Abstract
Histone deacetylases (HDACs) play important roles in the epigenetic control of development, and aberrant expression of HDACs has been implicated in human diseases including cancer. Among the mammalian HDACs, HDAC1 has been extensively studied, but its role in exocrine pancreatic morphogenesis and cancer is still poorly understood. The goal of this study is to determine the functional role of HDAC1 in normal development of exocrine pancreas using zebrafish as the model organism as well as in human pancreatic adenocarcinoma. The zebrafish germline loss-of-function mutation hdac1hi1618 caused impaired cell cycle progression in pancreatic epithelia, resulting in growth arrest and dysmorphogenesis of exocrine pancreas. In human pancreatic adenocarcinoma tissues and cell lines, HDAC1 was expressed at variably elevated levels. RNA interference-induced silencing of HDAC1 diminished proliferation of the cancer cells and cell cycle progression. The proliferative arrest in the developing exocrine pancreas and pancreatic cancer cells was associated with up-regulated expression of the cyclin-dependent kinase inhibitors and the sonic hedgehog signaling components. This study indicates that HDAC1 is required for pancreatic epithelial proliferation in development and cancer. We hypothesize that aberrant expression of HDAC1 modulates the developmental and signaling pathways in exocrine pancreatic epithelia and consequently the genes required for cellular proliferation during development and progression of pancreatic neoplasia.
Introduction
Epigenetic mechanisms such as histone modification and DNA methylation play crucial roles in normal and cancerous development. Histone deacetylases (HDACs) regulate gene transcription by removing acetyl group from nucleosomal histones and non-histone protein, restricting the access of transcription factors or repressors to the upstream regulatory sequences.Citation1 Among the various HDACs, the cellular and biochemical functions of HDAC1 are relatively well characterized. Studies of several vertebrate and non-vertebrate organisms have indicated an essential role of HDAC1 in early embryonic development and organogenesis.Citation2 Moreover, HDAC1 is overexpressed in human cancers such as gastric carcinoma, prostate carcinoma and breast carcinoma.Citation3–Citation7 Studies using small interfering RNA (siRNA) indicate a role of HDAC1 in proliferation of tumor cells.Citation8–Citation10 However, the functional role of HDAC1 in the developmental processes and neoplastic growth of exocrine pancreas remains to be determined. Understanding the role of HDAC1 in exocrine pancreas during morphogenesis is expected to help elucidate the epigenetic control of growth in human pancreatic cancer and its response to therapy.
Accumulating evidence has identified the genetic elements with crucial roles in exocrine pancreatic development, but little is known about its epigenetic control. In zebrafish, pancreatic progenitors migrate and aggregate to form the anlage, which develop into acini and ducts by epithelial proliferation, differentiation and migration, and these processes require the transcriptional activities of pancreas-specific transcription factor 1a (Ptf1a) and pancreas/duodenum homeobox protein 1 (Pdx1).Citation11–Citation15 Exocrine pancreatic epithelial proliferation is coordinately regulated with acinar and ductal branching morphogenesis, as demonstrated with the slimjim (slj) mutation that impairs the activity of RNA polymerase III,Citation15–Citation17 and the sweetbread (swd) mutation that affects transient receptor potential ion channel Trpm7.Citation15,Citation17,Citation18 Recent studies have also begun to reveal the epigenetic control of development of exocrine pancreas by DNA methylation, as loss-of-function mutation in the ducttrip locus-encoded S-adenosyl-homocysteine hydrolase that regulates cellular methionine homeostasis disrupts acinar and ductal morphogenesis.Citation15,Citation17,Citation19 How Hdacs, particularly Hdac1, influence epithelial proliferation, acinar and ductal cytodifferentiation and morphogenesis of exocrine pancreas remain to be explored.
Besides, the signaling pathways that play important roles in pancreatic organogenesis also contribute to the initiation and progression of pancreatic cancer.Citation14,Citation17,Citation18,Citation20 These include Sonic hedgehog (SHH), Notch, wingless-type mouse mammary tumor virus integration site family members (WNT), epidermal growth factor (EGF), transforming growth factor β (TGFβ), fibroblast growth factor (FGF) and TRPM7. The role of epigenetic mechanisms—particularly DNA methylation and histone acetylation—underlying the pathogenesis of pancreatic neoplasia has been examined.Citation21 Studies of human pancreatic adenocarcinoma cells using HDAC inhibitors suggest important roles of HDACs in cell division, growth and survival.Citation22–Citation29 However, the role of HDACs, particularly HDAC1, in pancreatic tumorigenesis and how they influence the expression of developmental regulators such as the SHH pathway in pancreatic cancer cells remain to be determined.
This study focuses on defining the role of HDAC1 in exocrine pancreatic development and cancer. Deficiency of Hdac1 in the developing zebrafish or targeted inhibition of HDAC1 expression in human pancreatic adenocarcinoma cells impairs cell cycle progression from G1 to S phase and diminishes epithelial proliferation. These effects are associated with elevated protein levels of acetylated histones H3 and H4, and increased mRNA levels of the cyclin-dependent kinase (CDK) inhibitors and the SHH signaling components. Results of this study indicate that HDAC1 is required for pancreatic epithelial proliferation and modulation of developmental pathways including SHH. These data help elucidate the epigenetic control of exocrine pancreatic morphogenesis and oncogenesis, and provide support for the combination of inhibitors of HDACs and SHH signaling as a therapeutic approach in pancreatic cancer.
Results
Hdac1 is required for epithelial proliferation and morphogenesis in exocrine pancreas by modulating histone acetylation and expression of developmental pathways.
We studied the epigenetic mechanism for regulation of exocrine pancreas development by initially focusing on the role of Hdac1 using zebrafish as the vertebrate model. The zebrafish mutant hdac1hi1618 carries a retroviral insertion-induced loss-of-function mutation in the gene encoding Hdac1, such that hdac1 is not expressed in the hdac1hi1618 mutants, and thus hdac1hi1618 is a null allele.Citation31,Citation32 We aimed to determine the role of Hdac1 in development of exocrine pancreas by analysis of the hdac1hi1618 mutants and by disrupting expression of hdac1 in wild-type (WT) zebrafish larvae.
In WT larvae, the exocrine pancreas expressing mRNA of the digestive enzyme trypsin was initially detected around 48 hour-post-fertilization (hpf), and it expanded over the next 72 h and became mature by 5 day-post-fertilization (dpf) (). In the hdac1hi1618 mutant, trypsin immunoreactivity was undetectable until 72 hpf, and the region that expressed trypsin remained relatively small over the next 48 h (). Using ptf1a as a marker of exocrine pancreatic progenitors, we showed that the expression pattern and levels of ptf1a is similar to that of trypsin in the hdac1hi1618 mutants (data not shown). The disrupted growth of exocrine pancreas in the hdac1hi1618 mutant is further supported by diminished immunoreactivity for another digestive enzyme carboxypeptidase A at 72 hpf (). As compared to the WT siblings, the exocrine pancreas of the hdac1hi1618 mutant appears dysmorphic (). The pancreatic epithelia in the hdac1hi1618 mutant lack the characteristic arrangement of cells as acini. In the hdac1hi1618 mutants, the pancreatic ducts as revealed by cytokeratin immunoreactivity could not be detected, whereas the pancreatic islets are essentially normal as indicated by insulin and glucagon immunoreactivity (data not shown). Of note, the hdac1hi1618 mutants are relatively small and dysmorphic, such that the pancreatic defect is not highly specific. However, these data indicate that deficiency of Hdac1 causes disruption of growth in exocrine pancreas, suggesting a primary defect in epithelial proliferation.
