Abstract
The p53 transcription factor, discovered in 19791;2, is well known as a potent suppressor of tumor development by inhibiting cell cycle progression, and promoting senescence or apoptosis, when the genome is compromised or under oncogenic stress3. Accumulating evidence has pointed to an alternative role of p53 in the curtailment of tumor progression and colonization of secondary sites by negatively regulating tumor cell metastasis4;5. Recently, we have found that p53 suppresses Src-induced formation of podosomes and associated invasive phenotypes in fibroblasts and vascular smooth muscle cells (VSMC)6;7. In this review, I will focus on some recent studies that have identified p53 as a suppressor of cell migration and invasion in general, and VSMC podosome formation and ECM degradation in particular.
A Lesser Known Role of p53: Suppression of Cell Migration and Invasion
Is p53, often dubbed “guardian of the genome,” also a suppressor of cell migration and invasion? There were hints. One arose from the identification of p53 transcriptional targets that code for regulators of cell migration and invasion, such as smooth muscle α-actin,Citation8 vascular endothelial growth factor (VEGF),Citation9 MMP1,Citation10 and caldesmon.Citation11 Another came from the finding that p53 associates with cytoskeletal structures such as actin filaments,Citation12 microtubulesCitation13 and intermediate filaments,Citation14 albeit without any clear physiological significance. Valuable clues can also be gleaned from studying the effects of wild-type and p53 mutants on the invasion potential of tumor cells. Over 50% of cancers are associated with p53 mutations, many of which involve point mutations clustered at the DNA-binding domain of the protein, and the mutated proteins are expressed in high level in tumors. Mutations in p53 that result in a loss of its wild-type function in cell growth suppression often cause a gain of functions in cell migration and invasion. For example, expression of two p53 mutants (R175H and R273H) drives invasion in lung cancer cells via enhanced integrin and EGFR trafficking.Citation15 Other p53 mutants (R248W, R280H and R273H) increase cancer cell invasion by inhibiting MDM2-mediated degradation of Slug, an invasion promoter that represses E-cadherin transcription; in contrast, wild-type p53 upregulates MDM2-mediated Slug degradation, and hence suppresses invasion.Citation16
The mechanism of regulation has not been clearly defined, but there is convincing evidence that downregulation of cell migration by p53 is mediated by RhoGTPases, the key modulators of cytoskeletal remodeling. For instance, depletion of p53 leads to upregulation of RhoGTPases, Rac 1 in particular, and induction of cell migration and cell proliferation in MEF cells.Citation17 Upregulation of wild-type p53 prevents Cdc42-mediated formation of filopodia,Citation18 cell polarization and cell spreadingCitation19 in MEF cells, while p53-deficient MEF cells cultured in 3D matrix acquire an enhanced invasive amoeboid phenotype that requires RhoA-ROCK signaling.Citation20
If p53 is engaged in the regulation of cell cycle and cytoskeleton remodeling, are the dual functions of p53 coordinated or independently executed? While a definitive answer must await further investigations, recent studies have suggested that JMY (junction-mediating and regulatory protein), a positive co-factor of p53 transcriptional functions, may act as a coordinator of p53 functions in the nucleus and the cytoskeleton.Citation21,Citation22 JMY is primarily nuclear in undifferentiated HL-60 cells assisting transcriptional functions of p53. However, in differentiated, highly mobile cells, JMY becomes exclusively cytosolic, co-localized with actin filaments at the cell cortex, where it nucleates actin filaments in an Arp2/3-dependent and -independent manner.
p53 and Atherosclerosis
What p53 does in vitro appears to be straightforward and consistent: it inhibits cell proliferation and promotes apoptosis, and suppresses cell invasion. Based on evidence from p53-knockout mice, its role in atherosclerosis has been surprising and often contradictory, although there appears to be a general consensus that global knockout of p53, for example, in p53-/-/apoE-/- mice, accelerates aortic atherosclerosis.Citation23,Citation24 While endogenous p53 appears to protect against apoptosis, increase in p53 promotes cell apoptosis in atherosclerotic plaques.Citation25 It is understandable that interpretation of atherosclerotic phenotypes in global p53-null mice is complicated by the fact that the role of p53 varies significantly in different cell types, such as macrophages and VSMC, which make up the complex plaque tissue. With recent findings implicating an alternative role of p53 in VSMC invasion, it would be of interest to limit p53-deletion in VSMC using a smooth muscle cell-targeted, conditional p53-knockout in adult mice to investigate the role of p53 in VSMC migration and ECM invasion in the initiation of intimal thickening and plaque formation, and subsequent plaque stability.
Migration of Vascular Smooth Muscle Cells Across Tissue Boundaries in Atherosclerosis
Cross-tissue migration and proliferation of VSMC in the arterial wall cause intimal thickening that sets the stage for the pathogenesis of atherosclerosis and plaque formation.Citation26 Atherosclerotic plaque formation and the danger of plaque rupture are major causes of atherosclerosis-related, acute cardiovascular events such as myocardial infarction. While the accumulation of VSMC and their collagen deposits in the fibrous cap stabilize the atherosclerotic plaque, macrophages and lipid contents destabilize it. Thus, migration and invasion potentials of VSMC have a direct impact on plaque formation and stability.
