Abstract
Excess reactive oxygen species (ROS) generated from ionizing radiation (IR) or endogenous sources like cellular respiration and inflammation produce cytotoxic effects that can lead to carcinogenesis. Resveratrol (RSV), a polyphenol with antioxidant and anticarcinogenic capabilities, has shown promise as a potential radiation modifier. The present study focuses on examining the effects of RSV or RSV metabolites as a radiation modifier in normal tissue. RSV or a RSV metabolite, piceatannol (PIC) did not protect human lung fibroblasts (1522) from the radiation-induced cell killing. Likewise, neither RSV nor PIC afforded protection against lethal total body IR in C3H mice. Additional research has shown protection in cells against hydrogen peroxide when treated with RSV. Therefore, clonogenic survival was measured in 1522 cells with RSV and RSV metabolites. Only the RSV derivative, piceatannol (PIC), showed protection against hydrogen peroxide mediated cytotoxicity; whereas, RSV enhanced hydrogen peroxide sensitivity at a 50 µM concentration; the remaining metabolites evaluated had little to no effect on survival. PIC also showed enhancement to peroxide exposure at a higher concentration (150 µM). A potential mechanism for RSV-induced sensitivity to peroxides could be its ability to block 1522 cells in the S-phase, which is most sensitive to hydrogen peroxide treatment. In addition, both RSV and PIC can be oxidized to phenoxyl radicals and quinones, which may exert cytotoxic effects. These cytotoxic effects were abolished when HBED, a metal chelator, was added. Taken together RSV and many of its metabolic derivatives are not effective as chemical radioprotectors and should not be considered for clinical use.
Introduction
The imbalance between the cellular production and detoxification of reactive oxygen species (ROS) can result in oxidative stress.Citation1 Endogenous sources of ROS include cellular respiration, activation of the immune system, and inflammation. Left unchecked, ROS can inflict significant damage to DNA, proteins, and lipids resulting in both acute and long-term consequences including a variety of pathological diseases such as cancer.Citation1 Oxidative stress can also be imposed by environmental factors such as ionizing radiation (IR). Exposure to IR can come from background cosmic radiation, intentional detonation of nuclear devices, nuclear reactor accidents and medical exposure. Because 50% of all cancer patients receive radiotherapy during the course of their disease, there is considerable interest in identifying means to protect against IR damage in normal tissues that are invariably exposed.Citation2
One approach toward reversing the untoward effects of IR-induced ROS and oxidative stress is the application of antioxidants. A considerable number of antioxidants have emerged over the past several years with varying protective capabilities. Of particular interest is resveratrol (RSV); a polyphenol compound found in the skin of grapes and wine, as well as in a variety of berries, nuts and herbs.Citation3 In vivo, RSV is rapidly converted into several conjugate forms once ingested including piceatannol (PIC).Citation4,Citation5 In vitro data demonstrate numerous pathways in which RSV and PIC can induce apoptosis, decrease angiogenesis and block the cell cycle.Citation6-Citation9
With respect to RSV protection against IR-mediated damage, there are conflicting reports. For example, RSV has been shown to enhance the radiosensitivity of cancer cell lines to IR,Citation10-Citation13 suggesting a possible clinical benefit to patients receiving radiotherapy.Citation11,Citation14-Citation16 Conversely, some reports show that RSV has protective effects against IR-mediated oxidative stress in non-cancerous normal tissues including myocardial cells, hepatic/ileal tissue and murine bone marrow.Citation17-Citation19 One specific study showed that RSV pre-treatment decreased IR-induced bone marrow chromosomal aberrations in C3H mice receiving total body irradiation (TBI).Citation18
These studies suggest that RSV can exert both protective and enhancement effects when exposed to oxidative stress. RSV-mediated protection against chromosomal aberrations suggests that RSV might protect against IR-induced lethality in vivo. To gain further insight into RSV radioprotective capabilities, IR survival data for normal human fibroblasts with and without RSV treatment and mice pre-treated with RSV and exposed to TBI are provided. Additionally, RSV and selected metabolites were evaluated with respect to exposure H2O2-mediated oxidative stress.
Results
The effects of RSV and PIC on IR survival in vitro
Since RSV was shown to decrease chromosomal aberrations in the bone marrow of C3H mice after exposed to IR,Citation18 experiments were conducted to determine if RSV or PIC could protect against IR-induced cell killing in 1522 normal human lung fibroblasts. As shown in , neither RSV nor PIC protected 1522 cells against IR-induced cell killing. In fact, RSV enhanced the cytotoxic effects of IR with a DMF of 0.71 ± 0.05 at 10% survival. PIC had no effect on radiosensitivity exhibiting a DMF of ~1.0 at 10% survival.
The effects of RSV and PIC on IR survival of C3H mice receiving TBI
Mice were administered RSV using a similar protocol used by Carsten et al.Citation18 consisting of three daily doses of RSV in C3H female mice (50, 100 and 300 mg/kg; 5–15 mice per group) prior to an acute lethal dose of IR (9 Gy). There was no change in survival observed in the groups given RSV compared with control, and all mice were deceased by day 14 (data not shown). Additionally similar lethal-dose experiments were done in C3H mice using PIC (25, 50 and 100 mg/kg; five mice per group) and showed no protection against 9 Gy TBI (data not shown). Since a 9 Gy dose resulted in no survival of mice with or without RSV or PIC pre-treatment, a lower sub-lethal IR dose of 6 Gy was evaluated with or without RSV pre-treatment (300 mg/kg; five mice per group). RSV pre-treatment did not significantly alter survival (data not shown). This finding differed from the in vitro enhancement observed by RSV ().
