Abstract
Poor prognosis cancers, such as pancreatic cancer, represent inherent challenges for ceramide-based nanotherapeutics due to metabolic pathways, which neutralize ceramide to less toxic or pro-oncogenic metabolites. We have recently developed a novel 80 nanometer diameter liposomal formulation that incorporates 30 molar percent C6-ceramide, a bioactive lipid that is pro-apoptotic to many cancer cells, but not to normal cells. In this manuscript, we evaluated the efficacy of combining nanoliposomal C6-ceramide (Lip-C6) with either gemcitabine or an inhibitor of glucosylceramide synthase. We first assessed the biological effect of Lip-C6 in PANC-1 cells, a gemcitabine-resistant human pancreatic cancer cell line, and found that low doses alone did not induce cell toxicity. However, cytotoxicity was achieved by combining Lip-C6 with either non-toxic sub-therapeutic concentrations of gemcitabine or with the glucosylceramide synthase inhibitor D-threo-1-phenyl-2-decanoylamino-3-morpholino-1-propanol (PDMP). Furthermore, these combinations with Lip-C6 cooperatively inhibited PANC-1 tumor growth in vivo. Mechanistically, Lip-C6 inhibited pro-survival Akt and Erk signaling, whereas the nucleoside analog gemcitabine did not. Furthermore, by including PDMP within the nanoliposomes, which halted ceramide neutralization as evidenced by LC-MS3, the cytotoxic effects of Lip-C6 were enhanced. Collectively, we have demonstrated that nanoliposomal ceramide can be an effective anti-pancreatic cancer therapeutic in combination with gemcitabine or an inhibitor of ceramide neutralization.
Introduction
Pancreatic cancer is the fourth leading cause of cancer-related deaths in the United States.Citation1 The high mortality of the disease is largely attributed to multidrug resistance and metastases. New therapeutic modalities delivering potent anti-neoplastic agents that target pro-survival signaling cascades may become an important therapeutic modality. The use of pro-apoptotic agents, such as ceramide which preferentially induces apoptosis in transformed cells, may offer the ability to enhance the efficacy of existing treatments without further exacerbating toxicities.Citation2,Citation3 Moreover, delivering these agents within nanoscale packages has the potential to overcome mechanisms of multi-drug resistance.Citation4
Sphingolipids have been recognized as important lipid-based mediators of cell growth, death and differentiation. The pro-apoptotic sphingolipid metabolite, ceramide, is endogenously generated by chemo- or radio-therapies,Citation5–Citation7 and exogenous short-chain ceramide has been shown to augment chemotherapy-induced cytotoxicity.Citation7–Citation9 One of the exciting aspects of using ceramide as a chemotherapeutic is the preferential selectivity for inducing apoptosis in cancer cells. For example, we previously demonstrated that nanoliposomal C6-ceramide induces cell growth arrest and apoptosis in breast cancer cells and melanomas, but not non-transformed mammary gland epithelial cells or melanocytes.Citation2,Citation10,Citation11 Mechanisms underlying these observations are not completely understood, but may reflect decreased metabolism of the nanoscale formulations in cancer cells and/or enhanced promitogenic signaling in transformed cells. Specific promitogenic signaling cascades such as protein kinase C (PKC), Erk and Akt, are activated or overexpressed in multiple cancers.Citation12–Citation14 Mechanistically, ceramide forms structured membrane microdomains, recruiting PKCζ to pre-formed Aktsignalsomes.Citation15 Ceramide-bound PKCζ inactivates pro-survival Akt via phosphorylation at serine 34.Citation15,Citation16 In a similar scenario, we have shown that ceramide inhibits PKCε/Erk interactions.Citation17
Despite the increased solubility of short-chain ceramide, its therapeutic efficacy is limited due to its impermeability and to its tendency to precipitate in biological fluids. To improve solubility and to protect from metabolism, systemic delivery for ceramide has embraced nano “solutions.” Recent reports have established the utility of ceramide delivery in nanoliposomes for the systemic treatment of breast cancer, hepatocellular carcinoma, large granular lymphocytic leukemia and melanoma animal models.Citation2,Citation10,Citation11,Citation18,Citation19 The Nanotechnology Characterization Laboratory of the National Cancer Institute has recently reported the pharmacokinetic profile, and the lack of toxicology, of ceramide-enriched nanoliposomes (http://ncl.cancer.gov/MK_022207_073007.pdf). Further limitations of ceramide as an anticancer therapeutic arises from metabolism into pro-mitogenic phosphorylated derivatives (ceramide-1-phosphate and sphingosine-1-phosphate), which have been implicated in multidrug resistant cellular phenotypes.Citation20–Citation22 Recently, we have shown that the fate of exogenously delivered C6-ceramide is cell type dependent and concentration dependent.Citation23 For example, in PANC-1 cells, higher concentrations of C6-ceramide were preferentially metabolized to glucosylceramide, a lipid linked to multidrug resistant phenotypes. Therefore, incorporation of glucosylceramide synthase inhibitors could improve the therapeutic efficacy of nanoliposomal ceramide.
In the present study, we successfully deliver C6-ceramide within non-toxic nanoliposomal formulations (Lip-C6) to the drug-resistant PANC-1 human pancreatic cancer model. Multiple labs, including our own, have reported that the PANC-1 cell line is more chemoresistant than other cell lines, often exhibiting higher IC50 values.Citation24–Citation29 In this study, we also demonstrate that D-threo-1-phenyl-2-decanoylamino-3-morpholino-1-propanol (PDMP), a glucosylceramide synthase inhibitor and gemcitabine, a nucleoside analog, enhance the antitumor activity of Lip-C6. We show that the biological effect of Lip-C6 is achieved through inhibition of Akt phosphorylation, and suggest that the distinctive action of the anti-metabolite gemcitabine can be used to prime the PANC-1 cells to the action of Lip-C6. Additionally, by using a nanoliposomal combination of PDMP and C6-ceramide (Lip-C6/PDMP), we show that the inhibition of glucosylceramide synthase improves the anti-pancreatic cancer action of C6-ceramide. Altogether this study demonstrates the utility of combinatorial C6-ceramide-containing nanotherapeutics as a potential new strategy in treating drug-resistant human pancreatic cancer.
Results
Lip-C6 cytotoxicity is synergistically enhanced by gemcitabine or Lip-PDMP.
