Abstract
Breast cancer is the most frequent spontaneous malignancy diagnosed in women and is characterized by a broad histological diversity. Progression of the disease has a metastasizing trend and can be resistant to hormonal and chemotherapy. Animal models have provided some understanding of these features and have allowed new treatments to be proposed. However, these models need to be revised because they have some limitations in predicting the clinical efficacy of new therapies. In this review, we discuss the biological criteria to be taken into account for a realistic animal model of breast cancer graft (tumor implantation site, animal immune status, histological diversity, modern imaging). We emphasize the need for more stringent monitoring criteria, and suggest adopting the human RECIST (Response Evaluation Criteria in Solid Tumors) criteria to evaluate treatments in animal models.
Introduction
Breast cancer is the most frequent spontaneous malignancy diagnosed in women and still bears a high mortality rate.Citation1 The breast epithelium is particularly sensitive to carcinogenesis because mammary glands undergo several marked changes during the life span of a reproductive female (puberty, pregnancy, lactation and menopause), which are mediated by different hormones and growth factors.Citation2 Although current treatments increase patient survival,Citation3 the benefits vary noticeably among different patient groups, and metastasized or chemoresistant cancers still have a poor prognosis. Thus, more effective treatments, particularly in the latter cases, are needed. Following in vitro studies, the typical development of a new cancer chemotherapy includes in vivo experiments conducted on animal models to define the efficacy (potential activity determination), the ADME pharmacological parameters (drug absorption, distribution, metabolism and elimination) and the toxicological profile.Citation4 These animal models are, therefore, crucial prerequisites for cancer therapy development.
Xenografts of human tumors in immunodeficient mice remain the models of reference for testing cancer drugs.Citation5 In addition to being among the best genetically characterized mammals, rodents are small in size, cheap, have a short generation time, a high incidence of human-tumor engraftment, and a lot of scientific tools available for their study (antibodies, markers). However these xenografts have limitations in cancer drug development. Although the approval rate of all investigational new molecules (non-cancer treatments included) is around 10%,Citation6 the rate for new oncology molecules is only around 5%.Citation7 This low rate may reflect a dysfunction in the drug development process for cancer, where preclinical animal studies play a central role. In contrast to original in-human tumors, an induced in-rodent tumor may to be easier to cure and may therefore generate false positive responses to treatments.Citation8,Citation9 Indeed, the number of anticancer agents that are effective in animal models only to fail in human clinical trials has markedly increased in recent years, and improvements in the selection procedures of new molecules are clearly required.
This review will focus on the scientific prerequisites which appear to be mandatory for the validation of clinically relevant breast cancer models. We will cover the following areas: the choice of species, immune status of animals, tumor type, tumor location and microenvironment, monitoring technologies and interpretation criteria.
What are the Current Therapeutic Challenges in Human Breast Cancer?
Despite recent improvements, human breast cancer remains a life-threatening disease with a mortality rate of around 20% at 5 y. This severity is due to several features of the disease including, (1) metastatic potential, (2) hormone resistance, (3) chemo-resistance, and (4) histological heterogeneity. The metastatic potential of breast cancer is well known, and lymph nodes as well as sentinel nodes are carefully examined during the early phases of staging.Citation10Metastases bear a particularly poor prognosis and the median survival of patients with metastatic breast cancer is only 2–3 y. The bones, lungs, liver, and brain are preferential sites for the development of breast metastases.Citation11 Development of bone metastases occurs in over 70% of metastatic breast cancer cases and is usually incurable.Citation12 Although some patients benefit from hormone treatment when their cancer is sensitive to these agents,Citation13 resistance is a frequent problem.Citation14 The period of time during which hormones are active is limited, and most tumors become resistant.Citation15 Furthermore, resistance to major anticancer therapies, including the most recent ones, is another major cause of death in patients. Finally, breast cancer is quite heterogeneous, as shown by recent molecular studies of tumor profiling, and it is more appropriate to speak of several types of breast cancers rather than of breast cancer as an entity. Recently, a classification of five histological types has been proposed based on gene expression studies.Citation16-Citation18 These features should be compared with the animal models of breast cancer, which are currently available for research.
What are the Currently Available Animal Models of Breast Cancer?
Several types of animal models are available: (1) chemo-induced cancers, (2) transgenic animals, and (3) grafted cancers. Chemo-induced cancers are obtained usually after injection DMBA or MNU.Citation2,Citation19 The tumors appear some weeks after exposure to the chemical agent but the site of occurrence is unpredictable and the same animal may have several tumors. Chemo-induced models are mainly used for studying prevention and early oncogenic phenomena and are not often used to test anticancer agents. In transgenic animal models, a gene is introduced into the animal’s DNA and the coded protein is over expressed or under expressed to study its role in the oncogenic process. Most of these models have been developed in the mouse and most of the studied proteins are controlled by an MMTV promoter.Citation20 Protein modification in these models increases the occurrence of breast cancer, although several tumors can been seen at the same time and the site of occurrence in the animals is unpredictable. Maintaining these animal lines is quite expensive. As with the chemo-induced models, transgenic models are not frequently used to test anticancer treatments. At present, the most commonly used animal models to test new therapies are grafted models, in particular human xenograft models.Citation21 These models have many advantages: several cell lines are available, the cancer cells can be grafted onto animals at a specific site on the same day, and tumor growth is usually reproducible among the different animals allowing the formation of treatment groups and reliable statistical analysis. However, there are also limitations to grafted animal models.
Animal models of breast cancer with bone metastases are uncommon. Several murine metastatic models exist that use transgenic rodents predisposed to mammary epithelium tumor formation and subsequently to metastases.Citation22,Citation23 However, bone metastases are rarely observed in these models. Another experimental rodent model of bone metastasis consists of a cancer cell injection directly into the circulation, either by injection into the tail vein or the cardiac left ventricle of an immunodeficient animal.Citation24,Citation25 These models can be useful to examine and identify the factors involved in the proliferation of breast cancer cells that have been directly deposited into bone. However, they do not replicate the early events of metastasis from the primary tumor site and may therefore not encompass the molecular mechanisms by which most breast cancer cells are attracted to bone in patients.
Breast cancer is a highly heterogeneous disease and heterogeneity is often obvious even within the same tumor. Based on gene-expression profiles from microarray studies, five major subtypes of human breast cancers have been described: normal-like, luminal A, luminal B, basal and ErbB2 positive.Citation26 In contrast, in most preclinical studies of breast cancer, only one of three human breast cancer cell lines (MCF-7, T47-D and MDA-MB-231) has been used.Citation21 Unfortunately, none of these cell lines gives rise to a typical human breast cancer. Discrepancies regarding heterogeneity appear to be at least partially responsible for the over prediction or under prediction of treatment efficacy.Citation5 To represent human tumor heterogeneity, the generation of several new rodent breast cancer cell lines could be an option. In this way, tumors from transgenic animals could be used, and many oncogenes have been placed under the control of MMTV resulting in various types of mammary tumors. Many of these transgenic mice induce tumors that have a distinctive pathology dependent upon the initiating oncogene.Citation27 For example, the polyomavirus middle T (PyV-mT) transgenic mouse model of breast cancer expresses six well-characterized phenotypes.Citation28 In addition, transgenic mice with the PIK3CA mutation develop carcinomas with different phenotypes composed of cells expressing luminal or/and basal markersCitation29 based on Perou’s classifcation.Citation16 Cell lines with resistance to different therapies such as hormonotherapy and chemotherapy could be very useful to study multiresistant tumors and some teams have generated new cell lines for this purpose. An adriamycin-resistant human breast tumor cell line (MDA-A1R) was generated by step-wise selection of increasing concentrations of drug from the parent cell line MDA-MB-231.Citation30 However the number and the use of these cell lines remain limited.
What are the Options to Generate New Animal Models of Breast Cancer?
To develop new and more reliable breast cancer animal models, several considerations have to be made.