Antioxidant activity of RSV and selected metabolites
Previous reports have demonstrated that RSV and PIC possess antioxidant activity with PIC being a more potent radical scavenger.Citation8,Citation20 No data exists that chemically show antioxidant activity in RSV metabolites; mainly the sulfate and glucuronide conjugates, which are abundant metabolites in the body. In this study, the antioxidant activity of RSV, PIC and several RSV metabolites was measured using EPR spectroscopy with spin trapping by monitoring the inhibition of the accumulation of the superoxide adduct of the spin trap DMPO in a reaction where the superoxide is generated in an aerobic mixture of hypoxanthine + xanthine oxidase in the presence of DMPO. shows the superoxide scavenging ability of the test compounds in the concentration range of 0–1.0 mM. Consistent with previous reports, PIC scavenged superoxide (24-fold) more efficiently when compared with RSV. However, the remaining metabolites showed little-to-no significant antioxidant activity.
Figure 2. Antioxidant measurements of RSV and RSV metabolites. The reaction between xanthine oxidase and hypxanthine creates superoxide that converts DMPO to DMPO-OH. Antioxidants scavenge superoxide, thus decreasing the amount of DMPO-OH generated. Only RSV and PIC produced a significant amount of antioxidant activity, with PIC being 24-fold more efficient (PICslope/RSVslope).
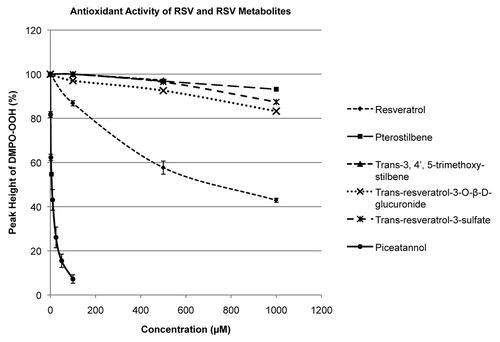
RSV and PIC protection against hydrogen peroxide cytotoxicity
The antioxidant properties of RSV and PIC are not effective in protecting against the radical damage produced by IR, but may protect against other forms of oxidative stress. Therefore, clonogenic survival experiments were conducted to screen for potential protection against single dose hydrogen peroxide cytotoxicity. RSV or RSV metabolites (50 µM) were added 1 h prior to a 1 h incubation of 100 µM hydrogen peroxide. RSV and most of the RSV metabolites had either no effect or sensitized normal human lung fibroblasts (1522) to hydrogen peroxide. The exception was in PIC-treated 1522 cells that exhibited a protection factor of 2.26.
Hydrogen peroxide dose response curves with and without RSV and PIC
Further analysis of RSV and PIC as potential protective agents against hydrogen peroxide was studied by generating full dose survival curves. The 1522 cells were pretreated with RSV (50 µM) for 1 or 24 h, followed by a 1 h hydrogen peroxide treatment. At this concentration for a 1 or 24 h exposure enhanced the cytotoxic effects of hydrogen peroxide (DMF = 0.64 ± 0.08 and 0.52 ± 0.07 at 10% survival, respectively) as shown in . PIC pre-treatment for 1 h at 50 µM and 150 µM protected against hydrogen peroxide cytotoxicity (DMF = 6.6 ± 0.01-and 4.6 ± 0.39 at 50% survival, respectively) as shown in . shows that pretreatment of PIC at 50 µM for 24 h provided modest protection (DMF = 1.2 ± 0.12); however, PIC at 150 µM markedly enhanced hydrogen peroxide-induced cytotoxicity (DMF = 0.65 ± 0.10 at 10% survival) in 1522 fibroblasts.
Figure 3. Hydrogen peroxide clonogenic survival of 1522 fibroblasts: (A) RSV (50 µM) was added to 1522 cells 1 or 24 h prior to H2O2 treatment. Enhanced cytotoxicity was seen at both time points (DMF = 0.64 ± 0.08 and 0.52 ± 0.07 at 10% survival, respectively). (B) PIC (50 and 150 µM) was added 1 h prior to H2O2 exposure. Both drug concentrations exhibited significant protection (DMF = 6.6 ± 0.01-and 4.6 ± 0.39 at 50% survival). (C) PIC (50 and 150 µM) was added 24 h prior to H2O2 exposure. Protection was seen for the 50 µM concentration (DMF = 1.2 ± 0.12 at 10% survival); however, enhancement of the peroxide cytotoxicity occurred using the 150 µM concentration (DMF = 0.65 ± 0.10 at 10% survival). (D) TBH clonogenic survival of 1522 fibroblasts. RSV or PIC (50 µM) was added to cells 1 h prior to a 1 h exposure to TBH. RSV enhanced TBH cytotoxicity whereas PIC provided protection (DMF = 0.42 ± 0.05 and 1.7 ± 0.02, respectively).
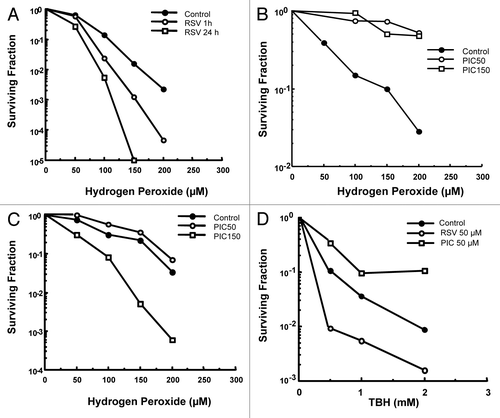
The effects of RSV and PIC on tertiary butyl hydroperoxide cytotoxicity
To examine the effects of RSV and PIC on an organic peroxide-induced oxidative stress, 1522 cells were exposed to tertiary butyl hydroperoxide (TBH). As shown in , RSV enhanced and PIC protected against TBH-induced cell death (DMF = 0.42 ± 0.05 and 1.7 ± 0.02, respectively.)
Cell cycle with RSV and PIC
To determine if differential perturbations in the cell cycle might explain the different responses of RSV and PIC to hydrogen peroxide, cell cycle analysis was conducted. There was a significant block in the G1/G0-phase when pretreated with PIC compared with untreated cells (80 vs. 70%, respectively; p = 0.012). An even more substantial block was seen in S-phase in cells treated with RSV when compared with control (49 vs. 18%, respectively; p = 0.016). Treatment with RSV resulted in significant changes in both G1/G0 and the S-phase.