We have previously reported that Lip-C6 induces cytotoxicity in a variety of cancer cell lines.Citation2,Citation10,Citation11,Citation18,Citation19 In this study, we evaluated the ability of Lip-C6, gemcitabine and Lip-PDMP, to trigger cell death of PANC-1 pancreatic cancer cells. Gemcitabine is a FDA-approved chemotherapeutic that is routinely used in the treatment of pancreatic cancer. We formulated Lip-PDMP as a nanoliposomal formulation designed to prevent the neutralization of ceramide to glucosylceramide. In this study, we hypothesized that gemcitabine or Lip-PDMP could improve the efficacy of Lip-C6. In dose and time evaluations of cellular viability, the IC50 in PANC-1 cells for Lip-C6 and Lip-PDMP at 48 h was determined to be approximately 26 and 48 µM, respectively (). In contrast, the IC50 for gemcitabine in PANC-1 cells was extrapolated to be substantially greater than 1,000 µM. This observation was consistent with previously published observations that indicated PANC-1 cells were highly resistant to gemcitabine.Citation30 Lip-C6, gemcitabine and Lip-PDMP were evaluated in combination using the Chou-Talalay method to quantify potential synergistic cell killing (). The combination index (CI) for different concentrations of Lip-C6 and gemcitabine revealed that these anticancer agents acted in synergy with each other. However, the CI for different concentrations of Lip-C6 and Lip-PDMP, or Lip-PDMP and gemcitabine, revealed that these agents could synergize with or antagonize each other. The common agent to these contradictory findings was Lip-PDMP, a regulator of sphingolipid metabolism that potentially could influence many different pro-survival or pro-apoptotic sphingolipids.
We next utilized the TUNEL method (terminal deoxynucleotidyl dUTP nick-end labeling) to determine if combinations of Lip-C6, gemcitabine or Lip-PDMP, at concentration that were not individually detrimental to cellular viability, could induce apoptosis of PANC-1 cells (). No apoptotic effect was observed with 5 µM Lip-C6 alone, 20 µM gemcitabine alone or Lip-PDMP 5 µM alone. However, significant apoptosis of PANC-1 cells was detected upon treatment with the combination of Lip-C6 and gemcitabine or a combinatorial nanoliposome encapsulating equal concentrations of both C6-ceramide and PDMP (Lip-C6/PDMP). We previously had showed that the Lip-C6/PDMP formulation elicited a more robust therapeutic response in neuroblastoma cells.Citation31 Of note, the combination of gemcitabine with Lip-C6/PDMP induced a dramatic increase in apoptosis of PANC-1 cells beyond that seen with Lip-C6/PDMP alone or the combination of Lip-C6 and gemcitabine ().
The metabolic fate of Lip-C6 is substantially altered by Lip-PDMP.
Short-chain ceramide species are targets of the same metabolic pathways which act upon endogenous ceramides. Intriguingly, these metabolic pathways also convert a substantial amount of short-chain ceramide to natural ceramides through de-acylation to yield sphingosine followed by subsequent re-acylation with a diversity of fatty acids. The most notable metabolism of short-chain ceramides is to corresponding short-chain cerebrosides and short-chain sphingomyelin. These particular pathways act to neutralize the pro-apoptotic lipid and play a primary role in the ability of a cancer cell to overcome the short-chain ceramide. In our study we evaluated the metabolism of nanoliposomal-delivered C6-ceramide (Lip-C6) by PANC-1 cells (). Indeed, Lip-C6 treatment was reflected by a substantial increase in C6-ceramide as well as C6-cerebroside and C6-sphingomyelin (). Not surprisingly, Lip-C6 treatment also resulted in a significant increase in sphingosine, via de-acylation, as well as subsequent increases in both sphingosine-1-phosphate and natural chain length ceramides (). The increase in sphingosine-1-phosphate is not without precedent as this has been observed in other cellular systems with short-chain ceramide analogs where it has explained seemingly similar observations with the use of short-chain ceramide analogs or sphingosine-1-phosphate.Citation32
In our study, we employed either gemcitabine or Lip-PDMP as means to improve the therapeutic efficacy of Lip-C6. As expected with an inhibitor of glucosylceramide synthase, the use of Lip-PDMP in combination with Lip-C6 yielded a near-complete loss in the conversion of C6-ceramide to C6-cerebroside with a concomitant increase in the amount of C6-ceramide in PANC-1 cells ( and B). In contrast, Lip-PDMP in combination with Lip-C6 treatment did not result in any increase in the conversion of C6-ceramide to C6-sphingomyelin (). However, the combinatorial use of Lip-PDMP and Lip-C6 resulted in a substantial, 5-fold (compared with Lip-Ghost), increase in sphingosine and an even more dramatic, 28-fold (compared with Lip-Ghost), increase in sphingosine-1-phosphate ( and F). The robust increase in the pro-survival sphingolipid sphingosine1-phosphate can explain the antagonistic effect noted in cellular viability studies of the combinatorial treatment at higher dosage (). Ultimately, the combination of Lip-PDMP with Lip-C6 also significantly increased the accumulation of natural C14:0 ceramide species beyond Lip-C6 alone (). While Lip-PDMP was specifically designed to influence ceramide metabolism to glucosylceramide, reports have recently emerged showing that gemcitabine can also elicit ceramide accumulation.Citation33–Citation37 In our study, we did not observe any alteration in C6-ceramide, its short-chain derivatives, sphingosine or sphingosine-1-phosphate, in response to treatment with gemcitabine alone or in separate combination with either Lip-C6 or Lip-PDMP (). However, combination of gemcitabine with Lip-C6 did result in an increase in natural ceramide species ( and ). Additionally, when combining gemcitabine with both Lip-C6 and Lip-PDMP, there was a further increase in several lipids beyond that observed with the combination treatment of Lip-PDMP and Lip-C6. This included increases in: C6-ceramide (), sphingosine (), sphingosine-1-phosphate (), and several natural ceramide species ( and ). Treatments with Lip-PDMP alone or gemcitabine alone revealed no notable changes in sphingosine, sphingosine-1-phosphate or natural ceramides (). Treatments with Lip-PDMP in combination with gemcitabine revealed a significant, near 4-fold (compared with Lip-Ghost), increase in sphingosine-1-phosphate (). Taken together, our data reveals that: (1) blocking glucosylceramide synthase can increase sphingosine-1-phosphate production in response to Lip-C6 treatment and (2) combining Lip-C6 with gemcitabine and/or glucosylceramide synthase blockade leads to an increase in C6-ceramide as well as natural ceramides.
Lip-C6, but not gemcitabine, inhibits Akt and Erk signaling pathways.
Activation of Erk and Akt pathways are considered two major mitogenic pathways important to the regulation of cell growth and survival. We have previously shown that Lip-C6 inhibits Akt phosphorylation in breast and melanoma cells.Citation10 In addition, ceramide has also been shown to inhibit the phosphorylation and activation of Erk in HEK293 cells.Citation17 We employed pharmacological inhibitors to further confirm the utility of interfering with Akt or Erk as a mechanism to elicit cytotoxicity toward PANC-1 cells. SH-6 effectively blocked the phosphorylation of Akt () and reduced the viability of PANC-1 cells (). Likewise, by using U0126 to inhibit MEK, a kinase upstream of Erk, the phosphorylation and viability of PANC-1 cells was reduced ( and B). The deleterious effect of SH-6 on PANC-1 viability mirrored that of Lip-C6 yet provided no additional benefit in combination (). However, the combination of U0126 and Lip-C6 led to a significantly further reduction in PANC-1 viability compared with Lip-C6 alone (). These findings confirm the utility of interfering with Akt and Erk as effective therapeutic strategies to treat PANC-1 pancreatic cancer cells. Furthermore, while the potent Akt antagonist Lip-C6 can interfere with Erk, greater therapeutic efficacy in PANC-1 cells can be achieved by combining Lip-C6 with more specific pharmacological inhibitors of the Erk signaling cascade.
