawa S, Kitamura Y, et al. Defects of B-cell lymphopoiesis and bone-marrow myelopoiesis in mice lacking the CXC chemokine PBSF/SDF-1. Nature 1996; 382:635 - 638
- Oberlin E, Amara A, Bachelerie F, Bessia C, Virelizier JL, Arenzana-Seisdedos F, et al. The CXC chemokine SDF-1 is the ligand for LESTR/fusin and prevents infection by T-cell-line-adapted HIV-1. Nature 1996; 382:833 - 835
- Bleul CC, Farzan M, Choe H, Parolin C, Clark-Lewis I, Sodroski J, et al. The lymphocyte chemoattractant SDF-1 is a ligand for LESTR/fusin and blocks HIV-1 entry. Nature 1996; 382:829 - 833
- Bleul CC, Fuhlbrigge RC, Casasnovas JM, Aiuti A, Springer TA. A highly efficacious lymphocyte chemoattractant, stromal cell-derived factor 1 (SDF-1). J Exp Med 1996; 184:1101 - 1109
- Begley LA, MacDonald JW, Day ML, Macoska JA. CXCL12 activates a robust transcriptional response in human prostate epithelial cells. J Biol Chem 2007; 282:26767 - 26774
- Yu L, Cecil J, Peng SB, Schrementi J, Kovacevic S, Paul D, et al. Identification and expression of novel isoforms of human stromal cell-derived factor 1. Gene 2006; 374:174 - 179
- Crump MP, Gong JH, Loetscher P, Rajarathnam K, Amara A, Arenzana-Seisdedos F, et al. Solution structure and basis for functional activity of stromal cell-derived factor-1; dissociation of CXCR4 activation from binding and inhibition of HIV-1. EMBO J 1997; 16:6996 - 7007
- De La Luz Sierra M, Yang F, Narazaki M, Salvucci O, Davis D, Yarchoan R, et al. Differential processing of stromal-derived factor-1alpha and stromal-derived factor-1beta explains functional diversity. Blood 2004; 103:2452 - 2459
- Davis DA, Singer KE, De La Luz Sierra M, Narazaki M, Yang F, Fales HM, et al. Identification of carboxypeptidase N as an enzyme responsible for C-terminal cleavage of stromal cell-derived factor-1alpha in the circulation. Blood 2005; 105:4561 - 4568
- Rueda P, Balabanian K, Lagane B, Staropoli I, Chow K, Levoye A, et al. The CXCL12gamma chemokine displays unprecedented structural and functional properties that make it a paradigm of chemoattractant proteins. PLoS ONE 2008; 3:2543
- Laguri C, Sadir R, Rueda P, Baleux F, Gans P, Arenzana-Seisdedos F, et al. The novel CXCL12gamma isoform encodes an unstructured cationic domain which regulates bioactivity and interaction with both glycosaminoglycans and CXCR4. PLoS ONE 2007; 2:1110
- Weidt C, Niggemann B, Kasenda B, Drell TL, Zanker KS, Dittmar T. Stem cell migration: a quintessential stepping stone to successful therapy. Curr Stem Cell Res Ther 2007; 2:89 - 103
- Boldajipour B, Mahabaleshwar H, Kardash E, Reichman-Fried M, Blaser H, Minina S, et al. Control of chemokine-guided cell migration by ligand sequestration. Cell 2008; 132:463 - 473
- Limatola C, Giovannelli A, Maggi L, Ragozzino D, Castellani L, Ciotti MT, et al. SDF1alpha-mediated modulation of synaptic transmission in rat cerebellum. Eur J Neurosci 2000; 12:2497 - 2504
- Stumm RK, Rummel J, Junker V, Culmsee C, Pfeiffer M, Krieglstein J, et al. A dual role for the SDF-1/CXCR4 chemokine receptor system in adult brain: isoform-selective regulation of SDF-1 expression modulates CXCR4-dependent neuronal plasticity and cerebral leukocyte recruitment after focal ischemia. J Neurosci 2002; 22:5865 - 5878