RSV and PIC production of phenoxyl radicals and quinones in the presence of hydrogen peroxide
Both RSV and PIC at high concentrations can exert cytotoxicity to 1522 cells. Previous studies have shown that RSV and PIC can be oxidized to generate phenoxyl radicals and quinones that have potential to produce cellular lesions.Citation20,Citation21 This may explain the enhancement in cell killing seen in normal human cells. To confirm this, HRP and H2O2 were added to RSV or PIC in the presence of the Tempol-H.Citation22 Phenoxyl radicals generated from the HRP/ H2O2 reaction can oxidize Tempol-H to generate Tempol, a stable nitroxide radical that can be detected and quantified using EPR as shown in . Therefore, an increase in Tempol implicates the intermediacy and level of these radical intermediates. shows that the concentration of Tempol increases as a function of time during the reaction and suggests that both RSV and PIC produce a significant amount of phenoxyl radicals.
Figure 4. Phenoxyl radical production. (A) Diagram illustrating the reaction used to measure phenoxyl radicals produced from RSV and PIC to oxidize Tempol-H to Tempol. (B) Both RSV and PIC produced a significant amount of phenoxyl radicals due to the increase in of Tempol produced over the course of 12 min. Quinone production. Phenoxyl radicals produced by RSV (C) or PIC (D) generate quinones as shown by a shift in UV abs when HRP and H2O2 were added.
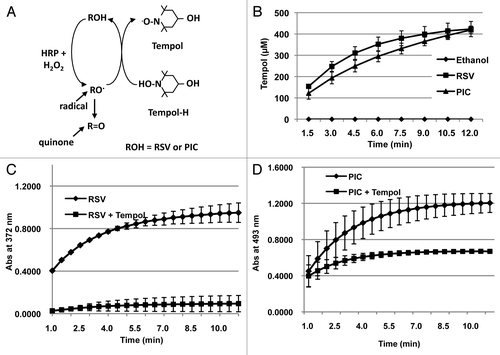
The actual formation of phenoxyl radicals can support formation of cellular lesions, but these compounds are short-lived. However, these radicals also have the potential to react with one another to form the original polyphenol along with an oxidized quinone. These quinones are more stable and have a longer half-life compared with their phenoxyl radical intermediates and can interact with cellular constituents like DNA to cause additional cellular damage.Citation22 The structure of the quinones produces a shift in the UV-visible spectrum, which can be monitored. The UV absorbance changes were monitored using the HRP and H2O2 chemical reaction along with RSV or PIC. A significant increase in the absorbance reading suggests that the phenoxyl radicals generated from RSV and PIC create polyphenolic quinones as shown in .
RSV and PIC cytotoxic effects in the presence of HBED
Low molecular weight complexes of copper and iron by redox transformation from reduced to oxidized state can drive the Fenton reaction to generate strong oxidants such as the hydroxyl radical and inflict oxidative damage. Metal chelators, which can render these metal ions redox-inactive, are used to limit such damage and also examine the role of metal catalyzed processes in oxidative damage. HBED is a hexadentate ligand that binds transition metals such as iron and copper and renders them redox-inactive and has been shown to exert protective effects in cells exposed to bolus amounts of H2O2. To test if exposure of the observed toxicity in cells exposed to RSV or PIC involved metal catalyzed oxidative damage mediated by adventitious transition metal ions, experiments were performed by exposing cells to RSV or PIC for 24 h in the presence or absence of HBED. By using the same compound concentrations (0, 50, 150, 300 and 500 µM) for the survival experiments, it was clear to see in that PIC was more cytotoxic compared with RSV. Furthermore, shows that RSV and PIC cytotoxicity in 1522 cells was lessened when co-incubated with HBED (DMF = 1.4 ± 0.5 and 2.3 ± 0.55, respectively at 10% survival.)
Figure 5. RSV and PIC cytotoxicity clonogenic survival in 1522 cells with HBED. (A) The effect of HBED (100 µM) on the cytotoxic effects of RSV exposure for 24 h in 1522 cells. (B) The survival curve showing similar experiments with PIC. HBED showed protective effects against RSV and PIC exposure suggesting that the oxidative damage associated with prolonged exposure with these agents is mediated at least in part to be mediated by redox-active transition metal complexes. (DMF for RSV = 1.4 ± 0.5 and PIC = 2.3 ± 0.55 at 10% survival).
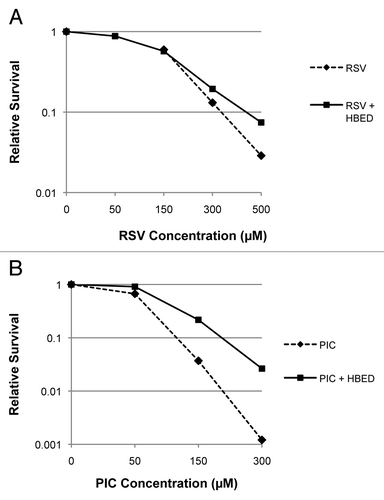
Discussion
There are several stringent requirements for a chemical to provide protection against IR-induced damage including timing, concentration, and location. IR deposits energy into biological material that results in the generation of highly reactive free radicals in a sub-microsecond timeframe.Citation23 Given that the lifetime of the primary radicals generated by IR is so short, a chemical radioprotector needs to be present during IR exposure to scavenge the radiation-induced radicals. Likewise, the concentration of the chemical needs to be sufficiently high (mM range) in order to compete with the rapidly generated radicals. Lastly, the location of the chemical is crucial. It has long been known that the target for IR-induced cell killing is DNA.Citation24-Citation26 Thus, chemicals that have a propensity to bind to DNA such as aminothiols and amino-substituted nitroxides and scavenge IR generated radicals at the target are very potent chemical IR protectors.Citation27-Citation30 In general most antioxidants do not meet the rigorous requirements necessary to be considered a chemical IR protector. Following the primary wave of IR-induced radicals, longer-lived secondary radicals and ROS are formed,Citation31 which would be amenable to reacting with antioxidants through electron donation and could be “chemically” repaired.