Differentiated VSMC in the medial layer of the arterial wall are furnished with an array of contractile myosin-actin filaments, aligned along the longitudinal axis of the cell for maintenance of vascular tone. In response to endothelial injuries, VSMC in the medial layer of arterial walls undergo a phenotypic modulation, characterized by a loss of contractile apparatus and remodeling of the cytoskeleton to acquire a migratory phenotype.Citation27 Before VSMC are able to migrate, however, they have to break down or invade the extracellular matrix (ECM), hence clearing a path for migration. ECM invasion is achieved by secretion of proteases, primarily metalloproteases such as MMP2, MMP9 and MT1-MMP, at discrete sites of membrane protrusions called podosomes.Citation28
Podosomes and Vascular Smooth Muscle Cell Invasion
Podosomes are dynamic, actin-based structures that act as sites of cell adhesion and active ECM remodeling.Citation28–Citation32 First discovered in monocytic cells such as macrophages, dendritic cells and osteoclasts, podosomes have since been found in many cell types including epithelial cells,Citation33,Citation34 endothelial cellsCitation35 and VSMC.Citation36 Src-transformed fibroblasts and VSMC spontaneously produce podosomes, some of which self assemble, with uncertain mechanisms, into more stable ring structures called rosettes that bear features resembling the sealing zones or belts of podosomes found in osteoclastsCitation37,Citation38 (). Invadopodia, which are podosome-related structures found in carcinoma cells,Citation39 appear in fewer numbers per cell as irregular, actin-rich dots. It remains a subject of debates whether podosomes and invadopodia represent different phases of the same structure, or unique, cell type-specific invasive organelles. Nevertheless, the discovery of podosomes and podosome-like structures in an increasingly wider spectrum of invasive cell types suggests a general requirement for these less well-understood cytoskeletal organelles in cell invasion in health and diseases. This view has received considerable attention and stimulated much interest in the study of podosomes and invadopodia in invasive cells, including tumor cells in cancers, osteoclasts in bone resorption, endothelial cells in angiogenesis and VSMC in vascular wound-healing and atherosclerosis.
A typical podosome is a finger-like vertical column arising from the ventral plasma membrane at sites of integrin clusters. The core of the podosome column (0.5 to several µm in diameter), which comprises linear and branched actin filaments, houses a squad of actin-nucleating and polymerizing proteins such as N-WASP, the Arp2/3 complex, cofilin, cortactin and caldesmon, and is surrounded by an outer ring of focal adhesion proteins such as α-actinin, vinculin, talin and paxillin. There is no known protein that exclusively localizes to podosomes. However, cortactin and its Tyr-phosphorylated counterpart always exist in podosomes, and have been widely used as podosome markers. Cortactin is a major Src-substrate that stabilizes branch points of actin filament network in lamellipodia and podosomes by binding to the Arp2/3 complex, N-WASP and F-actin.Citation40
The sequence of events leading to podosome assembly involves remodeling of focal adhesions at integrin clusters and recruitment of Src and Src-substrates such as cortactin, FAK, Tks5/FISH and N-WASP to initiate actin nucleation and polymerization at the sites of podosome biogenesis. Src induces self-assembly of podosomes into more stable bands and rosettes in VSMC. The actin cores of individual podosomes in a rosette are connected by a network of radial actin filaments, a so-called “actin cloud,” that transmits tangential forces to organize the podosome arrays in the rosette.Citation41 The transport of appropriate MMPs to podosomes and rosettes, which endows these structures with an ECM-degrading capacity, may be facilitated by molecular motors and the microtubule cytoskeletal system.Citation42
Podosomes are highly dynamic structures with a lifetime of a few minutes.Citation29,Citation31,Citation43 Elucidation of the mechanisms that regulate the assembly and disassembly of podosomes is an active area of research that has gained much momentum in recent years. Although a detailed picture is still wanting, it is clear that podosome dynamics are regulated by a network of coordinated signaling pathways involving cell migration and cytoskeleton regulators (RhoGTPases, integrins, N-WASP, Arp2/3, AFAP, caldesmon and cortactin),Citation44 lipid kinases [PtdIns(3)-kinase (PI3K)], protein kinases (PKCs, PAK, FAK),Citation33,Citation45 intracellular and secreted proteases (Calpain, MMPs),Citation46,Citation47 adaptor proteins (Tks5/FISH),Citation48 oncogenes (cSrc, Stat3)Citation48 and tumor suppressors (p53, PTEN),Citation5,Citation6 and undoubtedly others yet to be discovered.
p53 and Src Play Antagonistic Roles in Podosome Formation in Vascular Smooth Muscle Cells
We have identified p53 as a suppressor of Src-induced podosome formation and associated invasive phenotype in VSMC.Citation6 p53 acts via a two-prong mechanism: (1) by upregulating the expression of caldesmon, an actin-binding protein and a negative regulator of podosome assembly; and (2) by enhancing the expression of PTEN, thereby inhibiting the Src-mediated PI3K-Akt and Stat3 pro-invasion pathways.Citation7 In contrast, constitutively active Src (SrcY527F) suppresses endogenous p53 expression and induces podosome formation and an invasive phenotype in VSMCCitation6 by upregulating Stat3, which suppresses p53 and its target, caldesmon.Citation7 These data suggest the existence of mutually antagonistic cross-talks between the anti-invasion and pro-invasion pathways mediated by p53 and Src3, respectively, serving as a check and balance that dictates the outcome of either an invasive or non-invasive phenotypeCitation7 ().
