Abstract
Background: 5-fluorouracil (5FU)-based chemotherapy is the standard treatment for advanced stage colorectal cancer (CRC) patients. Several groups including ours have reported that stage II-III colorectal cancer patients whose tumors retain DNA Mismatch repair (MMR) function derive a benefit from 5FU, but patients with tumors that lost MMR function do not. Although, MMR recognition of 5FU incorporated in DNA has been demonstrated biochemically, it has not been demonstrated within cells to execute 5FU cytotoxicity.
Aim: To establish an efficient construction model for 5FU within DNA and demonstrate that 5FU incorporated into DNA can trigger cellular cytotoxicity executed by the DNA MMR system.
Methods: We constructed a 5FdU-containing heteroduplex plasmid (5FdU plasmid) and 5FdU-containing linear dsDNA (5FdU linear DNA), and transfected these into MMR-proficient, hMLH1-/- and hMSH6-/- cells. We observed cell growth characteristics of both transfectants for 5FU-induced cytotoxicity.
Results: MMR- proficient cells transfected with the 5FdU plasmid but not the 5FdU linear DNA showed reduced cell proliferation by MTS and clonogenic assays, and demonstrated cell morphological change consistent with apoptosis. In MMR-deficient cells, neither the 5FdU plasmid nor 5FdU linear DNA induced cell growth or morphological changes different from controls.
Conclusion: 5FdU as heteroduplex DNA in plasmid but not linear form triggered cytotoxicity in a MMR-dependent manner. Thus 5FU incorporated into DNA, separated from its effects on RNA, can be recognized by DNA MMR to trigger cell death.
Introduction
The fluoropyrimidine 5-fluorouracil (5-FU) is the cornerstone of chemotherapy and the standard of care for patients with advance staged colorectal cancer (CRC). In particular, 5-FU-based chemotherapy improves survival in patients with stage III colon cancer,Citation1–Citation3 and in patients with stage II and III rectal cancer.Citation4 However, individual patient tumor response rates are still overall poor (20–30%),Citation5 and there is not an efficient method to determine which patient will have the best tumor response to 5-FU.
5-FU is incorporated into the RNA of a cell, but also inhibits thymidylate synthetase (TS), depleting thymidine triphosphates (TTPs) available for DNA synthesis and enhancing the incorporation of UTP and FdUTP into a cell's DNA as substitutes for TTP.Citation6 Biochemically, we and others have demonstrated that DNA MMR, particularly its hMutSα complex (hMSH2–hMSH6) can bind 5-FU within DNA with high affinity.Citation7–Citation9 The inference from this recognition of incorporated 5-FU by DNA MMR extends to retrospective and prospective clinical studies in which patients whose colorectal tumors are MMR-deficient lack a survival benefit after 5-FU treatment, whereas patients whose tumors are MMR-proficient show an improvement in survival.Citation10–Citation13 Because 5-FU treatment of cells yields effects on RNA, TS, as well as DNA, it has been difficult to show a direct effect within a cell by DNA MMR upon DNA incorporated with 5-FU. Cells treated with 5-FU demonstrate evidence of cell damage, cell cycle checkpoint responses, and epigenetic changes that include reduction of histone H3 levels in a MMR-dependent manner.Citation8,Citation14,Citation15 These findings suggest that recognition of 5-FU incorporated into DNA by the MMR system is important for 5-FU-induced cytotoxicity. A direct effect has not been previously demonstrated due to lack of a method that can isolate some of 5-FU's effect on a cell.
The aim of this study is to assess MMR-dependent cytotoxicity induced by 5-FU that is incorporated into DNA. Here, we constructed plasmids and linear DNA containing a single molecule of 5FdU in order to isolate the DNA MMR effects for 5-FU. We herein show 5-FU plasmid transfection as an efficient model for measurement of a pure MMR effect that executes 5-FU cytotoxicity.
Results
Efficient construction of 5FdU containing heteroduplex dsDNA plasmid.