The data presented in the current study clearly show that RSV and PIC are not IR protectors against cell killing in vitro or lethality in vivo. In fact, RSV treatment exhibited modest IR sensitization to normal human fibroblasts. Thus, RSV and PIC do not meet the requirements for chemical IR protection as discussed above. This however, does not negate them from providing mitigation against IR damage. For example, the premise for evaluation of RSV as an IR protector stemmed from the report showing that RSV treatment resulted in a decrease in bone marrow chromosome aberrations in mice exposed to 3 Gy TBI.Citation18 Given that there is a direct correlation between IR-induced chromosome aberrations and cell killing,Citation26,Citation32 we hypothesized that RSV would protect against lethal TBI (9 Gy). Neither RSV nor PIC afforded protection against lethal TBI in C3H mice. One possible explanation could be that the IR dose used in chromosome aberration protection studyCitation18 was much lower than was used in the current study; thus the yield of chromosome aberrations would be anticipated to markedly higher with 9 Gy perhaps compromising the ability of RSV to protect against lethality. The mechanism by which RSV afforded protection against IR-induced chromosome aberrations in bone marrow cells is not clear. A possibility could be that RSV interacts with IR-induced secondary radicals or ROS. Additionally, IR can initiate inflammation, which can involve ROS generation, which could indirectly contribute to IR damage and be altered by the presence of RSV. Another possibility could be that any protection seen in vivo stems from a metabolic product of RSV. The common form of RSV used in experiments, as is the case in the present study, is trans-resveratrol. However, several reports show that trans-RSV is rapidly metabolized to several derivatives with the sulfate and glucuronide forms being the most abundant.Citation5,Citation33 These possibilities prompted evaluation comparison of RSV and RSV metabolites for antioxidant activity and potential protection against cell killing from a major component of ROS, hydrogen peroxide.
RSV and PIC, along with a few other RSV metabolites (including a sulfate and glucuronide derivative) were evaluated for antioxidant activity (). In agreement with previous studies,Citation10,Citation11 both RSV and PIC exhibited significant antioxidant activity, with PIC exhibiting the greatest activity. Interestingly, the remaining metabolites showed little to no antioxidant activity. Given the antioxidant activity of RSV and PIC and several reports demonstrating protection against peroxide exposure,Citation21,Citation34 we hypothesized that both RSV and PIC would protect against hydrogen peroxide induced cell killing in normal human lung fibroblasts. The screen conducted evaluating RSV and various metabolites to single dose hydrogen peroxide induced cell killing showed quite unexpectedly that RSV enhanced hydrogen peroxide cell killing while PIC afforded protection. Other metabolites showed a mixed response with some showing enhancement and others exhibiting modest protection. Based on this screen only RSV and PIC were selected for more in depth evaluation. Full dose survival curves showed that RSV markedly enhanced hydrogen peroxide cell killing (), while PIC afforded protection at two concentrations for short duration PIC pre-treatment (1 h) (). However, longer PIC pre-treatment (24 h) at higher concentration potentiated hydrogen peroxide cell killing (); whereas, the lower PIC concentration pre-treatment protection was blunted compared with the 1 h PIC pre-treatment. Similar findings were observed for the hydrophobic lipid radical generating hydroperoxide, TBH with RSV enhancing cell killing and PIC affording protection ().
Mechanistic studies were directed to examine the effects of RSV and PIC on the cell cycle and the possible chemical fates of both agents under conditions of oxidative stress. RSV and PIC were both found to exert significant effects on the cell cycle for a 24 h exposure, with RSV imposing a block in S-phase and PIC inducing a G1 block. How such blocks to normal cell cycle progression impact survival to hydrogen peroxide or radiation is not clear; however, it is known that cells in S-phase are significantly more sensitive to hydrogen peroxide than in other phases of the cell cycle.Citation35,Citation36 The enhancement in radiosensitivity by RSV in fibroblasts () is inconsistent with the S-phase block imposed by RSV treatment in that S-phase cells are known to be the most resistant cells to IR treatment.Citation37 The reason for this apparent discrepancy is unclear; however, it may involve RSV interference with IR damage repair or signaling of pro-survival pathways. RSV has been shown to inhibit COX-1 and COX-2 activity, and STAT3 and NF-kB signaling, while activating TRAIL expression.Citation10,Citation16,Citation33
Under oxidative conditions RSV and PIC were shown to generate phenoxyl radicals (), which can disproportionate to form quinones (). The significance of this finding perhaps contributes to a better understanding of how RSV and PIC may exert cytotoxicity and enhancement of peroxide and/or IR cytotoxicity. The formation of phenoxyl radicals can support formation of detrimental cellular lesions,Citation38,Citation39 but these compounds are short-lived. Phenoxyl radicals can react with one another to generate a more stable quinone that can interact with cellular constituents like DNA to cause additional cellular damage.Citation22 Thus, the formation of phenoxyl radicals and quinones most likely contribute to RSV and PIC cytotoxicity alone (data not shown), the enhancement of IR radiosensitivity () and enhancement of peroxide cytotoxicity (). For example, various quinones have been shown to enhance IR sensitivity,Citation40-Citation42 impose DNA damage and apoptosis,Citation43-Citation45 inhibit DNA damage repair,Citation43,Citation46 and inhibit tumor growth.Citation47,Citation48
The results of this study show that RSV and one of its metabolites, PIC do not protect against IR-induced lethality. Further, while both agents exhibit antioxidant activity, RSV treatment unexpectedly enhanced peroxide cytotoxicity. Underlying the detrimental effects of RSV, and to some extent PIC, is the capability for both agents to form toxic phenoxyl radicals and quinones, which have the propensity to mediate oxidative damage. Thus, the use of RSV as a protective agent against IR cell killing and lethality, ROS-mediated damage, and chemoprevention appears to be complex and problematic for multiple reasons. First, RSV metabolism in vivo yields a number of metabolites, which may or may not possess antioxidant activity. The heterogeneity and polymorphisms among human phase I and II enzymesCitation49 may act upon RSV in different ways with entirely different outcomes. With respect to therapy, RSV has been shown to enhance the radiosensitivity of human tumor cells and thus considered for clinical trials in radiotherapy. However, data presented here show that RSV enhanced cell killing by radiation in normal human lung fibroblasts. Little would be gained if RSV enhanced the IR sensitivity of both tumor and normal cells. Lastly, the production of phenoxyl radicals and quinones from RSV and its metabolites could be beneficial by damaging and killing pre-cancerous cells, but on the other hand can induce toxicity of normal tissues. These considerations should be taken into account before the use of RSV in IR clinical trials.
