Abstract
We had developed a conditional Laminin α 1 knockout-mouse model (Lama1cko) bypassing embryonic lethality of Lama1 deficient mice to study the role of this crucial laminin chain during late developmental phases and organogenesis. Here, we report a strong defect in the organization of the adult cerebellum of Lama1cko mice. Our study of the postnatal cerebellum of Lama1cko animals revealed a disrupted basement membrane correlated to an unexpected excessive proliferation of granule cell precursors in the external granular layer (EGL). This was counteracted by a massive cell death occurring between the postnatal day 7 (P7) and day 20 (P20) resulting in a net balance of less cells and a smaller cerebellum. Our data show that the absence of Lama1 has an impact on the Bergmann glia scaffold that aberrantly develops. This phenotype is presumably responsible for the observed misplacing of granule cells that may explain the overall perturbation of the layering of the cerebellum and an aberrant folia formation.
Introduction
Laminins are prototypic components of the extracellular matrix. This large family of heterotrimeric glycoproteins consisting of an a chain along with one β and one γ chain exhibits both structural and functional roles. Laminins are major constituents of basement membranesCitation1 that are also implicated in a variety of physiological and pathological processes including organogenesisCitation2 and cancer.Citation3 Consistently numerous reports provide evidence for the implication of laminins in cell proliferation, cell migration or cell differentiation both during embryonic development as well as in adult tissue homeostasis. The laminin-111 (LM-111) isoform (composed of α1, β1 and γ1 chains), was the first identified isoform and exhibits certainly the best example of the pleiotropic nature of laminins. Laminin α1 (LMα1) is expressed very early during embryonic development with persistent expression in some adult basement membranes including those of the kidney, liver, testis and ovary.Citation4 While clearly involved in the formation of the basement membrane,Citation5 LMα1 also impacts on cell migration particularly on tumorCitation6 and endothelial cells in vitro.Citation7 However, in vivo developmental studies have been limited by early lethality of LMα1 deficient mice that die at embryonic day E7 as a direct consequence of an impaired Reichert's membraneCitation5,Citation8 preventing epiblast differentiation.Citation9 To circumvent this issue we recently developed a conditional knockout mouse for the Lama1 gene (Lama1cko) preserving LMα1 function in extra-embryonic cells thereby bypassing the embryonic lethality but lacking LMα1 in all embryonic cells. Mice with a constitutive ablation of Lama1 are blind as a consequence of a defective inner limiting membrane and an abnormal differentiation of the Müller glial cells that are supporting the organization of the retina.Citation10 These defects were associated with alteration of the ganglion cell layer, one of the major neuronal populations of the retina. Since 20 years ago, a developmental function of laminins has been suggested by in vitro studies that showed a role in promoting neurite outgrowth or cell migration.Citation11 This is also the case in the cerebellum, an exquisite model for the central nervous system development allowing the precise analysis of neurogenesis and neuronal cell migration because of a unique cytoarchitecture acquired during a well-established cellular ballet described more than a century ago. Again, most of the studies describing the role of laminins in the cerebellum had used in vitro assays. Thus, the use of genetic tools allowing the molecular dissection of the roles of individual laminin chains in vivo provides a valuable strategy to unravel the exact mechanisms of cerebellar histogenesis. Here, we report the strong impairment of adult cerebellar organization in Lama1cko mice. Our study of the postnatal development of the cerebellum revealed an unexpected excessive proliferation of granule cell precursors in the external granular layer (EGL) of Lama1cko animals compensated by cell death between postnatal day 7 (P7) and day 20 (P20) resulting in a reduced size of the adult cerebellum. Hence, an abnormal development of Bergmann glia network concomitant with a partial disruption of the basement membrane could explain the observed misplacing of granule cells contributing to the global perturbation of cerebellar layering and folia formation.
Results
Adult mice with a laminin α1 deficiency exhibit an abnormal organization of the cerebellum.
To investigate the biological consequences of Lama1 gene ablation in the cerebellum we first examined its gross anatomy. As seen in , we observed a strong reduction in the size of the cerebellum ( and B) with marked reduction of lobule organization and fissures ( and B). Hemispheres were strongly atrophied and showed no clear delimitation of ansiform, simplex or paramedian lobules. Similarly, the vermis also showed a lack of lobular organization ( and B). Microphotographs of cresyl violet-stained cryosections revealed profound modifications of folium organization on coronal sections ( and D). Strikingly, a subset of cells, organized in the form of islets, was sparsely detected in Lama1cko mice () at the outer border of the molecular layer (ML). While the limits between lobules were clearly seen in control animals, Lama1cko mice showed an almost complete loss of folia in the anterior part of the cerebellum and numerous fusion lines between folia in the posterior part (). Thus, defective lobular organization was concomitant with abnormal folia individualization. Altogether, these results suggest a strong functional role of the LMα1 chain in cerebellum development.
Laminin α1 is expressed in the developing cerebellum and contributes to the integrity of the basement membrane.
