Abstract
Filamin A (FLNa), the first non-muscle actin filament cross-linking protein, was identified in 1975. Thirty five years of FLNa research has revealed its structure in great detail, discovered its isoforms (FLNb and c), and identified over 90 binding partners including channels, receptors, intracellular signaling molecules, and even transcription factors. Due to this diversity, mutations in human FLN genes result in a wide range of anomalies with moderate to lethal consequences. This review focuses on the structure and functions of FLNa in cell migration and adhesion.
Introduction
Filamin A (FLNa) is the first actin filament cross-linking protein or gelation factor identified in non-muscle cells,Citation1 and we now understand its structure in great detail. FLN is part of a family of three proteins (FLNb and c) that are products of distinct genes and are known to serve as scaffolds for over 90 binding partners including channels, receptors, intracellular signaling molecules, and even transcription factors ( summarizes the partners involved in cell adhesion and migration and Sup. Table 1 presents the other partners). Because of this extensive array of associated proteins, mutations in human FLN genes result in a wide range of cell and tissue anomalies.Citation2,Citation3 In this review, we focus on the structure and functions of FLNa in cell migration and adhesion. Other functions of FLNs are described in more detail in several recent review articles.Citation4–Citation6
Structure of FLNa
FLNa consists of two 280 kDa subunits that self-associate to form a 160 nm long semi-flexible strand (). Each FLN subunit has an N-terminal spectrin-related actin-binding domain (srABD) followed by 24 repeat β-pleated sheet units. Two intervening calpain-sensitive “hinges” separate the repeats into rod 1 (repeats 1–15), rod 2 (repeats 16–23) and the self-association domain (repeat 24).Citation4,Citation7 FLN repeats are Ig-like (IgFLN),Citation8 each a β-barrel structure assembled from seven runs of (A∼G) β-strands.Citation9,Citation10 The most C-terminal repeat, IgFLNa24, mediates dimerization and bestows a V-shape to the dimeric moleculesCitation11 that results in the perpendicular branching of F-actinCitation12 ( and left part). A secondary F-actin-binding domain of lower affinity resides in the rod-1 segment of FLNa. Rod 2, on the other hand, does not interact with F-actin, leaving it free to associate with partner proteins, and most partner interactions occur within the rod 2 domain.Citation12 Binding and positioning of multiple partners in close proximity on rod 2 facilitates signal transduction at FLNa-enriched sites in cells.
Detailed studies of purified FLNa and its subfragments in the electron microscope have shown rod 2 to have a folded structure ().Citation12 Rod 1 is an extended chain. Its 58 nm contour length, encompassing Ig repeats 1–15, corresponds to the predicted end-to-end lengths of 15 IgFLNa repeats (3.5 nm each from the N-terminal to the C-terminal). However, the eight Ig repeats in the rod 2 domain (repeats 16–23) form a structure that is far more compact (19 nm) than those of rod 1 segments containing equivalent numbers of FLNa Ig repeats (). This compact structure is generated when the even-numbered repeats 16, 18 and 20 pair with neighboring repeats 17, 19 and 21, respectively ().Citation13,Citation14 For example, IgFLNa16 and 17 interact through their B-G and A-G faces, respectively, while strand A of IgFLNa16 protrudes from its normal position in the Ig domain and elongates hinge-1. Strands A of IgFLNa18 and 20 are also excluded from their normal positions and interact with the CD faces of neighboring IgFLNa19 and 21, respectively. As a result, the overall configuration of repeats in rod 2 is nonlinear providing rod 2 with a more globular structure. The exact configuration of paired repeats relative to each other in the rod 2 domain remains to be solved at the atomic level.
Structure of the FLNa-Partner Complex
The atomic structures of the binding interfaces between FLNa and GPIbα (a subunit of the GPIb complex or von Willebrand factor receptor), FLNa and the β integrin cytoplasmic tail or FilGAP and FLNa reveal that the C and D β-strands of the interacting IgFLNa repeats form a binding pocket for an opposing β-strand, donated by the binding partner ().Citation15,Citation16 In similar fashion, the FLNa binding sites in migfilin, CFTR (cystic fibrosis transmembrane conductance regulator), and pro-prion protein are related to those of GPIbα, β integrins and FilGAP.Citation17–Citation22 Each binding interface is a β-strand run of nine residues that can correctively position itself in the CD repeat groove of a FLNa repeat (). Alternating residues of these β-strands, thus, either face or look away from the groove. Those facing the groove are restricted to hydrophobic amino acids, which suggests primarily a hydrophobic interaction.
A single binding event between FLNa and a given binding partner, however, fails to account for the measured binding specificity or strength of the native proteins' interaction. For example, a GPIbα peptide containing all the FLNa binding sequence binds to not one but seven FLNa repeats (IgFLNa 4, 9, 12, 17, 19, 21 and 23) that are structurally similar, although point mutations in IgFLNa17 in full-length FLNa are sufficient to disrupt all binding to the GPIb complex when it is expressed in CHO cells.Citation15,Citation23 The same holds true for the FLNa-binding site of FilGAP which is similar to that of other binding partners, and potentially interacts with other repeats, although FilGAP binds only to IgFLNa23 in cells.Citation19 Thus, there are structural features in the intact FLNa molecule or in the partner molecule that limit this promiscuous binding. Establishment of high affinity interactions between FLNa and partners requires binding between two or more binding sites, i.e., one on each FLNa subunit and two on the partner protein, as the binding affinities, determined with peptides encompassing the binding strands of GPIbα, β integrins or FilGAP, for an individual FLNa Ig repeat are weak.Citation15,Citation19 Taken together, the quaternary structure made by FLN bound to its partner protein, therefore, defines the overall binding specificity and strength. Thus, bivalent reagents should be considered when designing inhibitors for partner binding sites.
Since the CD faces of repeats 19 and 21 that are used to bind the integrin cytoplasmic tail or other partner proteins are occupied with strands A of their precedent repeats (), binding of partners within these cryptic sites may require conformational changes in the rod 2 domain. The CD faces of repeats 17 and 23, on the other hand, which interact with the GPIb complex and FilGAP, respectively, are not masked. Partner binding to and between the two FLNa subunits may also crosslink the FLNa molecule and stabilize its structure.Citation15,Citation19
Regulation of FLNa-Partner Interactions
FLNa-partner interactions are regulated by: (1) mechanical forces, (2) phosphorylation, (3) proteolysis, (4) competition between binding partners and/or (5) multimerization of partners.
Mechanical force-induced conformational changes.