Materials and Methods
Reagents
RSV (Sigma, R5010), PIC (Sigma: cat# P0453), polydatin (Sigma, 15721), pterostilbene (Alexis Biochemicals, ALX-385–034-M025), trans-3, 4’, 5-trimethoxy-stilbene (Alexis Biochemicals, ALX-350–345-M025), trans-resveratrol-3-sulfate (Toronto Research Chemicals, Inc., R150040, sodium salt) and trans-resveratrol-3-O-β-D-glucuronide (Toronto Research Chemicals, Inc., R150015) were placed in a stock solution of 50–100 mM using DMSO or ethanol and stored at -20°C. HBED was kindly provided by Dr. Martin Brechbiel, Radiation Biology Branch, National Cancer Institute. Horseradish peroxidase (HRP), hypoxanthine (HX), hydrogen peroxide, diethylenetriaminepentaacetic acid (DTPA), 5,5-dimethyl-1-pyrroline N-oxide (DMPO) were purchased from Sigma-Aldrich. 4-Hydroxy-2,2,6,6-tetramethyl-1-hydroxypiperidine (Tempol-H) was purchased from Molecular Probes. Xanthine oxidase (XO) and catalase were purchased from Roche Molecular Biochemicals.
DMPO was purified before use as described in the previous report.Citation50 The concentration of H2O2 solution was estimated by using e240nm of 43.6 M−1 cm−1. Water was distilled and deionized using a MILLI-Q water system.
Cell culture and clonogenic survival
Normal human lung fibroblasts (1522) were purchased from Coriell Institute for Medical Research. Cells were grown in RPMI 1640 medium supplemented with 10% fetal calf serum and antibiotics. For cell survival, 1522 cells were plated (5 × 105 cells/100 mm culture dish) and incubated overnight. Cells were then treated according to the protocols described below. Following treatment cells were trypsinized, counted, plated (250, 1.0 × 103, and 1.0 × 104 cells/60 mm culture dish) and allowed to grow for 10–14 d at 37°C. Once colonies were visible on the culture dishes, media was removed and cells were fixed using methanol/acetic acid (3:1) and stained with crystal violet. Colonies were counted if they possessed > 50 cells/colony.
To determine if RSV and PIC could protect against radiation-induced cell killing, 1522 cells were cells were pretreated (1 or 24 h) with drug (50 µM) and exposed to varying doses of IR using an Eldorado 8 cobalt-60 teletherapy unit (Theratronics International Ltd.) at dose rates of 2.0–2.5 Gy/min. Following radiation treatment cells were rinsed, trypsinized, counted, and plated for macroscopic colony formation (clonogenic assay). For generating full survival curves using RSV (50 µM) and PIC (10, 50 and 150 µM), cells were pretreated (1 or 24 h) with drug and given varying doses of hydrogen peroxide (50, 100, 150 and 200 µM) or tertiary butyl hydroperoxide (0.5, 1.0 and 2.0 mM) for 1 h at 37°C. For the cytotoxic studies, HBED (100 µM) and RSV (50, 150, 300 and 500 µM) or PIC (50, 150 and 300 µM) were added prior to cell plating. Cells were then trypsinized and plated according to clonogenic protocol previously mentioned. Dose modifying factors (DMF) were calculated: (Dosetreated / Doseuntreated) at 10 or 50% survival. DMF ratios < 1.0 indicate sensitization while ratios > 1.0 represent protection. To test potential protection of reagents against oxidative stress, RSV and RSV derivatives were added to 1522 cells for 1 h, and then exposed to a single dose of 100 µM hydrogen peroxide for an additional hour. Protection factor (PF) was determined using SurvivalPIC/SurvivalControl. Values greater than 1 demonstrate protection and values less than 1 show sensitivity.
Cell cycle analysis
Potential changes in the cell cycle by RSV and PIC were measured using a standard DNA stain protocol and flow cytometry. The 1522 fibroblasts were pretreated with RSV (50 µM) and PIC (50 µM) for 24 h. Cells were rinsed using PBS and then fixed using PBS and cold (4°C) ethanol. The pellet was rinsed with PBS and suspended in 1 mL of 20 μg/mL of propidium iodide solution containing 0.1% Triton X-100 and 500 ng of DNase-free RNase. Cell cycle distribution was immediately analyzed using a BD FACSCalibur (BD Biosciences) and CellQuest/MOD-Fit software (Verity Software House).
Animal studies
Female C3H/Hen mice, bred in the National Cancer Institute Animal Production Area, were used for this study. The mice were 8–10 weeks of age at the time of experimentation and weighed between 20–30 g. All experiments were performed under a protocol approved by the National Cancer Institute Animal Care and Use Committee and were in compliance with the Guide for the Care and Use of Laboratory Animal Resource, National Research Council. To determine if RSV or PIC showed radioprotection in vivo, mice were given a single IR dose of 6 or 9 Gy (DXT300 X-ray irradiator with a 2.0-mm Al filtration (300 kV peak) at a dose rate of 1.8 Gy/min; Pantak, Inc.) and survival times were recorded. For drug administration, mice were given the drug following a previously reported protocol with some modification.Citation18 Mice were given three doses (10, 100 and 300 mg/kg for RSV and 10, 25, 50 and 100 mg/kg for PIC; diluted in sterile water) of RSV or PIC every 24 h for 3 d by oral gavage. Mice were then irradiated 30 min after the last dose was administered and then observed every 24 h to determine lethality.