To further analyze the potential involvement of the LMα1 chain in cerebellum development we documented its precise distribution in adult and in postnatal sections using a specific anti-LMα1 antibody.Citation12 We chose P7 (peak of granule cell production) and P20 (end of proliferation and migration of granule cell precursors) to cover crucial stages of cerebellar development. At all developmental stages as well as in the adult, LMα1 staining is found located at the external meningeal basement membrane delineating the surface of cerebellar folia and in the basement membrane of some blood vessels (–). This staining matched that obtained with a pan-laminin antibody recognizing α1, β1 or γ1 laminin chains (, and ). In all cases we found no signal when using the LMα1 antibody in the Lama1cko (, and ). Importantly, a pan-laminin staining in Lama1cko mice revealed a discontinued meningeal basement membrane and an almost complete lack of staining at fusion lines of folia (, and ). Consistently, partial disruption of the basement membrane in the Lama1cko was confirmed using type IV collagen (, H, , H and and F) and perlecan antibodies ( and H), two other basement membrane constituents. Thus, the lack of the LMα1 chain altered the general organization of the basement membranes thereby potentially affecting cellular behaviors occurring in this region. Of note, the basement membrane surrounding the blood vessels of the cerebellar parenchyma looked intact in Lama1cko as judged by the pan-laminin and the type IV collagen staining.
Laminin α1 is a negative regulator of granule cell proliferation.
To address whether the reduced size of the cerebellum was related to abnormal proliferation we quantified the production of granule cells by counting Ki67 (a general marker of cell proliferation)Citation15 positive granule cell precursors in the external granular layer (EGL) at P7 (). Unexpectedly, we quantified an increased number of Ki67 positive cells in the EGL of Lama1cko mice (+26%, on a total of 8,402 counted cells, p < 0.01). Moreover, we observed Ki67 positive cells within the entire thickness of the EGL while proliferative granule precursor cells (GPC) were only detected in the external part of the EGL in control animals as expected from previous demonstration of compartmentalization of proliferation and differentiation of GPC in this layer.Citation16 Strikingly, the density of GPC was similar for both genotypes in the EGL (11,960 ± 455 cells/mm2 in control vs. 12,479 ± 636 cells/mm2 in Lama1cko, p = 0.5168). This result suggested that the LMα1 chain is not involved in early tangential migration of granule precursor cells (GPC) from the rhombic lip to the surface of the cerebellar primordium containing the nascent external granular layer (EGL). However, the ectopic Ki67 staining observed in the deep part of the EGL implied a role of the LMα1 chain in compartmentalization of cerebellar neurogenesis. This excessive proliferation was confirmed using the mitotic marker phospho-histone 3 (PH3),Citation17 (, +42% in Lama1cko, on a total of 13,117 cells counted, p < 0.01) that also revealed ectopic mitosis in the inner part of the EGL. Surprisingly, we observed persistent Ki67 positive GPC-like cells in the islets of cells bordering the pial surface at P20 () (4.6% of GC persisting at the outer border of the ML, on a total of 2,364 ectopic cells counted) while an established molecular layer with rare proliferating GPC was established in control animals. However, GPC-like cells of the islets were not positive for PH3 staining thereby suggesting that cells persisting in the EGL may have experienced cell cycle arrest after the S-phase (data not shown).
Laminin α1 chain is impacting on survival of granule cells.
Considering the global reduction of the cerebellum size, the granule cell survival is probably altered in Lama1cko mice to compensate the excessive proliferation of GPC. To address this possibility, we performed BrdU labeling of proliferating cells at P7 and quantified the number of surviving postmitotic cells at P20. As described in , we found that the number of BrdU positive cells detected at P20 was similar in control and Lama1cko (9.2% in control vs. 9.8% in Lama1cko mice) in spite of the increased proliferation of GPC in the EGL that had been detected with Ki67 and PH3 staining at P7. Thus, most of overproliferating cells generated at P7 already disappeared at P20. Consistently, we found an almost 2-fold increase of caspase-3 positive cells in Lama1cko mice compared with control animals (4.2% to 7.3% in control and Lama1cko respectively, p < 0.01, ). Hence, the aberrant proliferation observed in Lama1cko EGL is counteracted by cell death soon after the end of the mitotic phase of GPC.
Laminin α1 chain is impacting on migration of granular cells.
To determine how the LMα1 chain is involved in GC radial migration we also analyzed the position of P7 BrdU-labeled GC on P20 cryosections. This showed that in Lama1cko, GC failed to migrate properly to the IGL as some BrdU-positive granule cells were found in islets of cells persisting in the EGL. Quantitative analysis revealed that more than 19% of the cells in the islets found in the EGL of Lama1cko were BrdU positive. Because Bergmann glia (BG) processes are providing a scaffold for migrating GCCitation18 we explored the general organization of this network using GFAP (a marker of BG) immunofluorescence detection. At P7 (data not shown) and P20 () we observed alignments of BG fibers in control animals clearly delineating the routes from the Purkinje cell layer (PCL) to the pial surface. However, in Lama1cko mice, cell bodies of BG were seen randomly dispersed all over the ML, PCL and IGL. Moreover, BG fibers were not forming the classical scaffold with lateral appendages and failed to anchor properly in the pial surface. Thus, the general disorganization of cerebellar layers could be attributed to the lack of functionally relevant BG network.