Insight into the molecular structure of FLNa, its abundant C-terminal binding and interacting partners, and its mode of binding F-actin into orthogonal branches, all suggest that it is ideally suited for mechanical signal transduction. Deformation of FLNa's C-terminal, transduced through its actin filament connections, could alter interactions between neighboring repeat pairs (),Citation13,Citation19 and computational simulation analyses suggest that physiologically relevant forces are sufficient to expose the cryptic integrin binding site.Citation24 In vivo, FLNa is necessary for mechanosensing behavior of cells and tissue generation. FLNa-null cells are more susceptible to force-induced apoptosis,Citation25 and FLNa is required for ductal morphogenesis of breast epithelial cells induced by a mechanically compliant collagen matrix.Citation26 FLNa is also critical for stretch activation of ion channels regulated by polycystin-1/-2 whose genes are mutated in autosomal-dominant polycystic kidney disease, the most frequent monogenic cause of kidney failure.Citation27
Phosphorylation.
FLNa is phosphorylated at multiple sites by several protein kinases ( and Sup. Table 1).Citation28–Citation32 A heterodimer of Cyclin B1/Cdk1 has been reported to phosphorylate FLNa in vitro and thereby reduce its ability to gel actin.Citation33 Calpain-mediated cleavage of FLNa in the hinge regions disconnects rod 2 from actin filaments and monomerizes it. Phosphorylation of FLNa by cAMP-dependent protein kinase has been reported to stabilize it against proteolysis.Citation28,Citation34 Many protein kinases phosphorylate FLNa at Ser2152, which is located near the A-strand of repeat 20.Citation29,Citation31,Citation35 This phosphorylation site is believed to regulate integrin binding to FLNa, but neither a phospho-mimicking FLNa mutant S2152D or a non-phospho S2152A mimic has been found to effect integrin binding.Citation36 Consistent with these negative results, a molecular dynamics simulation suggested that phosphorylation at Ser2152 does not impact the “opening” of the cryptic binding site of repeat 21 by mechanical force.Citation24 In contrast, a recent computer simulation concluded that this phosphorylation event might facilitate force-induced dissociation of auto inhibition by decreasing the force requirement.Citation37
Phosphorylation of partners' binding sites can also regulate their interaction with FLN. Phosphorylation of the integrin β2 tail on Thr758 dissociates it from FLNa and promotes binding of 14-3-3 and talin to the integrin,Citation38 thereby maintaining it in an active state.
Proteolysis.
Cleavage within the two hinges of FLNa by calpain generates rod1, rod2 and self-association domain (repeat 24) subfragments.Citation8 Following proteolysis, C-terminal-derived FLNa fragments (IgFLNa16-23 or 16-24) translocate to the nucleus together with FLNa-binding transcription factors such as androgen receptor and FOXC1.Citation39–Citation41 Catastrophic proteolysis of FLN is mediated by an ankyrin repeat-containing protein with a suppressor of cytokine signaling box 2 (ASB2), which targets FLNs for proteosomal degradation at discrete stages of development and differentiation in cells.Citation42,Citation43 Proteolysis of FLN by calpain or ASB2 inhibits cell spreading, but not migration, presumably by altering the dynamics of focal adhesions.Citation42–Citation46
Competition with other molecules.
All FLN isotypes interact with β integrin cytoplasmic tails albeit with different affinities, which can be predicted by their atomic structures.Citation16,Citation47–Citation49 The FLN-binding site on integrin overlaps the site used by other integrin-binding proteins such as talin, kindlins, β3 endonexin, ICAP1, 14-3-3, CD98 and Shc.Citation50 Conversely, certain FLN-binding partners such as migfilin compete with the integrin for repeat 21 binding ( and Sup. Table S1). Therefore, partner competition for FLN or for the integrin tail regulate focal adhesion complexesCitation16,Citation23,Citation38 making the expression profile and concentration of each reactant critical in understanding focal adhesion turnover.
Oligomerization and/or clustering of partners.
While the topology of the binding groove in the FLNa repeat and that on opposing partner β-strand site determines the binding kinetics for a given site, high avidity binding requires engagement of two or more sites as discussed above: binding interactions with both FLNa subunits greatly increase binding avidities by ∼100 fold. For example, the kapps for the binding of polypeptide mimics of the GPIbα and FilGAP binding to IgFLNa17 and IgFLN23, respectively, are over 10 µM.Citation15,Citation19,Citation51 However, the binding affinity of the von Willebrand receptor complex for FLNa or FilGAP to FLNa are estimated for other experiments to be at 100 nM and ∼200 nM.Citation19,Citation51 In similar fashion, integrins clustered at focal adhesion sites may generate much higher avidities for FLNa.Citation18 Partner oligomerization through clustering, therefore, can potentially regulate the interaction with FLNa.Citation52
Filamin Mutations and Diseases
FLNa, encoded on the X chromosome,Citation8 is the most abundant and widely distributed member of the filamin family proteins. FLNb, a nonmuscle filamin, is encoded on human chromosome 3.Citation53–Citation55 The FLNc gene resides on chromosome 7, and is expressed primarily in adult cardiac, smooth and striated muscle tissues.Citation56,Citation57 FLN isoforms have both common and distinctive binding partners and features in their structures, expression level and localization.Citation3 Mutations and deletions in the FLN genes that prevent normal FLN protein expression result in wide range of congenital anomalies.Citation3,Citation58,Citation59 FLN mutations are also common in human breast and colon cancers.Citation60 Nuclear FLNa fragments produced by proteolysis are significantly more abundant in benign prostate than metastatic prostate cancers.Citation41 Additionally, FLNa mutations have been identified as the cause of the most common genetic heart valvular disorder, familiar cardiac valvular dystrophy.Citation61 In mice, complete loss of Flna expression causes embryonic lethality with severe defects in cardiovascular formation and bone development.Citation62–Citation64 Mutations or depletions of FLN orthologs in other organisms, such as Dictyostelium,Citation65,Citation66 Drosophila and C. elegans,Citation67 also result in developmental defects. Since numerous binding partners interact with FLNs ( and Sup. Table S1), the pathological mechanisms of the diseases are most likely attributed to loss of partner binding or aberrant interactions caused by mutations. However, the affect of most of these mutations on partner interactions remains to be elucidated at the molecular level.Citation19,Citation21,Citation68
Role of FLNa in Cell Mechanics
Cell adhesion and migration inevitably rely on active and reversible changes in the mechanical properties of cells. Leukocytes, for example, need to generate internal force to crawl through connective tissues to hunt and ingest pathogens. Cells also respond to external mechanical forces imposed by their environment. Vascular cells undergo morphological changes in response to alterations in the fluid and mechanical shear stresses that are imparted on their apical surface by blood flow and their basal-lateral surfaces by pulsatile vascular stetch and retraction, and these responses have profound implications in the physiological function of blood vessels, and can lead to disorders such as atherosclerosis and thrombosis.Citation69 In addition, depending on the stiffness of substrates, stem cells differentiate into distinct cells.Citation70 Therefore, understanding of cell mechanics is essential for elucidating many of the fundamental aspects of cell behavior from motility to differentiation and development.