The p53-Mediated Anti-Podosome/Invasion Pathways
p53-caldesmon.
We have demonstrated that expression of caldesmon positively correlates with the transcriptional function of p53, which in turn negatively impacts on Src-induced podosome formation.Citation6 This suggests that caldesmon may be a transcriptional target of p53 and is consistent with data obtained from the mapping of p53-binding sites in the human genome that identifies caldesmon as a potential p53 target gene.Citation11
Caldesmon is a Ca2+/calmodulin- and actin-binding protein that plays a key role in the regulation of cytoskeletal organization and cell motility of non-muscle and de-differentiated ‘synthetic’ smooth muscle cells.Citation49,Citation50 Caldesmon modulates cell contractility by inhibiting actin-activated myosin ATPase activity, and depending on its expression level and interaction with Ca2+/calmodulin, either destabilizes or stabilizes actin stress-fibers and focal adhesions.Citation51 Caldesmon was first identified in podosomes in Rous or avian sarcoma virus-transformed cells by Sobue's group in 1993.Citation52 More recently, caldesmon has been shown to be a prominent component of podosomes in VSMC and fibroblasts, and a repressor of podosome formation and cell invasion,Citation6,Citation51,Citation53 that is in part attributable to its ability to inhibit Arp2/3-mediated actin branching.Citation54 That caldesmon acts as an invasion suppressor is also consistent with findings that expression of caldesmon is repressed in a number of cancer and transformed cells.Citation52 It remains to be determined if p53 modulates caldesmon expression at the DNA level, or indirectly via microRNAs or at the protein level.
p53-PTEN.
The tumor suppressor PTEN (Phosphatase and Tensin homolog deleted on chromosome 10), which is mutated in the majority of advanced, invasive tumorsCitation55 and in vascular remodeling,Citation56 is another strong candidate for mediating p53-dependent podosome suppression. PTEN and p53 mutually enhance each other's expression and activity. Thus, PTEN has been shown to increase p53 protein levels and stability via phosphatase-dependent and -independent mechanisms.Citation57 On the other hand, we have found that enhanced p53 increases PTEN expression in VSMC and suppresses Src-induced podosome formation.Citation7 Together, these observations suggest that there is a positive feedback loop that regulates the expression and stability of p53 and PTEN.
PTEN is a phosphatase that exhibits both lipid- and protein-phosphatase activities in vitro. The lipid-phosphatase activity of PTEN has been well documented to play a dominant role in tumor suppression by negatively modulating the PI3K-Akt pathway.Citation58 Upregulation of PTEN by p53 would lead to a decrease in PI(3,4,5)P3 and PI(3,4)P2 in the plasma membrane and a reduction in podosome/rosette formation.Citation55 This is in line with the finding that PI(3,4)P2 is enriched in focal adhesions and podosomes, and is responsible for recruiting the adaptor protein, Tks5/FISH, which is essential for podosome formation, to sites of podosome biogenesis.Citation59
Recent studies using the PTEN(Y138L) and PTEN(G129E) mutants, which exhibit only lipid- or protein-phosphatase activity, respectively, have demonstrated that both activities are involved in cell motility and invasion.Citation60–Citation63 For example, Davidson et al.Citation60 have shown that both the lipid- and protein-phosphatase activities are required for PTEN to inhibit invasion into the surrounding matrix when expressed in PTEN-null glioblastoma cells, but expression of either activity alone is insufficient to block invasion. We have also shown that both the protein- and lipid-phosphatase activities of PTEN contribute to p53-mediated suppression of Src-induced podosome and rosette formation in VSMC.Citation64
A number of in vitro protein substrates of PTEN have been identified, but their physiological relevance remains to be firmly established. We have reported that shRNA-driven knockdown of PTEN increases the phosphorylation and activation of Stat3 (pY705Stat3); and that overexpression of PTEN decreases Stat3 activity, resulting in podosome suppression.Citation7 It is not clear whether PTEN has a direct role in Stat3 dephosphorylation via its protein-phosphatase activity or an indirect role via PI3K-Akt signaling. The identification of direct protein substrates of PTEN will be critical for our understanding of its role in cell migration. Taken together, these recent findings suggest that the p53-PTEN pathway inhibits cell invasion by inactivating podosome agonists via its protein-phosphatase activity on the one hand and by suppressing the pro-invasive PI3K-Akt pathway via its lipid-phosphatase activity on the other. Much work is required, however, to address the question of whether the protein- and lipid phosphatase activities of PTEN act independently or co-operatively.
The Src-Mediated Pro-Podosome/Invasion Pathways
Activation of c-Src by receptor Tyr kinases, such as PDGFR and EGFR, induces the formation of podosomes and rosettes in various cell types including VSMC, fibroblasts, endothelial cells and epithelial cells.Citation65,Citation66 Many Src-substrates, such as focal adhesion kinase (FAK), Stat3,Citation67 cortactin,Citation68 Tks5 and Tks4,Citation48,Citation69 and β-dystroglycanCitation70 are enriched in Src-induced podosomes and rosettes, and play distinct roles in the regulation of actin cytoskeleton remodeling and podosome formation. For instance, phosphorylation of cortactin by Src is involved in regulating the formation and/or turnover of podosomes in A7r5 smooth muscle cells.Citation68 Src-dependent phosphorylation of β-dystroglycan, a cell adhesion receptor, promotes its association with Tks5 and podosome formation in myoblasts.Citation70 Recently, it has been shown that in response to PDGF, Src inhibits p53 leading to a decrease in the expression of microRNAs, miR-143/145, in A7r5 VSMC lines, thereby upregulating podosome formation.Citation71
Src-Stat3.