Discussion
We previously described the generation of a conditional knockout of the Lama1 gene with selective deletion only in embryonic cells bypassing the early embryonic death observed in total Lama1 knockout mice.Citation10 This model allowed us to discover the importance of the Lama1 gene in retina development, its absence leading to blindness. We now used this animal model to analyze the cerebellar postnatal development, one of the most important structures of the central nervous system. Our results demonstrate multiple functional roles of the LMα1 exemplified by abnormal foliation of cerebellar lobules, disorganized cytoarchitecture including ectopic GC and PC positioning and excessive GPC proliferation counteracted by compensatory cell death, disturbed cell migration and Bergmann glia network malformation.
A striking feature observed in Lama1cko mice is the general alteration of the meningeal basement membrane. It appeared discontinuous and almost absent at fusion lines between folia. In contrast, the basement membrane of blood vessels in the cerebellar parenchyma looked intact. This suggested that the LMα1 deficit selectively perturbed meningeal basement membranes. Here, we provide further in vivo evidence for a role of Lama1 containing laminins in basement membrane assembly and stabilization. Besides this structural role, this laminin chain is usually associated with cell signaling through specific interactions with membrane receptors (integrins, dystroglycan) as clearly defined by in vitro experiments.Citation19,Citation20 Strikingly, the phenotype seen in the Lama1cko cerebellum is quite similar to the one described in the dystroglycan receptor conditional knockout mouse.Citation21 Ectopic EGL were also observed in knockout mice of Large and O-mannose UDPN-acetylglucosaminyl transferase 1 (POMGnT1) that are two enzymes required to ensure proper glycosylation of the α-dystroglycan receptor.Citation22,Citation23 Hence, because this post-translational modification is required for binding to the LMα1 G domain future studies will need to address to what extent a-dystroglycan receptor signaling is altered in our Lama1cko model.
Moreover, one of the functions of the basement membrane is to ensure efficient localization and anchoring of glial cell endfeet as recently reported by us for Müller glial cells in the retina.Citation24 We now found a similar role in the cerebellum because we observed a severe disorganization of the Bergmann glia network responsible for radial migration of GCCitation25 from the EGL to the IGL. Not only were glial fibers abnormally orientated but mispositioning of Bergmann glial cell bodies was also frequent. Consequently, numerous GC failed to reach their correct laminar position. The most striking case is the persistence of GC in the outer border of the ML which normally does not contain such cells in the adult. This abnormal localization of GC is furthermore consistent with previous in vitro studies suggesting that laminin-111 may represent a repulsive guidance cue for migrating GC.Citation26 However, we found the same number of GPC in control and Lama1cko mice therefore suggesting that LMα1 is not impacting on the tangential migration of GPC originating from the rhombic lip. Once reaching the EGL, GPC undergo rounds of proliferation to ensure sufficient production of GC, the most abundant neurons in the central nervous system. Because we observed a marked decrease of the cerebellum size in the adult, we expected reduced GPC proliferation in the EGL. Instead, both quantifications of Ki67 (a general marker of proliferative cells) and PH3 (a marker of the mitotic phase) demonstrated 26% and 42% increased proliferation of GPC, respectively. This apparent paradox is in fact in accordance with recent data suggesting that laminin acts as a repressor of sonic hedgehog (SHH)-induced proliferation of GPC.Citation27 The lack of LMα1 is obviously sufficient to perturb fine-tuning of GPC proliferation. In fact, the two different markers of proliferation used in our study revealed proliferative cells in the inner part of the EGL. This intriguing result suggests that LMα1 plays a crucial role in the compartmentalization of the EGL by controlling the transition from proliferating GPC to post-mitotic GC transiently accumulating in the deep part of the EGL before migration toward the IGL. Thus it is possible that LMα1 has an impact on the cell cycle which needs to be addressed in the future. This is particularly relevant because a significant number of ectopic GC located at the outer border of the ML in P20 animals were positive for KI67 (19%) but not for PH3 therefore suggesting an abortive cell cycle. We carefully analyzed the proliferation of GPC because the group of Arikawa-HirasawaCitation28 published in parallel to this work the description of a similar cerebellar malformation in a different model of Lama1 conditional knockout mouse. While most of the cerebellar phenotypes appeared similar (including abnormal foliation, ectopic GC positioning, defective Bergmann glia and disrupted basement membrane), the authors reported a decreased proliferation of GPC in the EGL using PH3 staining. They also mentioned no additional apoptosis in their Lama1 conditional knockout mice. In our study, the use of two different proliferation markers was complemented by BrdU incorporation studies that confirmed the increase of GPC proliferation at P7 (+17%) and suggested rapid elimination of the fraction of over-proliferative cells because the number of BrdU positive postmitotic GC was similar in both genotypes at P20. Indeed, we confirmed the existence of an increased cell death process by caspase-3 staining showing a significant difference between control and cko mice. This apparent discrepancy requires further temporal exploration of the functional roles of LMα1 as variation of few hours or days during this highly dynamic period of growth may reveal the precise spatiotemporal roles of laminins in cerebellar development.
Hence, the Lama1 gene has been recently reported in the list of the 50 genes important for recessive cognitive disorders in human.Citation29 Thus, our initial description of the Lama1cko mouse provides an important starting point to perform the molecular dissection of the functional roles of LMα1 in the development of key nervous system structures such as the cerebellum or the brain to identify the therapeutic potential of this secreted protein in central nervous system diseases.
Material and Methods
Generation of conditional Lama1 knockout mice (Lama1cko).