Unlike most conventional materials, cells behave in a highly nonlinear fashion (strain vs. stress) as strain increases. When a small force is applied on a short time scale, cells deform linearly and reversibly in proportion to the applied force. However, as the strain increases, cells deform less, a process called “strain-stiffening,” which extends the range of forces a cell can endure before undergoing mechanical collapse. When a relatively small continuous stress is applied on longer time scale, cells respond by deforming slowly and irreversibly. These behaviors demonstrate that cells have both elastic and viscous characteristics, and behaves as nonlinear viscoelastic materials.
The mechanical properties of cells are generated by the combined interactions of the cytoskeletal elements. One of the polymer systems, filamentous actin, concentrates underneath the plasma membrane and is necessary for both cell motility and the maintenance of cell shape. Purified F-actin forms entangled networks in solution that deform linearly. Hence, additional cohesive proteins are required to stiffen the networks to reconstitute cell like properties.Citation71 ABPs that can cross-link actin filaments account for this discrepancy.Citation72 FLNa-actin networks reconstitute many aspects of cell mechanics. They behave as weak elastic solids under low shear stress due to the flexible nature of actin-FLNa crosslinks, yet can support large shear stresses and have pronounced nonlinear strain-stiffening behaviors.Citation73–Citation75 These mechanical properties are attributed to FLNa's unique structure and how it interacts with F-actin to form orthogonal branches. High avidity binding to F-actin due to dimerization and multiple binding to F-actin through FLNa ABD and rod 1 confers strain-stiffening on actin networks.Citation12 The hinges account for the flexibility of FLNa molecule.Citation73 Orthogonal branching is the most efficient way to form the largest volume of actin gel with a minimal of material to support cellular integrity.
Effect of Genetic Loss of Filamin on Cell Migration and Development
Cultured FLNa-deficient melanoma cells fail to polarize and move because they have highly unstable surfaces that continuously expand and contract circumferential blebs.Citation76,Citation77,Citation89 Restoring normal levels of FLNa in these deficient cells rescues motility. In humans, null mutations in the FLNa gene disrupt long-range directed neuronal migration within the cerebral cortex in X-linked periventricular heterotopia.Citation78 Overexpression of Flna can also prevent migration,Citation79 presumably by sequestering signaling molecules from their normal position and by altering F-actin and focal adhesion turnover to enhance adhesion. Flna knock-out mice, however, do not develop periventricular heterotopia, and embryonic fibroblasts isolated from these animals do not bleb or have defects in migration and growth.Citation62,Citation63 Since FLNb is also ubiquitously expressed in these cells, FLNc is expressed in some nonmuscle cells during developmentCitation63 and many FLNa-binding partners also interact with these isoforms ( and Sup. Table 1), they could compensate for FLNa deficiency. In fact, expression of an shRNA-resistant FLNa in FLNab double knockdown cells completely rescues their spreading defect.Citation44 However, even some FLNa knockdown cells migrate at speeds comparable to wild-type cells once they overcome their diminished ability to spread on substrates and become attached.Citation44 Whether this is due to the residual expression of other FLN isotypes, or if there is a FLN-independent migration mode, remains to be elucidated. Additionally, developmental differences between humans and mice should also be considered. The mouse brain is small and less complex than its human counterpart. Neuronal migration in humans may also require different cues. However, Flna-null mice do exhibit a thinning of the cerebral cortex that may indicate a migration defect.Citation63
Cooperation of Filamin with its Partners in Cell Adhesion and Migration
FLN-binding partners interactions regulate cell adhesion, spreading and migration ( and ). The molecular mechanism by which FLN coordinates these partners is complex. Directed migration requires temporal polarization and spatial enrichment of molecules at specific sites. How does FLN, particularly when bound to actin that is dispersed through cells, perform this task? Immunofluorescent microscopy reveals FLNa and b to be enriched at the cell periphery and in the focal adhesions of cultured cells, while FLNc localizes in the muscle Z-disc.Citation56,Citation80,Citation81 One mechanism for the enrichment of FLNs at these sites is their recruitment by binding partners, such as receptors and adhesion molecules that preferentially reside at these sites ().Citation82 A second mechanism concentrating FLNa in newly assembled actin networks in lamellipodia occurs because of higher avidity for the branched F-actin junctions in these regions ().Citation12,Citation83 FLNa recruitment by one partner could allow it to scaffold additional signaling molecules and enhance the efficiency of signal transduction. In addition, while FLN-binding partners may potentially collect FLN molecules to specific locations within the cell, their function may require the conscription of additional partners by FLNa. It is unlikely that all FLN-binding partners are expressed by a given cell, as partner expression levels are tightly regulated in different cells and/or different developmental stages ( and Sup. Table 1). Therefore, the phenotype of FLN mutations must be studied within different cell types and/or developmental stages.
Role of Filamin in Integrin-Dependent Cell Adhesion and Migration
Although certain cells can migrate without integrins or swim without attaching to a substrate,Citation84,Citation85 most cells migrate in an integrin-dependent manner. Our current understanding of integrin function at focal adhesions in moving cells is summarized in . Since depletion of FLN results in integrin activation, FLN appears to act primarily as a negative regulator of integrin activation.Citation16 Therefore, any event that alters the FLN-integrin binding affects integrin activation status.Citation42,Citation86 Consistent with this notion, diminished expression of FLNa increases the invasiveness of human breast cancer cells.Citation87 Since FLNa silencing also induces activation of calpain, which leads to degradation of focal adhesion proteins,Citation88,Citation89 suppression of FLNa expression may facilitate focal adhesion turnover, thereby promoting the invasion. Recycling of integrin is also essential for sustained cell migration. The trafficking of β1 integrins to the cell membrane is regulated by PKCε-mediated phosphorylation of vimentin bound on the N-terminal of FLNa, as vimentin phosphorylation is impaired in FLNa knockdown cells.Citation90 Taken together, FLN regulates not only integrin activation, but also its recycling to coordinate integrin-dependent migration.
Despite increasing evidence supporting a FLN's suppressor function for integrin activation, degradation of FLN is not likely to be the only mechanism to affect cell migration, and it is not surprising that an opposite effect of FLNa expression on cell migration has been reported. This result indicates that the role of FLN in cell migration is cell type-dependent and that balanced FLNa/integrin interactions generate normal cell adhesion and migration.
Does FLNa Regulate Blebbing Migration?
Blebbing has recently received renewed attention in motility, as cancer cells can switch between mesenchymal (elongated) and amoeboid (blebbing or rounded) migration modes in three-dimensional environments.Citation91,Citation92 Since amoeboid cells can squeeze into the extracellular matrix, these cells escape and metastasize. Elucidating blebbing mechanisms and the conversion between migration modes is therefore of great importance.