Stat3 is a member of the Signal Transducer and Activator of Transcription family, which is activated by Jak and Src family kinases.Citation67 Stat3 is upregulated in many cancers and is implicated in the promotion of aggressive metastasis via the transactivation of MMPs.Citation72 We have shown recently that Stat3 is a required downstream effector of Src in inducing podosome formation and related invasive phenotypes. Stat3 promotes Src-induced invasive phenotypes through the suppression of p53 and the p53-caldesmon pathway. Furthermore, Stat3 and its activated form, pY705Stat3, localize to Src-induced podosomes.Citation7 This suggests that Stat3 is recruited to podosomes where it is activated by Src, and thus mediates a Src-induced invasive phenotype either through its transcriptional functions and/or transcriptional-independent activities.Citation73 Identification of downstream effectors of Stat3 that participate in the regulation of podosome dynamics will be critical to further our understanding of its role in cell invasion.
Src-PI3K-Akt.
Akt (also known as Protein Kinase B, PKB) is a Ser/Thr kinase that plays a pivotal role in signaling downstream of receptor Tyr kinases (RTKs) involved in cell growth, angiogenesis and cell migration.Citation74 PI3K is activated by either binding directly to RTKs, or indirectly through binding to Tyr-phosphorylated adaptor proteins such as IRS1. PI(3,4,5)P3 produced by PI3K recruits Akt to the plasma membrane, where PDK1 and mTOR complex 2 (mTORC2) phosphorylate Akt at T308 in the activation loop and S473 in the hydrophobic motif, respectively, resulting in Akt activation.
We have shown that activated Src upregulates Akt expression, accompanied by enhanced podosome/rosette formation and ECM digestion in VSMC.Citation7 However, how Akt promotes podosome/rosette formation and cell invasion remains unclear. This is further complicated by findings that the Akt1 and Akt2 isozymes, both of which are expressed in VSMC, often exhibit opposite roles in cell migration.Citation75 Numerous potential Akt substrates have been reported, many of which are involved in cell growth and proliferation, cell survival, metabolism and angiogenesis. However, relatively few Akt substrates have been implicated in cell migration. The sodium-hydrogen exchanger (NHE1) has been shown to be a bona fide Akt substrate necessary for growth factor-mediated remodeling of actin cytoskeleton.Citation76 Two other reports have provided evidence for a possible link between Akt-PDK1 and PAK1 (p21-Associated Kinase), a key modulator of cell migration and podosome formation in VSMC.Citation45 First, Akt phosphorylates PAK1 and modulates its binding to the adaptor protein, Nck, and cell migration.Citation77 Second, PAK1 acts as a scaffold to recruit Akt1 and PDK1 to PI(3,4,5)P3 in the plasma membrane for efficient activation of Akt.Citation78
PAK1 is a member of the PAK family of Ser/Thr kinases that are activated by Cdc42/Rac.Citation79,Citation80 Of the six PAK isoenzymes identified in mammalian tissues, PAK1, PAK2 and PAK3 are expressed in VSMC, with PAK1 being the predominant species. PAK1 may play a role in podosome formation by phosphorylating substrates such as LIM kinase,Citation81 caldesmonCitation82 and cortactin,Citation83 which are key regulators of actin polymerization in podosome dynamics. Alternatively, PAK1-induced formation of podosomes can be mediated by forming a PAK1/PIX/GIT complex, which targets PAK1 to focal adhesions, where GIT in turn binds to paxillin and initiates focal adhesion remodeling prior to podosome assembly.Citation84 PIX (PAK-Interacting exchange factor)Citation85,Citation86 is a multifunctional protein containing a number of protein interaction domains including a SH3 motif that binds to one of the Pro-rich domains of PAK1, a Pleckstrin-homology (PH) domain and a tandem Dbl homologous (DH) domain that is responsible for its role as a guanine nucleotide exchange factor (GEF) of Cdc42 and Rac, a GBD domain that binds the G protein-couple receptor kinase-interacting target (GIT).Citation87 It is quite possible that at the initiation of podosome assembly, PAK1 acts as a scaffold for Akt1 and PDK1 and targets them to PIX-GIT and PI(3,4,5)P3 at the sites of podosome biogenesis. This would facilitate the phosphorylation of PAK1 by activated Akt and invokes downstream events in podosome formation ().
Perspective and Conclusion
Cell proliferation and cell invasion are defining characteristics, not only of cancer cells, but also of VSMC in the pathogenesis of atherosclerosis and plaque formation. Accumulating data support the intriguing hypothesis that p53 plays a dual role in the regulation of cell growth, and cell migration/invasion. Much work is required to determine if and how transcriptional functions of p53 during nuclear stress may be coupled to cytoskeletal remodeling events. It is tempting to speculate that such coordination may be fulfilled by dual-functional factor(s) such as JMY that coordinates p53 nuclear functions in response to stress and cytoskeletal events in cell migration/invasion.Citation21,Citation22 Alternatively, it cannot be ruled out that p53 independently regulates the transcription of key regulators of cell cycle and cytoskeletal remodeling.