The choice of the species: rat vs. mouse
Rodents, usually rats or mice, are the most frequently used species for animal models. Although mice are the most commonly used species for cancer research, rats are an interesting alternative and have many advantages in the case of rodent syngeneic grafts. Indeed, several authors report that rat tumors more closely resemble many aspects of human breast cancer pathology when compared with mice tumors.Citation20,Citation31-Citation35 Like human tumors, rat mammary tumors are most of the time estrogen dependent (> 80 and > 70%, respectively), whereas mouse mammary tumors usually have an estrogen-independent growth.Citation31,Citation36-Citation38 Most rat mammary cancers are sensitive to hormone therapyCitation32 which is also the case for human breast cancers. Similar to most human breast cancers, chemically induced rat mammary tumors (DMBA) are of ductal origin,Citation38 with the ductal subtype accounting for 40–75% of all diagnosed cases.Citation37 There is no known viral etiology for breast cancer in human and rat tumors,Citation35 whereas mouse mammary tumors are often associated with a virus,Citation2,Citation33 usually the mouse mammary tumor virus (MMTV). For several authors, the rat strain is a species that more closely mimics the development of human breast cancer than the mouse strain.Citation39 The hormonal milieus, as well as the different steps of oncogenic transformation, are quite similar in rats and in humans. Thus, in rat transgeneic models, important genes could be overexpressed, such as neu, ras, mutated or deleted p53, such as BRCA1 or BRCA2, to produce a humanized cancer model.Citation34,Citation40 The modifications of gene expression could also be combined or be conditioned by using, for example the Cre-Lox system.Citation40 All of the gene modifications will have to be guided by gene-expression studies performed on human cancers. Transgenic rat models were found to more closely resemble human disease than transgenic mice harboring the same constructs.Citation34 Moreover, in rat models (chemo-induced, grafted, transgenic), benign tumors, such as intraductal papilloma, papillary cystoadenoma and adenoma, as well as malignant tumors, such as papillary carcinoma, cibriform carcinoma, comedo carcinoma, and tubular carcinoma have all been identified.Citation41 Most of these neoplasic lesions found in the rat mammary gland have their counterparts in human pathology. The exceptions are human-specific lesions, such as lobular carcinoma and Paget’s disease, which have no counterpart in the rat. These data indicate that the rat model may recapitulate more phenotypic heterogeneity as seen in human breast cancer. The rat species is also an interesting alternative to mice as the size of a rat makes it easier to use modern imaging in follow-up, such as PET-scan, Spect-scan or CT-Scan. This is critical to produce reliable data. The strong similarities between rats and humans regarding many aspects of breast cancer, in our opinion, make the rat species a better experimental species, even though rats are less frequently used than mice in preclinical research today.
Impact of immune status of the grafted animal
Clinical and experimental studies indicate that innate and adaptive immune cells play a major role in cancer progression and eradication. For example, in breast cancer, tumor infiltration by T cells is associated with a favorable prognosis.Citation42 Moreover, researchers are now investigating the efficacy of novel anticancer strategies based on immunotherapeutics that either bolster antitumor adaptive immunity or neutralize the cancer-promoting properties of innate immune cells.Citation43 However, most of the cell lines used in cancer studies today are grown in immunodeficient animals, which fail to take into account the impact of the immune system in treatment. We discuss the possible options regarding this important issue.
Immunodeficient strains
Immunodeficient rodent strains have been generated by genetic engineering. Today, there are over 30 loci where different mutations have been shown to produce an immunocompromised phenotype in mice or rats. The two most used models are the scid (severe combined immune deficiency) and nude mouse strains.Citation5 Rodents with scid mutations fail to rearrange the genes encoding antigen-specific receptors on T and B cells.Citation44 In these mutants, B cells cannot be detected in blood and the remaining T cells are defective. This model has no major defects in macrophages and natural killer (NK) cells, but the lack of lymphokines required for specific activities can result in functional impairments.Citation45 Rodents with the nu mutation are also called “athymic nude” rodents although a residual thymus is present in some cases. B-cell maturation is defective in these mutants but normal virgin B cells are present. T-lymphocyte levels are very low, as are responses to T-cell dependent antigens. The NK and macrophage activity in this model are comparable to wild-type rodents.Citation46 Both of the above-mentioned strains are deficient in their specific immunity. They require numerous precautions in handling and need to be kept under sterile housing conditions (food and water have to be sterilized). Their life span is quite short and they are expensive to breed. More importantly, as the immune system plays a tremendous role in cancer development and treatment, it appears pointless to use animals with an inactivated immune system when trying to develop a new anticancer therapy.
Immunodepressed animals
To avoid total inhibition of the immune system, in 1978 Steel et al. proposed to graft normal animals in combination with immunosuppression techniques such as thymectomy and whole-body animal irradiation.Citation47 This allowed successful grafts of human tumor cells in rats or mice. Animal irradiation was also combined with immunosuppressive drugs such as cyclosporin A or cyclophosphamide and resulted in a 100% engraftment of mice tumors. This percentage was lower when grafting rats with human cancer cells.Citation47 Later, cyclosporin A was used alone by Goodman et al.Citation48 who, for the first time, successfully grafted a human melanoma in an immunocompetent rat. Thus, immunocompetent animals treated with immunosuppressive agents are less restrictive regarding sterile housing conditions, as well as being expensive than immunodeficient animals. Moreover, these animals have a residual immune response and could thus be a good alternative to immunodeficient strains for xenografts. However in these models, immunity is still impaired which is still a drawback when trying to closely mimic human breast cancers.
Immunocompetent animals
The immune system plays a key role in cancer development, eradication and progression.Citation49 In addition, some chemotherapies also influence the immune response. Administration of taxanes to women who were surgically treated for regional breast cancer was associated with higher levels of T-cell activation and NK cell activity when compared with a group of women not receiving taxanes. In the long-term, this activation induced better control of tumor growth by the immune system.Citation50,Citation51 In contrast, cyclophosphamide decreases macrophage activity which is essential for innate immunity reactions.Citation52 In the latter case, knowing the impact of chemotherapeutic drugs on the immune system allows their side effects to be managed by giving a treatment which compensates (stimulates) macrophage activity. Interestingly, syngeneic grafts can be performed in immunocompetent animals. These grafts have the advantage of avoiding the need for immunosuppressed animals. Several types of rodent cell lines already exist which can be used in syngeneic grafts. They come from a spontaneous tumor or a transgenic induced tumor. summarizes most of the available rodent cell lines including the most frequently used human breast cancer cell lines. Recent progress in the fields of carcinogenic agents and transgenesis technologies has allowed the development of new rodent cell lines expressing specific receptors and growth factors.
Table 1. Common human and rodent cell lines used in breast cancer animal models. Some of their particular features are noted
Synergenic grafts vs. xenografts
Today, human cell lines are usually used as xenografts in immunodeficient rodents. However, species specificities may be important in cancer development. Kuperwasser et al.Citation23 developed an animal model bearing human breast cancer metastasis. In this model, female immunodeficient (nod/scid) mice were implanted with small human bone fragments in subcutaneous tissues and were orthotopically injected with human breast cancer cells a few days later. Bone metastases were found only in human implants and not in the mouse skeleton indicating a species-specific osteotropism. The behavior of breast cancer cells in the rodent model was altered in response to the varying microenvironment.Citation53 In contrast with human mammary stroma, rodent stroma contains a reduced number of fibroblastic cells.Citation54 Cytokines and hormones secreted from fibroblasts—which have a key role in tumor proliferation and vascularization (see next section)—also have a species specificity which may explain why human breast tumors have difficulty in growing in rodents, even under immunocompromised conditions. Moreover, human tumor cells generally metastasize poorly in mice/rats, and even when metastasis does occur, unexpected metastatic characteristics are often observed. In contrast, murine tumor cell models often metastasize more effectively and display metastatic characteristics closer to those observed in cancer patients.Citation55 Several syngeneic models have been developed, for example the murine 4T1 model.Citation56 The 4T1 tumor cell line is derived from a subpopulation line isolated from a spontaneously arising mammary tumor from a Balb/cfC3H mouse strain. This model is one of a few breast cancer models with the capacity to efficiently metastasize to sites usually affected in human breast cancerCitation57 (i.e., lungs, liver, brain and bone). Moreover, this model has the advantage of presenting several variant cell lines, some resistant to chemotherapies (6-thioguanine), and some non-metastatic.Citation58
We believe that human cells are generally not fully adapted to grow in rodents and do not behave as they would in a human body. Thus, syngeneic models appear to be a better strategy to mimic cancer development. However, much work still needs to be done on syngeneic cell lines (generation, isolation, characterization, classification) before they can replace human breast cell lines. To our knowledge, such an endeavor has not yet been started.
Impact of microenvironment: ectopic vs. orthotopic grafts
Tumor progression requires a constantly evolving network of interactions between neoplastic cells and their environment which is made of epithelial mammary cells, proteins of the extracellular matrix (ECM) synthesized by stromal cells (fibroblasts, adipocytes, vascular cells), and a mix of cytokines and growth factors.
Impact of mammary cell secreted factors on tumor development
Mammary exocrine glands produce many hormones, growth factors and cytokines which may stimulate carcinogenesis of normal epithelial cells and support progression of tumor cells. These factors not only trigger the multiplication of cancer cells, but also increase the protection against apoptosis and induce metastases. Furthermore, some breast cancer cells overexpress some of these factors. The main hormones secreted by the mammary glands are estrogens and prolactin, which in some cases lead to carcinogenesis.