Antioxidant studies
The following reaction was used to measure antioxidant properties of RSV and its metabolites:
Hypoxanthine/ XO → O2•− + DMPO → DMPO-OOH
In the presence of XO, the superoxide anion (O2•−) is generated from hypoxanthine and reacts with DMPO to yield DMPO-OOH, which can be detected using electron paramagnetic resonance spectroscopy (EPR). Antioxidants are able to scavenge the superoxide anion that will ablate the reaction, decreasing the amount of DMP-OOH produced. For spin trapping of O2•− radical, the final concentration of DMPO was adjusted to 100 mM, and the reaction was initiated by adding hypoxanthine (2.5 mM) and XO (0.2 U/ml) in the presence of catalase (200 U/mL) in PB (10 mM, pH 7.4). EPR spectra were measured after 1 min reaction at room temperature.
For EPR measurements, 150 µL of samples were drawn into agas-permeable Teflon capillary tube (Zeus Industries) of 0.81 mm inner diameter. Each capillary was folded twice, inserted into a narrow quartz tube open on both sides (2.9 mm inner diameter), and then placed in the cavity of a Varian E-109 X-band spectrometer. EPR parameters were: 3360 G field set, 100 G scan range, 9.358 GHz microwave frequency, 1.25 G modulation amplitude, 100 kHz modulation frequency, 0.064 sec time constant, 2 min scan time, and 10 mW microwave power.
Measurement of phenoxyl radicals and quinones
The formation of Tempol from Tempol-H (5 mM) during HRP (0.04 U/mL)-catalyzed oxidation of RSV (100 µM) and PIC (100 µM) was initiated by adding H2O2 (1 mM) in the presence of DTPA (0.1 mM) in sodium phosphate buffer (PB) (10 mM, pH 7.4) and monitored by EPR every 1.5 min. Similar protocols to that used to measure antioxidant activity were used to analyze Tempol concentrations.
The formation of quinones derived from RSV (0.5 mM) and PIC (0.5 mM) oxidation by HRP (0.04 U/mL)/H2O2 (1 mM) were monitored by measuring the absorbance spectra in the presence and absence of Tempol-H (5 mM) in PB (10 mM, pH 7.4) containing DTPA (0.1 mM). The absorbance spectra were measured every 30 sec at room temperature with a HP 8453 UV-Visible spectrophotometer.
Abbreviations: | ||
ROS | = | reactive oxygen species |
RSV | = | resveratrol |
PIC | = | piceatannol |
H2O2 | = | hydrogen peroxide |
HBED | = | hydroxybenzene ethylene diamine |
TBH | = | tertiary butyl hydroperoxide |
IR | = | ionizing radiation |
DMF | = | dose modifying factor |
PF | = | protection factor |
TBI | = | total body irradiation |
Gy | = | gray |
Acknowledgments
This research was supported by the Intramural Research Program of the Center of Cancer Research, National Cancer Institute, National Institutes of Health.
Disclosure of Potential Conflicts of Interest
No potential conflicts of interest were disclosed.
References
- Kryston TB, Georgiev AB, Pissis P, Georgakilas AG. Role of oxidative stress and DNA damage in human carcinogenesis. Mutat Res 2011; 711:193 - 201; http://dx.doi.org/10.1016/j.mrfmmm.2010.12.016; PMID: 21216256
- Citrin D, Cotrim AP, Hyodo F, Baum BJ, Krishna MC, Mitchell JB. Radioprotectors and mitigators of radiation-induced normal tissue injury. Oncologist 2010; 15:360 - 71; http://dx.doi.org/10.1634/theoncologist.2009-S104; PMID: 20413641
- Baur JA, Sinclair DA. Therapeutic potential of resveratrol: the in vivo evidence. Nat Rev Drug Discov 2006; 5:493 - 506; http://dx.doi.org/10.1038/nrd2060; PMID: 16732220
- Yu C, Shin YG, Chow A, Li Y, Kosmeder JW, Lee YS, et al. Human, rat, and mouse metabolism of resveratrol. Pharm Res 2002; 19:1907 - 14; http://dx.doi.org/10.1023/A:1021414129280; PMID: 12523673
- Delmas D, Aires V, Limagne E, Dutartre P, Mazue F, Ghiringhelli F, et al. Transport, stability, and biological activity of resveratrol. Ann N Y Acad Sci 2011; 1215:48 - 59; http://dx.doi.org/10.1111/j.1749-6632.2010.05871.x; PMID: 21261641
- Gagliano N, Aldini G, Colombo G, Rossi R, Colombo R, Gioia M, et al. The potential of resveratrol against human gliomas. Anticancer Drugs 2010; 21:140 - 50; http://dx.doi.org/10.1097/CAD.0b013e32833498f1; PMID: 20010425