The procedure for our preparation of the heteroduplex plasmids is depicted in , after we tried several different approaches. For isolation of ssDNA plasmid, we compared the purity of ssDNA plasmid generated from bacteria/phagemid/helper phage complex with one by the conventional M13KO7 infection method.Citation16 The amount of contaminated chromosomal phage DNA was less by the bacteria/phagemid/helper phage complex method than by the conventional method (, lane 2 and 3), which also reduced the amount of contamination after oligomer extension reaction (, lane 4 and 5). To confirm the DNA sequence of the heteroduplex site, purified heteroduplex plasmid was utilized as the PCR template, and the sequence of the PCR product from the 5FdU containing plasmid (5FdU plasmid) clearly showed the heteroduplex was present, similar to the positive control T:G heteroduplex plasmid ().
The DNA MMR system executes 5-FU cytotoxicity when 5-FU is present in DNA.
To examine the effect of 5-FU incorporated into DNA within cells, colorectal cancer cells in which the MMR status was known were transfected with the 5FdU heteroduplex plasmid. Twelve hours after transfection, we seeded a density of 1.5 × 105 cells per well onto 6-well plates containing culture medium, and began observing their morphology by microscopy (). By 24 h after seeding, only the MMR-proficient cells containing the 5FdU plasmid developed morphological changes consistent with cell death, while no morphological changes were observed for hMLH1-/- and hMLH6-/- cells. Neither MMR-proficient cells nor MMR-deficient cells transfected with negative and heteroduplex control plasmids showed any cell morphological changes. Utilizing an MTS assay, MMR-proficient cells containing the 5FdU plasmid demonstrated reduced cell proliferation compared with both the negative control plasmid (p < 0.01) and the heteroduplex control plasmid (p < 0.01). There was no change in cell proliferation rate for the MMR-deficient cells transfected with the 5-FU plasmid (). Neither MMR-proficient cells nor MMR-deficient cells showed any growth change after T:G heteroduplex positive control plasmid transfection. To confirm the results of MTS assay, we also performed clonogenic assays, further demonstrating that the 5FdU plasmid reduced colony formation in MMR-proficient cells compared with controls, but not in MMR-deficient cells. In addition, the T:G heteroduplex control plasmid did not reduce the number of colonies, indicating that 5FdU-containing heteroduplex plasmid triggers cytotoxicity in a MMR-dependent manner.
DNA MMR-triggered 5FdU cytotoxicity is observed with the plasmid but not the linear dsDNA construct.
To constitute a useful model for detecting the DNA MMR effect when 5-FU is incorporated into DNA, we also constructed 5FdU-containing heteroduplex linear dsDNA (5FdU linear DNA) by annealing a 61 mer 5FdU-containing oligonucleotide with a complementary sequence to the 61 mer oligonucleotide, and compared the cytotoxic effect with 5FdU plasmid. Cells were transfected with 5 µg of 5FdU linear DNA, which includes 49 times as many 5FdU molecules as in the 5 µg of transfected 5FdU plasmid. Interestingly, 5FdU linear DNA did not demonstrate reduced cell density as the 5FdU plasmid did in MMR-proficient cells (). Clonogenic assays showed that the number of colonies in MMR-proficient cells transfected with the 5FdU-heteroduplex plasmid were significantly lower than that of 5FdU linear dsDNA (p < 0.05, ). These observations demonstrate that 5FdU plasmid, not 5FdU linear dsDNA, constitutes an appropriate model for measurement of the DNA MMR induced cytotoxicity upon recognizing 5-FU within DNA in colorectal cancer cells.
Discussion
Clinical evidence suggests that patients with advance-staged colorectal cancer and whose tumors retain DNA MMR function derive a survival benefit with 5-FU-based chemotherapy, compared with patients with tumors that lost MMR function.Citation10–Citation13 There is biochemical demonstration that the MutSα complex (a heterodimer of the DNA MMR proteins MSH2 and MSH6) recognizes and bind 5-FU within DNACitation7,Citation9 and biological evidence that fluoropyrimidines are more sensitive in CRC cells that have intact MMR function than cells with loss of MMR function.Citation8,Citation14 Although it is surmised that MMR recognition and binding of 5-FU induces a DNA damage response,Citation17,Citation18 there was no direct evidence that 5-FU incorporated into DNA actually induced cytotoxicity in a MMR-dependent manner. This is largely because no model existed which isolated 5-FU's effects from within DNA from other effects on RNA and TS. The results of our study show (1) that we could establish efficient construction of a 5FdU plasmid for transfection after modification of techniques in prior reports in reference Citation19 and Citation20, (2) that the 5FdU plasmid triggers cytotoxicity only with MMR proficiency and (3) that the utilization of a 5FdU plasmid was more useful to measure MMR-dependent 5FdU cytotoxicity than 5FdU linear dsDNA.