Aromatase catalyzes the conversion of androgens to estrogens [Estradiol (E2), estriol, estrone],Citation59 which is the rate-limiting step in estrogen biosynthesis. Over the past few decades several groups of investigatorsCitation60 have localized aromatase enzyme in mammary adipocytes (which represent 70% of the mammary gland) and in epithelial cells. This enzyme can synthesize estrogen in situ.Citation61 The amount of E2 produced in breast tissue is considered to be the most important factor in the development of cancer.Citation62 As the concentration of estrogens produced by mammary glands is high, these estrogens are partially responsible for the proliferation and metastatic activities of mammary cells.Citation63 This may generate noticeable differences in tumor growth when a graft is performed in mammary tissue as opposed to subcutaneous tissue. In addition, some estradiol metabolites (particularly catechol estrogen-3,4-quinones) are genotoxic mitogens.Citation64 They can bind DNA and cause depurination, which results in additional mammary-cell mutations.Citation65 Prolactin is also an important hormone as it is almost exclusively produced by mammary cells and increases cell proliferation.Citation66 It also stimulates cytoskeleton modulation which induces breast cancer cell motility and the ability to metastasize.Citation67 Prolactin also protects breast cancer cells against apoptosis.Citation68 These hormones, which actively participate in cancer development, are secreted at a higher level in mammary cells than in subcutaneous cells, making the subcutaneous models a less reliable representation of human breast cancers.
Impact of microenvironment on vascular network formation
Studies have shown that the site of tumor implantation affects vascular development and has a tremendous impact on tumor growth,Citation69,Citation70 although this has not been reported for breast cancers. However, this impact is tumor-dependent and may be variable. Zechmann et al.Citation71 compared vascularization of prostate cancer grafts in ectopic (subcutaneous) and orthotopic sites. Ectopic prostate tumors were more vascularized than orthotopic ones. This was not confirmed by Tsuzuki et al., who used a pancreatic cancer model and found that ectopically grafted cancer cells are more vascularized.Citation72 Indeed, the growth rate of a cancer model is due to three main factors: cancer cells, mammary gland cells, and vascular cells. Clearly, the interactions of these cells must be considered because increases in tumor vascularization could either favor the access of chemotherapeutic drugs to cancer cells or facilitate tumor growth. This may have direct therapeutic consequences since numerous pro-angiogenic factors are targeted by anti-angiogenic therapies.
Impact of stroma and ECM on tumor development
Fibroblasts are a major stoma component and have a well-recognized role in carcinogenic processes.Citation73,Citation74 They are a source of factors that influence the growth of carcinoma cells and angiogenesis.Citation74 Several families of growth factors, implicated as autocrine and paracrine mediators of stromal-epithelial interactions, are involved in carcinoma initiation and progression. These include the FGF family, the insulin-like growth factor-1 (IGF-1) family, the EGF family, the hepatocyte growth factor (HGF), and the TGF-B family.Citation74 Most of these are predominantly stimulators of proliferation. Fibroblasts are also largely responsible for the synthesis, deposition and remodeling of the extra cellular matrix (ECM) in the stroma. ECM is a complex mixture of proteins, proteoglycans, and adhesive glycoproteins. It also contains enzymes responsible for remodeling of the tumor microenvironment such as matrix metalloproteinases (MMP).Citation75 The most important classes of this MMP family are collagenases (MMP-1, -8,-13) and gelatinase (MMP-2, -9). Most MMP are synthesized by fibroblasts and degrade and modify the EMC structure as well as alter cell-cell or cell-ECM interactions. These enzymes facilitate the epithelial to mesenchymal transition (EMT) involved in tumor invasion and the development of metastases.Citation76 Moreover, matrix degradation by MMPs allows growth-factor release and generates a space for endothelial cell migration.Citation77 ECM enzymes are thus indirectly implicated in tumor angiogenesis, invasion and metastasis. Tumor cells may also produce altered ECM proteins which are detected by the immune system. A recruitment of immune cells directed against these proteins allows the release of cytokines, which increase tumor growth.Citation78 The resulting inflammation leads to increased invasiveness and poor prognosis in a variety of cancers.Citation79
As mentioned above, adipose tissue, fibroblasts, vascular networks and epithelium play an important role in tumor development. As the cancer progresses, the surrounding microenvironment co-evolves into an activated state through continuous paracrine communication, thus creating a dynamic signaling circuitry that promotes cancer growth, and ultimately leads to a progressive disease. Grafting tumor cells in an adapted microenvironment therefore appears preferable to achieve a relevant animal model of the cancer of interest. In contrast to ectopic sites of implantation, orthotopic sites offer a tissue-specific microenvironment for grafted cells that will probably result in a more appropriate model for tumor growth, invasion, and therapeutic response. In this case, most hormones and growth factors secreted by the microenvironment could be targeted by anticancer therapies. For example, estrogens could be targeted by blocking their receptors (for example Tamoxifen, an estrogen receptor antagonist) or by suppressing their production using anti-aromatase molecules (for example Arimidex).Citation80 Angiogenesis regulatorsCitation81 can also be used like Bevazucimab, a monoclonal anti-VEGF antibody prescribed for the treatment of metastatic breast cancer. Other molecules targeting the microenvironment, such as MMP inhibitors, are currently in developmentCitation82 and require adapted models.
Tumor sample: fragment vs. cell suspension
A cancer can be implanted into an animal either by injecting a cell suspension or by grafting a fresh or frozen tumor fragment. In contrast to injection of cell suspensions of one cell type, a solid tumor section grafts several cell types at the same time: tumor cells, fibroblasts, vascular cells. This may explain why the engraftment could be noticeably different.Citation83 Both methods have their advantages and drawbacks. Tumor-fragment grafts more closely mimic the pathology observed in human breast cancer than cell suspension injection. However, this method has several drawbacks; the surgical technique is more traumatic than injection of cell-suspension and associated complications such as hemorrhage, infection, or abscess are more likely to occur.Citation84,Citation85 It is also difficult to control the number of viable tumor cells in every fragment; it is easier to do this using the cell suspension technique. Furthermore, failure of grafting may occur when necrotic tissues instead of viable tumor cells are implanted. To reduce the implantation of non-viable tumor cells, the tumor fragment to be grafted has to be taken carefully from the periphery of the ‘mother’ tumor. The periphery of the tumor usually contains more viable cells than the center which is often necrotic with a sludge-like consistency.Citation86 These measures also reduce variability in tumor growth and therefore also statistical variability of tumor sizes. Overall, choosing between fragment or cell suspension will depend on the model, the experiment load, the expertise of the investigator and the purpose of the study.
Tumor Monitoring of Drug Response
Tumor monitoring in animals has to be an integrated part of the experimental strategy. Monitoring gathers important data about in vivo tumor development, tumor characteristics and tumor responses to treatment (qualitative or quantitative evaluation). Classical methods of evaluation tend to be replaced with more modern methods.
Real-time monitoring
Classical methods
The measurement of tumor volume can be easily obtained using a simple caliper. This method is simple and cheap although it can lack accuracy. Tumor volume (V) is calculated by the Carlsson formula: V = a × b2/2, where “a” is the largest superficial visible tumor diameter and “b” the smallest.Citation87 Other tumor volumes are also used as, the ellipsoid volume formulaCitation88 (π/6 × L × W × H and 1/2 × L × W × H) or the standard 4/3πr3 formula. Obviously, these methods can only be applied to external tumors and do not accurately monitor metastasis. These methods also cannot precisely quantify the response to treatment which is a requirement of the RECIST criteria explained below.
Small animal imaging by bioluminescence
Optical imaging uses bioluminescenceCitation89 or fluorescence.Citation90 These methods are based on the detection of an optic signal produced by a reporter molecule. Reporter molecules produce a signal by constitutive expression (such as green fluorescent protein or DsRed producing fluorescence), or by enzymatic activation of an inactive substrate (such as luciferase producing bioluminescence). It has been recently demonstrated that the transfection of reporter molecules does not significantly alter tumor cell growth in vitro or in vivo.Citation91 These bioluminescent or fluorescent probes can be used in preclinical oncology to follow the course of disease progression, including metastases or responses to treatment. Furthermore, these methods could be used to monitor gene delivery and gene expression, evaluate tumor angiogenesisCitation92 and apoptosis, image protein-protein interactionCitation89 and cell trafficking,Citation93 and to assist in vivo mechanistic studies.Citation94 Optical imaging methods are non-invasive, non-radioactive, quantitative, sensitive and have a short acquisition time. They are also inexpensive compared with other methods. However, optical imaging of biological tissues is constrained by technical issues, particularly fluorescence. In fact, biological chromophores such as hemoglobin absorb light, decrease penetration and emission of light.Citation95 Since fluorescent proteins emitting short-wavelength photons in the blue or green range are detectable at only a few millimeters of depth, research groups are developing new probes with near and far infrared fluorescence.Citation96 Among these groups, R. Tsien and his team developed a set of genetically engineered fluorescent proteinsCitation97 from a bacterial phytochrome. These proteins, named IFP1.0 to IFP1.4, have a maximum absorption wavelength around 685 nm, emit around 720 nm, are sensitized by systemic injection of biliverdin and have been used successfully for deeper tissue imaging (1–2 cm) of cancers in mice. In a different experiment, Tsien and his group linked an activatable cell penetrating peptide (ACPP) to a fluorophore (Cyanide 5, excitation 640 nm/ emission 735 nm) and used this conjugate to delineate very accurately the limits of cancers grafted in mice before resection.Citation98 This led to a decrease residual cancer and an improved survival rate of the mice operated with the help of ACPP-Cy5 cancer detection. This ACPP-Cy5 was also used to perform a co-detection by Fluorescence and by MRI.Citation99 Furthermore, Tsien and coworkers conjugated a near infrared fluorophore (Cyanide 7, excitation 740 nm/ emission 820 nm) to a molecule able to be bind to radioactive Fluoride (18F) allowing a co-imaging by fluorescence (size and localization) and by Positron Emission Tomography (metabolic imaging).Citation100 Despite the fact that these methods are not currently used in human medicine,Citation95 no doubt their versatility will be of great help in the near future.