The Lama1cko (Lama1−/− Sox2 Cre) was obtained in 129sv/C57Bl6/CD1 mixed genetic background as previously described in reference Citation10. In the current study, Lama1+/−Sox2 Cre pups from the same littermates were used as controls. Mice were under a 12 h light/12 h dark cycle with water and ad libitum food. All experimental procedures were performed according to European guidelines and standard for animal care.
Histology and immunodetection.
Cerebella were collected at postnatal day P7, P20 and adult (8 weeks of age) and were fresh frozen in −50°C isopentane before conservation at −80°C. They were embedded in optimal cutting temperature compound (OCT Tissue-Tek) to prepare 7 µm-thick coronal sections using a cryostat (Leica CM3050 S). Sections were mounted on Superfrost Plus slides (Menzel-Gläser). For histological analysis, cryosections were fixed 15 min in 4% formaldehyde (FA) in phosphate-buffered saline (PBS) before staining with Cresyl Violet solution 2 g/L for 15 min at room temperature (Sigma). For immunofluorescence detection of extracellular matrix components, primary antibodies were incubated overnight at +4°C, slides were washed three times in PBS and incubated for 1 h with Alexa-488-conjugated goat anti-rat IgG secondary antibodies (Molecular Probes) and/or cyanine3-conjugated goat anti-rabbit IgG secondary antibodies (Jackson ImmunoResearch Laboratories). After washing, nuclei were counterstained with DAPI (1:30,000). Slides were mounted in FluorSave Reagent (Calbiochem). The following primary antibodies were used: rat monoclonal anti-laminin α1 chain G4 domain (1:100, mAb 200, a gift from L. Sorokin),Citation12 rabbit polyclonal pan-laminin (1:200, produced by the team),Citation13 rabbit polyclonal anti-type IV collagen (1:200, produced by the team)Citation14 and rat monoclonal anti-Perlecan (1:500, MAB1948, Chemicon). For other immunofluorescence detections, cryosections were fixed 15 min in 4% FA, rinsed with PBS, permeabilized for 10 min with 0.1% Triton X-100 in PBS, then blocked for 30 min with 5% normal goat serum in PBS. Primary antibodies were incubated in blocking buffer overnight at +4°C, slides were washed three times in PBS and incubated for 1 h and processed as previously mentioned. The following primary antibodies were used: rabbit monoclonal anti-Ki67 (1:500, SP6, Thermo Scientific) and mouse monoclonal anti-GFAP (1:1,000, MAB3402, Chemicon). For detection of phospho-histone H3, cryosections were blocked for 45 min with 5% normal goat serum in PBS then incubated with anti-phospho-histone H3 antibody (1:200, rabbit polyclonal Ser10 anti-phospho-histone H3, Millipore) in blocking buffer overnight at +4°C. Slides were then processed as previously described.
Caspase-3 staining.
Cryosections were permeabilized for 10 min with 0.1% Triton X-100 in PBS and blocked for 30 min in 5% normal goat serum in PBS before overnight incubation at +4°C with rabbit polyclonal anticleaved caspase-3 (1:200, 9661, Cell Signaling) in blocking buffer. Slices were rinsed with 0.1% Triton X-100 in PBS three times 5 min then incubated in goat anti-rabbit IgG biotinylated secondary antibody in PBS (1:200, Vector Labs). After washing with 0.1% Triton X-100 in PBS (three times 5 min), slices were incubated with 0.5% H2O2 in methanol for 30 min, rinsed again with 0.1% Triton X-100 in PBS (three times 5 min), then revealed with avidin-biotin-peroxidase (Vectastain ABC Kit, Vector Labs) and visualized using diaminobenzidine reaction. Nuclei were counterstained with Harris hematoxylin and sections were dehydrated before mounting with Eukitt (Sigma).
BrdU birth dating experiments.
To study migration of postmitotic granule cells (GC), mice were subjected to intraperitoneal injections of 5-bromodeoxyuridine (BrdU, 50 mg/kg body weight, Sigma) at P7 and were sacrificed at P20. Cryosections were fixed for 15 min in 4% FA in PBS and washed three times 5 min in the same buffer. Slices were boiled in citrate buffer 10 min in a microwave oven, rinsed with water 3 min and PBS 3 min, then soaked in hydrochloric acid 2 M at room temperature for 1 h. They were washed in PBS 0.1% Triton X-100 three times 5 min then incubated with polyclonal sheep anti-BrdU biotinylated antibody (1:200, AB2284, Abcam) overnight at +4°C. After washing with 0.1% Triton X-100 in PBS (three times 5 min), slices were incubated with 0.5% H2O2 in methanol for 30 min, rinsed again with 0.1% Triton X-100 in PBS (three times 5 min), then revealed with avidin-biotin-peroxidase (Vectastain ABC Kit, Vector Labs) and visualized using diaminobenzidine reaction. Nuclei were counterstained with Harris hematoxylin and sections were dehydrated before mounting with Eukitt (Sigma).
Microphotograph acquisition and statistical analyses.