Description of methods to measure DNA MMR repair activity have been previously reported in which cell nuclear extracts are incubated with mismatched DNA substrates, such as M13mp2 phage DNA,Citation21,Citation22 or by transfecting heteroduplex plasmids into cultured cells.Citation23,Citation24 However, these methods were utilized exclusively for measurement of DNA repair activity at the DNA mismatched or damaged base siteCitation20,Citation25 with no demonstration regarding a DNA damage response once the transfected DNA has been introduced into cells. In particular, since we and others have observed a clear MMR-dependent effect with (a) biochemical binding of DNA containing 5-FU,Citation7–Citation9 (b) cellular toxicity with 5-FU treatment of cells,Citation6,Citation8,Citation14 and (c) improved survival of patients with MMR-intact tumors,Citation10–Citation13 it became important to demonstrate a DNA MMR dependent effect for 5-FU cytotoxicity. We therefore constructed a 5-FU-containing heteroduplex plasmid to measure DNA MMR-dependent cytotoxicity in cells in which the DNA containing 5-FU was the sole lesion. Because MMR is directed to the newly synthesized strandCitation26 and a nick can act as the strand-discrimination signal in vivo,Citation22 nicked heteroduplex plasmids are typically used in biochemical measurement of MMR activity assays. However with live cells, Lei et al. reported that nicked and closed plasmid DNAs were repaired with equal efficiency when cells were transfected with heteroduplex plasmids,Citation24 which was confirmed by others.Citation23 Thus, with this in mind, our data confirms that a closed plasmid is also useful in live cells for examination of the MMR-associated DNA damage response induced by 5-FU in DNA, as well as MMR repair activity. We confirmed the 5FdU inserted site by conventional PCR reaction using non proof reading Taq polymerase under high pH (pH 8.7), which is the usual pH condition when conventional PCR is performed. Under physiological pH conditions in which the pH is closer to 7.4, 5-FU becomes ionized and tends to pair with guanine on the cDNA strand, and tends to pair with an opposing adenine at higher pH conditions when 5-FU is in its keto form.Citation27 Our sequence data showing that the 5FdU site is paired with an opposing adenine is consistent with previous data in reference Citation28 and Citation29.
There are two hypotheses for DNA MMR triggered cell death by 5-FU: a futile cycle of misrepair that is dependent on inhibition of TS and continued incorporation of 5-FU into DNA, and a direct recognition and damage response to 5-FU once it is incorporated into DNA. 5-FU is known to inhibit TS, which catalyzes the conversion of dUMP to dTMP and inhibition of TS results in decreased intracellular dTTP pools.Citation30 The dNTP pool imbalances lead to misincorporation of dUTP or/and 5FdUTP into DNA.Citation6,Citation8 The UTP/5FdUTP “mismatched” site is detected by hMutSα which subsequently recruits MutL homologs, triggering excision of UTP/5FdUTP. However, the UTP/5FdUTP site cannot be repaired appropriately due to depletion of TTPs and UTP/5FdUTP is resynthesized in the newly synthesized strand,Citation9 setting up a futile cycle for repair.Citation17,Citation18 If 5FdUTP is on the template strand, there is no “correct” nucleotide that pairs with it; however, adenine will likely be synthesized opposite 5FdUTP.Citation18,Citation28,Citation29 Prior reports indicate that murine mutant Msh2 (G674A) or Msh6 (T1217D) results in absence of MMR activity, but retains normal damage-induced apoptotic function, supporting the proposed model that direct interaction of DNA damage-bound MMR proteins triggers cell death signaling.Citation31,Citation32 Because the 5FdU plasmid cannot be replicated in mammalian cells due to lack of cellular 5FdUTP dNTPs, our results for our transfected 5FdU plasmid containing a single 5-FU molecule triggering cell death with intact DNA