To explore the molecular mechanisms underlying the synergistic cytotoxicity observed with treatment of PANC-1 cells with Lip-C6 and gemcitabine, we examined Akt and Erk phosphorylation. We chose to evaluate concentrations of Lip-C6 at which an effective inhibition of Akt or Erk was detected in our previous studies in reference Citation10. Phosphorylation (activation) of Akt was significantly decreased in the presence of Lip-C6 (35 µM) but not gemcitibine (20 µM) (). Likewise, phosphorylation (activation) of Erk was decreased by Lip-C6 (35 µM) but not gemcitibine (20 µM) (). In both cases of Akt activation and Erk activation, a combination of Lip-C6 and gemcitabine failed to elicit any additional inhibitory effect. More so, the combination of gemcitabine even interfered with the inhibitory effect of Lip-C6 toward Erk phosphorylation. These results suggested that Akt plays a more dominant role in Lip-C6-mediated effects in PANC-1 cells. These data also suggested that Lip-C6 and gemcitabine achieve a synergistic tumor suppression effect via distinct but complementary mechanisms. Taken together, the anti-metabolite gemcitabine enhances the efficacy of Lip-C6 but this enhancing effect is independent of the Lip-C6-inhibited Akt pathway.
The in vivo antitumor efficacy of Lip-C6 is enhanced by gemcitabine or Lip-PDMP.
To evaluate the in vivo antitumor activity of Lip-C6, and its combination with either gemcitabine or PDMP, subcutaneous PANC-1 tumors were established in athymic nude mice. A control nanoliposomal formulation with no C6-ceramide (Lip-Ghost), Lip-C6, gemcitabine, or a combination of Lip-C6 and gemcitabine, were routinely administered via tail-vein injection and tumor size was measured to evaluate improvement of the therapeutic efficacy of Lip-C6 by gemcitabine. We observed a modest antitumor effect from gemcitabine-treatment alone or Lip-C6-treatment alone. However, consistent with our in vitro findings, the combination treatment of Lip-C6 and gemcitabine further augmented the inhibition of PANC-1 tumor growth (). We next evaluated improvements to Lip-C6 by inclusion of PDMP within the same nanoliposome. A control nanoliposomal formulation Lip-Ghost, Lip-C6 or Lip-C6/PDMP, were routinely administered via tail-vein injection and tumor size was measured. We observed a modest antitumor effect from Lip-C6-treatment alone and a robust effect with Lip-C6/PDMP (). These results indicated that by increasing the intracellular concentration of endogenous ceramide, and by preventing the neutralization of exogenously delivered short-chain ceramide to glucosylceramide, an effective in vivo anti-pancreatic cancer effect could be achieved.
Discussion
Although gemcitabine is considered to be the most effective drug in treating pancreatic cancer, resistance is often observed due to mechanisms including activation of NFκB, increased PI3 kinase activity, and a high basal level of Akt phosphorylation.Citation38–Citation40 We have previously shown that Lip-C6 can synergize and augment the cytotoxic actions of the Raf/Mek/Erk inhibitor sorafanib in melanoma models.Citation10 Likewise, it has been demonstrated that inhibition of the Akt/PI3 kinase pathway by small molecules can synergize with gemcitabine to induce apoptosis in various human pancreatic cancer cell lines.Citation41–Citation43 Consistent with published literature, our present data demonstrate that the phosphorylation of Akt at serine 473 is not affected by gemcitabine in pancreatic cancer cells. This is not surprising considering that, as a nucleoside analog, gemcitabine's primary mechanism of action is to interfere with DNA synthesis. However, inhibition of Akt phosphorylation at serine 473 by Lip-C6 resulted in a significantly increased sensitivity to gemcitabine-induced cytotoxicity in drug-resistant PANC-1 pancreatic cancer cells. Lip-C6-mediated reduction of Akt phosphorylation alone was not sufficient to induce cytotoxicity.
From another perspective, it is important to consider that the PANC-1 cell line, like many advanced cancer cell lines, can convert C6-ceramide to less toxic and pro-survival metabolites. Studies have further suggested that gemcitabine itself can promote ceramide accumulation. In our study, treatment of PANC-1 cells with the triple combination of Lip-C6, Lip-PDMP, to block glucosylceramide synthase and gemcitabine substantially augmented the accumulation of C6-ceramide and natural ceramide species. These observations confirmed that the pro-apoptotic and anti-pancreatic cancer effect of Lip-C6 is enhanced by the anti-metabolic action of gemcitibine or by preventing ceramide metabolism with gemcitabine and/or Lip-PDMP. More so, the efficacy of Lip-C6 in vivo in a xenograft model of pancreatic cancer was improved with gemcitabine. We successfully employed an in vivo dose of gemcitabine in mice via tail vein injection that is similar to the maximum tolerated dose in humans (50 mg/kg in mice compared with approximately 56 mg/kg in humans). However, we used a dose frequency of three times per week in contrast to the single weekly dose used in humans. While this is a potential downfall, it is important to note that the rate of metabolism of gemcitabine in mice is considerably faster (serum half-life around 0.15 h).Citation44 Furthermore, our in vitro studies also indicated that a gemcitabine dose in combination with Lip-C6 may be synergistically effective even if reduced by 50-fold from the dose we used in vivo.