Next, we assayed for cellular proliferation by counting the cell number and by using 5-bromo-2′-deoxyuridine (BrdU) as a marker of cells in the S phase of the cell cycle. In the hdac1hi1618 mutants, the number of epithelia in the exocrine pancreas is greatly diminished, and the proportion of S-phase cells is significantly reduced (). There is no increased apoptotic cell death in the exocrine pancreas of the hdac1hi1618 mutants using Apoptag® assay (data not shown). To monitor the function of Hdac1, the expression levels of acetylated histones H3 and H4 were then analyzed, as most of this biochemical process involves the lysine residues at their amino termini.Citation33 Immunoblot analysis showed that the relative levels of acetylated histones H3 and H4 were increased in the hdac1hi1618 mutants at 72 hpf by 66 and 56%, respectively (). As compared with that in WT at 48 hpf, the relative level of acetylated histone H3 in the hdac1hi1618 mutants at 72 hpf was increased by 130% (Sup. Fig. 1).
The defect of exocrine pancreas in the hdac1hi1618 mutants could be recapitulated by anti-sense knock down of hdac1 with MOs in WT larvae. The hdac1-morphants developed relatively small exocrine pancreas as indicated by immunoreactivity against trypsin mRNA (), and the epithelia lacked the acinar morphology that is typical of WT (). The levels of acetylated histones H3 and H4 were increased by 180% and 190%, respectively, in the hdac1-MO-injected larvae (). This evidence is consistent with the data obtained by analyses of the hdac1hi1618 mutants, and it helps support the requirement of Hdac1 for growth and morphogenesis of exocrine pancreas.
Acetylation of nucleosomal histones has been shown to influence gene expression by modifying access of specific promoter sequences in the genome to transcriptional activators and repressors.Citation1 Using real-time PCR, we demonstrated increased mRNA level of the cdk inhibitor p21cdkn1a in the hdac1hi1618 mutants at 48 hpf by 150%, while the difference in p21cdkn1a expression between the hdac1hi1618 and WT at 72 hpf is noteworthy (). Furthermore, we examined the gene expression profiles of hdac1hi1618 mutants as compared with their WT siblings at 48 hpf. Consistent with the data shown in , the mRNA levels of exocrine pancreatic enzymes (trypsin and carboxypeptidase A) as well as ptf1a that is expressed in both progenitor cells and acinar cells of exocrine pancreas are downregulated in the hdac1hi1618 mutants (). Expression of the major signaling pathways with known functions in the development of exocrine pancreas, including Shh, Notch, Wnt, Tgfβ and Fgf are variably altered in the hdac1hi1618 mutants ().
In a recent study, we have demonstrated that the clinical HDAC inhibitor suberoylanilide hydroxamic acid (SAHA) upregulates the expression of hedgehog interacting protein (HHIP), a competitive antagonist of hedgehog at the Patched-1 receptor.Citation29 In the zebrafish hdac1hi1618 mutants, the hhip mRNA level is elevated by 160% as compared to that in the WT siblings (). This is verified by realtime PCR, which shows that expression of hhip mRNA is significantly upregulated in the hdac1hi1618 mutants at both 48 hpf and 72 hpf by 160 and 110%, respectively (). In our previous study, Polr3 activity including transcription of tRNA has been shown to be selectively critical for the development of exocrine pancreas.Citation16 Of note, the zebrafish hdac1hi1618 mutation causes upregulation of certain subunits of polr3 and tRNA synthetases for various amino acids ().
Therefore, loss-of-function of Hdac1 causes severe reduction of proliferative ability in the pancreatic epithelia, leading to failure of acinar morphogenesis and arrested growth of exocrine pancreas. These effects are associated with increased level of acetylated nucleosomal histones and upregulated expression of p21cdkn1a and hhip as well as modulated expression of various developmental pathways. These data indicate that during early development, Hdac1 is required for exocrine pancreatic epithelial proliferation that is regulated in coordination with acinar and ductal morphogenesis.
Expression of HDAC1 is variably elevated in human pancreatic adenocarcinoma, and HDAC1 contributes to uncontrolled proliferation the cancer cells.
Next, we determined the expression and role of HDAC1 in human pancreatic adenocarcinoma, which is the most common type of cancer in exocrine pancreas. There is an overall increased nuclear immunoreactivity against HDAC1 protein in pancreatic ductal adenocarcinoma as compared with normal pancreatic ducts ( and B; Sup. Fig. 2). The levels of HDAC1 mRNA in the human pancreatic adenocarcinoma cell lines BxPC-3 and PANC-1 are significantly elevated, relative to that in the immortalized but non-cancerous pancreatic epithelia H6c7 cells (). Therefore, HDAC1 is variably overexpressed in pancreatic adenocarcinoma, suggesting a contributory role of HDAC1 in pancreatic tumorigenesis.
To investigate the functional role of HDAC1 in pancreatic adenocarcinoma, we used target-specific siRNA to knock down expression of HDAC1. In the three tested cell lines including Panc 02.03, PANC-1 and BxPC-3, more than 90% HDAC1 mRNA expression was reduced as quantified by real-time PCR. Morphological examination revealed that the HDAC1-deficient cells are enlarged, flattened and irregularly shaped, and they contained micronuclei and cytoplasmic vacuoles (). The cellular morphology of the HDAC-1 deficient cells suggests that HDAC1 is required for preventing the cancer cells from entering a state of irreversible cell division arrest, such as mitotic catastrophe and cellular senescence.Citation34
In agreement with the morphological changes, proliferation of the HDAC-1 deficient Panc 02.03 cells was significantly reduced by 32% as determined by colorimetric absorbance using MTS as a metabolic indicator. This result was confirmed by determining the number of cells, as anti-HDAC1 siRNA significantly reduced proliferation by 18% (). HDAC1-deficient PANC-1 and BxPC-3 cells exhibited reduced proliferation by 4 and 29%, respectively, as determined by colorimetric absorbance using MTS. To determine the requirement of HDAC1 for cell cycle progression, the DNA content of PaCa 02.03 incubated in the presence of anti-HDAC1 siRNA or control siRNA was analyzed by flow cytometry. SiRNA-induced knock down of HDAC1 expression increased the proportion of cells in G0/G1 phases by 11% and reduced the proportion of cells in both S and G2/M phases by 11% ().
As in the zebrafish larvae with loss-of-function mutation in hdac1 () or knock down of hdac1 (), the protein levels of both acetylated histones H3 and H4 were elevated in the HDAC1-deficient pancreatic adenocarcinoma cells by 120% (). Consistent with the effect on cell cycle progression, the levels of the CDK inhibitors including p21CDKN1A and p27CDKN1B mRNA in the HDAC1-deficient cells were significantly increased by 81 and 46%, respectively, as compared with the cells treated with control siRNA (). No significant difference in the proportion of apoptotic cells as determined by flow cytometry, or the level of BAX protein by immunoblotting, was found between the anti-HDAC1 siRNA-treated cells and control (data not shown).
To gain insights into the mechanism underlying the role of HDAC1, we examined expression of the developmental pathways with an initial focus on SHH. SHH signaling plays important roles in regulating proliferation of pancreatic adenocarcinoma cells,Citation35 and expression of the SHH signaling components has been shown to be modulated by the HDAC inhibitor SAHA.Citation29 Here, we determined the effect of anti-HDAC1 siRNA on the expression of HHIP, and that of smoothened (SMO) that mediates SHH signaling. In Panc 02.03 cells treated with siRNA directed against HDAC1, the mRNA levels of both HHIP and SMO were significantly elevated by 150 and 78%, respectively, as determined by semi-quantitative PCR ().