Blebbing is believed to initiate following local disruption of plasma membrane-F-actin interactions and occurs because cells are under internal hydrostatic pressure powered by myosin contraction, as blebbistatin, a myosin II inhibitor, quenches most cellular blebbing. Blebbing is a prominent feature of FLNa-deficient melanoma cellsCitation93 that have weak cortical actin networks. In normal cells, both Ca2+ activated-gelsolin and Ca2+-calmodulin signaling can reduce stiffness of an FLNa-actin gel.Citation94,Citation95 The blebbing mode is also regulated by the RhoA-ROCK pathway that induces myosin contraction and antagonizes the Rac1-WAVE pathway, which induces actin polymerization-dependent protrusions (mesenchymal migration mode). Suppression of Rac1 activity is catalyzed by ARHGAP22 whose activity is regulated by actinomyosin contractility through an unknown mechanism, rather than by direct phosphorylation by ROCK.Citation91 Given that overexpression of FilGAP (ARHGAP24), a Rac-specific GAP that interacts with FLNa, induces blebbing,Citation96 and that the FLNa-FilGAP interaction is potentially regulated by mechanical force,Citation19,Citation97 force-induced regulation of the FLNa-FilGAP interaction could provide a simple mechanism for the conversion between mesenchymal and amoeboid migration modes.
Conclusion and Outlook
Since its discovery in 1975, studies on FLN have substantially advanced an understanding of its structure and functions. Nevertheless, important questions still remain: (1) How many of the FLN-partner interactions are regulated? (2) What is the quaternary structure formed between FLN and partners? and (3) What are the biochemical mechanisms of disease caused by FLN mutations? Disease-causing mutations distribute throughout the FLN molecule, but why do only a small number of binding partners interact in FLN's N-terminal rod 1 domain, although repeat 10 is a mutational hot spot?Citation3
The schematic FLNa model depicted in is consistent with all currently reported data. However, a more detailed structural analysis of FLNs, particularly complexed with partners, is required to understand how FLN mutations cause disease. Structural information will enable investigators to generate point mutations in FLNs or their partner proteins that lack only partner-related specific activity. Probing cell function with these molecules in vivo will provide deeper understanding of the dynamics of FLN-partner interaction during migration.
Abbreviations
FLNa | = | filamin A |
IgFLN | = | immunoglobulin-like filamin repeat |
ABP | = | actin-binding protein |
ABD | = | actin-binding domain |
GAP | = | GTPase-activating protein |
Figures and Tables
Figure 1 A schematic structure of FLNa molecule and the F-actin crosslink. This model was generated on PyMOL (www.pymol.org) by assembling Ig domains uploaded on protein data bank and by fitting them within rotary shadowed images of FLNa molecules. Structures were modeled using the Swiss model database (http://swissmodel.expasy.org). Rotary shadowed images of full-length FLNa and its subfragments are adopted from reference Citation12. The atomic structure of IgFLNa24 was generated on PyMOL (PDB accession number: 3CNK).
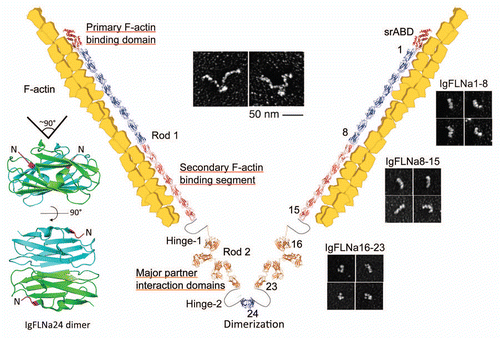
Figure 2 Atomic structures of FLNa rod 2 subdomains and the binding interfaces of known FLNa-partner complexes. The models were generated on PyMOL (PDB accession number: IgFLNa16–17, 2K7P; IgFLNa20–21, 2J3S; IgFLNa18–19, 2K7Q; IgFLNa17-GPIbβ, 2BP3; IgFLNa21-integrinβ7, 2BRQ). The CD faces of repeats and strand A are indicated with blue and red, respectively.
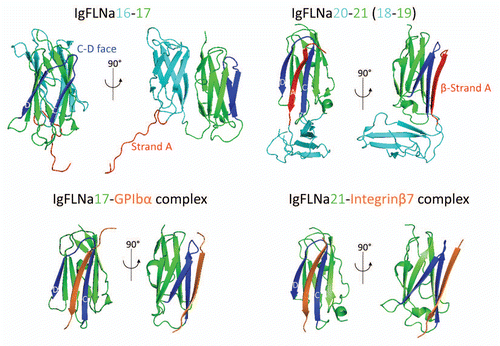
Figure 3 Model for how mechanical force regulates FLNa-partner interactions. Mechanical force changes the conformation of the rod 2 domain to release partner A by changing the geometry between the two FLNa subunits, while exposing the cryptic binding for partner B by unfolding the A strand interaction between repeats 20 and 21.
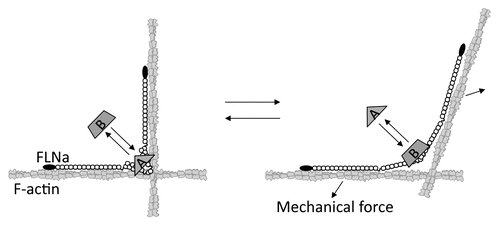
Figure 4 Schematic of how certain FLN-partner complexes regulate cell adhesion and motility. Top part: FLN crosslinks actin filaments and attaches them to signaling molecules, membrane proteins and the extracellular matrix through adhesion molecules such as integrins. FLN is required for the recycling, trafficking and stabilization of membrane proteins. FLN also scaffolds multiple partners in close proximity on rod 2, thereby facilitating signal transduction at specific locations within cells. Note that the expression levels of FLN-binding partners are dependent on the cell type and, therefore not all partners necessarily participate in cell adhesion and migration in the same cell. Bottom part: Model for how FLN regulates integrin activation. FLN acts as a negative regulator for integrin activation by blocking talin binding to the β integrin tail. Perturbation of this interaction allows talin to interact with β integrin, thereby activating integrin at the leading edge of a migrating cell.
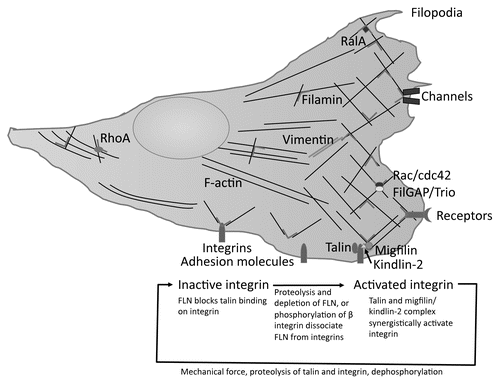
Table 1 Filamin binding partners involved in cell adhesion, spreading and migration
Additional material
Download Zip (137.7 KB)Acknowledgements
This work was supported by by National Institutes of Health Grants HL-19429 (T.P.S.) and HL-56252 (J.H.H.), the HUSEC Seed Fund for Interdisciplinary Science (T.P.S. and F.N.).