MMR support the direct damage signaling model. Moreover, Meyers et al. reported that MMR-deficient cells are resistant to fluoropyrimidines but not Tomudex™ (i.e., ICI D1694, ZD1694, Raltiterxed), a non-pyrimidine TS inhibitorCitation8 which supports the importance of incorporation of 5-FU into DNA for MMR-induced cytotoxicity independent of the inhibition of TS. On the other hand, our data also suggests the importance of the futile cycle model which for patients simulate long-term or sustained 5-FU treatment over time, compared with the direct damage model which may simulate a single treatment of 5-FU.Citation33 A single dose treatment allows for a rapid recovery of the dTTP pool, and any 5FdU-containing sites within DNA cannot remain for long periods due to subsequent repair with dTTPs. In this scenario, the opportunity for DNA MMR to trigger the majority of cancer cells to undergo cell death may be low. Sustained 5-FU treatment of MMR-proficient cells demonstrated a 90% reduction in cells by clonogenic assay,Citation8,Citation14 compared with our 5-FU plasmid model which showed a 38.2% reduction by clonogenic assay. Our method is a one-time transfection resembling a single dose treatment without TS inhibition. Overall, our study and previous reports indicated the importance of both the futile cycle model and direct damage signaling model. Importantly, our model focuses on the recognition of 5-FU within DNA, isolating this from the TS and RNA effects that could confound analysis.
It would be much easier and simpler if annealed short dsDNA could be utilized to assess DNA MMR induced cytotoxicity once it recognizes 5-FU in DNA, and like our plasmid construct, cytotoxicity had not been previously assessed with linear DNA. Although it is surprising that little data are available regarding cellular responses induced by transfected dsDNA, Igoucheva et al. demonstrated different cellular responses to exogenous DNA that depended upon the structure and length of the DNA.Citation34 According to their data, cells transfected with closed plasmid DNA showed a dramatic change in gene expression profiles compared with linear DNA. Notably, genomic stability/repair pathway-associated genes that included the DNA MMR genes were activated in respond to plasmid DNA, whereas cells transfected with short dsDNA did not induce this response. Furthermore with observation of a DNA MMR-dependent 5-FU cytotoxicity with our plasmid model, there was no such response utilizing the mismatched T:G heteroduplex plasmid. With these data in mind, we hypothesize that the triggering of cytotoxic responses with exogenous DNA is dependent on the structure of the transfected DNA independent of the DNA lesion. This could be due to differences in degradation speed between the closed dsDNA plasmid and the short DNA oligo (closed dsDNA may be degraded more slowly than short DNA oligo), or by variation in the recognition level by MMR proteins for 5-FU in DNA (MMR proteins may recognize 5-FU in dsDNA plasmid more strongly than in short DNA oligo). Our data demonstrate the utility of the 5-FU plasmid to study the direct DNA damage response by DNA MMR and to ascertain which molecules tend to react with 5-FU incorporated into DNA.
In conclusion, we constructed 5FdU-containing heteroduplex plasmids and utilized it to detect a pure DNA MMR system response executing 5-FU's cytotoxicity. Our data indicates a direct DNA damage response by DNA MMR upon recognition of 5-FU within DNA. These results bridge the gap between prior clinical and biochemical evidence regarding how DNA MMR recognizes 5-FU within DNA and a cell's subsequent response to that recognition. Our plasmid method should prove practical to explore 5-FU sensitivity of cells by DNA MMR molecules and to further an approach how to do personalize treatment for colorectal cancer patients based on the biological and genetic make-up of their tumor.
Materials and Methods
Cell lines and cultures.