Images of cresyl violet staining were acquired with a Zeiss Axio Imager A1 microscope equipped with an A-Plan x5/0.12, an A-Plan x20/0.45 objective and a Zeiss Axiocam Icc3 color camera. Images of immunofluorescence were acquired with a Zeiss Axio Imager Z2 fluorescence microscope equipped with a Plan Apochromat x20/0.8, a Plan Apochromat x40/0.95 objectives and a Zeiss Axiocam MRm black and white camera. All images were compiled using GIMP. Statistical analyses were performed using ChiCitation2 test in assays comparing cellular location and Student's t-test in assays determining cell densities. A p-value less of 0.05 was considered statistically significant.
Disclosure of Potential Conflicts of Interest
No potential conflicts of interest were disclosed.
Abbreviations
GC | = | granule cells |
GPC | = | granule precursor cells |
EGL | = | external granular layer |
IGL | = | internal granular layer |
ML | = | molecular layer |
PCL | = | purkinje cell layer |
GFAP | = | glial fibrillary acidic protein |
PH3 | = | phospho histone 3 |
Figures and Tables
Figure 1 Abnormal cerebellar organization in adult Lama1cko animals. Macroscopic observation of whole cerebella (A and B) reveals a reduced size of Lama1cko adult cerebellum compare with control. Histological examination of coronal sections of adult control (C) and Lama1cko (D) cerebella stained with cresyl violet demonstrates abnormal foliation and global perturbation of cerebellar layers (E and F). Black arrow heads are pointing out the limits between folia in control mice (C). White arrows are pointing out islets of ectopic cells abnormally located in the outer part of the molecular layer of Lama1cko (F). Scale bars: 1 mm (A), 100 µm (C–F). ML, Molecular Layer; IGL, Internal Granular Layer.
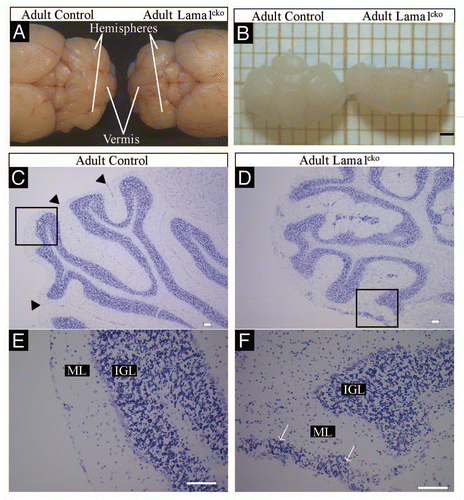
Figure 2 Basement membrane alteration in P7 Lama1cko animals. Coronal sections of P7 control (A, C, E and G) and Lama1cko (B, D, F and H) cerebella stained with cresyl violet (A and B), laminin α1 antibody (C and D), pan-laminin antibody (E and F) and Type IV collagen (G and H). Scale bar: 100 µm. EGL, External Granular Layer; IGL, Internal Granular Layer.
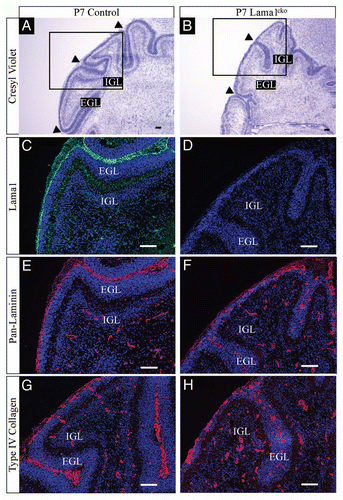
Figure 3 Basement membrane alteration in P20 Lama1cko animals. Coronal sections of P20 control (A, C, E and G) and Lama1cko (B, D, F and H) cerebella stained with cresyl violet (A and B), laminin α1 antibody (C and D), pan-laminin antibody (E and F) and Type IV collagen (G and H). Scale bar: 100 µm. ML, Molecular Layer; IGL, Internal Granular Layer.
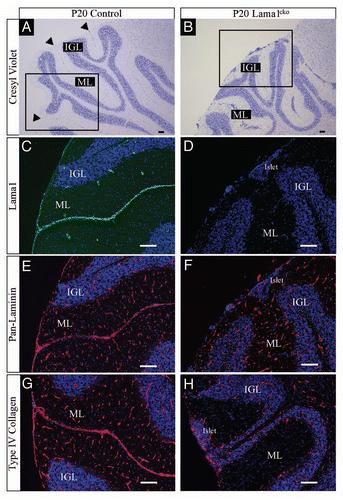
Figure 4 Basement membrane alteration in adult Lama1cko animals. Coronal sections of adult control (A, C, E and G) and Lama1cko (B, D, F and H) cerebella stained with laminin α1 antibody (A and B), panlaminin antibody (C and D), Type IV collagen (E and F) and Perlecan (G and H). Scale bar: 100 µm. ML, Molecular Layer; IGL, Internal Granular Layer.
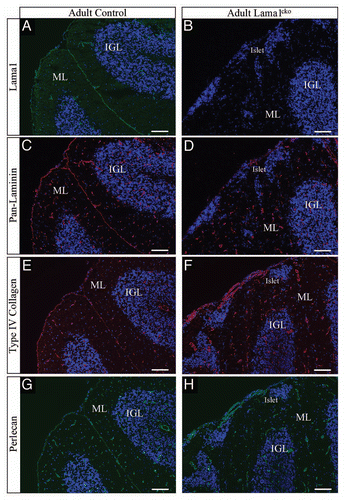
Figure 5 Increased proliferation (Ki67) of granule cell precursors in P7 Lama1cko animals. Coronal sections of P7 control (A and C) and Lama1cko (B and D) cerebella stained for the proliferation marker Ki67 and counterstained with DAPI. Camera lucida drawing highlights the localization of Ki67 positive cells (E and F). The dashed line in the EGL of control animals (E) represents the limit between the outer and inner part of this layer. (G) Quantification of Ki67 positive cells (**p < 0.01). Scale bar: 100 µm. EGL, External Granular Layer; IGL, Internal Granular Layer.