Over the past several years, sphingolipid metabolites have been recognized for roles in modulating cell proliferation, apoptosis, cell migration and angiogenesis. Clinically, the concentration of the pro-apoptotic sphingolipid metabolite ceramide is significantly reduced in multiple cancers including pancreatic and colon cancer.Citation45–Citation47 Multiple laboratories, including our own, have shown that increasing endogenous ceramide levels via pharmacological or molecular strategies lead to cancer cell cytotoxicity.Citation2,Citation10,Citation11,Citation45–Citation48 However, these strategies are often limited by drug efflux mechanisms and/or ceramide metabolism.Citation21,Citation22 Recently we have shown that the metabolism of exogenously delivered short-chain ceramide is cell type dependent and concentration dependent.Citation23 In PANC-1 cells high concentrations of C6-ceramide were metabolized to glucosylceramide, a related sphingolipid that is closely tied to multidrug resistance.Citation23 This creates a particular problem for the use of C6-ceramide as a therapeutic for pancreatic cancer, however, one that may be overcome by inhibitors of glucosylceramide biosynthesis. We also recently reported the in vitro efficacy of a nanoliposome incorporating both C6-ceramide and the glucosylceramide synthase inhibitor PDMP in the treatment of neuroblastoma.Citation31 In our current study, we employed this same combination-nanoliposome, Lip-C6/PDMP, in the treatment of drug-resistant pancreatic cancer. With PDMP preventing the neutralization of ceramide to glucosylceramide (cerebroside), Lip-C6 was able to exert a robust toxicity in vitro toward PANC-1 cells. Not surprisingly, treatment in vitro with both Lip-C6/PDMP and gemcitabine, which augmented C6-ceramide and natural ceramide even more so, elicited an even greater induction of PANC-1 cell apoptosis. The development of Lip-C6/PDMP was not limited solely to improvement of Lip-C6 therapy, but also to the ability to simultaneously deliver therapeutics in vivo in a non-toxic nanoscale formulation.Citation2,Citation10,Citation11 In vivo, Lip-C6 alone was somewhat effective while the combinationnanoliposome Lip-C6/PDMP near completely blocked PANC-1 tumor growth.
Overall, rationally designed combinatorial therapies have the potential to achieve synergistic treatment of cancer. Our second generation Lip-C6/PDMP formulation offers vast therapeutic improvement with essentially no change to the size, charge and stability of the original Lip-C6 formulation. “Designer” nanoscale ceramidecontaining liposomes can be engineered to co-deliver the nucleoside analog gemcitibine, as well as antagonists of ceramide metabolism such as PDMP. Nanomaterials functionalized with polyethylene glycol (PEG), such as our ceramide-containing nanoliposome formulations, have the ability to passively accumulate within the leaky vasculature of tumors through enhanced permeation and retention.Citation49 Further improvements can possibly be achieved by selective tumor targeting by coupling antibodies, antibody fragments, peptides, peptide fragments or small ligands, to the PEGylations on the nanoparticles.Citation50 Altogether, second-generation nanoliposomes containing combinations of short-chain ceramide analogs, and other therapeutics designed to augment or complement the effects of ceramide, offer a promising solution for the treatment of highly resistant cancers such as pancreatic cancer.
Materials and Methods
Cell culture.
PANC-1 human pancreatic cancer cells were maintained at 37°C and 5% CO2, in Dulbecco's Modified Eagle Medium (DMEM) supplemented with 10% fetal bovine serum (FBS) and 1% penicillin/streptomycin. For subculture, cells were subject to trypsin/EDTA detachment, centrifuged, resuspended in growth media and replated at appropriate cell density.
Liposome preparation.
Nanoliposomes were prepared based upon earlier studies.Citation2,Citation11 Briefly, lipids dissolved in chloroform, were combined in specific molar ratios (), dried to a film under a stream of nitrogen, and then hydrated by addition of 0.9% NaCl. Solutions were sealed, heated at 60°C (60 min), and subjected to vortex mixing and sonicated until light no longer diffracted through the suspension. The lipid vesicle-containing solution was quickly extruded at 60°C by passing the solution ten times through 100 nm polycarbonate filters in an Avanti Mini-Extruder (Avanti Polar Lipids, Alabaster, AL). Nanoliposomal size (), and a neutral charge were validated using a Malvern Zetasizer Nano ZS at 25°C. Nanoliposome solutions were stored at room temperature until use.
Cellular viability assay.
PANC-1 cells were plated at 4 × 103 cells per well in 96-well tissue culture plates and grown in 10% serum fortified media for 24 h prior to treatment. Cells were then treated for 24 h in media containing 2.5% FBS. Following treatment, cellular viability was assessed using a Cell Titer 96 AQueous Non-Radioactive Cell Proliferation Assay according to the manufacturer's instructions (Promega, Madison, WI). Viability was determined by measuring absorbance at 490 nm using a microplate reader and normalizing to the viability observed under control conditions.
TUNEL (terminal deoxynucleotide transferase dUTP nick-end labeling) assay.
PANC-1 cells were plated at 2.5 × 104 cells per well in 8-well chamber slides, and grown in 10% serum fortified media for 24 h prior to treatment. Cells were treated for 24 h in media containing 2.5% FBS. Fragmented DNA of apoptotic cells was stained using an ApopTag Red In Situ Apoptosis Detection Kit according to the manufacturer's instructions (Chemicon, Temecula, CA), and visualized by fluorescence microscopy using appropriate filters. The percent of apoptotic cells was quantified by counting TUNEL-positive cells and by dividing by the total number of cells in five high power fields.
Protein gel blotting.
PANC-1 cells were seeded in 6-well tissue culture plates and grown for 24 h. The cells were treated for 24 h in the DMEM media containing 2.5% FBS. Cells were harvested and lysates were prepared in lysis buffer (0.1% NP40, 50 mM HEPES, 137 mM NaCl, 10 mM Na4P2O7, 50 mM NaF, 5 mM β-glycerolphosphate, 1 mM EGTA, 2 mM EDTA, 1% glycerol, 2 mM) containing protease inhibitor (EMD Biosciences, San Diego, CA) for 20 min on ice followed by centrifugation at 4°C for 15 min to sediment particulate materials. Protein concentrations were measured using Bio-Rad protein assay kit (Bio-Rad Laboratories, Hercules, CA). Proteins (30 µg) from whole cell extracts were separated by electrophoresis on SDS-polyacrylamide gels and transferred onto nitrocellulose membranes. Membranes were blocked with 1% BSA in TBS containing 0.05% Tween and incubated with primary antibodies targeting phospho-Akt (Ser473) and phospho-Erk1/2 (Thr202/Tyr204), as well as total Akt and total Erk (Cell Signaling, Beverly, MA), followed by washing and incubation with horseradish peroxidase-conjugated secondary antibodies (Santa Cruz Biotechnology, Santa Cruz, CA). Protein gel blots were visualized with enhanced chemiluminescence detection (Thermo Scientific, Rockport, IL).
In vivo tumor model.
Bilateral human pancreatic tumor xenografts were established in 6-wk-old female athymic nude mice (Harlan Sprague Dawley, Indianapolis, IN) by subcutaneous injection of PANC-1 cells over the rib cage. For each tumor, 1 × 107 cells were resuspended in 200 µl of cell culture media. Tumors were allowed to establish for one week prior to commencement of treatment regimes. Treatments occurred three times per week via tail vein injection. Each treatment group consisted of at least four animals. Tumor volumes were quantified by measuring with calipers and multiplying tumor length, width and height. In the “gemcitabine” experiment the treatment groups were: Lip-C6 (9 mg/kg C6-ceramide), gemcitabine (50 mg/kg), a combination of Lip-C6 and gemcitabine and Lip-Ghost (equivalent total lipid mass). In the “PDMP” experiment the treatment groups were: Lip-C6 (18 mg/kg C6-ceramide), Lip-C6/PDMP liposome (18 mg/kg C6-ceramide and 23 mg/kg PDMP) and Lip-Ghost (equivalent total lipid mass). All animal procedures were approved by, and performed according to the standards and guidelines of the Pennsylvania State University College of Medicine Institutional Animal Care and Use Committee.