- Kraft TE, Parisotto D, Schempp C, Efferth T. Fighting cancer with red wine? Molecular mechanisms of resveratrol. Crit Rev Food Sci Nutr 2009; 49:782 - 99; http://dx.doi.org/10.1080/10408390802248627; PMID: 20443159
- Leonard SS, Xia C, Jiang BH, Stinefelt B, Klandorf H, Harris GK, et al. Resveratrol scavenges reactive oxygen species and effects radical-induced cellular responses. Biochem Biophys Res Commun 2003; 309:1017 - 26; http://dx.doi.org/10.1016/j.bbrc.2003.08.105; PMID: 13679076
- Gullett NP, Ruhul Amin AR, Bayraktar S, Pezzuto JM, Shin DM, Khuri FR, et al. Cancer prevention with natural compounds. Semin Oncol 2010; 37:258 - 81; http://dx.doi.org/10.1053/j.seminoncol.2010.06.014; PMID: 20709209
- Zoberi I, Bradbury CM, Curry HA, Bisht KS, Goswami PC, Roti Roti JL, et al. Radiosensitizing and anti-proliferative effects of resveratrol in two human cervical tumor cell lines. Cancer Lett 2002; 175:165 - 73; http://dx.doi.org/10.1016/S0304-3835(01)00719-4; PMID: 11741744
- Baatout S, Derradji H, Jacquet P, Ooms D, Michaux A, Mergeay M. Enhanced radiation-induced apoptosis of cancer cell lines after treatment with resveratrol. Int J Mol Med 2004; 13:895 - 902; PMID: 15138632
- Baatout S, Derradji H, Jacquet P, Mergeay M. Increased radiation sensitivity of an eosinophilic cell line following treatment with epigallocatechin-gallate, resveratrol and curcuma. Int J Mol Med 2005; 15:337 - 52; PMID: 15647852
- Fiore M, Festa F, Cornetta T, Ricordy R, Cozzi R. Resveratrol affects X-ray induced apoptosis and cell cycle delay in human cells in vitro. Int J Mol Med 2005; 15:1005 - 12; PMID: 15870907
- Kao CL, Huang PI, Tsai PH, Tsai ML, Lo JF, Lee YY, et al. Resveratrol-induced apoptosis and increased radiosensitivity in CD133-positive cells derived from atypical teratoid/rhabdoid tumor. Int J Radiat Oncol Biol Phys 2009; 74:219 - 28; http://dx.doi.org/10.1016/j.ijrobp.2008.12.035; PMID: 19362240
- Scarlatti F, Sala G, Ricci C, Maioli C, Milani F, Minella M, et al. Resveratrol sensitization of DU145 prostate cancer cells to ionizing radiation is associated to ceramide increase. Cancer Lett 2007; 253:124 - 30; http://dx.doi.org/10.1016/j.canlet.2007.01.014; PMID: 17321671
- Johnson GE, Ivanov VN, Hei TK. Radiosensitization of melanoma cells through combined inhibition of protein regulators of cell survival. Apoptosis 2008; 13:790 - 802; http://dx.doi.org/10.1007/s10495-008-0212-y; PMID: 18454317
- Velioğlu-Oğünç A, Sehirli O, Toklu HZ, Ozyurt H, Mayadagli A, Eksioglu-Demiralp E, et al. Resveratrol protects against irradiation-induced hepatic and ileal damage via its anti-oxidative activity. Free Radic Res 2009; 43:1060 - 71; http://dx.doi.org/10.1080/10715760903171100; PMID: 19707923
- Carsten RE, Bachand AM, Bailey SM, Ullrich RL. Resveratrol reduces radiation-induced chromosome aberration frequencies in mouse bone marrow cells. Radiat Res 2008; 169:633 - 8; http://dx.doi.org/10.1667/RR1190.1; PMID: 18494544
- Ye K, Ji CB, Lu XW, Ni YH, Gao CL, Chen XH, et al. Resveratrol attenuates radiation damage in Caenorhabditis elegans by preventing oxidative stress. J Radiat Res (Tokyo) 2010; 51:473 - 9; http://dx.doi.org/10.1269/jrr.10009; PMID: 20679743
- Murias M, Jager W, Handler N, Erker T, Horvath Z, Szekeres T, et al. Antioxidant, prooxidant and cytotoxic activity of hydroxylated resveratrol analogues: structure-activity relationship. Biochem Pharmacol 2005; 69:903 - 12; http://dx.doi.org/10.1016/j.bcp.2004.12.001; PMID: 15748702
- Ovesná Z, Kozics K, Bader Y, Saiko P, Handler N, Erker T, et al. Antioxidant activity of resveratrol, piceatannol and 3,3′,4,4′,5,5′-hexahydroxy-trans-stilbene in three leukemia cell lines. Oncol Rep 2006; 16:617 - 24; PMID: 16865264
- Samuni AM, Chuang EY, Krishna MC, Stein W, DeGraff W, Russo A, et al. Semiquinone radical intermediate in catecholic estrogen-mediated cytotoxicity and mutagenesis: chemoprevention strategies with antioxidants. Proc Natl Acad Sci USA 2003; 100:5390 - 5; http://dx.doi.org/10.1073/pnas.0930078100; PMID: 12702779
- Hall EJ, Giaccia AJ. Radiobiology for the Radiologist. Philadelphia: Lippincott Williams & Wilkins 2006.