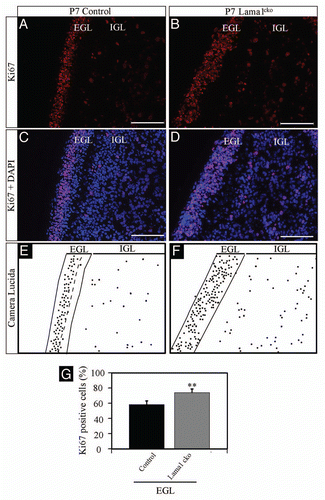
Figure 6 Increased proliferation of granule cell precursors in P7 Lama1cko animals (PH3). Coronal sections of P7 control (A and C) and Lama1cko (B and D) cerebella stained for the proliferation marker PH3 and counterstained with DAPI. Camera lucida drawing highlights the localization of PH3 positive cells (E and F). The dashed line represents the limit between the EGL and IGL. (G) Quantification of PH3 positive cells (**p < 0.01). Scale bar: 100 µm. EGL, External Granular Layer; IGL, Internal Granular Layer.
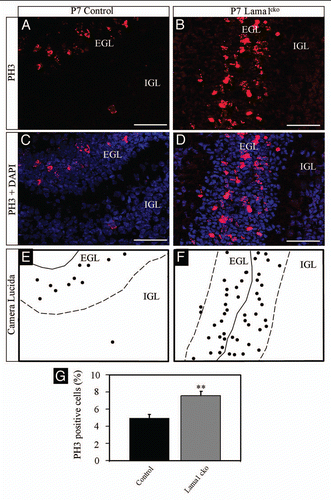
Figure 7 Persistence of Ki67-positive cells in the ML of P20 Lama1cko animals. Coronal sections of P20 control (A and C) and Lama1cko (B and D) cerebella stained for the proliferation marker Ki67 and counterstained with DAPI. Camera lucida drawing highlights the localization of Ki67 positive cells (E and F). The dashed line represents the limit between the ML and IGL. Scale bar: 100 µm. ML, Molecular Layer; IGL, Internal Granular Layer.
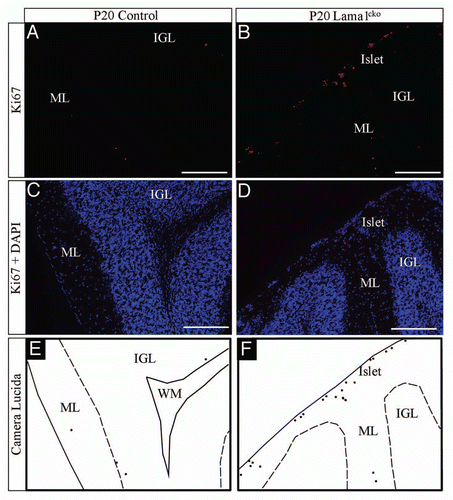
Figure 8 Decreased survival of GC in P20 Lama1cko animals. Coronal sections of P20 control (A) and Lama1cko (B) cerebella stained with BrdU antibody to reveal number of surviving postmitotic P7-BrdU labeled GC. (C) Quantification of BrdU positive cells in the IGL. In spite of increased proliferation at P7 in Lama1cko mice (leading to increased BrdU uptake) a similar number of BrdU positive cells is quantified in both genotypes at P20 thereby suggesting decreased survival of GC in Lama1cko mice. Scale bar = 100 µm. ML, Molecular Layer, IGL, Internal Granular Layer
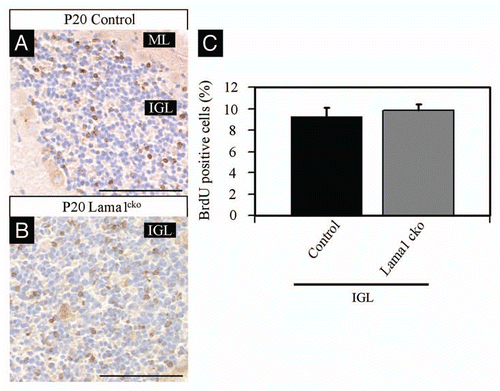
Figure 9 Increased apoptosis in P20 Lama1cko animals. Coronal sections of P20 control (A) and Lama1cko (B) cerebella stained with cleaved caspase-3 antibody to reveal apoptotic cells. (C) Quantification of caspase-3 positive cells in the IGL (**p < 0.01). Scale bar: 100 µm. ML, Molecular Layer; IGL, Internal Granular Layer.