Figures and Tables
Figure 1 Src-induced formation of podosomes and rosettes in rat aortic smooth muscle cells that actively digest extracellular matrix. (A) A control rat aortic smooth muscle cell (RASMC) showing prominent actin-stress fibers stained with FITC-phalloidin. (B) RASMC line expressing constitutively active Src(Y527F) encoded in retroviral vectors. Note the disappearance of actin-stress fibers and formation of podosomes that self-assemble into rosettes. (C) Digestion of TRITC-labeled fibronectin (red) in ECM by rosettes appears as dark spots. Actin is stained with FITC-phalloidin (green). Scales bars represent 20 µm.
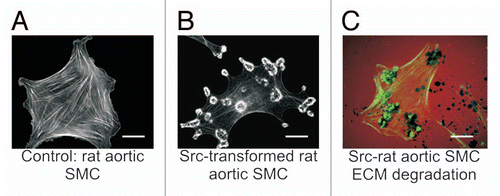
Figure 2 Proposed mechanisms for anti- and pro-podosome formation mediated by p53 and Src, respectively. p53 suppresses podosome formation in VSMC by upregulating caldesmon and PTEN. Caldesmon acts as a podosome antagonist by inhibiting Arp2/3-mediated actin polymerization. Both PTEN protein- and lipid-phosphatase activities contribute to podosome suppression by downregulating the Src-Stat3 and Src-PI3K-Akt pro-podosome and invasion pathways. Membrane receptor tyrosine kinases activate Src, which induces podosome formation and cell invasion by phosphorylating podosomal proteins such as cortactin and Tks5/FISH. Activation of PI3K by receptor tyrosine kinases or Src increases PI(3,4,5)P3, which together with GIX-PIX recruit the Akt-PAK1-PDK1 ternary complex to sites of podosome biogenesis at the plasma membrane. Activated Akt and PAK1 proceed to modulate regulatory proteins engaged in podosome dynamics. While p53 and Src mutually antagonize each other, a positive feedback loop appears to exist between p53 and PTEN.
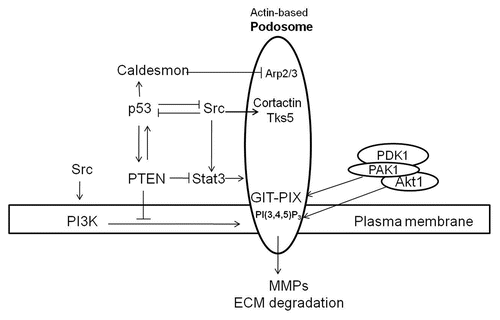
Acknowledgements
ASM is funded by the Canadian Institute of Health Research and Heart and Stroke Foundation of Ontario. Infrastructure supports provided by the Canada Foundation of Innovation (CFI)-funded Protein Function Discovery facilities are gratefully acknowledged. I thank Graham Côtí and Robert Eves for reading the manuscript.
References
- Lane DP, Crawford LV. T antigen is bound to a host protein in SV40-transformed cells. Nature 1979; 278:261 - 263
- Linzer DI, Levine AJ. Characterization of a 54 K dalton cellular SV40 tumor antigen present in SV40-transformed cells and uninfected embryonal carcinoma cells. Cell 1979; 17:43 - 52
- Efeyan A, Serrano M. p53: guardian of the genome and policeman of the oncogenes. Cell Cycle 2007; 6:1006 - 1010
- Roger L, Gadea G, Roux P. Control of cell migration: a tumour suppressor function for p53?. Biol Cell 2006; 98:141 - 152
- Mukhopadhyay UK, Mak AS. p53: is the guardian of the genome also a suppressor of cell invasion?. Cell Cycle 2009; 8:2481
- Mukhopadhyay UK, Eves R, Jia L, Mooney P, Mak AS. p53 suppresses Src-induced podosome and rosette formation and cellular invasiveness through the upregulation of caldesmon. Mol Cell Biol 2009; 29:3088 - 3098
- Mukhopadhyay UK, Mooney P, Jia L, Eves R, Raptis L, Mak AS. Doubles game: Src-Stat3 versus p53-PTEN in cellular migration and invasion. Mol Cell Biol 2010; 30:4980 - 4995
- Comer KA, Dennis PA, Armstrong L, Catino JJ, Kastan MB, Kumar CC. Human smooth muscle alpha-actin gene is a transcriptional target of the p53 tumor suppressor protein. Oncogene 1998; 16:1299 - 1308
- Mukhopadhyay D, Tsiokas L, Sukhatme VP. Wild-type p53 and v-Src exert opposing influences on human vascular endothelial growth factor gene expression. Cancer Res 1995; 55:6161 - 6165
- Sun Y, Sun Y, Wenger L, Rutter JL, Brinckerhoff CE, Cheung HS. Human metalloproteinase-1 (collagenase-1) is a tumor suppressor protein p53 target gene. Ann NY Acad Sci 1999; 878:638 - 641
- Wei CL, Wu Q, Vega VB, Chiu KP, Ng P, Zhang T, et al. A global map of p53 transcription-factor binding sites in the human genome. Cell 2006; 124:207 - 219
- Okorokov AL, Rubbi CP, Metcalfe S, Milner J. The interaction of p53 with the nuclear matrix is mediated by F-actin and modulated by DNA damage. Oncogene 2002; 21:356 - 367
- Giannakakou P, Sackett DL, Ward Y, Webster KR, Blagosklonny MV, Fojo T. p53 is associated with cellular microtubules and is transported to the nucleus by dynein. Nat Cell Biol 2000; 2:709 - 717