Statistical analysis.
One-way, or two-way, analysis of variance (ANOVA), were used to determine statistically significant differences between treatments (p < 0.05). At least three independent experiments were performed for each condition. Post hoc comparisons of specific treatments were performed using a Bonferroni test. All error bars represent standard error from the mean (SEM). All statistical analyses were performed using GraphPad Prism 4 software (La Jolla, CA).
Disclosure of Potential Conflicts of Interest
The Penn State Research Foundation has licensed ceramide nanoliposomes, and other nonliposomal nanotechnology, to Keystone Nano, Inc., (State College, PA). M.K. is the Chief Medical Officer of Keystone Nano, Inc.
Abbreviations
PDMP | = | D-threo-1-phenyl-2-decanoylamino-3-morpholino-1-propanol |
Lip-Ghost | = | ghost nanoliposome |
Lip-C6 | = | C6-ceramide nanoliposome |
Lip-PDMP | = | PDMP nanoliposome |
Lip-C6/PDMP | = | C6-ceramide and PDMP nanolipososme |
PKC | = | protein kinase C |
TUNEL | = | terminal deoxynucleotidyl dUTP nick-end labeling |
PEG | = | polyethylene glycol |
Figures and Tables
Figure 1 Cytotoxicity was induced by Lip-C6, Lip-PDMP and gemcitabine in highly drug-resistant PANC-1 pancreatic cancer cells. Cellular viability of PANC-1 cells was determined at 24, 48 and 72 h in a dose response utilizing: (A) Lip-C6, (B) Lip-PDMP and (C) gemcitabine (Gem). (D) IC50 values for individual treatments at 48 h were calculated. All data points are representative of n = 8 experimental conditions.
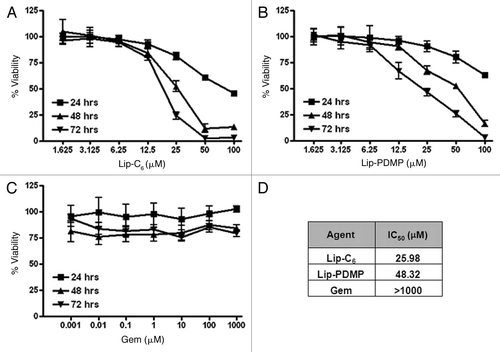
Figure 2 Lip-C6, Lip-PDMP and gemcitabine cooperatively induce apoptosis of PANC-1 cells. Apoptosis of PANC-1 cells was detected by TUNEL assay following 24 h treatments with: (A) saline control, (B) Lip-C6 (5 µM C6-ceramide), (C) 20 µM gemcitabine (Gem), (D) Lip-C6 (5 µM C6-ceramide) + 20 µM Gem, (E) Lip-Ghost (empty nanoliposome), (F) Lip-PDMP (5 µM PDMP), (G) Lip-C6/PDMP (5 µM C6-ceramide and 5 µM PDMP), and (H) Lip-C6/PDMP (5 µM C6-ceramide and 5 µM PDMP) + 20 µM Gem. (I) Apoptotic cells were quantified as a percent of the total cell number. One-way ANOVA: *p < 0.001 compared with control, Lip-Ghost, Lip-C6, Gem and Lip-PDMP, #p < 0.05 compared with Lip-C6 + Gem and Lip-C6/Lip-PDMP, n = 5.
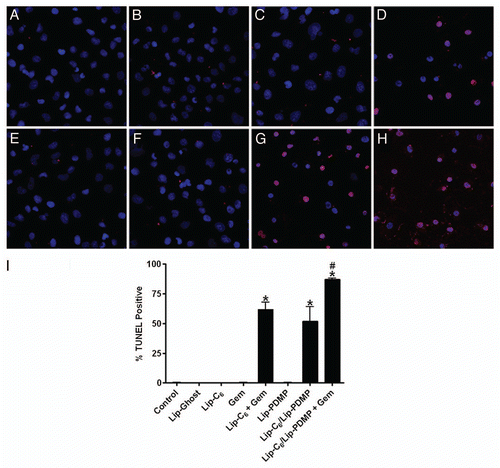
Figure 3 The metabolic fate of Lip-C6 is altered by Lip-PDMP alone or in combination with gemcitabine. PANC-1 cells were treated for 24 h with 12.5 µM Lip-C6, 24 µM Lip-PDMP, 40 µM gemcitabine (Gem) or various combinations. Cells were harvested and lipids were extracted and analyzed using LC-MS/MS/MS. Abundance relative to total cellular protein was determined for: (A) C6-ceramide, (B) C6-cerebroside, (C) C6-sphingosmyelin, (D) total natural (endogenous) ceramide, (E) sphingosine and (F) sphingosine-1-phosphate. One-way ANOVA: *p < 0.05 compared with control and Lip-Ghost, #p < 0.05 compared with Lip-Ghost only, $p < 0.05 compared with Lip-C6 (Lip-C6-containing combinations only), %p < 0.05 comparing triple combination with Lip-C6 + Gem only, &p < 0.05 comparing triple combination with Lip-C6 + Lip-PDMP only, @p <0.05 comparing triple combination with Lip-C6 + Gem and Lip-C6 + Lip-PDMP, n = 4.
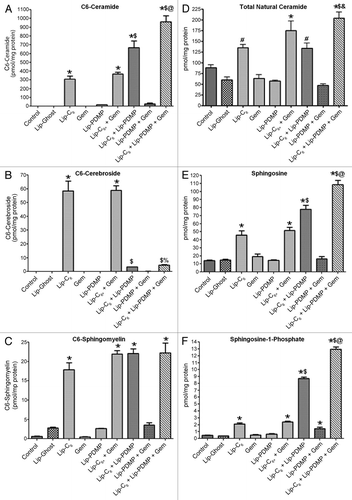
Figure 4 Pharmacological inhibition of Akt or Erk in PANC-1 cells replicated the effect of Lip-C6 on these signaling pathways. PANC-1 cells were exposed to the Akt inhibitor SH-6 or the MEK inhibitor U0126 (a kinase upstream of Erk). (A) Phosphorylation of Akt was blocked by 48 h treatment with SH-6 (9.5 µM), and phosphorylation of Erk was blocked by 48 h treatment with U0126 (17.5 µM). (B) Cellular viability was determined at 48 h in a dose response utilizing SH-6. (C) Cellular viability was determined at 48 h in a dose response utilizing U0126. (D) The effects of SH-6 (4.25 µM) on cellular viability were compared with Lip-C6 (25 µM) treatment or were evaluated in combination. (E) The effects of U0126 (17.5 µM) on cellular viability were compared with Lip-C6 (25 µM) treatment or were evaluated in combination. One-way ANOVA: *p < 0.001 compared with Lip-Ghost + DMSO or Lip-Ghost + SH-6, **p < 0.001 compared with Lip-C6 + DMSO, n = 8.