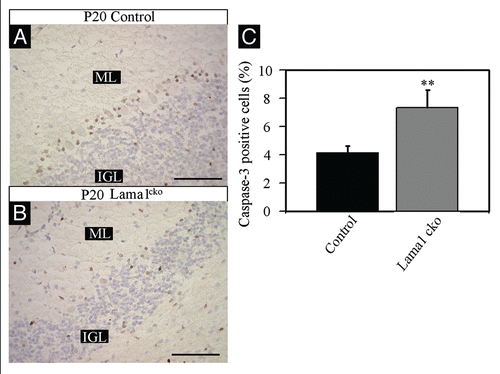
Figure 10 Abnormal Bergmann glia network in P20 Lama1cko animals. Coronal sections of P20 control (A, C and E) and Lama1cko (B, D and F) cerebella stained with DAPI to reveal cellular organization (A and B), GFAP antibody to reveal glial fibers (green color) and pan-laminin antibody to reveal the basement membrane (red color) (C–F). Scale bar: 100 µm. ML, Molecular Layer; IGL, Internal Granular Layer.
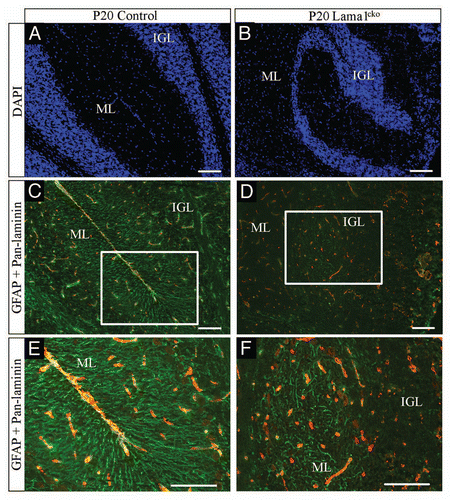
Acknowledgments
We thank Lydia Sorokin (University of Münster, Germany) for providing the laminin α1 antibody.
References
- Yurchenco PD, Patton BL. Developmental and pathogenic mechanisms of basement membrane assembly. Curr Pharm Des 2009; 15:1277 - 1294; PMID: 19355968; http://dx.doi.org/10.2174/138161209787846766
- Miner JH. Organogenesis of the kidney glomerulus: focus on the glomerular basement membrane. Organogenesis 2011; 7:75 - 82; PMID: 21519194; http://dx.doi.org/10.4161/org.7.2.15275
- Guess CM, Quaranta V. Defining the role of laminin-332 in carcinoma. Matrix Biol 2009; 28:445 - 455; PMID: 19686849; http://dx.doi.org/10.1016/j.matbio.2009.07.008
- Falk M, Ferletta M, Forsberg E, Ekblom P. Restricted distribution of laminin alpha1 chain in normal adult mouse tissues. Matrix Biol 1999; 18:557 - 568; PMID: 10607917; http://dx.doi.org/10.1016/S0945-053X(99)00047-5
- Miner JH, Li C, Mudd JL, Go G, Sutherland AE. Compositional and structural requirements for laminin and basement membranes during mouse embryo implantation and gastrulation. Development 2004; 131:2247 - 2256; PMID: 15102706; http://dx.doi.org/10.1242/dev.01112
- Kuratomi Y, Nomizu M, Nielsen PK, Tanaka K, Song SY, Kleinman HK, et al. Identification of metastasis-promoting sequences in the mouse laminin alpha1 chain. Exp Cell Res 1999; 249:386 - 395; PMID: 10366438; http://dx.doi.org/10.1006/excr.1999.4497
- Simon-Assmann P, Orend G, Mammadova-Bach E, Spenlé C, Lefebvre O. Role of laminins in physiological and pathological angiogenesis. Int J Dev Biol 2011; 55:455 - 465; PMID: 21858771; http://dx.doi.org/10.1387/ijdb.103223ps
- Alpy F, Jivkov I, Sorokin L, Klein A, Arnold C, Huss Y, et al. Generation of a conditionally null allele of the laminin alpha1 gene. Genesis 2005; 43:59 - 70; PMID: 16100707; http://dx.doi.org/10.1002/gene.20154
- Schéele S, Falk M, Franzén A, Ellin F, Ferletta M, Lonai P, et al. Laminin alpha1 globular domains 4–5 induce fetal development but are not vital for embryonic basement membrane assembly. Proc Natl Acad Sci USA 2005; 102:1502 - 1506; PMID: 15668394; http://dx.doi.org/10.1073/pnas.0405095102
- Edwards MM, Mammadova-Bach E, Alpy F, Klein A, Hicks WL, Roux M, et al. Mutations in Lama1 disrupt retinal vascular development and inner limiting membrane formation. J Biol Chem 2010; 285:7697 - 7711; PMID: 20048158; http://dx.doi.org/10.1074/jbc.M109.069575
- Colognato H, ffrench-Constant C, Feltri ML. Human diseases reveal novel roles for neural laminins. Trends Neurosci 2005; 28:480 - 486; PMID: 16043237; http://dx.doi.org/10.1016/j.tins.2005.07.004
- Sorokin LM, Conzelmann S, Ekblom P, Battaglia C, Aumailley M, Timpl R. Monoclonal antibodies against laminin A chain fragment E3 and their effects on binding to cells and proteoglycan and on kidney development. Exp Cell Res 1992; 201:137 - 144; PMID: 1612119; http://dx.doi.org/10.1016/0014-4827(92)90357-E
- Simo P, Simon-Assmann P, Bouziges F, Leberquier C, Kedinger M, Ekblom P, et al. Changes in the expression of laminin during intestinal development. Development 1991; 112:477 - 487; PMID: 1794317
- De Arcangelis A, Neuville P, Boukamel R, Lefebvre O, Kedinger M, Simon-Assmann P. Inhibition of laminin alpha1-chain expression leads to alteration of basement membrane assembly and cell differentiation. J Cell Biol 1996; 133:417 - 430; PMID: 8609173; http://dx.doi.org/10.1083/jcb.133.2.417