- Nishio K, Inoue A. Senescence-associated alterations of cytoskeleton: extraordinary production of vimentin that anchors cytoplasmic p53 in senescent human fibroblasts. Histochem Cell Biol 2005; 123:263 - 273
- Muller PA, Caswell PT, Doyle B, Iwanicki MP, Tan EH, Karim S, et al. Mutant p53 drives invasion by promoting integrin recycling. Cell 2009; 139:1327 - 1341
- Wang SP, Wang WL, Chang YL, Wu CT, Chao YC, Kao SH, et al. p53 controls cancer cell invasion by inducing the MDM2-mediated degradation of Slug. Nat Cell Biol 2009; 11:694 - 704
- Guo F, Zheng Y. Rho family GTPases cooperate with p53 deletion to promote primary mouse embryonic fibroblast cell invasion. Oncogene 2004; 23:5577 - 5585
- Gadea G, Roger L, Anguille C, de TM, Gire V, Roux P. TNFalpha induces sequential activation of Cdc42- and p38/p53-dependent pathways that antagonistically regulate filopodia formation. J Cell Sci 2004; 117:6355 - 6364
- Gadea G, Lapasset L, Gauthier-Rouviere C, Roux P. Regulation of Cdc42-mediated morphological effects: a novel function for p53. EMBO J 2002; 21:2373 - 2382
- Gadea G, De Toledo M, Anguille C, Roux P. Loss of p53 promotes RhoA-ROCK-dependent cell migration and invasion in 3D matrices. J Cell Biol 2007; 178:23 - 30
- Coutts AS, Weston L, La Thangue NB. A transcription co-factor integrates cell adhesion and motility with the p53 response. Proc Natl Acad Sci USA 2009; 106:19872 - 19877
- Zuchero JB, Coutts AS, Quinlan ME, Thangue NB, Mullins RD. p53-cofactor JMY is a multifunctional actin nucleation factor. Nat Cell Biol 2009; 11:451 - 459
- Mercer J, Bennett M. The role of p53 in atherosclerosis. Cell Cycle 2006; 5:1907 - 1909
- Guevara NV, Kim HS, Antonova EI, Chan L. The absence of p53 accelerates atherosclerosis by increasing cell proliferation in vivo. J Clin Invest 1999; 5:335 - 339
- van Vlijmen BJ, Gerritsen G, Franken AL, Boesten LS, Kockx MM, Gijbels MJ, et al. Macrophage p53 deficiency leads to enhanced atherosclerosis in APOE*3-Leiden transgenic mice. Circ Res 2001; 88:780 - 786
- Rudijanto A. The role of vascular smooth muscle cells on the pathogenesis of atherosclerosis. Acta Med Indones 2007; 39:86 - 93
- Owens GK, Kumar MS, Wamhoff BR. Molecular regulation of vascular smooth muscle cell differentiation in development and disease. Physiol Rev 2004; 84:767 - 801
- Linder S. The matrix corroded: podosomes and invadopodia in extracellular matrix degradation. Trends Cell Biol 2007; 17:107 - 117
- Yamaguchi H, Pixley F, Condeelis J. Invadopodia and podosomes in tumor invasion. Eur J Cell Biol 2006; 85:213 - 218
- Gimona M, Buccione R, Courtneidge SA, Linder S. Assembly and biological role of podosomes and invadopodia. Curr Opin Cell Biol 2008; 20:235 - 241
- Abiges-Rizo C, Destaing O, Fourcade B, Planus E, Block MR. Actin machinery and mechanosensitivity in invadopodia, podosomes and focal adhesions. J Cell Sci 2009; 122:3037 - 3049
- Block MR, Badowski C, Millon-Fremillon A, Bouvard D, Bouin A, Faurobert E, et al. Podosome-type adhesions and focal adhesions, so alike yet so different. Eur J Cell Biol 2008; 87:491 - 506
- Xiao H, Eves R, Yeh C, Kan W, Xu F, Mak A, et al. Phorbol ester-induced podosomes in normal human bronchial epithelial cells. J Cell Physiol 2009; 218:366 - 375
- Xiao H, Bai XH, Kapus A, Lu WY, Mak AS, Liu M. PKC cascade regulates recruitment of MMP-9 to podosomes and its release and activation. Mol Cell Biol 2010; 30:5545 - 5561
- Rottiers P, Saltel F, Daubon T, Chaigne-Delalande B, Tridon V, Billottet C, et al. TGFbeta-induced endothelial podosomes mediate basement membrane collagen degradation in arterial vessels. J Cell Sci 2009; 122:4311 - 4318
- Hai CM, Hahne P, Harrington EO, Gimona M. Conventional protein kinase C mediates phorboldibutyrate-induced cytoskeletal remodeling in a7r5 smooth muscle cells. Exp Cell Res 2002; 280:64 - 74
- Machesky L, Jurdic P, Hinz B. Grab, stick, pull and digest: the functional diversity of actin-associated matrix-adhesion structures. Workshop on Invadopodia, Podosomes and Focal Adhesions in Tissue Invasion. EMBO Rep 2008; 9:139 - 143