- Munro TR. The relative radiosensitivity of the nucleus and cytoplasm of Chinese hamster fibroblasts. Radiat Res 1970; 42:451 - 70; http://dx.doi.org/10.2307/3572962; PMID: 5463516
- Ward JF. DNA damage produced by ionizing radiation in mammalian cells: identities, mechanisms of formation, and reparability. Prog Nucleic Acid Res Mol Biol 1988; 35:95 - 125; http://dx.doi.org/10.1016/S0079-6603(08)60611-X; PMID: 3065826
- Cornforth MN, Bedford JS. A quantitative comparison of potentially lethal damage repair and the rejoining of interphase chromosome breaks in low passage normal human fibroblasts. Radiat Res 1987; 111:385 - 405; http://dx.doi.org/10.2307/3576926; PMID: 3659275
- Hosseinimehr SJ. Trends in the development of radioprotective agents. Drug Discov Today 2007; 12:794 - 805; http://dx.doi.org/10.1016/j.drudis.2007.07.017; PMID: 17933679
- Rasey JS, Nelson NJ, Mahler P, Anderson K, Krohn KA, Menard T. Radioprotection of normal tissues against gamma rays and cyclotron neutrons with WR-2721: LD50 studies and 35S-WR-2721 biodistribution. Radiat Res 1984; 97:598 - 607; http://dx.doi.org/10.2307/3576151; PMID: 6328565
- Patt HM, Tyree EB, Straube RL, Smith DE. Cysteine Protection against X Irradiation. Science 1949; 110:213 - 4; http://dx.doi.org/10.1126/science.110.2852.213; PMID: 17811258
- Mitchell JB, Krishna MC. Nitroxides as radiation protectors. Mil Med 2002; 167:49 - 50; PMID: 11873514
- Riley PA. Free radicals in biology: oxidative stress and the effects of ionizing radiation. Int J Radiat Biol 1994; 65:27 - 33; http://dx.doi.org/10.1080/09553009414550041; PMID: 7905906
- Ostashevsky JY. A model relating cell survival to DNA fragment loss and unrepaired double-strand breaks. Radiat Res 1989; 118:437 - 66; http://dx.doi.org/10.2307/3577405; PMID: 2727270
- Kovacic P, Somanathan R. Multifaceted approach to resveratrol bioactivity: Focus on antioxidant action, cell signaling and safety. Oxid Med Cell Longev 2010; 3:86 - 100; http://dx.doi.org/10.4161/oxim.3.2.11147; PMID: 20716933
- Quincozes-Santos A, Andreazza AC, Goncalves CA, Gottfried C. Actions of redox-active compound resveratrol under hydrogen peroxide insult in C6 astroglial cells. Toxicol In Vitro 2010; 24:916 - 20; http://dx.doi.org/10.1016/j.tiv.2009.11.016; PMID: 19945524
- Leroy C, Mann C, Marsolier MC. Silent repair accounts for cell cycle specificity in the signaling of oxidative DNA lesions. EMBO J 2001; 20:2896 - 906; http://dx.doi.org/10.1093/emboj/20.11.2896; PMID: 11387222
- Frankenberg-Schwager M, Becker M, Garg I, Pralle E, Wolf H, Frankenberg D. The role of nonhomologous DNA end joining, conservative homologous recombination, and single-strand annealing in the cell cycle-dependent repair of DNA double-strand breaks induced by H(2)O(2) in mammalian cells. Radiat Res 2008; 170:784 - 93; http://dx.doi.org/10.1667/RR1375.1; PMID: 19138034
- Terasima T, Tolmach LJ. X-ray sensitivity and DNA synthesis in synchronous populations of HeLa cells. Science 1963; 140:490 - 2; http://dx.doi.org/10.1126/science.140.3566.490; PMID: 13980636
- Kagan VE, Tyurina YY. Recycling and redox cycling of phenolic antioxidants. Ann N Y Acad Sci 1998; 854:425 - 34; http://dx.doi.org/10.1111/j.1749-6632.1998.tb09921.x; PMID: 9928449
- Kensler T, Guyton K, Egner P, McCarthy T, Lesko S, Akman S. Role of reactive intermediates in tumor promotion and progression. Prog Clin Biol Res 1995; 391:103 - 16; PMID: 8532709
- Seo HR, Seo WD, Pyun BJ, Lee BW, Jin YB, Park KH, et al. Radiosensitization by celastrol is mediated by modification of antioxidant thiol molecules. Chem Biol Interact 2011; 193:34 - 42; http://dx.doi.org/10.1016/j.cbi.2011.04.009; PMID: 21570383
- Lee JH, Choi KJ, Seo WD, Jang SY, Kim M, Lee BW, et al. Enhancement of radiation sensitivity in lung cancer cells by celastrol is mediated by inhibition of Hsp90. Int J Mol Med 2010; 27:441 - 6; PMID: 21249311
- Liao HF, Kuo CD, Yang YC, Lin CP, Tai HC, Chen YY, et al. Resveratrol enhances radiosensitivity of human non-small cell lung cancer NCI-H838 cells accompanied by inhibition of nuclear factor-kappa B activation. J Radiat Res (Tokyo) 2005; 46:387 - 93; http://dx.doi.org/10.1269/jrr.46.387; PMID: 16394628
- Gurung RL, Lim SN, Khaw AK, Soon JF, Shenoy K, Mohamed Ali S, et al. Thymoquinone induces telomere shortening, DNA damage and apoptosis in human glioblastoma cells. PLoS ONE 2010; 5:e12124; http://dx.doi.org/10.1371/journal.pone.0012124; PMID: 20711342
- Rietjens IM, Boersma MG, van der Woude H, Jeurissen SM, Schutte ME, Alink GM. Flavonoids and alkenylbenzenes: mechanisms of mutagenic action and carcinogenic risk. Mutat Res 2005; 574:124 - 38; http://dx.doi.org/10.1016/j.mrfmmm.2005.01.028; PMID: 15914212
- Barreto G, Madureira D, Capani F, Aon-Bertolino L, Saraceno E, Alvarez-Giraldez LD. The role of catechols and free radicals in benzene toxicity: an oxidative DNA damage pathway. Environ Mol Mutagen 2009; 50:771 - 80; http://dx.doi.org/10.1002/em.20500; PMID: 19449395
- Bentle MS, Bey EA, Dong Y, Reinicke KE, Boothman DA. New tricks for old drugs: the anticarcinogenic potential of DNA repair inhibitors. J Mol Histol 2006; 37:203 - 18; http://dx.doi.org/10.1007/s10735-006-9043-8; PMID: 16868862
- Banerjee S, Padhye S, Azmi A, Wang Z, Philip PA, Kucuk O, et al. Review on molecular and therapeutic potential of thymoquinone in cancer. Nutr Cancer 2010; 62:938 - 46; http://dx.doi.org/10.1080/01635581.2010.509832; PMID: 20924969
- Bolton JL, Thatcher GR. Potential mechanisms of estrogen quinone carcinogenesis. Chem Res Toxicol 2008; 21:93 - 101; http://dx.doi.org/10.1021/tx700191p; PMID: 18052105
- Zhou SF, Liu JP, Chowbay B. Polymorphism of human cytochrome P450 enzymes and its clinical impact. Drug Metab Rev 2009; 41:89 - 295; http://dx.doi.org/10.1080/03602530902843483; PMID: 19514967
- Samuni AM, Afeworki M, Stein W, Yordanov AT, DeGraff W, Krishna MC, et al. Multifunctional antioxidant activity of HBED iron chelator. Free Radic Biol Med 2001; 30:170 - 7; http://dx.doi.org/10.1016/S0891-5849(00)00459-7; PMID: 11163534