References
- Hartwig JH, Stossel TP. Isolation and properties of actin, myosin and a new actinbinding protein in rabbit alveolar macrophages. J Biol Chem 1975; 250:5696 - 5705
- Feng Y, Walsh CA. The many faces of filamin: a versatile molecular scaffold for cell motility and signalling. Nat Cell Biol 2004; 6:1034 - 1038
- Robertson SP, Filamin A. phenotypic diversity. Curr Opin Genet Dev 2005; 15:301 - 307
- Stossel TP, Condeelis J, Cooley L, Hartwig JH, Noegel A, Schleicher M, et al. Filamins as integrators of cell mechanics and signalling. Nat Rev Mol Cell Biol 2001; 2:138 - 145
- Popowicz GM, Schleicher M, Noegel AA, Holak TA. Filamins: promiscuous organizers of the cytoskeleton. Trends Biochem Sci 2006; 31:411 - 419
- Zhou AX, Hartwig JH, Akyurek LM. Filamins in cell signaling, transcription and organ development. Trends Cell Biol 2010; 20:113 - 123
- van der Flier A, Sonnenberg A. Structural and functional aspects of filamins. Biochim Biophys Acta 2001; 1538:99 - 117
- Gorlin JB, Yamin R, Egan S, Stewart M, Stossel TP, Kwiatkowski DJ, et al. Human endothelial actin-binding protein (ABP-280, nonmuscle filamin): a molecular leaf spring. J Cell Biol 1990; 111:1089 - 1105
- Fucini P, Renner C, Herberhold C, Noegel AA, Holak TA. The repeating segments of the F-actin cross-linking gelation factor (ABP-120) have an immunoglobulin-like fold. Nat Struct Biol 1997; 4:223 - 230
- Pudas R, Kiema TR, Butler PJ, Stewart M, Ylanne J. Structural basis for vertebrate filamin dimerization. Structure 2005; 13:111 - 119
- Seo MD, Seok SH, Im H, Kwon AR, Lee SJ, Kim HR, et al. Crystal structure of the dimerization domain of human filamin A. Proteins 2009; 75:258 - 263
- Nakamura F, Osborn TM, Hartemink CA, Hartwig JH, Stossel TP. Structural basis of filamin A functions. J Cell Biol 2007; 179:1011 - 1025
- Lad Y, Kiema T, Jiang P, Pentikainen OT, Coles CH, Campbell ID, et al. Structure of three tandem filamin domains reveals auto-inhibition of ligand binding. EMBO J 2007; 26:3993 - 4004
- Heikkinen OK, Ruskamo S, Konarev PV, Svergun DI, Iivanainen T, Heikkinen SM, et al. Atomic structures of two novel immunoglobulin-like domain pairs in the actin cross-linking protein filamin. J Biol Chem 2009; 284:25450 - 25458
- Nakamura F, Pudas R, Heikkinen O, Permi P, Kilpelainen I, Munday AD, et al. The structure of the GPIb-filamin A complex. Blood 2006; 107:1925 - 1932
- Kiema T, Lad Y, Jiang P, Oxley CL, Baldassarre M, Wegener KL, et al. The molecular basis of filamin binding to integrins and competition with talin. Mol Cell 2006; 21:337 - 347
- Lad Y, Jiang P, Ruskamo S, Harburger DS, Ylanne J, Campbell ID, et al. Structural basis of the migfilin-filamin interaction and competition with integrin beta tails. J Biol Chem 2008; 283:35154 - 35163
- Ithychanda SS, Das M, Ma YQ, Ding K, Wang X, Gupta S, et al. Migfilin, a molecular switch in regulation of integrin activation. J Biol Chem 2009; 284:4713 - 4722
- Nakamura F, Heikkinen O, Pentikainen OT, Osborn TM, Kasza KE, Weitz DA, et al. Molecular basis of filamin A-FilGAP interaction and its impairment in congenital disorders associated with filamin A mutations. PLoS ONE 2009; 4:4928
- Smith L, Page RC, Xu Z, Kohli E, Litman P, Nix JC, et al. Biochemical basis of the interaction between cystic fibrosis transmembrane conductance regulator and immunoglobulin-like repeats of filamin. J Biol Chem 2010; 285:17166 - 17176
- Playford MP, Nurminen E, Pentikainen OT, Milgram SL, Hartwig JH, Stossel TP, et al. Cystic fibrosis transmembrane conductance regulator interacts with multiple immunoglobulin domains of filamin A. J Biol Chem 2010; 285:17156 - 17165
- Li C, Yu S, Nakamura F, Pentikainen OT, Singh N, Yin S, et al. Pro-prion binds filamin A facilitating its interaction with integrin beta1 and contributes to melanomagenesis. J Biol Chem 2010; 285:30328 - 30339
- Ithychanda SS, Hsu D, Li H, Yan L, Liu D, Das M, et al. Identification and characterization of multiple similar ligand-binding repeats in filamin: implication on filamin-mediated receptor clustering and cross-talk. Biol Chem 2009; 284:35113 - 35121
- Pentikainen U, Ylanne J. The regulation mechanism for the auto-inhibition of binding of human filamin A to integrin. J Mol Biol 2009; 393:644 - 657
- Glogauer M, Arora P, Chou D, Janmey PA, Downey GP, McCulloch CA. The role of actin-binding protein 280 in integrin-dependent mechanoprotection. J Biol Chem 1998; 273:1689 - 1698
- Gehler S, Baldassarre M, Lad Y, Leight JL, Wozniak MA, Riching KM, et al. Filamin A-beta1 integrin complex tunes epithelial cell response to matrix tension. Mol Biol Cell 2009; 20:3224 - 3238
- Sharif-Naeini R, Folgering JH, Bichet D, Duprat F, Lauritzen I, Arhatte M, et al. Polycystin-1 and -2 dosage regulates pressure sensing. Cell 2009; 139:587 - 596
- Chen M, Stracher A. In situ phosphorylation of platelet actin-binding protein by cAMP-dependent protein kinase stabilizes it against proteolysis by calpain. J Biol Chem 1989; 264:14282 - 14289
- Vadlamudi RK, Li F, Adam L, Nguyen D, Ohta Y, Stossel TP, et al. Filamin is essential in actin cytoskeletal assembly mediated by p21-activated kinase 1. Nat Cell Biol 2002; 4:681 - 690
- Tigges U, Koch B, Wissing J, Jockusch BM, Ziegler WH. The F-actin cross-linking and focal adhesion protein filamin A is a ligand and in vivo substrate for protein kinase C alpha. J Biol Chem 2003; 278:23561 - 23569