Small animal imaging by CT-Scan, MRI, or PET-Scan
More recent techniques have tremendously improved tumor monitoring although these methods are not always easy to perform and are sometimes quite expensive. Imaging techniques provided by CT-scan (CT), X-ray, magnetic resonance imagery (MRI), or positron emission tomography (PET-scan) have allowed real-time monitoring, and thus, early diagnosis and longitudinal follow-up of external as well as internal tumors (). X-ray CT has been long used in human patients but its application in rodents is more recent.Citation101 This method is powerful for imaging soft-tissue and bone structures and has a high resolution (20 µm). Given that breast cancer preferentially metastasizes to bone and lungs, X-ray CT is very useful for the detection of these types of metastases. This method has a relatively short acquisition time (< 15 min) and is relatively cheap. MRI is another useful technique as it provides a high spatial resolution and can be very valuable for detecting internal tumors and measuring morphologic parameters.Citation102 However, its use is limited by its cost and a longer acquisition time compared with CT-scans.
Figure 1. PET-imaging and CT-scan leading to fusion images and allowing early detection of metastasis. A rat grafted with an osteosarcoma (no image from a metastatic breast cancer was available) in the left leg was explored by a by regular CT-Scan (A, D) by a PET-Scan technique (C, F). The images (B, E) were obtained by superposition of A and B, and D and F, respectively. A 3 mm metastasis (arrow) is detected in the lung both by CT-Scan and PET-imaging. Modern imaging techniques allow the early detection of metastasis, the measurement of metabolic activity of the primary cancer and of metastases, and the fusion of images obtained with different modalities. They allow the staging according to RECIST criteria.Citation104
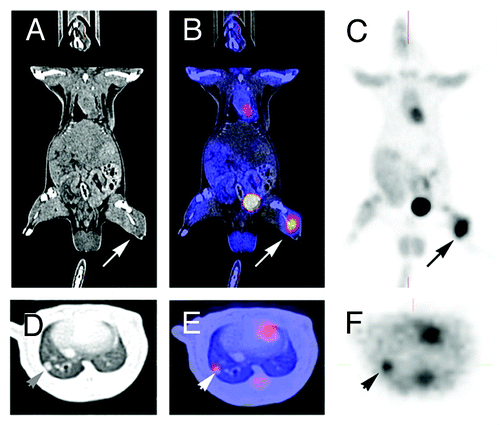
Positron emission tomography (PET) is a more functional technique and requires the use of radioactive isotopes such as 18FDG labeled with an isotopic fluoride.Citation103 PET offers valuable physiological/functional information such as glucose consumption, blood flow, and perfusion. The data obtained with PET-imaging can be merged with those obtained with CT-scan or MRI. The images obtained with merged data are tightly correlated with tumor progression and allow its proliferative profile to be monitored. Imaging is usually performed using PET cameras but may also be performed with a dualhead coincidence gamma camera (DHC). DHC represents an interesting alternative to PET imaging for rat experiments. This equipment is cheaper and gives informative results, at the cost of a slight loss of sensitivity compared with ring PET camera. In addition, DHC studies do not require dedicated equipment for rats.Citation104
All of these modern imaging techniques will probably gain even more attention in the future as they give the possibility of assessing internal tumor progression, metabolic activity, and metastases in a non-invasive manner during the animal’s life. Furthermore, they provide the opportunity to assess tumor response according to the human criteria as proposed below.
Evaluation of tumor response to treatment: toward animal RECIST criteria
In animal models, monitoring response to treatment relies upon the quantification of tumor size variation. In human patients, a decrease in tumor(s) size and increase of life span [disease-free survival (DFS) and progression free survival (PFS)] have been widely adopted as standard end-points to assess the efficacy of anticancer drugs. In addition to these prospective endpoints, the World Health Organization (WHO), the National Cancer Institute (NCI) (USA), and the European Organization for Research and Treatment of Cancer (EORTC) added a new set of human tumor response criteria in 2000: the Response Evaluation Criteria in Solid Tumors (RECIST).Citation105 The RECIST criteria stage a tumor response into four categories (). Complete response (CR) is defined by the disappearance of all cancer lesions. Partial response (PR) is defined by at least 30% reduction of the longest diameter of target lesions, which corresponds to a 70% reduction of tumor volume of a spherical tumor.Citation106 These two categories require a confirmation 4 weeks later. Progressive disease (PR) is defined by increase of the longest diameter by at least 20%. If neither PR nor PD criteria are met an intermediate state called stable disease (SD) is given. Often, CR and PR are combined as the responder group whereas SD and PD are combined to form the non-responder group. As we can see, these criteria developed for humans are very stringent. Unfortunately, they are not frequently used to assess animal models. In many preclinical studies of new anticancer treatments in animal models, a statistically significant decrease of tumor growth rate is often considered as a fair response to treatment. However, this would not be the case if the human RECIST criteria were used.
Table 2. Definition of the different types of response according to the RECIST criteria used in humans, adapted from Padhani et al.Citation106
We believe that these stringent RECIST criteria should be used in animal models to reduce the risk of false-positive preclinical studies. These criteria can obviously only be used if real-time imaging techniques are readily available.
Histological, transcriptomic and proteomic studies
Rigorous criteria are required for animal models to predict the clinical efficacy of anticancer drugs. The simplistic criteria often used in rodent models do not match the challenging criteria of clinical or pathological complete responses and this contributes to the persisting debate on the relevance of rodent cancer models. To date, the main evaluation tools employed by research laboratories are tumor growth, histological studies (immunostaining), and protein gel blot analyses. In contrast, diagnosis and monitoring in the clinic are supported by a combination of clinical (imaging, blood analyses), histological and genomic data.Citation27 Genomic and proteomic studies have been widely used over the last decade to identify other specific signaling pathways in breast cancer which could be responsible for metastasis,Citation107 recurrence,Citation108 or response to treatment.Citation109 Microarray studies highlight the importance of dissecting breast cancer into different subgroups to individualize therapies. This is illustrated by the use of Herceptin® which is an effective therapy in breast cancer by pre-selecting patients who might respond.Citation110 These technologies should be used to compare cancer models with human cancers and to select those which are close to the human pathology. Rodent and human mammary carcinomas may have comparable epigenetic aberrations in their epigenome.Citation111,Citation112 Furthermore, rat tumors may be classified on the basis of the identity of the inducing agent. Therefore, analyses of human tumors may be valuable in retrospectively determining the role of specific carcinogens in the formation of human breast cancer.Citation113 Although expensive, transcriptomic and proteomic studies should be used to evaluate the common targets in human tumors and animal models to match molecular targets between the two situations.
Discussion
Breast cancer is the most frequent malignancy in women. Despite successful treatments of the primary tumor, relapse and subsequent metastasis often occurs in several sites including bone, lung, liver and brain.Citation114,Citation115 As clinical studies for breast cancer have an increased ratio of failure,Citation6,Citation7 animal models of breast cancer somehow lack clinical reliability and could probably be improved.Citation116-Citation120
Considering the important aspects of an animal model that need to be fulfilled for breast cancer drug development, no current animal model is really appropriate. Most of the models used today are too sensitive to drugs, do not metastasize, are xenografts, are grown in immunodeficient mice at ectopic sites. This may explain the poor efficacy of anticancer drugs.