- Manetti M, Liakouli V, Fatini C, Cipriani P, Bonino C, Vettori S, et al. Association between a stromal cell-derived factor 1 (SDF-1/CXCL12) gene polymorphism and microvascular disease in systemic sclerosis. Ann Rheum Dis 2008;
- Colobran R, Pujol-Borrell R, Armengol MP, Juan M. The chemokine network II. On how polymorphisms and alternative splicing increase the number of molecular species and configure intricate patterns of disease susceptibility. Clin Exp Immunol 2007; 150:1 - 12
- Gleichmann M, Gillen C, Czardybon M, Bosse F, Greiner-Petter R, Auer J, et al. Cloning and characterization of SDF-1gamma, a novel SDF-1 chemokine transcript with developmentally regulated expression in the nervous system. Eur J Neurosci 2000; 12:1857 - 1866
- Pillarisetti K, Gupta SK. Cloning and relative expression analysis of rat stromal cell derived factor-1 (SDF-1)1: SDF-1 alpha mRNA is selectively induced in rat model of myocardial infarction. Inflammation 2001; 25:293 - 300
- Amara A, Gall SL, Schwartz O, Salamero J, Montes M, Loetscher P, et al. HIV coreceptor downregulation as antiviral principle: SDF-1alpha-dependent internalization of the chemokine receptor CXCR4 contributes to inhibition of HIV replication. J Exp Med 1997; 186:139 - 146
- Altenburg JD, Broxmeyer HE, Jin Q, Cooper S, Basu S, Alkhatib G. A naturally occurring splice variant of CXCL12/stromal cell-derived factor 1 is a potent human immunodeficiency virus type 1 inhibitor with weak chemotaxis and cell survival activities. J Virol 2007; 81:8140 - 8148
- Schols D, Este JA, Henson G, De Clercq E. Bicyclams, a class of potent anti-HIV agents, are targeted at the HIV coreceptor fusin/CXCR-4. Antiviral Res 1997; 35:147 - 156
- Hendrix CW, Collier AC, Lederman MM, Schols D, Pollard RB, Brown S, et al. Safety, pharmacokinetics, and antiviral activity of AMD3100, a selective CXCR4 receptor inhibitor, in HIV-1 infection. J Acquir Immune Defic Syndr 2004; 37:1253 - 1262
- Phillips RJ, Burdick MD, Lutz M, Belperio JA, Keane MP, Strieter RM. The stromal derived factor-1/CXCL12-CXC chemokine receptor 4 biological axis in non-small cell lung cancer metastases. Am J Respir Crit Care Med 2003; 167:1676 - 1686
- Zhang JP, Lu WG, Ye F, Chen HZ, Zhou CY, Xie X. Study on CXCR4/SDF-1alpha axis in lymph node metastasis of cervical squamous cell carcinoma. Int J Gynecol Cancer 2007; 17:478 - 483
- Yoshitake N, Fukui H, Yamagishi H, Sekikawa A, Fujii S, Tomita S, et al. Expression of SDF-1 alpha and nuclear CXCR4 predicts lymph node metastasis in colorectal cancer. Br J Cancer 2008; 98:1682 - 1689
- Kaifi JT, Yekebas EF, Schurr P, Obonyo D, Wachowiak R, Busch P, et al. Tumor-cell homing to lymph nodes and bone marrow and CXCR4 expression in esophageal cancer. J Natl Cancer Inst 2005; 97:1840 - 1847
- Woo IS, Hong SH, Byun JH, Kang JH, Jeon HM, Choi MG. Circulating stromal cell derived factor-1alpha (SDF-1alpha) is predictive of distant metastasis in gastric carcinoma. Cancer Invest 2008; 26:256 - 261
- Hermann PC, Huber SL, Herrler T, Aicher A, Ellwart JW, Guba M, et al. Distinct populations of cancer stem cells determine tumor growth and metastatic activity in human pancreatic cancer. Cell Stem Cell 2007; 1:313 - 323
- Sung B, Jhurani S, Ahn KS, Mastuo Y, Yi T, Guha S, et al. Zerumbone downregulates chemokine receptor CXCR4 expression leading to inhibition of CXCL12-induced invasion of breast and pancreatic tumor cells. Cancer Res 2008; 68:8938 - 8944