- Woo MS, Ohta Y, Rabinovitz I, Stossel TP, Blenis J. Ribosomal S6 kinase (RSK) regulates phosphorylation of filamin A on an important regulatory site. Mol Cell Biol 2004; 24:3025 - 3035
- Zhong Z, Yeow WS, Zou C, Wassell R, Wang C, Pestell RG, et al. Cyclin d1/cyclin-dependent kinase 4 interacts with filamin a and affects the migration and invasion potential of breast cancer cells. Cancer Res 2010; 70:2105 - 2114
- Cukier IH, Li Y, Lee JM. Cyclin B1/Cdk1 binds and phosphorylates Filamin A and regulates its ability to cross-link actin. FEBS Lett 2007; 581:1661 - 1672
- Fox JE, Reynolds CC, Phillips DR. Calcium-dependent proteolysis occurs during platelet aggregation. J Biol Chem 1983; 258:9973 - 9981
- Jay D, Garcia EJ, de la Luz Ibarra M. In situ determination of a PKA phosphorylation site in the C-terminal region of filamin. Mol Cell Biochem 2004; 260:49 - 53
- Travis MA, van der Flier A, Kammerer RA, Mould AP, Sonnenberg A, Humphries MJ. Interaction of filamin A with the integrin beta7 cytoplasmic domain: role of alternative splicing and phosphorylation. FEBS Lett 2004; 569:185 - 190
- Chen HS, Kolahi KS, Mofrad MR. Phosphorylation facilitates the integrin binding of filamin under force. Biophys J 2009; 97:3095 - 3104
- Takala H, Nurminen E, Nurmi SM, Aatonen M, Strandin T, Takatalo M, et al. Integrin {beta}2 phosphorylation on THR758 acts as a molecular switch to regulate 14-3-3 and filamin binding. Blood 2008; 112:1853 - 1862
- Loy CJ, Sim KS, Yong EL. Filamin-A fragment localizes to the nucleus to regulate androgen receptor and coactivator functions. Proc Natl Acad Sci USA 2003; 100:4562 - 4567
- Berry FB, O'Neill MA, Coca-Prados M, Walter MA. FOXC1 transcriptional regulatory activity is impaired by PBX1 in a filamin A-mediated manner. Mol Cell Biol 2005; 25:1415 - 1424
- Wang Y, Kreisberg JI, Bedolla RG, Mikhailova M, deVere White RW, Ghosh PM. A 90 kDa fragment of filamin A promotes Casodex-induced growth inhibition in Casodex-resistant androgen receptor positive C4-2 prostate cancer cells. Oncogene 2007; 26:6061 - 6070
- Heuze ML, Lamsoul I, Baldassarre M, Lad Y, Leveque S, Razinia Z, et al. ASB2 targets filamins A and B to proteasomal degradation. Blood 2008; 112:5130 - 5140
- Bello NF, Lamsoul I, Heuze ML, Metais A, Moreaux G, Calderwood DA, et al. The E3 ubiquitin ligase specificity subunit ASB2beta is a novel regulator of muscle differentiation that targets filamin B to proteasomal degradation. Cell Death Differ 2009; 16:921 - 932
- Baldassarre M, Razinia Z, Burande CF, Lamsoul I, Lutz PG, Calderwood DA. Filamins regulate cell spreading and initiation of cell migration. PLoS ONE 2009; 4:7830
- Kwak KB, Chung SS, Kim OM, Kang MS, Ha DB, Chung CH. Increase in the level of m-calpain correlates with the elevated cleavage of filamin during myogenic differentiation of embryonic muscle cells. Biochim Biophys Acta 1993; 1175:243 - 249
- Mammoto A, Huang S, Ingber DE. Filamin links cell shape and cytoskeletal structure to Rho regulation by controlling accumulation of p190RhoGAP in lipid rafts. J Cell Sci 2007; 120:456 - 467
- Sharma CP, Ezzell RM, Arnaout MA. Direct interaction of filamin (ABP-280) with the beta2-integrin subunit CD18. J Immunol 1995; 154:3461 - 3470
- van der Flier A, Kuikman I, Kramer D, Geerts D, Kreft M, Takafuta T, et al. Different splice variants of filamin-B affect myogenesis, subcellular distribution and determine binding to integrin [beta] subunits. J Cell Biol 2002; 156:361 - 376
- Gontier Y, Taivainen A, Fontao L, Sonnenberg A, van der Flier A, Carpen O, et al. The Z-disc proteins myotilin and FATZ-1 interact with each other and are connected to the sarcolemma via muscle-specific filamins. J Cell Sci 2005; 118:3739 - 3749
- Legate KR, Fassler R. Mechanisms that regulate adaptor binding to beta-integrin cytoplasmic tails. J Cell Sci 2009; 122:187 - 198
- Andrews RK, Fox JE. Interaction of purified actin-binding protein with the platelet membrane glycoprotein Ib-IX complex. J Biol Chem 1991; 266:7144 - 7147
- Carman CV, Springer TA. Integrin avidity regulation: are changes in affinity and conformation underemphasized?. Curr Opin Cell Biol 2003; 15:547 - 556
- Takafuta T, Wu G, Murphy GF, Shapiro SS. Human beta-filamin is a new protein that interacts with the cytoplasmic tail of glycoprotein Ibalpha. J Biol Chem 1998; 273:17531 - 17538
- Krakow D, Robertson SP, King LM, Morgan T, Sebald ET, Bertolotto C, et al. Mutations in the gene encoding filamin B disrupt vertebral segmentation, joint formation and skeletogenesis. Nat Genet 2004; 36:405 - 410
- Zhou X, Tian F, Sandzen J, Cao R, Flaberg E, Szekely L, et al. Filamin B deficiency in mice results in skeletal malformations and impaired microvascular development. Proc Natl Acad Sci USA 2007; 104:3919 - 3924
- Thompson TG, Chan YM, Hack AA, Brosius M, Rajala M, Lidov HG, et al. Filamin 2 (FLN2): A muscle-specific sarcoglycan interacting protein. J Cell Biol 2000; 148:115 - 126
- Dalkilic I, Schienda J, Thompson TG, Kunkel LM. Loss of FilaminC (FLNc) results in severe defects in myogenesis and myotube structure. Mol Cell Biol 2006; 26:6522 - 6534
- Ferland RJ, Gaitanis JN, Apse K, Tantravahi U, Walsh CA, Sheen VL. Periventricular nodular heterotopia and Williams syndrome. Am J Med Genet A 2006; 140:1305 - 1311
- Unger S, Mainberger A, Spitz C, Bahr A, Zeschnigk C, Zabel B, et al. Filamin A mutation is one cause of FG syndrome. Am J Med Genet A 2007; 143:1876 - 1879
- Sjoblom T, Jones S, Wood LD, Parsons DW, Lin J, Barber TD, et al. The consensus coding sequences of human breast and colorectal cancers. Science 2006; 314:268 - 274