Taken together, expression of HDAC1 is generally upregulated in human pancreatic adeno-carcinoma, and HDAC1-deficient cells exhibit senescence-like phenotype with reduced ability to progress through the cell cycle and to proliferate. These effects are associated with hyperacetylation of nucleosomal histones and upregulated expression of the cell cycle inhibitors and SHH signaling components. This study suggests that overexpression of HDAC1 contributes to the uncontrolled proliferation of pancreatic cancer cells during tumorigenesis.
Discussion
Epigenetic mechanisms such as histone acetylation control transcription of genes that play crucial roles in embryonic and cancerous development. How acetylation of nucleosomal histones influences exocrine pancreatic organogenesis and tumorigenesis is largely unexplored. In this study, we provide evidence that HDAC1 is required for epithelial cell division that is linked with pancreatic acinar and ductal morphogenesis in zebrafish, and for cell cycle progression and proliferation of human pancreatic adenocarcinoma cells. HDAC1 mediates these functions through modulated acetylation of histones H3 and H4 as well as expression of CDK inhibitors and the SHH pathway components. These data indicate that HDAC1 plays a proliferative role in organogenesis and tumorigenesis of exocrine pancreas, and help provide insights into the interaction between HDACs and SHH that has important implications for treatment of pancreatic cancer.
Hdac1 as a developmental regulator of exocrine pancreas.
Results of this study establish a crucial role of Hdac1 in regulating epithelial proliferation in exocrine pancreas, as indicated by the impaired cell cycle progression of exocrine pancreatic epithelia and dysmorphogenesis in the hdac1hi1618 mutants. Recent reports have also examined the role of Hdac1 in zebrafish pancreas. Farooq et al. showed that anti-sense knock down of hdac1 diminishes expression of the pancreatic enzyme elastase B on 5 dpf. Similarly, Noel et al. presented evidence that expression of trypsin is reduced in the zebrafish larvae carrying the hdac1s436 null allele. Results of our study are in agreement with those findings that Hdac1 is important for regional specification and cytodifferentiation, and for proliferation of exocrine pancreatic epithelia. Our data are also consistent with the studies in mice by genetic disruption of Hdac1 that suggest a proliferative role of Hdac1 during early embryonic development.Citation38,Citation39 Functional characterization of the target genes of Hdac1 including the various developmental pathways and other molecules as revealed by transcriptional profiling of the hdac1hi1618 mutants ( and Sup. Table 1), will be important for understanding the epigenetic mechanisms that regulate organogenesis of exocrine pancreas and providing insights into the mechanisms that mediate malignant transformation of pancreatic epithelia.
The finding of upregulated expression of hhip in the hdac1hi1618 mutants may help shed light into the role of Shh signaling in exocrine pancreatic morphogenesis. Cunliffe demonstrated that knockdown of hdac1 expression prevents responsiveness to Shh-induced neuronal formation.Citation40 This finding is in agreement with our data showing that hhip expression is upregulated in the hdac1hi1618 mutants. We hypothesize that during embryogenesis, Hdac1 is required to repress hhip in order to facilitate the pleiotropic actions of Shh, such as morphogenesis of exocrine pancreas and central nervous system. On the other hand, diIorio et al. provided evidence that supports requirement of Shh in the development of pancreatic islet but not exocrine pancreas.Citation41 Although it remains to be confirmed by directly testing the role of Shh in the hdac1hi1618 phenotype, it is likely that additional pathways and complex interactions with Shh signaling are involved in the morphogenesis of exocrine pancreas.
HDAC1 contributes to uncontrolled proliferation of human pancreatic adenocarcinoma cells.
In agreement with the notion that the developmental pathways are aberrantly involved in tumor growth, our data using human pancreatic adenocarcinoma tissues and cell lines along with those findings in a recent report in reference Citation42, suggest that HDAC1 contributes to the uncontrolled proliferation during pancreatic tumorigenesis. This is supported by the observation that the level of HDAC1 increases through progressive stages of benign pancreatic intraepithelial neoplasia (PanINs) and intraductal papillary mucinous neoplasia to a peak in pancreatic adenocarcinoma.Citation43 However, the mechanism underlying the upregulated expression of HDAC1 from ductal epithelia through PanINs to invasive adenocarcinoma remains to be determined.
Although PANC-1 and BxPC-3 express relatively high level of HDAC1 (), anti-HDAC1 siRNA produces considerable inhibition of proliferation in BxPC-3 and Panc 02.03 but relatively little effect on PANC-1. This is consistent with the fact that various cell lines of pancreatic adenocarcinoma respond variably to different cytotoxic agents such as gemcitabine, the HDAC inhibitor suberoylanilide hydroxamic acid, or the Smoothened antagonist SANT-1.Citation29 This is likely due to the unique profile of genetic mutations in each cancer cell lineCitation44 and factors other than HDAC1 also contributing to determine the proliferative capacity of the cancer cells.Citation42
It is interesting to note that high level of HDAC1 or its expression in association with either hypoxiainducible factor 1α (HIF-1α) or metastasis-associated protein 1 (MTA1) correlates with relatively low survival rate.Citation43,Citation45 Thus, HDAC1 is potentially a prognostic biomarker, in addition to being a therapeutic target, of pancreatic adenocarcinoma. Future studies by determining the role of HDAC1 in the development of PanINs and their progression to invasive adenocarcinoma using transgenic zebrafish or mice with K-Ras or p53 mutation are expected to generate insights into the mechanism underlying malignant transformation of pancreatic epithelia.
HDAC1-controlled expression of CDK inhibitors and SHH signaling.
The elevated expression of p21cdkn1a in the zebrafish hdac1hi1618 mutants and that of p21CDKN1A in the HDAC1-deficient human pancreatic cancer cells is in agreement with the established role of HDAC1 at the Sp1-binding site of the p21CDKN1A promoter.Citation46 It is noteworthy that p21cdkn1a is not listed in the transcriptional profile (Sup. Table 1). It is possible that the change of p21cdkn1a expression may be below the 1.5-fold threshold being used, or the p21cdkn1a cDNA is not present in the microarray being used for hybridization. In follow-up studies, we plan to directly examine the functional role of p21Cdkn1a and Hhip in the effect of Hdac1 on pancreatic epithelial proliferation by gain-of-function experiments. However, interpretation of the results of these planned experiments is expected to be complicated by the fact that complex interacting mechanisms among multiple pathways are likely involved.
The upregulated expression of HHIP and SMO by RNA interference-mediated silencing of HDAC1 suggests that HDAC1 plays a repressive role at the promoters of these genes. It is interesting to note that the HHIP promoter was found to be aberrantly methylated in pancreatic tumors with diminished HHIP expression, which could be restored by an inhibitor of DNA methylation.Citation47 Of note, SMO mRNA is expressed in PANC-1, BxPC-3, and Panc 02.03 to variable extents, whereas HHIP mRNA is undetectable in those cell lines.Citation48
However, HDAC1-mediated transcriptional modulation of the SHH signaling components in pancreatic adenocarcinoma cells have therapeutic implications. Using a combination of small molecule inhibitors of HDACs and SMO, we have demonstrated enhanced cytotoxicity with augmented repression of the SHH pathway activity in human pancreatic adenocarcinoma.Citation29 These data are supported by the recent evidence that HDAC1 activates the effectors of the hedgehog (HH) pathway, glioma-associated oncogene protein (GLI), such that inhibition of HDAC1 abrogates HH-dependent growth of neural cells.Citation49 Therefore, we hypothesize that HDAC1 contributes to therapeutic resistance of pancreatic cancer cells by selective modulation of gene expression of the SHH pathway including HHIP and SMO, such that small molecules that inhibit the pro-oncogenic SMO, whose expression is upregulated by HDAC inhibitors, produces enhanced cytotoxicity in pancreatic adenocarcinoma.