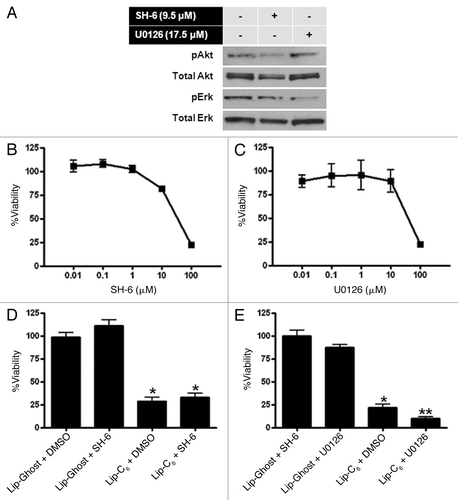
Figure 5 Lip-C6, but not gemcitibine, inhibits Akt and Erk signaling pathways in PANC-1 cells. PANC-1 cells were maintained in media containing 2.5% FBS to reduce the background level of phosphorylation. Cells were treated with control (media only), Lip-Ghost (total lipid weight-matched), Lip-C6 (35 µM C6-ceramide), 20 µM gemcitabine (Gem), or a combination of Lip-C6 and Gem, for 24 h. Cells were harvested, lysed and total proteins were subjected to protein gel blotting. (A) Phosphorylated-Erk (pErk) and (B) phosphorylated-Akt (pAkt), were detected using monoclonal antibodies against pErk and pAkt, respectively. Total Erk and total Akt, protein levels were quantified using anti-Erk and anti-Akt, antibodies, respectively one-way ANOVA: #p < 0.01 compared with control or Gem, *p < 0.05 compared with control, Lip-Ghost or Gem, n = 3).
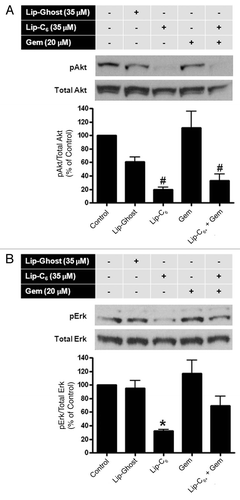
Figure 6 The in vivo antitumor efficacy of Lip-C6 is augmented by gemcitabine or Lip-PDMP. Bilateral subcutaneous PANC-1 tumors were established on the flanks of athymic nude mice. (A) Lip-Ghost (equivalent total lipid dosage), Lip-C6 (9 mg/kg C6-ceramide), 50 mg/kg gemcitabine (Gem) or a combination of Lip-C6 and Gem, were routinely administered via tail vein injection. Two-way ANOVA: *p < 0.05, Lip-Ghost compared with all other treatments (large box over days 36 to 54), #p < 0.05, Lip-Ghost compared with Lip-C6 + Gem (small boxes over day 54), n ≥ 4. (B) Lip-Ghost (equivalent total lipid dosage), Lip-C6 (18 mg/kg C6-ceramide) or Lip-C6/PDMP (18 mg/kg C6-ceramide and 23 mg/kg PDMP), were routinely administered via tail vein injection. Two-way ANOVA: **p < 0.05, Lip-Ghost compared with Lip-C6/PDMP (small boxes over days 60–63), n ≥ 4.
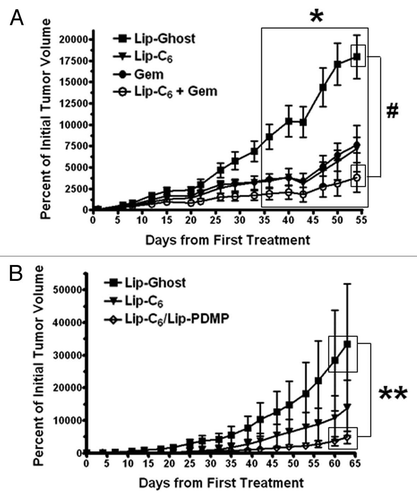
Table 1 Nanoliposome formulations
Table 2 Synergy of combinatorial therapies
Table 3 The conversion of Lip-C6-delivered C6-ceramide to natural ceramide species is altered by Lip-PDMP alone or in combination with gemcitabine
Acknowledgments
This work was supported by National Institutes of Health Grant R01HL076789 (M.K.) and State of Pennsylvania Tobacco Settlement Funds (M.K.).
References
- Jemal A, Siegel R, Ward E, Murray T, Xu J, Thun MJ. Cancer statistics 2007. CA Cancer J Clin 2007; 57:43 - 66; PMID: 17237035; http://dx.doi.org/10.3322/canjclin.57.1.43
- Stover TC, Sharma A, Robertson GP, Kester M. Systemic delivery of liposomal short-chain ceramide limits solid tumor growth in murine models of breast adenocarcinoma. Clin Cancer Res 2005; 11:3465 - 3474; PMID: 15867249; http://dx.doi.org/10.1158/1078-0432.CCR-04-1770
- Jabr-Milane L, van Vlerken L, Devalapally H, Shenoy D, Komareddy S, Bhavsar M, et al. Multi-functional nanocarriers for targeted delivery of drugs and genes. J Control Release 2008; 130:121 - 128; PMID: 18538887; http://dx.doi.org/10.1016/j.jconrel.2008.04.016
- Cho K, Wang X, Nie S, Chen ZG, Shin DM. Therapeutic nanoparticles for drug delivery in cancer. Clin Cancer Res 2008; 14:1310 - 1316; PMID: 18316549; http://dx.doi.org/10.1158/1078-0432.CCR-07-1441
- Segui B, Andrieu-Abadie N, Jaffrezou JP, Benoist H, Levade T. Sphingolipids as modulators of cancer cell death: Potential therapeutic targets. Biochim Biophys Acta 2006; 1758:2104 - 2120
- Taha TA, Hannun YA, Obeid LM. Sphingosine kinase: Biochemical and cellular regulation and role in disease. J Biochem Mol Biol 2006; 39:113 - 131; PMID: 16584625; http://dx.doi.org/10.5483/BMBRep.2006.39.2.113
- Liu YY, Yu JY, Yin D, Patwardhan GA, Gupta V, Hirabayashi Y, et al. A role for ceramide in driving cancer cell resistance to doxorubicin. FASEB J 2008; 22:2541 - 2551; PMID: 18245173; http://dx.doi.org/10.1096/fj.07-092981
- Devalapally H, Duan Z, Seiden MV, Amiji MM. Paclitaxel and ceramide co-administration in biodegradable polymeric nanoparticulate delivery system to overcome drug resistance in ovarian cancer. Int J Cancer 2007; 121:1830 - 1838; PMID: 17557285; http://dx.doi.org/10.1002/ijc.22886
- Alphonse G, Bionda C, Aloy MT, Ardail D, Rousson R, Rodriguez-Lafrasse C. Overcoming resistance to gamma-rays in squamous carcinoma cells by poly-drug elevation of ceramide levels. Oncogene 2004; 23:2703 - 2715; PMID: 15048093; http://dx.doi.org/10.1038/sj.onc.1207357
- Tran MA, Smith CD, Kester M, Robertson GP. Combining nanoliposomal ceramide with sorafenib synergistically inhibits melanoma and breast cancer cell survival to decrease tumor development. Clin Cancer Res 2008; 14:3571 - 3581; PMID: 18519791; http://dx.doi.org/10.1158/1078-0432.CCR-07-4881
- Stover T, Kester M. Liposomal delivery enhances short-chain ceramide-induced apoptosis of breast cancer cells. J Pharmacol Exp Ther 2003; 307:468 - 475; PMID: 12975495; http://dx.doi.org/10.1124/jpet.103.054056