- Kyndt F, Gueffet JP, Probst V, Jaafar P, Legendre A, Le Bouffant F, et al. Mutations in the gene encoding filamin A as a cause for familial cardiac valvular dystrophy. Circulation 2007; 115:40 - 49
- Hart AW, Morgan JE, Schneider J, West K, McKie L, Bhattacharya S, et al. Cardiac malformations and midline skeletal defects in mice lacking filamin A. Hum Mol Genet 2006; 15:2457 - 2467
- Feng Y, Chen MH, Moskowitz IP, Mendonza AM, Vidali L, Nakamura F, et al. Filamin A (FLNA) is required for cell-cell contact in vascular development and cardiac morphogenesis. Proc Natl Acad Sci USA 2006; 103:19836 - 19841
- Zhou X, Boren J, Akyurek LM. Filamins in cardiovascular development. Trends Cardiovasc Med 2007; 17:222 - 229
- Khaire N, Muller R, Blau-Wasser R, Eichinger L, Schleicher M, Rief M, et al. Filamin-regulated F-actin assembly is essential for morphogenesis and controls phototaxis in Dictyostelium. J Biol Chem 2007; 282:1948 - 1955
- Annesley SJ, Bandala-Sanchez E, Ahmed AU, Fisher PR. Filamin repeat segments required for photosensory signalling in Dictyostelium discoideum. BMC Cell Biol 2007; 8:48
- Kovacevic I, Cram EJ. FLN-1/Filamin is required for maintenance of actin and exit of fertilized oocytes from the spermatheca in C. elegans. Dev Biol 2010; 347:247 - 257
- Vorgerd M, van der Ven PF, Bruchertseifer V, Lowe T, Kley RA, Schroder R, et al. A mutation in the dimerization domain of filamin c causes a novel type of autosomal dominant myofibrillar myopathy. Am J Hum Genet 2005; 77:297 - 304
- Gimbrone MA Jr, Topper JN, Nagel T, Anderson KR, Garcia-Cardena G. Endothelial dysfunction, hemodynamic forces and atherogenesis. Ann NY Acad Sci 2000; 902:230 - 239
- Engler AJ, Sen S, Sweeney HL, Discher DE. Matrix elasticity directs stem cell lineage specification. Cell 2006; 126:677 - 689
- Janmey PA, Hvidt S, Kas J, Lerche D, Maggs A, Sackmann E, et al. The mechanical properties of actin gels. Elastic modulus and filament motions. J Biol Chem 1994; 269:32503 - 32513
- Matsudaira P. Actin crosslinking proteins at the leading edge. Semin Cell Biol 1994; 5:165 - 174
- Gardel ML, Nakamura F, Hartwig JH, Crocker JC, Stossel TP, Weitz DA. Prestressed F-actin networks cross-linked by hinged filamins replicate mechanical properties of cells. Proc Natl Acad Sci USA 2006; 103:1762 - 1767
- Kasza KE, Koenderink GH, Lin YC, Broedersz CP, Messner W, Nakamura F, et al. Nonlinear elasticity of stiff biopolymers connected by flexible linkers. Phys Rev E Stat Nonlin Soft Matter Phys 2009; 79:41928
- Kasza KE, Nakamura F, Hu S, Kollmannsberger P, Bonakdar N, Fabry B, et al. Filamin A is essential for active cell stiffening but not passive stiffening under external force. Biophys J 2009; 96:4326 - 4335
- Cunningham CC, Gorlin JB, Kwiatkowski DJ, Hartwig JH, Janmey PA, Byers HR, et al. Actin-binding protein requirement for cortical stability and efficient locomotion. Science 1992; 255:325 - 327
- Simpson KJ, Selfors LM, Bui J, Reynolds A, Leake D, Khvorova A, et al. Identification of genes that regulate epithelial cell migration using an siRNA screening approach. Nat Cell Biol 2008; 10:1027 - 1038
- Fox JW, Lamperti ED, Eksioglu YZ, Hong SE, Feng Y, Graham DA, et al. Mutations in filamin 1 prevent migration of cerebral cortical neurons in human periventricular heterotopia. Neuron 1998; 21:1315 - 1325
- Sarkisian MR, Bartley CM, Chi H, Nakamura F, Hashimoto-Torii K, Torii M, et al. MEKK4 signaling regulates filamin expression and neuronal migration. Neuron 2006; 52:789 - 801
- Pavalko FM, Otey CA, Burridge K. Identification of a filamin isoform enriched at the ends of stress fibers in chicken embryo fibroblasts. J Cell Sci 1989; 94:109 - 118
- Calderwood DA, Huttenlocher A, Kiosses WB, Rose DM, Woodside DG, Schwartz MA, et al. Increased filamin binding to beta-integrin cytoplasmic domains inhibits cell migration. Nat Cell Biol 2001; 3:1060 - 1068
- Bellanger JM, Astier C, Sardet C, Ohta Y, Stossel TP, Debant A. The Rac1- and RhoG-specific GEF domain of Trio targets filamin to remodel cytoskeletal actin. Nat Cell Biol 2000; 2:888 - 892
- Urban E, Jacob S, Nemethova M, Resch GP, Small JV. Electron tomography reveals unbranched networks of actin filaments in lamellipodia. Nat Cell Biol 2010; 12:429 - 435
- Lammermann T, Bader BL, Monkley SJ, Worbs T, Wedlich-Soldner R, Hirsch K, et al. Rapid leukocyte migration by integrin-independent flowing and squeezing. Nature 2008; 453:51 - 55
- Barry NP, Bretscher MS. Dictyostelium amoebae and neutrophils can swim. Proc Natl Acad Sci USA 2010; 107:11376 - 11380
- O'Connell MP, Fiori JL, Baugher KM, Indig FE, French AD, Camilli TC, et al. Wnt5A activates the calpain-mediated cleavage of filamin A. J Invest Dermatol 2009; 129:1782 - 1789
- Xu Y, Bismar TA, Su J, Xu B, Kristiansen G, Varga Z, et al. Filamin A regulates focal adhesion disassembly and suppresses breast cancer cell migration and invasion. J Exp Med 2010; 207:2421 - 2437
- Franco SJ, Rodgers MA, Perrin BJ, Han J, Bennin DA, Critchley DR, et al. Calpain-mediated proteolysis of talin regulates adhesion dynamics. Nat Cell Biol 2004; 6:977 - 983
- Flevaris P, Stojanovic A, Gong H, Chishti A, Welch E, Du X. A molecular switch that controls cell spreading and retraction. J Cell Biol 2007; 179:553 - 565
- Kim H, Nakamura F, Lee W, Hong C, Perez-Sala D, McCulloch CA. Regulation of cell adhesion to collagen via beta1 integrins is dependent on interactions of filamin A with vimentin and protein kinase C epsilon. Exp Cell Res 2010; 316:1829 - 1844