Conclusion and prospective.
In this study, we presented genetic evidence indicating that Hdac1 is required for epithelial proliferation and acinar/ductal morphogenesis in exocrine pancreas through hypoacetylation of nucleosomal histones and downregulation of cdk inhibitors. These developmental events are recapitulated in human pancreatic adenocarcinoma cells, in which HDAC1 mediates cell cycle progression through modulation of the expression of CDK inhibitors and the SHH pathway components. Based on these results, we hypothesize that aberrant increase in the expression and/or function of HDAC1 alters developmental signaling including the SHH pathway and causes modulation of specific genes involved in cell cycle progression and cell survival, leading to development of PanINs and their progression to invasive adenocarcinoma. Moreover, our data provide support for utilization of the zebrafish as a model for genetic and chemical dissection of Hdac1-mediated pathways in exocrine pancreatic development, as well as generating new insights into the mechanism underlying the combination of small molecule inhibitors of HDACs and the SHH pathway for improving treatment response in pancreatic cancer.
Materials and Methods
Zebrafish stock.
Zebrafish husbandry is described in details in reference Citation50. WT zebrafish of the AB strain and the hdac1hi1618 mutant line were obtained from the Zebrafish International Resource Center (Portland, OR). To facilitate visualization of exocrine pancreas in the larvae, 1-phenyl-2-thiourea (PTU) (Sigma®) was added to the embryo medium (E3) at 0.003% to inhibit skin pigmentation as indicated.
Immunohistochemistry, in situ hybridization and histological analysis.
The procedures are described in details in reference Citation14, Citation15 and Citation18. For immunohistochemistry, rabbit anti-carboxypeptidase A (Cpa) antibodies (Rockland), mouse anti-5-bromo-2′-deoxyuridine (BrdU, Roche), rabbit anti-pan cadherin (Cad) antibodies (Sigma®) were used. For in situ hybridization, trypsin anti-sense riboprobes were generated as described in reference Citation14 and Citation15. Zebrafish larvae were examined under a stereo-dissecting microscope (Leica MZ16F) with bright light. Images were captured using a color digital camera and QCapture software (QImaging) and constructed using Adobe® Photoshop® 7.0. For histological analysis, zebrafish larvae were embedded in JB-4® Plus solution (Polysciences), and sectioned at 5 µm intervals using a microtome (Leica RM2255, Plymouth, MI). The sections were examined under compound microscope using bright light or fluorescence (Olympus BX51), and the images were acquired using a DP71 digital camera (Olympus), analyzed using DP Manager software (Olympus) and constructed using Adobe® Photoshop® 7.0.
Anti-sense inhibition of hdac1.
WT embryos were microinjected with morpholino antisense oligos (MOs) directed against zebrafish hdac1 (5′-TTG TTC CTT GAG AAC TCA GCG CCA T-3′) as described review in reference Citation40 or hdac1-5-mispair-MO (5′-TTc TTg CTT cAG AAC TgA cCG CCA T-3′) as control. The MOs were obtained from Gene Tools, LLC (Philomath, OR). Each MO was dissolved in water (Milli Q, Millipore™) at 1 mM and stored in aliquots at −80°C. Each MO stock solution was diluted with water to a final concentration of 0.45 mM. For each embryo, 0.45 pmole of MO in 1 nl containing 10% phenol red solution (Sigma®) was injected into the yolk sac at the onecell stage as described in reference Citation14.
Immunoblot analysis of zebrafish protein.
Total protein of zebrafish larvae or cultured cells was extracted using lysis buffer containing 63 mM Tris-HCl, pH 6.8, 10% glycerol, 5% β-mercaptoethanol, 3.5% sodium dodecyl sulfate, protease inhibitors (Roche Diagnostics) at 4°C for 1 h, and the supernatant was collected after centrifugation at 2 × 104 g for 10 min at 4°C. The protein concentration of the supernatant was quantified using the BCA Protein Assay Kit (Bio-Rad). For each sample, 20 µg protein was separated by electrophoresis using 4–12% BIS-TRIS gels (NuPage, Invitrogen™) and then transferred to a polyvinylidene difluoride membrane (Bio-Rad). The membrane was blocked with a buffer of 5% skim milk and 0.1% Tween® 20 (Sigma) for 1 h. Primary antibodies including rabbit anti-acetylated histone H3, rabbit anti-acetylated histone H4, rabbit anti-histone H3, rabbit anti-histone H4 (Upstate Biotechnology/Millipore™) and rabbit anti-human β-actin (Sigma®) were used according to the manufacturers' instructions. The membranes were washed with phosphate buffered saline with 0.1% Tween-20 (PBS-T) buffer at room temperature and incubated with goat anti-rabbit immunoglobulin G (IgG) conjugated with horseradish peroxidase (HRP) at 25°C for 1 h. The protein antigens were detected using SuperSignal West Pico chemiluminescent substrate (Pierce) and exposure to HyBlot CL film (Denville). The intensity of the protein bands was quantified using Adobe® Photoshop® 7.0. The relative intensity of acetylated histones H3 and H4 were compared with that of total histones H3 and H4, respectively.
Semi-quantitative real-time polymerase chain reaction (PCR) (zebrafish).
The procedures are essentially as described in reference Citation18. Briefly, total RNA isolated from twenty larvae of each experimental group was reverse transcribed and then amplified using real-time PCR quantification. The sequences of the primers used for analysis of p21cdkn1a and hhip mRNA are based on zebrafish cDNA sequences available at NCBI (accession numbers AL912410 and DQ177323, respectively) as follows:
p21cdkn1a: 5′-ATG CAG CTC CAG ACA GAT GA-3′, 5′-CGC AAA CAG ACC AAC ATC AC-3′
hhip: 5′-AAG AAC GGC CAC TGT ACA CC-3′; 5′-GCT CCA GGA TGC TGT CTT TC-3′
Glyceraldehyde-3-phosphate dehydrogenase (gapdh) was used as internal control to normalize for the amount of RNA in each sample. The primers used for amplification are based on gapdh cDNA sequence at the National Center of Biotechnology Information (NCBI) (accession number BC095386):
gapdh: 5′-GAT ACA CGG AGC ACC AGG TT-3′, 5′-CGT TGA GAG CAA TAC CAG CA-3′.
The conditions for quantitative PCR are as follows: 2 min at 50°C, 10 min at 95°C, followed by 40 cycles: 15 s at 95°C, 1 min at 60°C and 10 min at 72°C. Each sample was run in triplicate and each experiment performed three times. The p21cdkn1a, hhip and gapdh mRNA levels were analyzed. The mean ratio of p21cdkn1a or hhip mRNA to gapdh mRNA within the same sample was determined using the Comparative CT Method (Applied Biosystems®). Samples of PCR using primers directed against p21cdkn1a, hhip or gapdh were analyzed by agarose gel electrophoresis to validate specificity of amplification products.
Transcriptional profiling using cDNA microarray.
The procedures are essentially as described in reference Citation18. Briefly, total RNA was extracted from twenty hdac1hi1618 mutants or their WT siblings at 48 hpf, reversed transcribed, amplified and hybridized onto Affymetrix® Zebrafish arrays. The microarray data were analyzed using analysis of variance (ANOVA) with the Partek Genomic Suite Software.
Immunohistochemistry of human pancreatic adenocarcinoma tissues.
The procedures are essentially as described in reference Citation51. Briefly, human pancreatic adenocarcinoma and matched normal tissues were embedded in paraffin and obtained from the Tissue Procurement Core of the University of Iowa (with Institutional Review Board approval). The tissue sections were deparaffinized, processed for antigen retrieval by heating, incubated with rabbit polyclonal anti-HDAC1 antibodies (Upstate Biotechnology/Millipore™), followed by incubation with horseradish peroxidase-conjugated anti-rabbit antibodies. The signals were detected by brown color reaction using diaminobenzidine as substrate and the sections were counterstained with hematoxylin (blue). The images shown are representative of matched specimens from five patients.