The human colorectal cancer cell lines SW480 (MMR-proficient), HCT116 (hMLH1-/- and hMSH3-/-) and DLD-1 (hMSH6-/-) were obtained from American Type Culture Collection and maintained in Iscove's modified Dulbeeco's medium (IMDM, Invitrogen) with 10% fetal bovine serum and penicillin (100 U/ml)/Streptomycin (100 µg/ml) (Invitrogen) as supplements at 37°C in a 5% CO2/95% air incubator. HCT116 cells are a model of complete DNA MMR deficiency, DLD-1 cells are a model of hMutSα deficiency and SW480 cells are a model of MMR-proficiency. We have previously shown that hMutSα is important in the binding of 5-FU within DNA.Citation7
Generation of single-stranded DNA (ssDNA) plasmid.
Generation of ssDNA was performed as described in reference Citation19, with modification (). Briefly, E. coli JM109 competent cells (Promega) were transformed with phagemid pGEM7Zf(+) (Promega) and spread onto 100 µg/ml ampicillin-containing Luria-Bertani (LB) plates. Single colonies of JM109/pGEM7Zf(+) cells was picked from the LB plate, resuspended in 6 ml of LB medium containing 100 µg/ml ampicillin and incubated for 5 h with shaking at 240 rpm at 37°C. Subsequently, 3.0 × 109 pfu of M13KO7 helper phage (New England Biolabs, Beverly, MA) was added to the culture and incubation with shaking was continued at 240 rpm at 37°C for another 1 h. Then, 20 µl of the culture solution was spread onto a selective LB plate containing 100 µg/ml ampicillin and 70 µg/ml kanamycin and incubated at 37°C. Because M13 KO7 confers kanamycin resistance, a number of colonies of JM109/pGEM7Zf(+)/M13KO7 complex were observed after overnight incubation. Individual colonies of JM109/pGEM7Zf(+)/M13KO7 were grown in 40 ml of LB medium containing 100 µg/ml ampicillin and 70 µg/ml kanamycin. The supernatant was transferred to a 50 ml centrifuge tube (Corning Inc.) and mixed with 12.5 ml of polyethylene glycol 6,000—2.5 M liquid mixture that was centrifuged at 10,000 rpm at 4°C. After the supernatant was removed, the precipitant was mixed with 500 µl of Tris-EDTA (TE) buffer, followed by phenol/chloroform extraction and ethanol precipitation for isolation of ssDNA from phage particles.
Construction of heteroduplex plasmids.
The 5′ phosphorylated 25 mer oligonucleotides containing 5FdU (5′-CTT GGC GTA ATC ATG GT-5FdU-ATA GCT G-3′), mismatched thymine (5′-CTT GGC GTA ATC ATG GT-T-ATA GCT G-3′) as a positive control and unaltered cytosine (5′-CTT GGC GTA ATC ATG GT-C-ATA GCTG-3′) as a negative control at the minus strand nucleotide position 160 of pGEM7Zf(+) were synthesized (Sigma-Aldrich). Twenty micrograms of plus single-stranded plasmid DNA were annealed with 5-fold molar excess of the 5′ phosphorylated 25mer oligonucleotides in a 100 µl reaction mixture containing 1× T4 DNA ligase reaction buffer (New England Biolabs) at 86°C for 6 min. Then the mixture was slowly cooled to room temperature. After the annealing reaction, 25 U T4 DNA polymerase (New England Biolabs), 2,400 U T4 DNA ligase (New England Biolabs), 600 µM dNTP and 1 mM ATP were added and primer extension was allowed to proceed at 37°C for 30 min. Closed circular DNA was isolated by agarose gel electrophoresis and extracted using the QIAquick® Gel Extraction Kit (Qiagen), then purified by consecutive isoamyl alcohol extraction and ethanol precipitation and re-dissolved in TE buffer (). It has been demonstrated previously that 5-FU becomes ionized at physiological pH (closer to 7.4) and tends to pair with guanine on the cDNA strand, whereas 5-FU tends to pair with an opposing adenine at higher pH conditions such as under conventional PCR conditions (pH 8.7) when 5-FU is in its keto form.Citation27 Thus the 5FdU-inserted site within the plasmid was confirmed by polymerase chain reaction (PCR) using HotStarTaq DNA polymerase (Qiagen) at pH 8.7, which can detect the 5-FU:G pair site as an A:G hetero-wave with direct DNA sequencing utilizing a primer that anneals to (−)strand of pGEM7Zf(+) (5′-CGC AAC GCA ATT AAT GTG AG-3′).