- Symons M. Cell biology: watching the first steps of podosome formation. Curr Biol 2008; 18:925 - 927
- Weaver AM. Invadopodia: specialized cell Structures for cancer invasion. Clin Exp Metastasis 2006; 23:97 - 105
- Weaver AM, Heuser JE, Karginov AV, Lee WL, Parsons JT, Cooper JA. Interaction of cortactin and N-WASp with Arp2/3 complex. Curr Biol 2002; 12:1270 - 1278
- Luxenburg C, Geblinger D, Klein E, Anderson K, Hanein D, Geiger B, et al. The architecture of the adhesive apparatus of cultured osteoclasts: from podosome formation to sealing zone assembly. PLoS ONE 2007; 2:179
- Linder S, Hufner K, Wintergerst U, Aepfelbacher M. Microtubule-dependent formation of podosomal adhesion structures in primary human macrophages. J Cell Sci 2000; 113:4165 - 4176
- Wolfenson H, Henis YI, Geiger B, Bershadsky AD. The heel and toe of the cell's foot: a multifaceted approach for understanding the structure and dynamics of focal adhesions. Cell Motil Cytoskeleton 2009; 66:1017 - 1029
- Dovas A, Gevrey JC, Grossi A, Park H, bou-Kheir W, Cox D. Regulation of podosome dynamics by WASp phosphorylation: implication in matrix degradation and chemotaxis in macrophages. J Cell Sci 2009; 122:3873 - 3882
- Webb BA, Eves R, Crawley SW, Zhou S, Cote GP, Mak AS. PAK1 induces podosome formation in A7r5 vascular smooth muscle cells in a PAK-interacting exchange factor-dependent manner. Am J Physiol Cell Physiol 2005; 289:898 - 907
- Wells A, Huttenlocher A, Lauffenburger DA. Calpain proteases in cell adhesion and motility. Int Rev Cytol 2005; 245:1 - 16
- Kuzuya M, Iguchi A. Role of matrix metalloproteinases in vascular remodeling. J Atheroscler Thromb 2003; 10:275 - 282
- Courtneidge SA, Azucena EF, Pass I, Seals DF, Tesfay L. The SRC substrate Tks5, podosomes (invadopodia) and cancer cell invasion. Cold Spring Harb Symp Quant Biol 2005; 70:167 - 171
- Sobue K, Muramoto Y, Fujita M, Kakiuchi S. Purification of a calmodulin-binding protein from chicken gizzard that interacts with F-actin. Proc Natl Acad Sci USA 1981; 78:5652 - 5655
- Lin JJ, Li Y, Eppinga RD, Wang Q, Jin JP. Chapter 1: roles of caldesmon in cell motility and actin cytoskeleton remodeling. Int Rev Cell Mol Biol 2009; 274:1 - 68
- Eves R, Webb BA, Zhou S, Mak AS. Caldesmon is an integral component of podosomes in smooth muscle cells. J Cell Sci 2006; 119:1691 - 1702
- Tanaka J, Watanabe T, Nakamura N, Sobue K. Morphological and biochemical analyses of contractile proteins (actin, myosin, caldesmon and tropomyosin) in normal and transformed cells. J Cell Sci 1993; 104:595 - 606
- Morita T, Mayanagi T, Yoshio T, Sobue K. Changes in the balance between caldesmon regulated by p21-activated kinases and the Arp2/3 complex govern podosome formation. J Biol Chem 2007; 282:8454 - 8463
- Yamakita Y, Oosawa F, Yamashiro S, Matsumura F. Caldesmon inhibits Arp2/3-mediated actin nucleation. J Biol Chem 2003; 278:17937 - 17944
- Jiang BH, Liu LZ. PI3K/PTEN signaling in angiogenesis and tumorigenesis. Adv Cancer Res 2009; 102:19 - 65
- Nemenoff RA, Simpson PA, Furgeson SB, Kaplan-Albuquerque N, Crossno J, Garl PJ, et al. Targeted deletion of PTEN in smooth muscle cells results in vascular remodeling and recruitment of progenitor cells through induction of stromal cell-derived factor-1alpha. Circ Res 2008; 102:1036 - 1045
- Freeman DJ, Li AG, Wei G, Li HH, Kertesz N, Lesche R, et al. PTEN tumor suppressor regulates p53 protein levels and activity through phosphatase-dependent and -independent mechanisms. Cancer Cell 2003; 3:117 - 130
- Chalhoub N, Baker SJ. PTEN and the PI3-kinase pathway in cancer. Annu Rev Pathol 2009; 4:127 - 150
- Oikawa T, Itoh T, Takenawa T. Sequential signals toward podosome formation in NIH-src cells. J Cell Biol 2008; 182:157 - 169
- Davidson L, Maccario H, Perera NM, Yang X, Spinelli L, Tibarewall P, et al. Suppression of cellular proliferation and invasion by the concerted lipid and protein phosphatase activities of PTEN. Oncogene 2010; 29:687 - 697
- Dey N, Crosswell HE, De P, Parsons R, Peng Q, Su D, et al. The protein phosphatase activity of PTEN regulates SRC family kinases and controls glioma migration. Cancer Res 2008; 68:1862 - 1871