Cell culture.
The human pancreatic adenocarcinoma cell lines BxPC-3, Capan-1, HPAF-II, MIA PaCa-2, Panc 02.03, PANC-1 and PL45 were obtained from the American Type Culture Collection (ATCC, Manassas, VA) and maintained according to the instructions provided from ATCC. The immortalized human pancreatic ductal epithelia H6c7 was generously provided by Dr. Ming-Sound Tsao (University of Toronto) and cultured as described in reference Citation52. All cell culture media were supplemented with 10% heat-inactivated fetal bovine serum (FBS, Hyclone®), 100 U/ml penicillin (Gibco™) and 100 µg/ml streptomycin (Gibco™). The cells were grown at 37°C in a humidified atmosphere containing 5% CO2. All experiments were performed using culture medium unless otherwise specified. The cells were used within twenty passages of the frozen stocks in liquid nitrogen.
RNA interference.
The procedures are essentially as described in reference Citation51. Briefly, Panc 02.03, PANC-1 and BxPC-3 cells were grown to 70–80% confluency, trypsinized and resuspended at 106 cells in 100 µl of Nucleofector® Solution (Amaxa®/Lonza) in a cuvette. The cells were mixed with 100 nM siRNA directed against human HDAC1 mRNA (M-003494, Dharmacon, Inc.). Transfection was performed by electrical pulsation using Nucleofector® (Amaxa®/Lonza, Cologne, Germany). For controls performed in parallel, 106 cells were transfected with or without 100 nM non-targeting control siRNA (D-001206; Dharmacon, Inc.). Immediately following transfection, 500 µl of pre-warmed (37°C) culture medium was added to each cuvette and the cells transferred to a six-well plate containing 1.5 ml prewarmed (37°C) culture medium. The siRNA-treated cells and controls were incubated at 37°C with 5% CO2 for 72 h. The cells were then analyzed by quantitative real-time PCR to verify knock down of HDAC1 mRNA.
Cellular morphology.
Panc 02.03 cells were transfected with anti-HDAC1 siRNA, non-targeting control siRNA, or no siRNA, plated in six-well tissue culture dishes at a density of 5 × 105 per well and incubated for 24 h. Images of the cells were captured under an inverted light microscope with phase contrast (Olympus IX81, Tokyo, Japan).
Proliferation assay.
Cellular proliferation was quantified using the CellTiter 96® AQueous One Solution Cell Proliferation Assay (Promega) using 3-(4,5-dimethylthiazol-2-yl)-5-(3-carboxymethoxy-phenyl)-2-(4-sulfonylphenyl)-2H-tetrazolium, inner salt (MTS) as described in reference Citation51. Briefly, 4 × 104 siRNA-transfected Panc 02.03, PANC-1 or BxPC-3 cells were seeded per well of a 96-well microtiter plate containing 100 µl of culture medium and incubated at 37°C for 72 h and fresh medium was replaced 48 h following cell seeding. Following incubation of the cells with the MTS dye, absorbance of the samples at 490 nm was measured and analyzed. The results of the MTS assay were confirmed by determining the number of viable cells using trypan blue exclusion.
Flow cytometric analysis of cell cycle.
Panc 02.03 cells were deprived of serum for 24 h and then transfected with anti-HDAC1 siRNA or non-specific control siRNA. The cells were analyzed for DNA content at 72 h post-transfection by fluorescence activated cell sorting (FACS) as described in reference Citation51. Non-specific sub-G0/G1 events were gated out of analyses, such that the cell cycle distribution curves could be accurately analyzed.
Immunoblot analysis of human acetylated histones.
The procedure is essentially as described above for immunoblotting of zebrafish protein. For human pancreatic adenocarcinoma cells, total protein was extracted by fixing the cells with 1% paraformaldehyde, then treated with glycine, washed with PBS and lysed using M-PER® Mammalian Protein Extraction Reagent (Thermo Scientific). The lysates were then sonicated on ice for 30 s, centrifuged at 2 × 104 g for 10 min at 4°C and the protein concentration of the supernatant was quantified using the BCA Protein Assay Kit (Bio-Rad).
Quantification of human HDAC1, p21CDKN1A, p27CDKN1B, HHIP and SMO mRNA.
For HDAC1, total RNA of each cell line, or the anti-HDAC1 siRNA-treated or control cells, was extracted and reverse transcribed as described in reference Citation51. The absolute level of HDAC1 mRNA was determined using standard curves. The sequences of the primers used for analysis of HDAC1 mRNA are based on cDNA sequences available at NCBI (BC000301) as follows:
HDAC1: 5′-GAA TCC GCA TGA CTC ATA AT-3′, 5′-TGG TAC TTG GTC ATC TCC TC-3′.
For p21CDKN1A, p27CDKN1B, HHIP and SMO, the anti-HDAC1 siRNA-treated or control cells, was extracted and reverse transcribed. The relative levels of p21CDKN1A, p27CDKN1B, HHIP and SMO mRNA were determined relative to GAPDH as described in reference Citation46. The sequences of the primers used are based on human cDNA sequences of p21CDKN1A, p27CDKN1B, HHIP, SMO and GAPDH (NCBI accession number NM_000389, BC001971, NM_022475, U84401, NM_002046, respectively):
p21CDKN1A: 5′-ATG CAG CTC CAG ACA GAT GA-3′, 5′-CGC AAA CAG ACC AAC ATC AC-3′
p27CDKN1B: 5′-TGA AGC CTG GAA CTT CGA CT-3′, 5′-TGT GAA TAT CGG AGC CCT TC-3′
HHIP: 5′-GCA GAG GAG ACC TCA GCA TC-3′, 5′-GCA GTT GTG CCA GTG TCA GT-3′
SMO: 5′-TCA CTC CCC TTT GTC CTC AC-3′, 5′-TGG TCT CGT TGA TCT TGC TG-3′
GAPDH: 5′-GAG TCA ACG GAT TTG GTC GT-3′, 5′-TTG ATT TTG GAG GGA TCT CG-3′.
Statistical analysis.
The significance of the difference between the experimental group and control was analyzed using the Student's t-test. Statistical significance was considered at a p value <0.05.