Construction of heteroduplex linear dsDNA.
We synthesized 61 mer oligonucleotides containing 5FdU (5′-AGA ACC CAC TGC TTA CTG GCG GGC GGG CGG-5FdU-GGC GGG CGG GGC CTT CTA GTT GCC AGC CAT-3′), mismatched thymine (5′-AGA ACC CAC TGC TTA CTG GCG GGC GGG CGG-T-GGC GGG CGG GGC CTT CTA GTT GCC AGC CAT-3′) as a positive mismatch paired control and unaltered cytosine (5′-AGA ACC CAC TGC TTA CTG GCG GGC GGG CGG-C-GGC GGG CGG GGC CTT CTA GTT GCC AGC CAT-3′) as a negative control (Sigma-Aldrich). To complete the dsDNA molecule, the complementary sequence of the 61 mer was synthesized (5′-ATG GCT GGC AAC TAG AAG GCC CCG CCC GCC GCC GCC CGC CCG CCA GTA AGC AGT GGG TTC T-3′) (Sigma-Aldrich) and equal molar ratios of the 61 mer containing the 5FdU, mismatched thymine or unaltered strand were mixed with the complementary strand were mixed, heated to 95°C and allowed to cool slowly to room temperature.
Transfection of constructs into cells.
Cells were transfected with 5 µg of pGEM7Zf(+) 5FdU, mismatched T containing heteroduplex plasmids/linear dsDNA or unaltered negative controlled plasmids/linear dsDNA by using Nucleofector kit V (for SW480 and HCT116) or L (for DLD-1) (Amaxa), following the manufacturer's instructions. After 12 h from transfection, cells were used for cell proliferation assays.
Cell growth assays.
Twelve hours after transfection of plasmids or linear dsDNA, cells were seeded at a density of 5,000 cells per well into 96-well plates in culture medium. After 24, 48 and 72 h, the number of viable cells was counted by using a CellTiter 96® AQueous One Solution Cell Proliferation Assay Kit (Promega) according to the manufacturer's instructions. The kit detects mitochondrial nicotinamide adenine dinucleotide dehydrogenase activity in live cells by measuring reduction of the 3-(4,5-dimethylthiazol-2-yl)-5-(3-carboxymethoxyphenyl)2-(4-sulfophenyl)-2H-tetrazolium, inner salt (MTS). Measurement of the absorbance of the formazan was performed in 96 well microplates read at 490 nm. For clonogenic survival assays after plasmids or linear dsDNA transfection, transfected cells were diluted to 5 × 102 cells/100 mm dishes, plated in complete medium and cultured for 14 d. The colonies obtained were stained with Giemsa (Sigma-Aldrich) and counted.
Statistical analysis.
Comparisons were made using Student's t-test: p value less than 0.05 taken as statistically significant.
Disclosure of Potential Conflicts of Interest
No potential conflicts of interest were disclosed.