- Iwamoto T, Okamoto H, Toyama Y, Momohara S. Molecular aspects of rheumatoid arthritis: chemokines in the joints of patients. Febs J 2008; 275:4448 - 4455
- Kim KW, Cho ML, Kim HR, Ju JH, Park MK, Oh HJ, et al. Upregulation of stromal cell-derived factor 1 (CXCL12) production in rheumatoid synovial fibroblasts through interactions with T lymphocytes: role of interleukin-17 and CD40L-CD40 interaction. Arthritis Rheum 2007; 56:1076 - 1086
- McCandless EE, Piccio L, Woerner BM, Schmidt RE, Rubin JB, Cross AH, et al. Pathological expression of CXCL12 at the blood-brain barrier correlates with severity of multiple sclerosis. Am J Pathol 2008; 172:799 - 808
- Mikami S, Nakase H, Yamamoto S, Takeda Y, Yoshino T, Kasahara K, et al. Blockade of CXCL12/CXCR4 axis ameliorates murine experimental colitis. J Pharmacol Exp Ther 2008; 327:383 - 392
- Elisseeva EL, Slupsky CM, Crump MP, Clark-Lewis I, Sykes BD. NMR studies of active N-terminal peptides of stromal cell-derived factor-1. Structural basis for receptor binding. J Biol Chem 2000; 275:26799 - 26805
- Tamamura H, Tsutsumi H, Masuno H, Fujii N. Development of low molecular weight CXCR4 antagonists by exploratory structural tuning of cyclic tetra- and pentapeptidescaffolds towards the treatment of HIV infection, cancer metastasis and rheumatoid arthritis. Curr Med Chem 2007; 14:93 - 102
- Hachet-Haas M, Balabanian K, Rohmer F, Pons F, Franchet C, Lecat S, et al. Small neutralizing molecules to inhibit actions of the chemokine CXCL12. J Biol Chem 2008; 283:23189 - 23199
- Marquez-Curtis LA, Turner AR, Larratt LM, Letcher B, Lee SF, Janowska-Wieczorek A. CD34+ cell responsiveness to stromal cell-derived factor-1alpha underlies rate of engraftment after peripheral blood stem cell transplantation. Transfusion 2008; 49:161 - 169
- Marquez-Curtis L, Jalili A, Deiteren K, Shirvaikar N, Lambeir AM, Janowska-Wieczorek A. Carboxypeptidase M expressed by human bone marrow cells cleaves the C-terminal lysine of stromal cell-derived factor-1alpha: another player in hematopoietic stem/progenitor cell mobilization?. Stem Cells 2008; 26:1211 - 1220
- Uy GL, Rettig MP, Cashen AF. Plerixafor, a CXCR4 antagonist for the mobilization of hematopoietic stem cells. Expert Opin Biol Ther 2008; 8:1797 - 1804
- Pelus LM, Fukuda S. Chemokine-mobilized adult stem cells; defining a better hematopoietic graft. Leukemia 2008; 22:466 - 473
- Zhu Y, Yu T, Zhang XC, Nagasawa T, Wu JY, Rao Y. Role of the chemokine SDF-1 as the meningeal attractant for embryonic cerebellar neurons. Nat Neurosci 2002; 5:719 - 720
- Yacubova E, Komuro H. Cellular and molecular mechanisms of cerebellar granule cell migration. Cell Biochem Biophys 2003; 37:213 - 234
- Reiss K, Mentlein R, Sievers J, Hartmann D. Stromal cell-derived factor 1 is secreted by meningeal cells and acts as chemotactic factor on neuronal stem cells of the cerebellar external granular layer. Neuroscience 2002; 115:295 - 305
- Liapi A, Pritchett J, Jones O, Fujii N, Parnavelas JG, Nadarajah B. Stromal-derived factor 1 signalling regulates radial and tangential migration in the developing cerebral cortex. Dev Neurosci 2008; 30:117 - 131
- Bagri A, Gurney T, He X, Zou YR, Littman DR, Tessier-Lavigne M, et al. The chemokine SDF1 regulates migration of dentate granule cells. Development 2002; 129:4249 - 4260