Abbreviations
ATCC | = | american type culture collection |
BrdU | = | 5-bromo-2′-deoxyuridine |
CDK | = | cyclin-dependent kinases |
Cpa | = | carboxypeptidase A |
dpf | = | day-post-fertilization |
EGF | = | epidermal growth factor |
FACS | = | fluorescence-activated cell sorting |
FBS | = | fetal bovine serum |
FGF | = | fibroblast growth factor |
GAPDH | = | glyceraldehyde-3-phosphate dehydrogenase |
GLI | = | glioma-associated oncogene protein |
HDACs | = | histone deacetylases |
HH | = | hedgehog |
HHIP | = | hedgehog-interacting protein |
hpf | = | hour-post-fertilization |
HRT | = | horseradish peroxidase |
IgG | = | immunoglobulin G |
MOs | = | morpholino antisense oligos |
MTS | = | 3-(4,5-dimethylthiazol-2-yl)-5-(3-carboxymethoxy-phenyl)-2-(4-sulfonylphenyl)-2H-tetrazolium, inner salt |
PBS-T | = | phosphate buffered saline with 0.1% tween-20 |
PCR | = | polymerase chain reaction |
Pdx1 | = | pancreas/duodenum homeobox protein 1 |
Ptf1a | = | pancreas-specific transcription factor 1a |
PTU | = | 1-phenyl-2-thiourea |
SAHA | = | suberoylanilide hydroxamic acid |
SHH | = | sonic hedgehog |
siRNA | = | small interfering RNA |
slj | = | slimjim |
SMO | = | smoothened |
swd | = | sweetbread |
TGF | = | transforming growth factor |
TRP | = | transient receptor potential |
WNT | = | wingless-type mouse mammary tumor virus integration site family members |
WT | = | wild type |
Figures and Tables
Figure 1 Zebrafish hdac1hi1618 mutation impairs exocrine pancreas development by reducing epithelial proliferation, perturbing morphogenesis and inducing acetylation of nucleosomal histones H3 and H4. (A) Exocrine pancreas (green arrow) by in situ hybridization using trypsin anti-sense riboprobes in hdac1hi1618 mutant larvae and WT siblings from 48 to 120 hpf. The images represent dorsal view of the larvae with exocrine pancreas located on the right side. (B) Acinar cytodifferentiation and morphology of exocrine pancreas in hdac1hi1618 mutant larvae and WT siblings at 72 hpf by immunohistochemistry using anti-Cpa antibodies (red) and anti-cadherin antibodies (green), respectively, followed by transverse histological sectioning. The nuclei were stained with DAPI (blue). e.p., exocrine pancreas; i, intestine. Note that, in the hdac1hi1618 mutant, the morphology of the intestine is grossly normal but relatively small. (C) Proportion of exocrine pancreatic epithelia in S phase of cell cycle in hdac1hi1618 mutant larvae and WT siblings at 72 hpf by immunohistochemistry using anti-BrdU antibodies, followed by transverse histological sectioning. The number (#) of DAPI+ nuclei, the number of BrdU+ nuclei (cells in S phase) and the proportion of BrdU+ nuclei are represented as the mean ± standard deviation. *p < 0.05 indicates statistical significance. Five larvae in each group were scored. (D) Immunoblot analysis of acetylated histones H3 and H4 in hdac1hi1618 mutants and WT siblings at 72 hpf. Anti-total histones H3 and H4 antibodies and anti-actin antibodies were used as control.
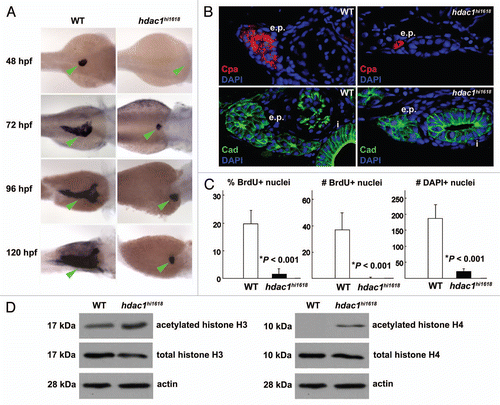
Figure 2 Anti-sense inhibition of hdac1 expression recapitulates defects of exocrine pancreas in hdac1hi1618 mutants. Exocrine pancreas of 72 hpf WT larvae injected with hdac1-MO or hdac1-5-mispair-MO analyzed by (A) in situ hybridization using trypsin anti-sense riboprobes; (B) by immunohistochemistry using anti-cadherin antibodies (green) and DAPI (blue) followed by histological sectioning. e.p., exocrine pancreas; i, intestine. Note that both exocrine pancreas and intestine are hypomorphic in the hdac1-MO-injected larvae. (C) Immunoblot analysis of acetylated histones H3 and H4 in hdac1-MO or control MO-injected WT larvae at 72 hpf. Anti-total histones H3 and H4 antibodies and anti-actin antibodies were used as controls.
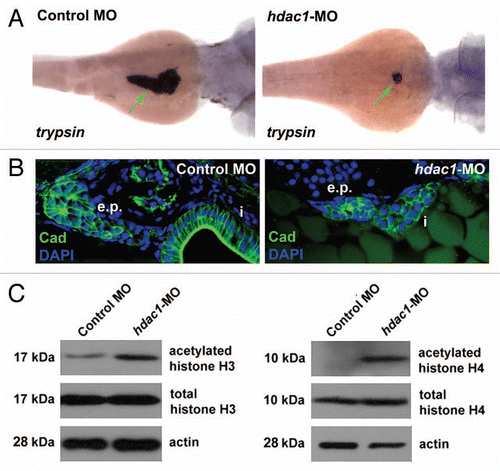
Figure 3 Expression of p21cdkna1 and hhip mRNA is upregulated in the hdac1hi1618 mutants. Semi-quantitative analysis of (A) p21cdkn1a and (B) hhip mRNA in hdac1hi1618 mutants and WT siblings at 48 hpf by real-time PCR using gapdh mRNA as internal control. Each column represents the mean relative value of mRNA level using RNA extracted from three independent groups of hdac1hi1618 mutant and WT larvae, with triplicates per sample for real-time PCR. Bars represent mean ± SD. *p < 0.05 indicates statistical significance.
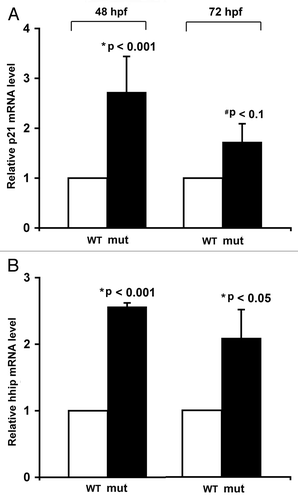
Figure 4 Expression of HDAC1 is upregulated in human pancreatic adenocarcinoma tissues and cells. (A and B) Immunohistochemical analysis of HDAC1 protein in normal pancreatic tissue and pancreatic adenocarcinoma obtained from different regions of the pancreas from the same patient. These images are representative of the normal-tumor pairs of pancreatic tissues from five patients. Red arrows point at the nuclei of the ductal cells. Pre-immune serum was used as control for specificity of anti-HDAC1 antibodies (data not shown). (C) Quantitative analysis of HDAC1 mRNA in human pancreatic adenocarcinoma cell lines by real-time PCR. Each value represents the relative HDAC1 mRNA level in the cell line as compared with that in H6c7. Each column represents the mean and the bars represent SD, as determined from three independent experiments. *Indicates statistically significant difference relative to H6c7.
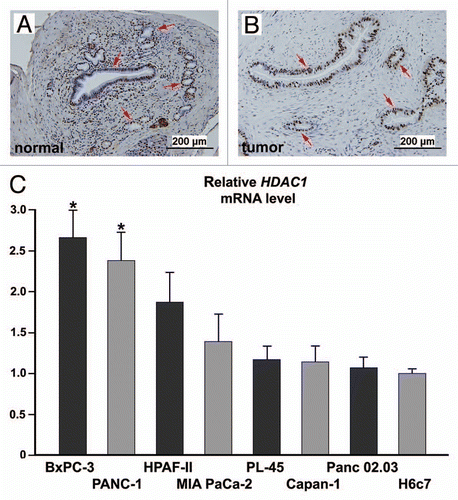
Figure 5 RNA interference-induced silencing of HDAC1 reduces proliferation of pancreatic adenocarcinoma cells by impairing cell cycle progression. Panc 02.03 cells treated with anti-HDAC1 siRNA, non-specific control siRNA or no siRNA were analyzed. (A) Bright field images with phase contrast at 24 h post-transfection. Multi-nucleated cells are indicated by arrows and cytoplasmic vacuoles by arrowheads. (B) Proliferation assays by MTS colorimetric absorbance and by counting viable cells. Columns and bars represent the mean and SD, respectively. (C) Cell cycle by FACS analysis of DNA content. The proportion of cells in each phase of the cell cycle is indicated. Non-specific sub-G0/G1 events were gated out of analyses, allowing for more accurate analysis of cell cycle distribution curves. These experiments were repeated with essentially the same results.