An ideal model is probably unattainable, as it is likely impossible to fully mimic the clinical situation using a different species. Similarly, one animal model is unlikely to be representative of all breast cancers since human breast cancers are heterogeneous. However, we believe that improved models must: (1) use syngeneic and orthotopic graft of cell suspensions, (2) use the rat species, (3) use several breast cancer cell lines mimicking the different types of cancers described in humans, (4) be hormonosensitive but soon become resistant to hormone treatment, (5) have a clear tendency to metastasize to the bones (6) become resistant to most anticancer drugs such as taxanes or anthracyclins, (7) measure tumor growth with PET scan and/or MRI, whereas bone metastases should be diagnosed by X-ray CT scan, and (8) be classified according to RECIST criteria.
Alternatives to rodent models should be discussed. Rodents are commonly used for practical reasons although they differ from humans in various physiological and metabolic areas. The maximal tolerated dose (MTD) for numerous anticancer drugs is higher in rodents than in humans whereas the clinically effective dose is lower.Citation121 The therapeutic drug ranges for rodents are therefore not applicable to humans. Although algorithms exist to extrapolate doses obtained in rodents to humans, the replacement of rodents by a species closer to humans, such as pigs or dogs, could produce more relevant preclinical results, albeit at a much higher cost. Paolini et al. reviewed the recent progresses in this field.Citation122 Naturally occurring tumors in pets have several clinical and biological similarities to human cancers. In fact, pets are exposed to similar carcinogens as humans.Citation123 However, the use of pet dogs with cancer in the development of human cancer treatments has been sparsely used over the past 30 yCitation124 as these tumors are difficult to obtain and standardize.
A clear endeavor of the scientific community to generate better animal models will be invaluable for the entire field. We believe that the changes proposed here for breast cancer models will decrease the number of false positive results and improve the approval rate of new investigational molecules in oncology.
Disclosure of Potential Conflicts of Interest
No potential conflicts of interest were disclosed.
References
- Malvezzi M, Arfe A, Bertuccio P, Levi F, La Vecchia C, Negri E.. European cancer mortality predictions for the year 2011. Ann Oncol 2011; 22:947 - 56; http://dx.doi.org/10.1093/annonc/mdq774; PMID: 21303801
- Russo J, Russo IH. Experimentally induced mammary tumors in rats. Breast Cancer Res Treat 1996; 39:7 - 20; http://dx.doi.org/10.1007/BF01806074; PMID: 8738602
- Mannocci A, De Feo E, de Waure C, Specchia ML, Gualano MR, Barone C, et al. Use of trastuzumab in HER2-positive metastatic breast cancer beyond disease progression: a systematic review of published studies. Tumori 2010; 96:385 - 91; PMID: 20845797
- Venkatesh S, Lipper RA. Role of the development scientist in compound lead selection and optimization. J Pharm Sci 2000; 89:145 - 54; http://dx.doi.org/10.1002/(SICI)1520-6017(200002)89:2<145::AID-JPS2>3.0.CO;2-6; PMID: 10688744
- Clarke R. The role of preclinical animal models in breast cancer drug development. Breast Cancer Res 2009; 11:Suppl 3 S22; PMID: 20030874
- Kola I, Landis J. Can the pharmaceutical industry reduce attrition rates?. Nat Rev Drug Discov 2004; 3:711 - 5; http://dx.doi.org/10.1038/nrd1470; PMID: 15286737
- Kinders R, Parchment RE, Ji J, Kummar S, Murgo AJ, Gutierrez M, et al. Phase 0 clinical trials in cancer drug development: from FDA guidance to clinical practice. Mol Interv 2007; 7:325 - 34; http://dx.doi.org/10.1124/mi.7.6.9; PMID: 18199854
- Burchill SA. What do, can and should we learn from models to evaluate potential anticancer agents?. Future Oncol 2006; 2:201 - 11; http://dx.doi.org/10.2217/14796694.2.2.201; PMID: 16563089
- Voskoglou-Nomikos T, Pater JL, Seymour L. Clinical predictive value of the in vitro cell line, human xenograft, and mouse allograft preclinical cancer models. Clin Cancer Res 2003; 9:4227 - 39; PMID: 14519650
- Hindié E, Groheux D, Brenot-Rossi I, Rubello D, Moretti JL, Espie M. The sentinel node procedure in breast cancer: nuclear medicine as the starting point. J Nucl Med 2011; 52:405 - 14; http://dx.doi.org/10.2967/jnumed.110.081711; PMID: 21321267
- Fantozzi A, Christofori G. Mouse models of breast cancer metastasis. Breast Cancer Res 2006; 8:212; http://dx.doi.org/10.1186/bcr1530; PMID: 16887003
- Doré-Savard L, Otis V, Belleville K, Lemire M, Archambault M, Tremblay L, et al. Behavioral, medical imaging and histopathological features of a new rat model of bone cancer pain. PLoS ONE 2010; 5:e13774; http://dx.doi.org/10.1371/journal.pone.0013774; PMID: 21048940
- Chia YH, Ellis MJ, Ma CX. Neoadjuvant endocrine therapy in primary breast cancer: indications and use as a research tool. Br J Cancer 2010; 103:759 - 64; http://dx.doi.org/10.1038/sj.bjc.6605845; PMID: 20700118
- Eroles P, Bosch A, Bermejo B, Lluch A. Mechanisms of resistance to hormonal treatment in breast cancer. Clin Transl Oncol 2010; 12:246 - 52; http://dx.doi.org/10.1007/s12094-010-0500-1; PMID: 20462833
- Hayes E, Nicholson RI, Hiscox S. Acquired endocrine resistance in breast cancer: implications for tumour metastasis. Front Biosci 2011; 16:838 - 48; http://dx.doi.org/10.2741/3723; PMID: 21196206
- Perou CM, Sorlie T, Eisen MB, van de Rijn M, Jeffrey SS, Rees CA, et al. Molecular portraits of human breast tumours. Nature 2000; 406:747 - 52; http://dx.doi.org/10.1038/35021093; PMID: 10963602
- Sorlie T, Perou CM, Tibshirani R, Aas T, Geisler S, Johnsen H, et al. Gene expression patterns of breast carcinomas distinguish tumor subclasses with clinical implications. Proc Natl Acad Sci USA 2001; 98:10869 - 74; http://dx.doi.org/10.1073/pnas.191367098; PMID: 11553815
- Sørlie T, Tibshirani R, Parker J, Hastie T, Marron JS, Nobel A, et al. Repeated observation of breast tumor subtypes in independent gene expression data sets. Proc Natl Acad Sci USA 2003; 100:8418 - 23; http://dx.doi.org/10.1073/pnas.0932692100; PMID: 12829800
- Thompson HJ, Singh M. Rat models of premalignant breast disease. J Mammary Gland Biol Neoplasia 2000; 5:409 - 20; http://dx.doi.org/10.1023/A:1009582012493; PMID: 14973385
- Kim IS, Baek SH. Mouse models for breast cancer metastasis. Biochem Biophys Res Commun.