- Sanz-Moreno V, Gadea G, Ahn J, Paterson H, Marra P, Pinner S, et al. Rac activation and inactivation control plasticity of tumor cell movement. Cell 2008; 135:510 - 523
- Fackler OT, Grosse R. Cell motility through plasma membrane blebbing. J Cell Biol 2008; 181:879 - 884
- Charras GT, Yarrow JC, Horton MA, Mahadevan L, Mitchison TJ. Non-equilibration of hydrostatic pressure in blebbing cells. Nature 2005; 435:365 - 369
- Yin HL, Zaner KS, Stossel TP. Ca2+ control of actin gelation. Interaction of gelsolin with actin filaments and regulation of actin gelation. J Biol Chem 1980; 255:9494 - 9500
- Nakamura F, Hartwig JH, Stossel TP, Szymanski PT. Ca2+ and calmodulin regulate the binding of filamin A to actin filaments. J Biol Chem 2005; 280:32426 - 32433
- Ohta Y, Hartwig JH, Stossel TP. FilGAP, a Rho- and ROCK-regulated GAP for Rac binds filamin A to control actin remodelling. Nat Cell Biol 2006; 8:803 - 814
- Shifrin Y, Arora PD, Ohta Y, Calderwood DA, McCulloch CA. The role of FilGAP-filamin A interactions in mechanoprotection. Mol Biol Cell 2009; 20:1269 - 1279
- Gawecka JE, Griffiths GS, Ek-Rylander B, Ramos JW, Matter ML. R-Ras regulates migration through an interaction with filamin A in melanoma cells. PLoS One 2010; 5:11269
- Falet H, Pollitt AY, Begonja AJ, Weber SE, Duerschmied D, Wagner DD, et al. A novel interaction between FlnA and Syk regulates platelet ITAM-mediated receptor signaling and function. J Exp Med 2010; 207:1967 - 1979
- Smith TC, Fang Z, Luna EJ. Novel interactors and a role for supervillin in early cytokinesis. Cytoskeleton (Hoboken) 2010; 67:346 - 364
- Li C, Yu S, Nakamura F, Yin S, Xu J, Petrolla AA, et al. Binding of pro-prion to filamin A disrupts cytoskeleton and correlates with poor prognosis in pancreatic cancer. J Clin Invest 2009; 119:2725 - 2736
- Nikki M, Merilainen J, Lehto VP. FAP52 regulates actin organization via binding to filamin. J Biol Chem 2002; 277:11432 - 11440
- Armstrong LJ, Heath VL, Sanderson S, Kaur S, Beesley JF, Herbert JM, et al. ECSM2, an endothelial specific filamin a binding protein that mediates chemotaxis. Arterioscler Thromb Vasc Biol 2008; 28:1640 - 1646
- Nagano T, Yoneda T, Hatanaka Y, Kubota C, Murakami F, Sato M. Filamin A-interacting protein (FILIP) regulates cortical cell migration out of the ventricular zone. Nat Cell Biol 2002; 4:495 - 501
- Fox JE. Identification of actin-binding protein as the protein linking the membrane skeleton to glycoproteins on platelet plasma membranes. J Biol Chem 1985; 260:11970 - 11977
- Li Y, Lu J, Prochownik EV. c-Myc-mediated genomic instability proceeds via a megakaryocytic endomitosis pathway involving Gp1balpha. Proc Natl Acad Sci USA 2007; 104:3490 - 3495
- Kanters E, van Rijssel J, Hensbergen PJ, Hondius D, Mul FP, Deelder AM, et al. Filamin B mediates ICAM-1-driven leukocyte transendothelial migration. J Biol Chem 2008; 283:31830 - 31839
- Tu Y, Wu S, Shi X, Chen K, Wu C. Migfilin and Mig-2 link focal adhesions to filamin and the actin cytoskeleton and function in cell shape modulation. Cell 2003; 113:37 - 47
- Takafuta T, Saeki M, Fujimoto TT, Fujimura K, Shapiro SS. A new member of the LIM protein family binds to filamin B and localizes at stress fibers. J Biol Chem 2003; 278:12175 - 12181
- Maceyka M, Alvarez SE, Milstien S, Spiegel S. Filamin A links sphingosine kinase 1 and sphingosine-1-phosphate receptor 1 at lamellipodia to orchestrate cell migration. Mol Cell Biol 2008; 28:5687 - 5697
- Ott I, Fischer EG, Miyagi Y, Mueller BM, Ruf W. A role for tissue factor in cell adhesion and migration mediated by interaction with actin-binding protein 280. J Cell Biol 1998; 140:1241 - 1253
- Klaile E, Muller MM, Kannicht C, Singer BB, Lucka L. CEACAM1 functionally interacts with filamin A and exerts a dual role in the regulation of cell migration. J Cell Sci 2005; 118:5513 - 5524
- Ohta Y, Suzuki N, Nakamura S, Hartwig JH, Stossel TP. The small GTPase RalA targets filamin to induce filopodia. Proc Natl Acad Sci USA 1999; 96:2122 - 2128
- Del Valle-Perez B, Martinez VG, Lacasa-Salavert C, Figueras A, Shapiro SS, Takafuta T, et al. Filamin B plays a key role in VEGF-induced endothelial cell motility through its interaction with Rac-1 and Vav-2. J Biol Chem 2010; 285:10748 - 10760
- Jeon YJ, Choi JS, Lee JY, Yu KR, Ka SH, Cho Y, et al. Filamin B serves as a molecular scaffold for type I interferon-induced c-Jun NH2-terminal kinase signaling pathway. Mol Biol Cell 2008; 19:5116 - 5130
- Ueda K, Ohta Y, Hosoya H. The carboxy-terminal pleckstrin homology domain of ROCK interacts with filamin-A. Biochem Biophys Res Commun 2003; 301:886 - 890
- Kwon M, Hanna E, Lorang D, He M, Quick JS, Adem A, et al. Functional characterization of filamin a interacting protein 1-like, a novel candidate for antivascular cancer therapy. Cancer Res 2008; 68:7332 - 7341
- Johansen LD, Naumanen T, Knudsen A, Westerlund N, Gromova I, Junttila M, et al. IKAP localizes to membrane ruffles with filamin A and regulates actin cytoskeleton organization and cell migration. J Cell Sci 2008; 121:854 - 864
- Pi M, Spurney RF, Tu Q, Hinson T, Quarles LD. Calcium-sensing receptor activation of rho involves filamin and rho-guanine nucleotide exchange factor. Endocrinology 2002; 143:3830 - 3838
- McDonough WS, Tran NL, Berens ME. Regulation of glioma cell migration by serine-phosphorylated P311. Neoplasia 2005; 7:862 - 872