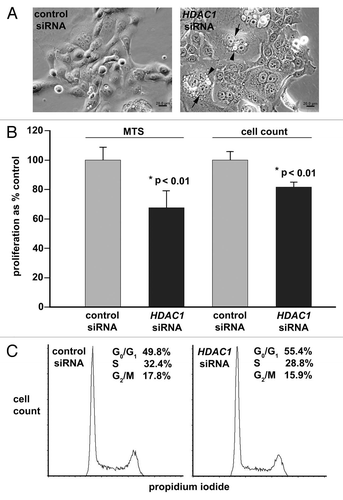
Figure 6 Targeted knock down of HDAC1 induces acetylation of nucleosomal histones and upregulates expression of CDK inhibitors and SHH signaling components. Panc 02.03 cells were transfected with anti-HDAC1 siRNA or non-targeting control siRNA. Total protein and RNA were extracted and analyzed at 48 h post-transfection. (A) Immunoblot analysis of acetylated histones H3 and H4 and total histones H3 and H4. The immunoblots are representative of two independent experiments with essentially the same results. (B) Semi-quantitative analysis of p21CDKN1A, p27CDKN1B, HHIP and SMO mRNA by real-time PCR. Columns and bars represent mean and SD, respectively for mRNA of each gene in the cells transfected with anti-HDAC1 siRNA as % of those in the control siRNA-transfected cells. *p < 0.05 indicates statistical significance.
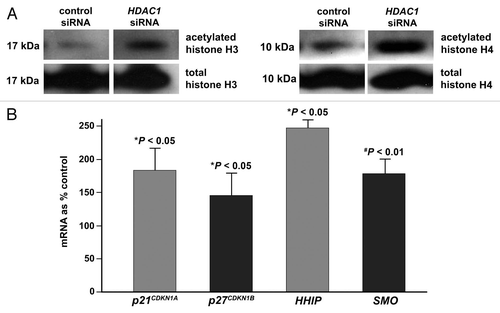
Table 1 Selected transcriptional profile of hdac1hi1618 mutants as compared to WT siblings using RNA extracted at 48 hpf
Additional material
Download Zip (622.3 KB)Acknowledgements
The authors wish to acknowledge the Tissue Procurement Core Facility, Comparative Pathology Laboratory, DNA Facility and Flow Cytometry Facility of the University of Iowa for technical support and equipments. This work is supported by the Pilot Grant in Translational Research by the Department of Internal Medicine of the University of Iowa Carver College of Medicine (N.S.Y.), the Holden Comprehensive Cancer Center Designated Gift Fund for pancreatic cancer research (N.S.Y.) and the Cancer Center Support Grant (P30 CA 086862) by the National Cancer Institute to the Holden Comprehensive Cancer Center of the University of Iowa (N.S.Y.). The Zebrafish International Resource Center is supported by grant P40 RR12546 from the NIH-NCRR.
References
- Haberland M, Montgomery RL, Olson EN. The many roles of histone deacetylases in development and physiology: implications for disease and therapy. Nat Rev Genet 2009; 10:32 - 42
- Brunmeir R, Lagger S, Seiser C. Histone deacetylase 1 and 2-controlled embryonic development and cell differentiation. Int J Dev Biol 2009; 53:275 - 289
- Choi JH, Kown HJ, Yoon BI, Kim JH, Han SU, Joo HJ, et al. Expression profile of histone deacetylase 1 in gastric cancer tissue. Jpn J Cancer Res 2001; 92:1300 - 1304
- Kim JH, Choi YK, Kwon HJ, Yang HK, Choi JH, Kim DY. Downregulation of gelsolin and retinoic acid receptor beta expression in gastric cancer tissues through histone deacetylase 1. J Gastroenterol Hepatol 2004; 19:218 - 224
- Halkidou K, Gaughan L, Cook S, Leung HY, Neal DE, Robson CN. Upregulation and nuclear recruitment to HDAC1 in hormone refractory prostate cancer. Prostate 2004; 59:177 - 189
- Krusche CA, Wulfing P, Kersting C, Vloet A, Bocker W, Kiesel L, et al. Histone deacetylase-1 and -3 protein expression in human breast cancer: a tissue microarray analysis. Breast Cancer Res Treat 2005; 90:15 - 23
- Nakagawa M, Oda Y, Eguchi T, Aishima SI, Yao T, Hosoi F, et al. Expression profile of class I histone deacetylases in human cancer tissues. Oncol Rep 2007; 18:769 - 774
- Glaser KB, Li J, Staver M, Ru-Qi W, Albert DH, Davidsen SK. Role of class I and class II histone deacetylases in carcinoma cells using siRNA. Biochem Biophys Res Commun 2003; 310:529 - 536
- Senese S, Zaragoza K, Minardi S, Muradore I, Ronzoni S, Passafaro A, et al. Role for histone deacetylase 1 in human tumor cell proliferation. Mol Cell Biol 2007; 27:4784 - 4795
- Haberland M, Johnson A, Mokalled MH, Montgomery RL, Olson EN. Genetic dissection of histone deacetylase requirement in tumor cells. Proc Natl Acad Sci USA 2009; 106:7751 - 7755
- Yee NS, Yusuff S, Pack M. Zebrafish pdx1 morphant displays defects in pancreas development and digestive organ chirality and potentially identifies a multipotent pancreas progenitor cell. Genesis 2001; 30:137 - 140
- Lin JW, Biankin AV, Horb ME, Ghosh B, Prasad NB, Yee NS, et al. Differential requirement for ptf1a in endocrine and exocrine lineages of developing zebrafish pancreas. Dev Biol 2004; 270:474 - 486
- Zecchin E, Mavropoulos A, Devos N, Filippi A, Tiso N, Meyer D, et al. Evolutionary conserved role of ptfla in the specification of exocrine pancreatic fates. Dev Biol 2004; 268:174 - 184
- Yee NS, Pack M. Zebrafish as a model for pancreatic cancer research. Meth Mol Med 2005; 130:273 - 298
- Yee NS, Lorent K, Pack M. Exocrine pancreas development in zebrafish. Dev Biol 2005; 284:84 - 101
- Yee NS, Gong W, Huang Y, Lorent K, Dolan AC, Maraia RJ, et al. Mutation of RNA pol III subunit rpc2/polr3b leads to deficiency of subunit rpc11 and disrupts zebrafish digestive development. PLoS Biol 2007; 5:2484 - 2492
- Yee NS. Han H, Grippo PJ. Zebrafish as a biological system for identifying and evaluating therapeutic targets and compounds. Drug discovery in pancreatic cancer 2010; New York Springer 95 - 112
- Yee NS, Zhou W, Liang IC. Transient receptor potential ion channel Trpm7 regulates exocrine pancreatic epithelial proliferation by Mg2+-sensitive Socs3a signaling in development and cancer. Dis Model Mech 2011; 4:240 - 254
- Matthews RP, Lorent K, Manoral-Mobias R, Huang Y, Gong W, Murray IVJ, et al. TNFα-dependent hepatic steatosis and liver degeneration caused by mutation of zebrafish s-adenosyl-homocysteine hydrolase. Development 2009; 136:865 - 875
- Koorstra JB, Hustinx SR, Offerhaus GJ, Maitra A. Pancreatic carcinogenesis. Pancreatology 2008; 8:110 - 125
- Omura N, Goggins M. Epigenetics and epigenetic alterations in pancreatic cancer. Int J Clin Exp Pathol 2009; 2:310 - 326
- Garcia-Morales P, Gomez-Martinez A, Carrato A, Martinez-Lacaci I, Barbera VM, Soto JL, et al. Histone deacetylase inhibitors induced caspase-independent apoptosis in human pancreatic adenocarcinoma cell lines. Mol Cancer Ther 2005; 4:1222 - 1230