- Regala RP, Thompson EA, Fields AP. Atypical protein kinase C iota expression and aurothiomalate sensitivity in human lung cancer cells. Cancer Res 2008; 68:5888 - 5895; PMID: 18632643; http://dx.doi.org/10.1158/0008-5472.CAN-08-0438
- El-Rayes BF, Ali S, Philip PA, Sarkar FH. Protein kinase C: A target for therapy in pancreatic cancer. Pancreas 2008; 36:346 - 352; PMID: 18437080; http://dx.doi.org/10.1097/MPA.0b013e31815ceaf7
- Friday BB, Adjei AA. Advances in targeting the Ras/Raf/MEK/Erk mitogen-activated protein kinase cascade with MEK inhibitors for cancer therapy. Clin Cancer Res 2008; 14:342 - 346; PMID: 18223206; http://dx.doi.org/10.1158/1078-0432.CCR-07-4790
- Fox TE, Houck KL, O'Neill SM, Nagarajan M, Stover TC, Pomianowski PT, et al. Ceramide recruits and activates protein kinase Czeta (PKCzeta) within structured membrane microdomains. J Biol Chem 2007; 282:12450 - 12457; PMID: 17308302; http://dx.doi.org/10.1074/jbc.M700082200
- Powell DJ, Hajduch E, Kular G, Hundal HS. Ceramide disables 3-phosphoinositide binding to the pleckstrin homology domain of protein kinase B (PKB)/Akt by a PKCzeta-dependent mechanism. Mol Cell Biol 2003; 23:7794 - 7808; PMID: 14560023; http://dx.doi.org/10.1128/MCB.23.21.7794-808.2003
- Bourbon NA, Yun J, Berkey D, Wang Y, Kester M. Inhibitory actions of ceramide upon PKC-epsilon/ERK interactions. Am J Physiol Cell Physiol 2001; 280:1403 - 1411; PMID: 11350735
- Tagaram HR, Divittore NA, Barth BM, Kaiser JM, Avella D, Kimchi ET, et al. Nanoliposomal ceramide prevents in vivo growth of hepatocellular carcinoma. Gut 2011; 60:695 - 701; PMID: 21193455; http://dx.doi.org/10.1136/gut.2010.216671
- Liu X, Ryland L, Yang J, Liao A, Aliaga C, Watts R, et al. Targeting of survivin by nanoliposomal ceramide induces complete remission in a rat model of NK-LGL leukemia. Blood 2010; 116:4192 - 4201; PMID: 20671121; http://dx.doi.org/10.1182/blood-2010-02271080
- Nussbaumer P, Hornillos V, Ghobrial M, Ullrich T. An efficient, one-pot synthesis of various ceramide-1-phosphates from sphingosine-1-phosphate. Chem Phys Lipids 2008; 151:125 - 128; PMID: 18039471; http://dx.doi.org/10.1016/j.chemphyslip.2007.10.005
- Pallis M, Russell N. P-glycoprotein plays a drug-effluxindependent role in augmenting cell survival in acute myeloblastic leukemia and is associated with modulation of a sphingomyelin-ceramide apoptotic pathway. Blood 2000; 95:2897 - 2904; PMID: 10779437
- Raggers RJ, van Helvoort A, Evers R, van Meer G. The human multidrug resistance protein MRP1 translocates sphingolipid analogs across the plasma membrane. J Cell Sci 1999; 112:415 - 422; PMID: 9885294
- Chapman JV, Gouaze-Andersson V, Messner MC, Flowers M, Karimi R, Kester M, et al. Metabolism of short-chain ceramide by human cancer cells-implications for therapeutic approaches. Biochem Pharmacol 2010; 80:308 - 315; PMID: 20385104; http://dx.doi.org/10.1016/j.bcp.2010.04.001
- Réjiba S, Wack S, Aprahamian M, Hajri A. K-ras oncogene silencing strategy reduces tumor growth and enhances gemcitabine chemotherapy efficacy for pancreatic cancer treatment. Cancer Sci 2007; 98:1128 - 1136; PMID: 17489984; http://dx.doi.org/10.1111/j.1349-7006.2007.00506.x
- Christgen M, Schniewind B, Jueschke A, Ungefroren H, Kalthoff H. Gemcitabine-mediated apoptosis is associated with increased CD95 surface expression but is not inhibited by DN-FADD in Colo357 pancreatic cancer cells. Cancer Lett 2005; 227:193 - 200; PMID: 16112422; http://dx.doi.org/10.1016/j.canlet.2005.01.016
- Hering J, Garrean S, Dekoj TR, Razzak A, Saied A, Trevino J, et al. Inhibition of proliferation by omega-3 fatty acids in chemoresistant pancreatic cancer cells. Ann Surg Oncol 2007; 14:3620 - 3628; PMID: 17896154; http://dx.doi.org/10.1245/s10434-007-9556-8
- Jiang PH, Motoo Y, Sawabu N, Minamoto T. Effect of gemcitabine on the expression of apoptosis-related genes in human pancreatic cancer cells. World J Gastroenterol 2006; 12:1597 - 1602; PMID: 16570353
- Lau JP, Weatherdon KL, Skalski V, Hedley DW. Effects of gemcitabine on APE/ref-1 endonuclease activity in pancreatic cancer cells, and the therapeutic potential of antisense oligonucleotides. Br J Cancer 2004; 91:1166 - 1173; PMID: 15316562
- Duxbury MS, Ito H, Zinner MJ, Ashley SW, Whang EE. siRNA directed against c-src enhances pancreatic adenocarcinoma cell gemcitabine chemosensitivity. J Am Coll Surg 2004; 198:953 - 959; PMID: 15194078; http://dx.doi.org/10.1016/j.jamcollsurg.2004.01.037
- Ishida M, Sunamura M, Furukawa T, Akada M, Fujimura H, Shibuya E, et al. Elucidation of the relationship of BNIP3 expression to gemcitabine chemosensitivity and prognosis. World J Gastroenterol 2007; 13:4593 - 4597; PMID: 17729412
- Barth BM, Gustafson SJ, Young MM, Fox TE, Shanmugavelandy SS, Kaiser JM, et al. Inhibition of NADPH oxidase by glucosylceramide confers chemoresistance. Cancer Biol Ther 2010; 10:1126 - 1136; PMID: 20935456; http://dx.doi.org/10.4161/cbt.10.11.13438