- Tham TN, Lazarini F, Franceschini IA, Lachapelle F, Amara A, Dubois-Dalcq M. Developmental pattern of expression of the alpha chemokine stromal cell-derived factor 1 in the rat central nervous system. Eur J Neurosci 2001; 13:845 - 856
- Berger O, Li G, Han SM, Paredes M, Pleasure SJ. Expression of SDF-1 and CXCR4 during reorganization of the postnatal dentate gyrus. Dev Neurosci 2007; 29:48 - 58
- Tran PB, Banisadr G, Ren D, Chenn A, Miller RJ. Chemokine receptor expression by neural progenitor cells in neurogenic regions of mouse brain. J Comp Neurol 2007; 500:1007 - 1033
- Thored P, Arvidsson A, Cacci E, Ahlenius H, Kallur T, Darsalia V, et al. Persistent production of neurons from adult brain stem cells during recovery after stroke. Stem Cells 2006; 24:739 - 747
- Ohab JJ, Carmichael ST. Poststroke neurogenesis: emerging principles of migration and localization of immature neurons. Neuroscientist 2008; 14:369 - 380
- Ragozzino D, Renzi M, Giovannelli A, Eusebi F. Stimulation of chemokine CXC receptor 4 induces synaptic depression of evoked parallel fibers inputs onto Purkinje neurons in mouse cerebellum. J Neuroimmunol 2002; 127:30 - 36
- Ohshima Y, Kubo T, Koyama R, Ueno M, Nakagawa M, Yamashita T. Regulation of axonal elongation and pathfinding from the entorhinal cortex to the dentate gyrus in the hippocampus by the chemokine stromal cell-derived factor 1 alpha. J Neurosci 2008; 28:8344 - 8353
- Balabanian K, Lagane B, Infantino S, Chow KY, Harriague J, Moepps B, et al. The chemokine SDF-1/CXCL12 binds to and signals through the orphan receptor RDC1 in T lymphocytes. J Biol Chem 2005; 280:35760 - 35766
- Burns JM, Summers BC, Wang Y, Melikian A, Berahovich R, Miao Z, et al. A novel chemokine receptor for SDF-1 and I-TAC involved in cell survival, cell adhesion and tumor development. J Exp Med 2006; 203:2201 - 2213
- Law NM, Rosenzweig SA. Characterization of the G-protein linked orphan receptor GPRN1/RDC1. Biochem Biophys Res Commun 1994; 201:458 - 465
- Mazzinghi B, Ronconi E, Lazzeri E, Sagrinati C, Ballerini L, Angelotti ML, et al. Essential but differential role for CXCR4 and CXCR7 in the therapeutic homing of human renal progenitor cells. J Exp Med 2008; 205:479 - 490
- Dambly-Chaudiere C, Cubedo N, Ghysen A. Control of cell migration in the development of the posterior lateral line: antagonistic interactions between the chemokine receptors CXCR4 and CXCR7/RDC1. BMC Dev Biol 2007; 7:23
- Gerrits H, van Ingen Schenau DS, Bakker NE, van Disseldorp AJ, Strik A, Hermens LS, et al. Early postnatal lethality and cardiovascular defects in CXCR7-deficient mice. Genesis 2008; 46:235 - 245
- Sierro F, Biben C, Martinez-Munoz L, Mellado M, Ransohoff RM, Li M, et al. Disrupted cardiac development but normal hematopoiesis in mice deficient in the second CXCL12/SDF-1 receptor, CXCR7. Proc Natl Acad Sci USA 2007; 104:14759 - 14764
- Schonemeier B, Schulz S, Hoellt V, Stumm R. Enhanced expression of the CXCl12/SDF-1 chemokine receptor CXCR7 after cerebral ischemia in the rat brain. J Neuroimmunol 2008; 198:39 - 45
- Schonemeier B, Kolodziej A, Schulz S, Jacobs S, Hoellt V, Stumm R. Regional and cellular localization of the CXCl12/SDF-1 chemokine receptor CXCR7 in the developing and adult rat brain. J Comp Neurol 2008; 510:207 - 220