- Lacroix M, Leclercq G. Relevance of breast cancer cell lines as models for breast tumours: an update. Breast Cancer Res Treat 2004; 83:249 - 89; http://dx.doi.org/10.1023/B:BREA.0000014042.54925.cc; PMID: 14758095
- Guy CT, Webster MA, Schaller M, Parsons TJ, Cardiff RD, Muller WJ. Expression of the neu protooncogene in the mammary epithelium of transgenic mice induces metastatic disease. Proc Natl Acad Sci USA 1992; 89:10578 - 82; http://dx.doi.org/10.1073/pnas.89.22.10578; PMID: 1359541
- Kuperwasser C, Dessain S, Bierbaum BE, Garnet D, Sperandio K, Gauvin GP, et al. A mouse model of human breast cancer metastasis to human bone. Cancer Res 2005; 65:6130 - 8; http://dx.doi.org/10.1158/0008-5472.CAN-04-1408; PMID: 16024614
- Blouin S, Basle MF, Chappard D. Rat models of bone metastases. Clin Exp Metastasis 2005; 22:605 - 14; http://dx.doi.org/10.1007/s10585-006-9002-5; PMID: 16670964
- Hu Z, Zhang Z, Guise T, Seth P. Systemic delivery of an oncolytic adenovirus expressing soluble transforming growth factor-beta receptor II-Fc fusion protein can inhibit breast cancer bone metastasis in a mouse model. Hum Gene Ther 2010; 21:1623 - 9; http://dx.doi.org/10.1089/hum.2010.018; PMID: 20712434
- Rouzier R, Perou CM, Symmans WF, Ibrahim N, Cristofanilli M, Anderson K, et al. Breast cancer molecular subtypes respond differently to preoperative chemotherapy. Clin Cancer Res 2005; 11:5678 - 85; http://dx.doi.org/10.1158/1078-0432.CCR-04-2421; PMID: 16115903
- Andrechek ER, Nevins JR. Mouse models of cancers: opportunities to address heterogeneity of human cancer and evaluate therapeutic strategies. J Mol Med 2010; 88:1095 - 100; http://dx.doi.org/10.1007/s00109-010-0644-z; PMID: 20574808
- Maglione JE, McGoldrick ET, Young LJ, Namba R, Gregg JP, Liu L, et al. Polyomavirus middle T-induced mammary intraepithelial neoplasia outgrowths: single origin, divergent evolution, and multiple outcomes. Mol Cancer Ther 2004; 3:941 - 53; PMID: 15299077
- Meyer DS, Brinkhaus H, Muller U, Muller M, Cardiff RD, Bentires-Alj M. Luminal expression of pik3ca mutant h1047r in the mammary gland induces heterogeneous tumors. Cancer Res 2011; 71:4344 - 51; http://dx.doi.org/10.1158/0008-5472.CAN-10-3827; PMID: 21482677
- Schneider SL, Fuqua SA, Speeg KV, Tandon AK, McGuire WL. Isolation and characterization of an adriamycin-resistant breast tumor cell line. In Vitro Cell Dev Biol 1990; 26:621 - 8; http://dx.doi.org/10.1007/BF02624212; PMID: 1972704
- Medina D. Chemical carcinogenesis of rat and mouse mammary glands. Breast Dis 2007; 28:63 - 8; PMID: 18057544
- Wang B, Kennan WS, Yasukawa-Barnes J, Lindstrom MJ, Gould MN. Difference in the response of neu and ras oncogene-induced rat mammary carcinomas to early and late ovariectomy. Cancer Res 1992; 52:4102 - 5; PMID: 1353410
- Gould MN. Rodent models for the study of etiology, prevention and treatment of breast cancer. Semin Cancer Biol 1995; 6:147 - 52; http://dx.doi.org/10.1006/scbi.1995.0023; PMID: 7495982
- Smits BM, Cotroneo MS, Haag JD, Gould MN. Genetically engineered rat models for breast cancer. Breast Dis 2007; 28:53 - 61; PMID: 18057543
- Zarbl H. Toxicogenomic analyses of genetic susceptibility to mammary gland carcinogenesis in rodents: implications for human breast cancer. Breast Dis 2007; 28:87 - 105; PMID: 18057546
- Kim JB, O'Hare MJ, Stein R. Models of breast cancer: is merging human and animal models the future?. Breast Cancer Res 2004; 6:22 - 30; http://dx.doi.org/10.1186/bcr645; PMID: 14680482
- Bombonati A, Sgroi DC. The molecular pathology of breast cancer progression. J Pathol 2011; 223:307 - 17; http://dx.doi.org/10.1002/path.2808; PMID: 21125683
- Russo J, Russo IH. Atlas and histologic classification of tumors of the rat mammary gland. J Mammary Gland Biol Neoplasia 2000; 5:187 - 200; http://dx.doi.org/10.1023/A:1026443305758; PMID: 11149572
- Nandi S, Guzman RC, Yang J. Hormones and mammary carcinogenesis in mice, rats, and humans: a unifying hypothesis. Proc Natl Acad Sci USA 1995; 92:3650 - 7; http://dx.doi.org/10.1073/pnas.92.9.3650; PMID: 7731959
- Huang G, Tong C, Kumbhani DS, Ashton C, Yan H, Ying QL. Beyond knockout rats: new insights into finer genome manipulation in rats. Cell Cycle 2011; 10:1059 - 66; http://dx.doi.org/10.4161/cc.10.7.15233; PMID: 21383544
- Matsuoka Y, Hamaguchi T, Fukamachi K, Yoshida M, Watanabe G, Taya K, et al. Molecular analysis of rat mammary carcinogenesis: an approach from carcinogenesis research to cancer prevention. Med Mol Morphol 2007; 40:185 - 90; http://dx.doi.org/10.1007/s00795-007-0369-4; PMID: 18085376
- Pagès F, Galon J, Dieu-Nosjean MC, Tartour E, Sautes-Fridman C, Fridman WH. Immune infiltration in human tumors: a prognostic factor that should not be ignored. Oncogene 2010; 29:1093 - 102; http://dx.doi.org/10.1038/onc.2009.416; PMID: 19946335
- de Visser KE, Eichten A, Coussens LM. Paradoxical roles of the immune system during cancer development. Nat Rev Cancer 2006; 6:24 - 37; http://dx.doi.org/10.1038/nrc1782; PMID: 16397525
- Bosma MJ, Carroll AM. The SCID mouse mutant: definition, characterization, and potential uses. Annu Rev Immunol 1991; 9:323 - 50; http://dx.doi.org/10.1146/annurev.iy.09.040191.001543; PMID: 1910681
- Carroll AM, Bosma MJ. T-lymphocyte development in scid mice is arrested shortly after the initiation of T-cell receptor delta gene recombination. Genes Dev 1991; 5:1357 - 66; http://dx.doi.org/10.1101/gad.5.8.1357; PMID: 1869046
- Fridman R, Kibbey MC, Royce LS, Zain M, Sweeney M, Jicha DL, et al. Enhanced tumor growth of both primary and established human and murine tumor cells in athymic mice after coinjection with Matrigel. J Natl Cancer Inst 1991; 83:769 - 74; http://dx.doi.org/10.1093/jnci/83.11.769; PMID: 1789823
- Steel GG, Courtenay VD, Rostom AY. Improved immune-suppression techniques for the exongrafting of human tumours. Br J Cancer 1978; 37:224 - 30; http://dx.doi.org/10.1038/bjc.1978.30; PMID: 343803
- Goodman MM, McCullough JL, Biren CA, Barr RJ. A model of human melanoma in cyclosporine-immunosuppressed rats. J Invest Dermatol 1987; 88:141 - 4; http://dx.doi.org/10.1111/1523-1747.ep12525289; PMID: 3492567
- Denkert C, Darb-Esfahani S, Loibl S, Anagnostopoulos I, Johrens K. Anti-cancer immune response mechanisms in neoadjuvant and targeted therapy. Semin Immunopathol 2011.
- Carson WE 3rd, Shapiro CL, Crespin TR, Thornton LM, Andersen BL. Cellular immunity in breast cancer patients completing taxane treatment. Clin Cancer Res 2004; 10:3401 - 9; http://dx.doi.org/10.1158/1078-0432.CCR-1016-03; PMID: 15161695
- Chan OT, Yang LX. The immunological effects of taxanes. Cancer Immunol Immunother 2000; 49:181 - 5; http://dx.doi.org/10.1007/s002620000122; PMID: 10941900
- Santosuosso M, Divangahi M, Zganiacz A, Xing Z. Reduced tissue macrophage population in the lung by anticancer agent cyclophosphamide: restoration by local granulocyte macrophage-colony-stimulating factor gene transfer. Blood 2002; 99:1246 - 52; http://dx.doi.org/10.1182/blood.V99.4.1246; PMID: 11830472
- Xia TS, Wang J, Yin H, Ding Q, Zhang YF, Yang HW, et al. Human tissue-specific microenvironment: an essential requirement for mouse models of breast cancer. Oncol Rep 2010; 24:203 - 11; PMID: 20514463
- Wagner KU. Models of breast cancer: quo vadis, animal modeling?. Breast Cancer Res 2004; 6:31 - 8; http://dx.doi.org/10.1186/bcr723; PMID: 14680483
- Vernon AE, Bakewell SJ, Chodosh LA. Deciphering the molecular basis of breast cancer metastasis with mouse models. Rev Endocr Metab Disord 2007; 8:199 - 213; http://dx.doi.org/10.1007/s11154-007-9041-5; PMID: 17657606
- Aslakson CJ, Miller FR. Selective events in the metastatic process defined by analysis of the sequential dissemination of subpopulations of a mouse mammary tumor. Cancer Res 1992; 52:1399 - 405; PMID: 1540948
- Tao K, Fang M, Alroy J, Sahagian GG. Imagable 4T1 model for the study of late stage breast cancer. BMC Cancer 2008; 8:228; http://dx.doi.org/10.1186/1471-2407-8-228; PMID: 18691423
- Pulaski BA, Ostrand-Rosenberg S. Mouse 4T1 breast tumor model. Curr Protoc Immunol 2001; Chapter 20:Unit 20 2.