- Leslie NR, Yang X, Downes CP, Weijer CJ. PtdIns(3,4,5)P(3)-dependent and -independent roles for PTEN in the control of cell migration. Curr Biol 2007; 17:115 - 125
- Leslie NR, Maccario H, Spinelli L, Davidson L. The significance of PTEN's protein phosphatase activity. Adv Enzyme Regul 2009; 49:190 - 196
- Poon JS, Eves R, Mak AS. Both lipid- and protein-phosphatase activities of PTEN contribute to the p53-PTEN anti-invasion pathway. Cell Cycle 2010; 9:4450 - 4454
- Bharti S, Inoue H, Bharti K, Hirsch DS, Nie Z, Yoon HY, et al. Src-dependent phosphorylation of ASAP1 regulates podosomes. Mol Cell Biol 2007; 27:8271 - 8283
- Destaing O, Sanjay A, Itzstein C, Home WC, Toomre D, Camilli PD, et al. The tyrosine kinase activity of c-Src regulates actin dynamics and organization of podosomes in osteoclasts. Mol Biol Cell 2008; 19:394 - 404
- Silva CM. Role of STATs as downstream signal transducers in Src family kinase-mediated tumorigenesis. Oncogene 2004; 23:8017 - 8023
- Zhou S, Webb BA, Eves R, Mak AS. Effects of tyrosine phosphorylation of cortactin on podosome formation in A7r5 vascular smooth muscle cells. Am J Physiol Cell Physiol 2006; 290:463 - 471
- Buschman MD, Bromann PA, Cejudo-Martin P, Wen F, Pass I, Courtneidge SA. The novel adaptor protein Tks4 (SH3PXD2B) is required for functional podosome formation. Mol Biol Cell 2009; 20:1302 - 1311
- Thompson O, Kleino I, Crimaldi L, Gimona M, Saksela K, Winder SJ. Dystroglycan, Tks5 and Src mediated assembly of podosomes in myoblasts. PLoS ONE 2008; 3:3638
- Quintavalle M, Elia L, Condorelli G, Courtneidge SA. MicroRNA control of podosome formation in vascular smooth muscle cells in vivo and in vitro. J Cell Biol 2010; 189:13 - 22
- Gao SP, Bromberg JF. Touched and moved by STAT3. Sci STKE 2006; 2006:30
- Ng DC, Lin BH, Lim CP, Huang G, Zhang T, Poli V, et al. Stat3 regulates microtubules by antagonizing the depolymerization activity of stathmin. J Cell Biol 2006; 172:245 - 257
- Manning BD, Cantley LC. AKT/PKB signaling: navigating downstream. Cell 2007; 129:1261 - 1274
- McKenna LB, Zhou GL, Field J. Isoform-specific functions of Akt in cell motility. Cell Mol Life Sci 2007; 64:2723 - 2725
- Meima ME, Webb BA, Witkowska HE, Barber DL. The sodium-hydrogen exchanger NHE1 is an Akt substrate necessary for actin filament reorganization by growth factors. J Biol Chem 2009; 284:26666 - 26675
- Zhou GL, Zhuo Y, King CC, Fryer BH, Bokoch GM, Field J. Akt phosphorylation of serine 21 on Pak1 modulates Nck binding and cell migration. Mol Cell Biol 2003; 23:8058 - 8069
- Higuchi M, Onishi K, Kikuchi C, Gotoh Y. Scaffolding function of PAK in the PDK1-Akt pathway. Nat Cell Biol 2008; 10:1356 - 1364
- Bokoch GM. Biology of the p21-Activated Kinases. Annu Rev Biochem 2003; 72:743 - 781
- Zhao ZS, Manser E. PAK and other Rho-associated kinases: effectors with surprisingly diverse mechanisms of regulation. Biochem J 2005; 386:201 - 214
- Song X, Chen X, Yamaguchi H, Mouneimne G, Condeelis JS, Eddy RJ. Initiation of cofilin activity in response to EGF is uncoupled from cofilin phosphorylation and dephosphorylation in carcinoma cells. J Cell Sci 2006; 119:2871 - 2881
- Foster DB, Shen LH, Kelly J, Thibault P, Van Eyk JE, Mak AS. Phosphorylation of Caldesmon by p21-activated Kinase. Implications for the Ca(2+) sensitivity of smooth muscle contraction. J Biol Chem 2000; 275:1959 - 1965
- Webb BA, Zhou S, Eves R, Shen L, Jia L, Mak AS. Phosphorylation of cortactin by p21-activated kinase. Arch Biochem Biophys 2006; 456:183 - 193
- Zhao ZS, Manser E, Loo TH, Lim L. Coupling of PAK-interacting exchange factor PIX to GIT1 promotes focal complex disassembly. Mol Cell Biol 2000; 20:6354 - 6363
- Manser E, Loo TH, Koh CG, Zhao ZS, Chen XQ, Tan L, et al. PAK kinases are directly coupled to the PIX family of nucleotide exchange factors. Mol Cell 1998; 1:183 - 192
- Mott HR, Nietlispach D, Evetts KA, Owen D. Structural Analysis of the SH3 Domain of beta-PIX and Its Interaction with alpha-p21 Activated Kinase (PAK). Biochemistry 2005; 44:10977 - 10983
- Hoefen RJ, Berk BC. The multifunctional GIT family of proteins. J Cell Sci 2006; 119:1469 - 1475