- Takeuchi H, Natsume A, Wakabayashi T, Aoshima C, Shimato S, Ito M, et al. Intravenously transplanted human neural stem cells migrate to the injured spinal cord in adult mice in an SDF-1- and HGF-dependent manner. Neurosci Lett 2007; 426:69 - 74
- Wang Y, Deng Y, Zhou GQ. SDF-1alpha/CXCR4-mediated migration of systemically transplanted bone marrow stromal cells towards ischemic brain lesion in a rat model. Brain Res 2008; 1195:104 - 112
- Ma N, Stamm C, Kaminski A, Li W, Kleine HD, Muller-Hilke B, et al. Human cord blood cells induce angiogenesis following myocardial infarction in NOD/scid-mice. Cardiovasc Res 2005; 66:45 - 54
- Ma J, Ge J, Zhang S, Sun A, Shen J, Chen L, et al. Time course of myocardial stromal cell-derived factor 1 expression and beneficial effects of intravenously administered bone marrow stem cells in rats with experimental myocardial infarction. Basic Res Cardiol 2005; 100:217 - 223
- Shichinohe H, Kuroda S, Yano S, Hida K, Iwasaki Y. Role of SDF-1/CXCR4 system in survival and migration of bone marrow stromal cells after transplantation into mice cerebral infarct. Brain Res 2007; 1183:138 - 147
- Ji JF, He BP, Dheen ST, Tay SS. Interactions of chemokines and chemokine receptors mediate the migration of mesenchymal stem cells to the impaired site in the brain after hypoglossal nerve injury. Stem Cells 2004; 22:415 - 427
- Corti S, Locatelli F, Papadimitriou D, Donadoni C, Del Bo R, Fortunato F, et al. Multipotentiality, homing properties, and pyramidal neurogenesis of CNS-derived LeX(ssea-1)+/CXCR4+ stem cells. Faseb J 2005; 19:1860 - 1862
- Segers VF, Tokunou T, Higgins LJ, MacGillivray C, Gannon J, Lee RT. Local delivery of protease-resistant stromal cell derived factor-1 for stem cell recruitment after myocardial infarction. Circulation 2007; 116:1683 - 1692
- Shyu WC, Liu DD, Lin SZ, Li WW, Su CY, Chang YC, et al. Implantation of olfactory ensheathing cells promotes neuroplasticity in murine models of stroke. J Clin Invest 2008; 118:2482 - 2495
- Zhang M, Mal N, Kiedrowski M, Chacko M, Askari AT, Popovic ZB, et al. SDF-1 expression by mesenchymal stem cells results in trophic support of cardiac myocytes after myocardial infarction. Faseb J 2007; 21:3197 - 3207
- Elmadbouh I, Haider H, Jiang S, Idris NM, Lu G, Ashraf M. Ex vivo delivered stromal cell-derived factor-1alpha promotes stem cell homing and induces angiomyogenesis in the infarcted myocardium. J Mol Cell Cardiol 2007; 42:792 - 803
- Zemani F, Silvestre JS, Fauvel-Lafeve F, Bruel A, Vilar J, Bieche I, et al. Ex vivo priming of endothelial progenitor cells with SDF-1 before transplantation could increase their proangiogenic potential. Arterioscler Thromb Vasc Biol 2008; 28:644 - 650
- Pasha Z, Wang Y, Sheikh R, Zhang D, Zhao T, Ashraf M. Preconditioning enhances cell survival and differentiation of stem cells during transplantation in infarcted myocardium. Cardiovasc Res 2008; 77:134 - 142
- Willing AE, Lixian J, Milliken M, Poulos S, Zigova T, Song S, et al. Intravenous versus intrastriatal cord blood administration in a rodent model of stroke. J Neurosci Res 2003; 73:296 - 307
- Borlongan CV, Hadman M, Sanberg CD, Sanberg PR. Central nervous system entry of peripherally injected umbilical cord blood cells is not required for neuroprotection in stroke. Stroke 2004; 35:2385 - 2389