- Holland WL, Miller RA, Wang ZV, Sun K, Barth BM, Bui HH, et al. Receptor-mediated activation of ceramidase activity initiates the pleiotropic actions of adiponectin. Nat Med 2011; 17:55 - 63; PMID: 21186369; http://dx.doi.org/10.1038/nm.2277
- Saddoughi SA, Song P, Ogretmen B. Roles of bioactive sphingolipids in cancer biology and therapeutics. Subcell Biochem 2008; 49:413 - 440; PMID: 18751921; http://dx.doi.org/10.1007/978-1-4020-8831-5_16
- Dumitru CA, Sandalcioglu IE, Wagner M, Weller M, Gulbins E. Lysosomal ceramide mediates gemcitabine-induced death of glioma cells. J Mol Med 2009; 87:1123 - 1132; PMID: 19763526; http://dx.doi.org/10.1007/s00109-009-0514-8
- Dumitru CA, Weller M, Gulbins E. Ceramide metabolism determines glioma cell resistance to chemotherapy. J Cell Physiol 2009; 221:688 - 695; PMID: 19711353; http://dx.doi.org/10.1002/jcp.21907
- Modrak DE, Leon E, Goldenberg DM, Gold DV. Ceramide regulates gemcitabine-induced senescence and apoptosis in human pancreatic cancer cell lines. Mol Cancer Res 2009; 7:890 - 896; PMID: 19531570; http://dx.doi.org/10.1158/1541-7786.MCR-08-0457
- Wu BX, Zeidan YH, Hannun YA. Downregulation of neutral ceramidase by gemcitabine: Implications for cell cycle regulation. Biochim Biophys Acta 2009; 1791:730 - 739; PMID: 19345744
- Arlt A, Gehrz A, Muerkoster S, Vorndamm J, Kruse ML, Folsch UR, et al. Role of NFkappaB and Akt/PI3K in the resistance of pancreatic carcinoma cell lines against gemcitabine-induced cell death. Oncogene 2003; 22:3243 - 3251; PMID: 12761494; http://dx.doi.org/10.1038/sj.onc.1206390
- Liptay S, Weber CK, Ludwig L, Wagner M, Adler G, Schmid RM. Mitogenic and antiapoptotic role of constitutive NFkappaB/Rel activity in pancreatic cancer. Int J Cancer 2003; 105:735 - 746; PMID: 12767057; http://dx.doi.org/10.1002/ijc.11081
- Mortenson MM, Galante JG, Gilad O, Schlieman MG, Virudachalam S, Kung HJ, et al. BCL-2 functions as an activator of the AKT signaling pathway in pancreatic cancer. J Cell Biochem 2007; 102:1171 - 1179; PMID: 17960583; http://dx.doi.org/10.1002/jcb.21343
- Bondar VM, Sweeney-Gotsch B, Andreeff M, Mills GB, McConkey DJ. Inhibition of the phosphatidylinositol-3′-kinase-AKT pathway induces apoptosis in pancreatic carcinoma cells in vitro and in vivo. Mol Cancer Ther 2002; 1:989 - 997; PMID: 12481421
- Ito D, Fujimoto K, Mori T, Kami K, Koizumi M, Toyoda E, et al. In vivo antitumor effect of the mTOR inhibitor CCI-779 and gemcitabine in xenograft models of human pancreatic cancer. Int J Cancer 2006; 118:2337 - 2343; PMID: 16331623; http://dx.doi.org/10.1002/ijc.21532
- Takeda A, Osaki M, Adachi K, Honjo S, Ito H. Role of the phosphatidylinositol-3′-kinase-akt signal pathway in the proliferation of human pancreatic ductal carcinoma cell lines. Pancreas 2004; 28:353 - 358; PMID: 15084985; http://dx.doi.org/10.1097/00006676200404000-00026
- Moog R, Burger AM, Brandl M, Schuler J, Schubert R, Unger C, et al. Change in pharmacokinetic and pharmacodynamic behavior of gemcitabine in human tumor xenografts upon entrapment in vesicular phospholipid gels. Cancer Chemother Pharmacol 2002; 49:356 - 366; PMID: 11976829; http://dx.doi.org/10.1007/s00280002-0428-4
- Verheij M, van Blitterswijk WJ, Bartelink H. Radiation-induced apoptosis—the ceramide-SAPK signaling pathway and clinical aspects. Acta Oncol 1998; 37:575 - 581; PMID: 9860316; http://dx.doi.org/10.1080/028418698430287
- Chmura SJ, Mauceri HJ, Advani S, Heimann R, Beckett MA, Nodzenski E, et al. Decreasing the apoptotic threshold of tumor cells through protein kinase C inhibition and sphingomyelinase activation increases tumor killing by ionizing radiation. Cancer Res 1997; 57:4340 - 4347; PMID: 9331096
- Chmura SJ, Nodzenski E, Beckett MA, Kufe DW, Quintans J, Weichselbaum RR. Loss of ceramide production confers resistance to radiation-induced apoptosis. Cancer Res 1997; 57:1270 - 1275; PMID: 9102212
- Selzner M, Bielawska A, Morse MA, Rudiger HA, Sindram D, Hannun YA, et al. Induction of apoptotic cell death and prevention of tumor growth by ceramide analogues in metastatic human colon cancer. Cancer Res 2001; 61:1233 - 1240; PMID: 11221856
- Altinoglu EI, Russin TJ, Kaiser JM, Barth BM, Eklund PC, Kester M, et al. Near-infrared emitting fluorophore-doped calcium phosphate nanoparticles for in vivo imaging of human breast cancer. ACS Nano 2008; 2:2075 - 2084; PMID: 19206454; http://dx.doi.org/10.1021/nn800448r
- Barth BM, Sharma R, Altinoglu EI, Morgan TT, Shanmugavelandy SS, Kaiser JM, et al. Bioconjugation of calcium phosphosilicate composite nanoparticles for selective targeting of human breast and pancreatic cancers in vivo. ACS Nano 2010; 4:1279 - 1287; PMID: 20180585; http://dx.doi.org/10.1021/nn901297q