Abbreviations
5-FU | = | 5-fluorouracil |
CRC | = | colorectal cancer |
MMR | = | mismatch repair |
5FdU plasmid | = | 5FdU-containing heteroduplex plasmid |
5FdU linear DNA | = | 5FdU-containing linear dsDNA |
LB | = | luria-bertani |
TS | = | thymidylate synthetase |
PCR | = | polymerase chain reaction |
TTP | = | thymidine triphosphate |
dTTP | = | deoxythymidine triphosphate |
5FdU | = | 5-fluorodeoxiuridine |
dUMP | = | deoxyuridine monophosphate |
dUTP | = | deoxyuridine triphosphate |
dRTP | = | deoxyribonucleotide triphosphate |
MTS | = | 3-(4,5-dimethylthiazol-2-yl)-5-(3-carboxymethoxyphenyl)-2-(4-sulfophenyl)-2H-tetrazolium, inner salt |
Figures and Tables
Figure 1 Efficient construction of 5FdU containing heteroduplex plasmid. (A) Flowchart of for preparation of the 5FdU containing heteroduplex plasmid. (B) Electrophoretic analysis of various DNA preparations. Lane 1; parental pGEM7Zf(+) dsDNA plasmid isolated by an alkaline lysis method. Almost of all the plasmid was in supercoiled form. Lane 2; single-stranded DNA plasmid generated by conventional M13KO7 method (17). Lane 3; Single-stranded DNA isolated from JM109/pGEM7Zf(+)/M13KO7 complex. Lane 4; 5FdU-containing heteroduplex plasmid constructed by ssDNA plasmid generated by conventional M13KO7 method. Lane 5; 5FdU-containing heteroduplex plasmid constructed by ssDNA plasmid isolated from JM109/pGEM7Zf(+)/M13KO7 complex. Lane 6; linear dsDNA marker (1 kb Ladder N3232S, New England Biolabs). We generated less contaminated ssDNA by the bacteria/phagemid/helper phage complex method than by the conventional M13KO7 infection method (17) (lane 2 and 3), which also reduced the amount of contamination after the oligomer extension reaction (lane 4 and 5). (C) Sequence at the heteroduplex site. PCR was performed using the constructed plasmid as a template. After purifying the PCR product, direct sequencing was performed to confirm the heteroduplex site. KM, kanamycin; Amp, ampicillin; Nicked, nicked dsDNA plasmid; Supercoiled, supercoiled plasmid.
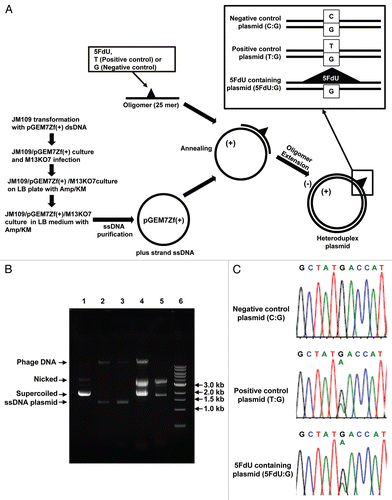
Figure 2 5FdU-containing heteroduplex plasmid leads to cellular morphological changes in MMR-proficient cells. Twelve hours after transfection, hMLH1-/- (A), hMSH6-/- (B) and MMR-proficient (C) cells were seeded at a density of 1.5 × 105 cells per well onto 6-well plates and observed by microscope. By 24 h after seeding, the 5FdU plasmid induced morphological changes consistent with cell death in the MMR-proficient cells (C), while no morphological changes were observed in the hMLH1-/- (A) and hMSH6-/- (B) cells. Neither MMR-proficient cells nor MMR-deficient cells demonstrated morphological changes by heteroduplex (T:G) and negative (C:G) control plasmids. Scale bars; 10 µm. 5FdU:G; 5FdU containing heteroduplex plasmid, T:G; positive control heteroduplex plasmid, C:G; unaltered plasmid (negative control).
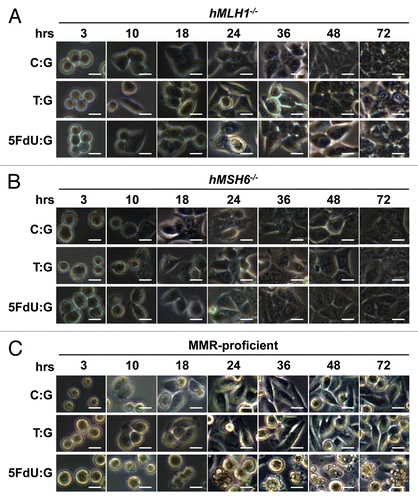
Figure 3 5FdU containing heteroduplex plasmid induces cytotoxicity in MMR-proficient cells. (A) MTS assay. Cells (5,000 cells/well) of each population were seeded in 100 µl of culture medium in the wells of three 96-well plastic plates and plates were incubated in a 5% CO2/95% air incubator for 24 , 48 and 72 h. After incubation, 10 µl of MTS reagent solution was added to each well, and the plate was incubated for an additional 4 h in the 5% CO2/95% air incubator. Cell viability was measured by scanning with a microplate reader at 490 nm. This experiment was performed three times. (B–D) Clonogenic assay. Twelve hours after transfection, hMLH1-/- (B), hMSH6-/- (C) and MMR-proficient (D) cells were seeded at a density of 5 × 102 cells/100 mm dish and colonies were stained with Giemsa and counted 14 days after seeding. Survival fraction was shown as a percentage of negative controls. This experiment was performed at least three times.