- Chumsri S, Howes T, Bao T, Sabnis G, Brodie A. Aromatase, aromatase inhibitors, and breast cancer. J Steroid Biochem Mol Biol 2011; 125:13 - 22; http://dx.doi.org/10.1016/j.jsbmb.2011.02.001; PMID: 21335088
- Lipton A, Santen RJ, Santner SJ, Harvey HA, Sanders SI, Matthews YL. Prognostic value of breast cancer aromatase. Cancer 1992; 70:1951 - 5; http://dx.doi.org/10.1002/1097-0142(19921001)70:7<1951::AID-CNCR2820700723>3.0.CO;2-#; PMID: 1525771
- Jefcoate CR, Liehr JG, Santen RJ, Sutter TR, Yager JD, Yue W, et al. Tissue-specific synthesis and oxidative metabolism of estrogens. J Natl Cancer Inst Monogr 2000; 95 - 112; PMID: 10963622
- Yue W, Wang JP, Hamilton CJ, Demers LM, Santen RJ. In situ aromatization enhances breast tumor estradiol levels and cellular proliferation. Cancer Res 1998; 58:927 - 32; PMID: 9500452
- Ferguson AT, Davidson NE. Regulation of estrogen receptor alpha function in breast cancer. Crit Rev Oncog 1997; 8:29 - 46; PMID: 9516085
- Cavalieri E, Rogan E. Catechol quinones of estrogens in the initiation of breast, prostate, and other human cancers: keynote lecture. Ann N Y Acad Sci 2006; 1089:286 - 301; http://dx.doi.org/10.1196/annals.1386.042; PMID: 17261777
- Santen R. Estrogen mediation of breast tumor formation involves estrogen receptor-dependent, as well as independent, genotoxic effects. Ann N Y Acad Sci 2009; 1155:132 - 40; http://dx.doi.org/10.1111/j.1749-6632.2008.03685.x; PMID: 19250200
- Clevenger CV, Gadd SL, Zheng J. New mechanisms for PRLr action in breast cancer. Trends Endocrinol Metab 2009; 20:223 - 9; http://dx.doi.org/10.1016/j.tem.2009.03.001; PMID: 19535262
- Maus MV, Reilly SC, Clevenger CV. Prolactin as a chemoattractant for human breast carcinoma. Endocrinology 1999; 140:5447 - 50; http://dx.doi.org/10.1210/en.140.11.5447; PMID: 10537179
- Perks CM, Keith AJ, Goodhew KL, Savage PB, Winters ZE, Holly JM. Prolactin acts as a potent survival factor for human breast cancer cell lines. 2005. Br J Cancer 2004; 91:305 - 11; http://dx.doi.org/10.1038/sj.bjc.6601947; PMID: 15213724
- Lunt SJ, Kalliomaki TM, Brown A, Yang VX, Milosevic M, Hill RP. Interstitial fluid pressure, vascularity and metastasis in ectopic, orthotopic and spontaneous tumours. BMC Cancer 2008; 8:2; http://dx.doi.org/10.1186/1471-2407-8-2; PMID: 18179711
- Wilmanns C, Fan D, O'Brian CA, Bucana CD, Fidler IJ. Orthotopic and ectopic organ environments differentially influence the sensitivity of murine colon carcinoma cells to doxorubicin and 5-fluorouracil. Int J Cancer 1992; 52:98 - 104; http://dx.doi.org/10.1002/ijc.2910520118; PMID: 1500231
- Zechmann CM, Woenne EC, Brix G, Radzwill N, Ilg M, Bachert P, et al. Impact of stroma on the growth, microcirculation, and metabolism of experimental prostate tumors. Neoplasia 2007; 9:57 - 67; http://dx.doi.org/10.1593/neo.06688; PMID: 17325744
- Tsuzuki Y, Mouta Carreira C, Bockhorn M, Xu L, Jain RK, Fukumura D. Pancreas microenvironment promotes VEGF expression and tumor growth: novel window models for pancreatic tumor angiogenesis and microcirculation. Lab Invest 2001; 81:1439 - 51; PMID: 11598156
- Franco OE, Shaw AK, Strand DW, Hayward SW. Cancer associated fibroblasts in cancer pathogenesis. Semin Cell Dev Biol 2010; 21:33 - 9; http://dx.doi.org/10.1016/j.semcdb.2009.10.010; PMID: 19896548
- Bhowmick NA, Neilson EG, Moses HL. Stromal fibroblasts in cancer initiation and progression. Nature 2004; 432:332 - 7; http://dx.doi.org/10.1038/nature03096; PMID: 15549095
- Kessenbrock K, Plaks V, Werb Z. Matrix metalloproteinases: regulators of the tumor microenvironment. Cell 2010; 141:52 - 67; http://dx.doi.org/10.1016/j.cell.2010.03.015; PMID: 20371345
- Radisky ES, Radisky DC. Matrix metalloproteinase-induced epithelial-mesenchymal transition in breast cancer. J Mammary Gland Biol Neoplasia 2010; 15:201 - 12; http://dx.doi.org/10.1007/s10911-010-9177-x; PMID: 20440544
- Gialeli C, Theocharis AD, Karamanos NK. Roles of matrix metalloproteinases in cancer progression and their pharmacological targeting. FEBS J 2011; 278:16 - 27; http://dx.doi.org/10.1111/j.1742-4658.2010.07919.x; PMID: 21087457
- Pupa SM, Menard S, Forti S, Tagliabue E. New insights into the role of extracellular matrix during tumor onset and progression. J Cell Physiol 2002; 192:259 - 67; http://dx.doi.org/10.1002/jcp.10142; PMID: 12124771
- Coussens LM, Werb Z. Inflammation and cancer. Nature 2002; 420:860 - 7; http://dx.doi.org/10.1038/nature01322; PMID: 12490959
- Cole LK, Jacobs RL, Vance DE. Tamoxifen induces triacylglycerol accumulation in the mouse liver by activation of fatty acid synthesis. Hepatology.
- Tabruyn SP, Griffioen AW. Molecular pathways of angiogenesis inhibition. Biochem Biophys Res Commun 2007; 355:1 - 5; http://dx.doi.org/10.1016/j.bbrc.2007.01.123; PMID: 17276388
- Döme B, Hendrix MJ, Paku S, Tovari J, Timar J. Alternative vascularization mechanisms in cancer: Pathology and therapeutic implications. Am J Pathol 2007; 170:1 - 15; PMID: 17200177
- Evan GI, Vousden KH. Proliferation, cell cycle and apoptosis in cancer. Nature 2001; 411:342 - 8; http://dx.doi.org/10.1038/35077213; PMID: 11357141
- Chen JH, Lin YC, Huang YS, Chen TJ, Lin WY, Han KW. Induction of VX2 carcinoma in rabbit liver: comparison of two inoculation methods. Lab Anim 2004; 38:79 - 84; http://dx.doi.org/10.1258/00236770460734434; PMID: 14979992
- Fei XF, Zhang QB, Dong J, Diao Y, Wang ZM, Li RJ, et al. Development of clinically relevant orthotopic xenograft mouse model of metastatic lung cancer and glioblastoma through surgical tumor tissues injection with trocar. J Exp Clin Cancer Res 2010; 29:84; http://dx.doi.org/10.1186/1756-9966-29-84; PMID: 20587035
- Thorstensen O, Isberg B, Svahn U, Jorulf H, Venizelos N, Jaremko G. Experimental tissue transplantation using a biopsy instrument and radiologic methods. Invest Radiol 1994; 29:469 - 71; http://dx.doi.org/10.1097/00004424-199404000-00015; PMID: 8034455
- Carlsson G, Gullberg B, Hafstrom L. Estimation of liver tumor volume using different formulas - an experimental study in rats. J Cancer Res Clin Oncol 1983; 105:20 - 3; http://dx.doi.org/10.1007/BF00391826; PMID: 6833336
- Tomayko MM, Reynolds CP. Determination of subcutaneous tumor size in athymic (nude) mice. Cancer Chemother Pharmacol 1989; 24:148 - 54; http://dx.doi.org/10.1007/BF00300234; PMID: 2544306
- O'Neill K, Lyons SK, Gallagher WM, Curran KM, Byrne AT. Bioluminescent imaging: a critical tool in pre-clinical oncology research. J Pathol 2010; 220:317 - 27; PMID: 19967724
- Bremer C, Ntziachristos V, Weissleder R. Optical-based molecular imaging: contrast agents and potential medical applications. Eur Radiol 2003; 13:231 - 43; PMID: 12598985
- Tiffen JC, Bailey CG, Ng C, Rasko JE, Holst J. Luciferase expression and bioluminescence does not affect tumor cell growth in vitro or in vivo. Mol Cancer 2010; 9:299; http://dx.doi.org/10.1186/1476-4598-9-299; PMID: 21092230
- Sanz L, Santos-Valle P, Alonso-Camino V, Salas C, Serrano A, Vicario JL, et al. Long-term in vivo imaging of human angiogenesis: critical role of bone marrow-derived mesenchymal stem cells for the generation of durable blood vessels. Microvasc Res 2008; 75:308 - 14; http://dx.doi.org/10.1016/j.mvr.2007.11.007; PMID: 18252255
- Hong H, Yang Y, Zhang Y, Cai W. Non-invasive cell tracking in cancer and cancer therapy. Curr Top Med Chem 2010; 10:1237 - 48; http://dx.doi.org/10.2174/156802610791384234; PMID: 20388105
- Naik S, Piwnica-Worms D. Real-time imaging of beta-catenin dynamics in cells and living mice. Proc Natl Acad Sci USA 2007; 104:17465 - 70; http://dx.doi.org/10.1073/pnas.0704465104; PMID: 17954915
- Koba W, Kim K, Lipton ML, Jelicks L, Das B, Herbst L, et al. Imaging devices for use in small animals. Semin Nucl Med 2011; 41:151 - 65; http://dx.doi.org/10.1053/j.semnuclmed.2010.12.003; PMID: 21440693
- Piatkevich KD, Verkhusha VV. Advances in engineering of fluorescent proteins and photoactivatable proteins with red emission. Curr Opin Chem Biol 2010; 14:23 - 9; http://dx.doi.org/10.1016/j.cbpa.2009.10.011; PMID: 19914857
- Shu X, Royant A, Lin MZ, Aguilera TA, Lev-Ram V, Steinbach PA, et al. Mammalian expression of infrared fluorescent proteins engineered from a bacterial phytochrome. Science 2009; 324:804 - 7; http://dx.doi.org/10.1126/science.1168683; PMID: 19423828
- Nguyen QT, Olson ES, Aguilera TA, Jiang T, Scadeng M, Ellies LG, et al. Surgery with molecular fluorescence imaging using activatable cell-penetrating peptides decreases residual cancer and improves survival. Proc Natl Acad Sci USA 2010; 107:4317 - 22; http://dx.doi.org/10.1073/pnas.0910261107; PMID: 20160097
- Olson ES, Jiang T, Aguilera TA, Nguyen QT, Ellies LG, Scadeng M, et al. Activatable cell penetrating peptides linked to nanoparticles as dual probes for in vivo fluorescence and MR imaging of proteases. Proc Natl Acad Sci USA 2010; 107:4311 - 6; http://dx.doi.org/10.1073/pnas.0910283107; PMID: 20160077
- Ting R, Aguilera TA, Crisp JL, Hall DJ, Eckelman WC, Vera DR, et al. Fast 18F labeling of a near-infrared fluorophore enables positron emission tomography and optical imaging of sentinel lymph nodes. Bioconjug Chem 2010; 21:1811 - 9; http://dx.doi.org/10.1021/bc1001328; PMID: 20873712
- Holdsworth DW. Micro-CT in small animal and specimen imaging. Trends Biotechnol 2002.