- Ryu JK, Lee WJ, Lee KH, Hwang JH, Kim YT, Yoon YB, et al. SK-7041, a new histone deacetylase inhibitor, induces G2-M cell cycle arrest and apoptosis in pancreatic cancer cell lines. Cancer Lett 2006; 237:143 - 154
- Arnold NB, Arkus N, Gunn J, Korc M. The histone deacetylase inhibitor suberoylanilide hydroxamic acid induces growth inhibition and enhances gemcitabine-induced cell death in pancreatic cancer. Clin Cancer Res 2007; 13:18 - 26
- Kumagai T, Wakimoto N, Yin D, Gery S, Kawamata N, Takai N, et al. Histone deacetylase inhibitor, suberoylanilide hydroxamic acid (vorinostat, SAHA) profoundly inhibits the growth of human pancreatic cancer cells. Int J Cancer 2007; 121:656 - 665
- Haefner M, Bluethner T, Niederhagen T, Moebius C, Wittekind C, Mossner J, et al. Experimental treatment of pancreatic cancer with two novel histone deacetylase inhibitors. World J Gastroenterol 2008; 14:3681 - 3692
- Jones J, Bentas W, Blaheta RA, Makarevic J, Hudak L, Wedel S, et al. Modulation of adhesion and growth of colon and pancreatic cancer cells by the histone deacetylase inhibitor valproic acid. Int J Mol Med 2008; 22:293 - 299
- Pellizzaro C, Speranza A, Zorzet S, Crucil I, Sava G, Scarlata I, et al. Inhibition of human pancreatic cell line MIA PaCa2 proliferation by HA-but, a hyaluronic butyric ester: A preliminary report. Pancreas 2008; 36:15 - 23
- Chun SG, Zhou W, Yee NS. Combined targeting of histone deacetylases and hedgehog signaling produces enhanced cytotoxicity in pancreatic cancer. Cancer Biol Ther 2009; 8:1328 - 1339
- Amsterdam A, Burgess S, Golling G, Chen W, Sun Z, Townsend K, et al. A large-scale insertional mutagenesis screen in zebrafish. Genes Dev 1999; 13:2713 - 2724
- Golling G, Amsterdam A, Sun Z, Antonelli M, Maldonado E, Chen W, et al. Insertional mutagenesis in zebrafish rapidly identifies genes essential for early vertebrate development. Nat Genet 2002; 31:135 - 140
- Amsterdam A, Nissen RM, Sun Z, Swindell EC, Farrington S, Hopkins N. Identification of 315 genes essential for early zebrafish development. Proc Natl Acad Sci 2004; 101:12792 - 12797
- Kouzarides T, Berger SL. Allis CD, Jenuwein T, Reinberg D, Caparros ML. Chromatin modifications and their mechanism of action. Epigenetics 2007; New York Cold Spring Harbor Laboratory Press 196
- Okada H, Mak TW. Pathways of apoptotic and non-apoptotic death in tumour cells. Nat Rev Cancer 2004; 4:592 - 603
- Morton JP, Mongeau ME, Klimstra DS, Morris JP, Lee YC, Kawaguchi Y, et al. Sonic hedgehog acts at multiple stages during pancreatic tumorigenesis. Proc Natl Acad Sci 2006; 104:5103 - 5108
- Farooq M, Sulochana KN, Pan X, To J, Sheng D, Gong Z, et al. Histone Deacetylase 3 (hdac3) is specifically required for liver development in zebrafish. Dev Biol 2008; 317:336 - 353
- Noel ES, Casal-Sueiro A, Busch-Nentwich E, Verkade H, Dong PDS, Stemple DL, et al. Organ-specific requirements for Hdac1 in liver and pancreas formation. Dev Biol 2008; 322:237 - 250
- Lagger G, O'Carroll D, Rembold M, Khier H, Tischler J, Weitzer G, et al. Essential function of histone deacetylase 1 in proliferation control and CDK inhibitor repression. EMBO J 2002; 21:2672 - 2681
- Ma P, Schultz RM. Histone deacetylase 1 (HDAC1) regulates histone acetylation, development and gene expression in preimplantation mouse embryo. Dev Biol 2008; 319:110 - 120
- Cunliffe VT. Histone deacetylases 1 is required to repress Notch target gene expression during zebrafish neurogenesis and to maintain the production of motoneurones in response to hedgehog signaling. Development 2004; 131:2983 - 2995
- diIorio PJ, Moss JB, Sbrogna JL, Karlstrom RO, Moss LG. Sonic hedgehog is required early in pancreatic islet development. Dev Biol 2002; 244:75 - 84
- Ouaissi M, Cabral S, Tavares J, da Silva AC, Mathieu Daude F, Mas E, et al. Histone Deacetylase (HDAC) encoding gene expression in pancreatic cancer cell lines and cell sensitivity to HDAC inhibitors. Cancer Biol Ther 2008; 7:523 - 531
- Wang W, Gao J, Man XH, Li ZS, Gong YF. Significance of DNA methyltransferase-1 and histone deacetylase-1 in pancreatic cancer. Oncol Rep 2009; 21:1439 - 1447
- Deer EL, Gonzalez-Hernandez J, Coursen JD, Shea JE, Ngatia J, Scaife CL, et al. Phenotype and genotpye of pancreatic cancer cell lines. Pancreas 2010; 39:425 - 435
- Miyake K, Yoshizumi T, Imura S, Sugimoto K, Batmunkh E, Kanemura H, et al. Expression of hypoxia-inducible factor-1(alpha), Histone deacetylase 1 and metastasis-associated protein 1 in pancreatic carcinoma. Pancreas 2008; 36:1 - 9
- Lagger G, Doetzlhofer A, Schuettengruber B, Haidweger E, Simboeck E, Tischler J, et al. The tumor suppressor p53 and histone deacetylase 1 are antagonistic regulators of the cyclin-dependent kinase inhibitor p21/WAF1/CIP1 gene. Mol Cell Biol 2003; 23:2669 - 2679
- Martin ST, Sato N, Dhara S, Chang R, Hustinx SR, Abe T, et al. Aberrant methylation of the human hedgehog interacting protein (HHIP) gene in pancreatic neoplasms. Cancer Biol Ther 2005; 4:728 - 733
- Thayer SP, Pasca di Magliano M, Heiser PW, Nielsen CM, Roberts DJ, Lauwers GY, et al. Hedgehog is an early and late mediator of pancreatic cancer tumorigenesis. Nature 2003; 425:851 - 856
- Canettieri G, Di Marcotullio L, Greco A, Coni S, Antonucci L, Infante P, et al. Histone deacetylase and Cullin3-RENKCTD11 ubiquitin ligase interplay regulates Hedgehog signalling through Gli acetylation. Nat Cell Biol 2010; 12:132 - 144
- Westerfield M. The zebrafish book: a guide for the laboratory use of zebrafish (Danio rerio) 2007; 5th ed Eugene, Oregon University of Oregon Press
- Yee NS, Zhou W, Lee M. Transient receptor potential channel TRPM8 is overexpressed and required for cellular proliferation in pancreatic adenocarcinoma. Cancer Lett 2010; 297:49 - 55
- Furukawa T, Duguid WP, Rosenberg L, Viallet J, Galloway DA, Tsao MS. Long-term culture and immortalization of epithelial cells from normal adult human pancreatic ducts transfected by the E6E7 gene of human papilloma virus 16. Am J Path 1996; 148:1763 - 1770