- Urruticoechea A, Smith IE, Dowsett M. Proliferation marker Ki-67 in early breast cancer. J Clin Oncol 2005; 23:7212 - 7220; PMID: 16192605; http://dx.doi.org/10.1200/JCO.2005.07.501
- Altman J. Postnatal development of the cerebellar cortex in the rat. I. The external germinal layer and the transitional molecular layer. J Comp Neurol 1972; 145:353 - 397; PMID: 4113154; http://dx.doi.org/10.1002/cne.901450305
- Li JY, Langford LA, Adesina A, Bodhireddy SR, Wang M, Fuller GN. The high mitotic count detected by phospho-histone H3 immunostain does not alter the benign behavior of angiocentric glioma. Brain Tumor Pathol 2011; PMID: 21892765; http://dx.doi.org/10.1007/s10014-011-0062-0
- Hoogland TM, Kuhn B. Recent developments in the understanding of astrocyte function in the cerebellum in vivo. Cerebellum 2010; 9:264 - 271; PMID: 19904577; http://dx.doi.org/10.1007/s12311-009-0139-z
- Turck N, Lefebvre O, Gross I, Gendry P, Kedinger M, Simon-Assmann P, et al. Effect of laminin-1 on intestinal cell differentiation involves inhibition of nuclear nucleolin. J Cell Physiol 2006; 206:545 - 555; PMID: 16245305; http://dx.doi.org/10.1002/jcp.20501
- Weir ML, Oppizzi ML, Henry MD, Onishi A, Campbell KP, Bissell MJ, et al. Dystroglycan loss disrupts polarity and beta-casein induction in mammary epithelial cells by perturbing laminin anchoring. J Cell Sci 2006; 119:4047 - 4058; PMID: 16968749; http://dx.doi.org/10.1242/jcs.03103
- Satz JS, Barresi R, Durbeej M, Willer T, Turner A, Moore SA, et al. Brain and eye malformations resembling Walker-Warburg syndrome are recapitulated in mice by dystroglycan deletion in the epiblast. J Neurosci 2008; 28:10567 - 10575; PMID: 18923033; http://dx.doi.org/10.1523/JNEUROSCI.2457-08.2008
- Li X, Zhang P, Yang Y, Xiong Y, Qi Y, Hu H. Differentiation and developmental origin of cerebellar granule neuron ectopia in protein O-mannose UDPN-acetylglucosaminyl transferase 1 knockout mice. Neuroscience 2008; 152:391 - 406; PMID: 18295407; http://dx.doi.org/10.1016/j.neuroscience.2007.06.041
- Michele DE, Barresi R, Kanagawa M, Saito F, Cohn RD, Satz JS, et al. Post-translational disruption of dystroglycan-ligand interactions in congenital muscular dystrophies. Nature 2002; 418:417 - 422; PMID: 12140558; http://dx.doi.org/10.1038/nature00837
- Edwards MM, Mammadova-Bach E, Alpy F, Klein A, Hicks WL, Roux M, et al. Mutations in Lama1 disrupt retinal vascular development and inner limiting membrane formation. J Biol Chem 2010; 285:7697 - 7711; PMID: 20048158; http://dx.doi.org/10.1074/jbc.M109.069575
- Yamada K, Watanabe M. Cytodifferentiation of Bergmann glia and its relationship with Purkinje cells. Anat Sci Int 2002; 77:94 - 108; PMID: 12418089; http://dx.doi.org/10.1046/j.0022-7722.2002.00021.x
- Lom B, Hockberger PE. Is laminin-1 a guidance cue for cerebellar granule cell migration?. J Neurobiol 1997; 33:72 - 84; PMID: 9212071; http://dx.doi.org/10.1002/(SICI)1097-4695(199707)33:1<72::AIDNEU7>3.0.CO;2-A
- Gupta SK, Meiri KF, Mahfooz K, Bharti U, Mani S. Coordination between extrinsic extracellular matrix cues and intrinsic responses to orient the centrosome in polarizing cerebellar granule neurons. J Neurosci 2010; 30:2755 - 2766; PMID: 20164359; http://dx.doi.org/10.1523/JNEUROSCI.4218-09.2010
- Ichikawa-Tomikawa N, Ogawa J, Douet V, Xu Z, Kamikubo Y, Sakurai T, et al. Laminin α1 is essential for mouse cerebellar development. Matrix Biol 2011; In press PMID: 21983115; http://dx.doi.org/10.1016/j.matbio.2011.09.002
- Najmabadi H, Hu H, Garshasbi M, Zemojtel T, Abedini SS, Chen W, et al. Deep sequencing reveals 50 novel genes for recessive cognitive disorders. Nature 2011; 478:57 - 63; PMID: 21937992; http://dx.doi.org/10.1038/nature10423