- Dufort S, Sancey L, Wenk C, Josserand V, Coll JL. Optical small animal imaging in the drug discovery process. Biochim Biophys Acta 2010; 1798:2266 - 73; http://dx.doi.org/10.1016/j.bbamem.2010.03.016; PMID: 20346346
- Levin CS. Primer on molecular imaging technology. Eur J Nucl Med Mol Imaging 2005; 32:Suppl 2 S325 - 45; http://dx.doi.org/10.1007/s00259-005-1973-y; PMID: 16341514
- Monteil J, Dutour A, Akla B, Chianea T, Le Brun V, Grossin L, et al. In vivo follow-up of rat tumor models with 2-deoxy-2-[F-18]fluoro-D-glucose/dual-head coincidence gamma camera imaging. Mol Imaging Biol 2005; 7:220 - 8; http://dx.doi.org/10.1007/s11307-005-4115-9; PMID: 15912426
- Therasse P, Arbuck SG, Eisenhauer EA, Wanders J, Kaplan RS, Rubinstein L, et al. New guidelines to evaluate the response to treatment in solid tumors. European Organization for Research and Treatment of Cancer, National Cancer Institute of the United States, National Cancer Institute of Canada. J Natl Cancer Inst 2000; 92:205 - 16; http://dx.doi.org/10.1093/jnci/92.3.205; PMID: 10655437
- Padhani AR, Ollivier L. The RECIST (Response Evaluation Criteria in Solid Tumors) criteria: implications for diagnostic radiologists. Br J Radiol 2001; 74:983 - 6; PMID: 11709461
- Blanco MA, Kang Y. Signaling pathways in breast cancer metastasis - novel insights from functional genomics. Breast Cancer Res 2011; 13:206; http://dx.doi.org/10.1186/bcr2831; PMID: 21457525
- Goodison S, Sun Y, Urquidi V. Derivation of cancer diagnostic and prognostic signatures from gene expression data. Bioanalysis 2010; 2:855 - 62; http://dx.doi.org/10.4155/bio.10.35; PMID: 21083217
- Polyak K. Molecular markers for the diagnosis and management of ductal carcinoma in situ. J Natl Cancer Inst Monogr 2010; 2010:210 - 3; http://dx.doi.org/10.1093/jncimonographs/lgq019; PMID: 20956832
- Slamon DJ, Leyland-Jones B, Shak S, Fuchs H, Paton V, Bajamonde A, et al. Use of chemotherapy plus a monoclonal antibody against HER2 for metastatic breast cancer that overexpresses HER2. N Engl J Med 2001; 344:783 - 92; http://dx.doi.org/10.1056/NEJM200103153441101; PMID: 11248153
- Hattori N, Okochi-Takada E, Kikuyama M, Wakabayashi M, Yamashita S, Ushijima T. Methylation silencing of angiopoietin-like 4 in rat and human mammary carcinomas. Cancer Sci 2011; 102:1337 - 43; http://dx.doi.org/10.1111/j.1349-7006.2011.01955.x; PMID: 21489049
- Demircan B, Dyer LM, Gerace M, Lobenhofer EK, Robertson KD, Brown KD. Comparative epigenomics of human and mouse mammary tumors. Genes Chromosomes Cancer 2009; 48:83 - 97; http://dx.doi.org/10.1002/gcc.20620; PMID: 18836996
- Szpirer C.. Cancer research in rat models. Methods Mol Biol 2010; 597:445 - 58; http://dx.doi.org/10.1007/978-1-60327-389-3_30; PMID: 20013251
- Coleman RE. Future directions in the treatment and prevention of bone metastases. Am J Clin Oncol 2002; 25:S32 - 8; http://dx.doi.org/10.1097/00000421-200212001-00006; PMID: 12562049
- Mundy GR. Metastasis to bone: causes, consequences and therapeutic opportunities. Nat Rev Cancer 2002; 2:584 - 93; http://dx.doi.org/10.1038/nrc867; PMID: 12154351
- Engel LW, Young NA. Human breast carcinoma cells in continuous culture: a review. Cancer Res 1978; 38:4327 - 39; PMID: 212193
- Chow LW, Cheung MN, Loo WT, Guan XY. A rat cell line derived from DMBA-induced mammary carcinoma. Life Sci 2003; 73:27 - 40; http://dx.doi.org/10.1016/S0024-3205(03)00253-4; PMID: 12726884
- Eil C, Douglass EC, Rosenburg SM, Kano-Sueoka T. Receptor characteristics of the rat mammary carcinoma cell line 64-24. Cancer Res 1981; 41:42 - 8; PMID: 6256064
- Oikawa T, Matsuzawa A, Iwaguchi T. Progression from hormone dependence to autonomy and angiogenesis in mouse mammary tumours. Br J Cancer 1986; 54:91 - 6; http://dx.doi.org/10.1038/bjc.1986.156; PMID: 2425838
- Nakanishi H, Taylor RM, Chrest FJ, Masui T, Utsumi K, Tatematsu M, et al. Progression of hormone-dependent adenocarcinoma cells to hormone-independent spindle carcinoma cells in vitro in a clonal spontaneous rat mammary tumor cell line. Cancer Res 1995; 55:399 - 407; PMID: 7529136
- Peterson JK, Houghton PJ. Integrating pharmacology and in vivo cancer models in preclinical and clinical drug development. Eur J Cancer 2004; 40:837 - 44; http://dx.doi.org/10.1016/j.ejca.2004.01.003; PMID: 15120039
- Paoloni M, Khanna C. Translation of new cancer treatments from pet dogs to humans. Nat Rev Cancer 2008; 8:147 - 56; http://dx.doi.org/10.1038/nrc2273; PMID: 18202698
- Knapp DW, Waters DJ. Naturally occurring cancer in pet dogs: important models for developing improved cancer therapy for humans. Mol Med Today 1997; 3:8 - 11; http://dx.doi.org/10.1016/S1357-4310(96)20031-0; PMID: 9021736
- Gordon I, Paoloni M, Mazcko C, Khanna C. The Comparative Oncology Trials Consortium: using spontaneously occurring cancers in dogs to inform the cancer drug development pathway. PLoS Med 2009; 6:e1000161; http://dx.doi.org/10.1371/journal.pmed.1000161; PMID: 19823573