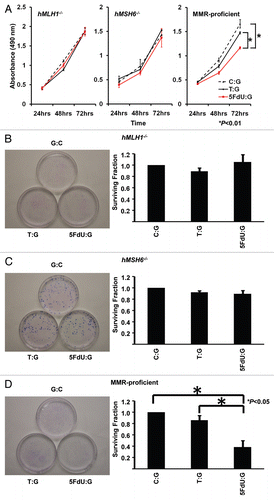
Figure 4 5FdU-containing heteroduplex plasmid is more useful for measurement of cytotoxicity than linear dsDNA. (A) Cell density. Twelve h after transfection, cells were seeded at a density of 1.5 × 105 cells per well into 6-well plates. 72 h after seeding, the cell density was observed by microscopy. 5FdU-plasmid transfected MMR-proficient cells showed lower cell density than 5FdU linear dsDNA transfected cells. (B) Clonogenic assay. Twelve hours after transfection, cells were seeded at a density of 5 × 102 cells/100 mm dish and colonies were stained with Giemsa and counted 14 days after seeding. Survival fraction was shown as a percentage of negative controls. This experiment was performed at least three times. Scale bars; 100 µm.
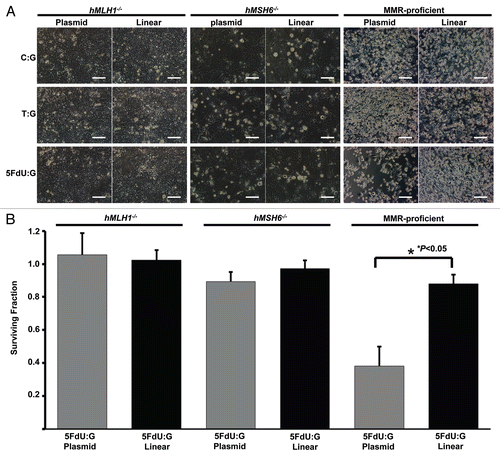
Acknowledgments
Supported by the United States Public Health Service (DK067287 to J.M.C.).
References
- Laurie JA, Moertel CG, Fleming TR, Wieand HS, Leigh JE, Rubin J, et al. Surgical adjuvant therapy of large-bowel carcinoma: an evaluation of levamisole and the combination of levamisole and fluorouracil. The North Central Cancer Treatment Group and the Mayo Clinic. J Clin Oncol 1989; 7:1447 - 1456; PMID: 2778478
- Moertel CG, Fleming TR, Macdonald JS, Haller DG, Laurie JA, Goodman PJ, et al. Levamisole and fluorouracil for adjuvant therapy of resected colon carcinoma. N Engl J Med 1990; 322:352 - 358; PMID: 2300087; http://dx.doi.org/10.1056/NEJM199002083220602
- Moertel CG, Fleming TR, Macdonald JS, Haller DG, Laurie JA, Tangen CM, et al. Fluorouracil plus levamisole as effective adjuvant therapy after resection of stage III colon carcinoma: a final report. Ann Intern Med 1995; 122:321 - 326; PMID: 7847642
- Boland CR, Sinicrope FA, Brenner DE, Carethers JM. Colorectal cancer prevention and treatment. Gastroenterology 2000; 118:115 - 128; PMID: 10868902; http://dx.doi.org/10.1016/S0016-5085(00)70010-2
- Efficacy of intravenous continuous infusion of fluorouracil compared with bolus administration in advanced colorectal cancer. Meta-analysis Group In Cancer. J Clin Oncol 1998; 16:301 - 308; PMID: 9440757
- Carethers JM, Chauhan DP, Fink D, Nebel S, Bresalier RS, Howell SB, et al. Mismatch repair proficiency and in vitro response to 5-fluorouracil. Gastroenterology 1999; 117:123 - 131; PMID: 10381918; http://dx.doi.org/10.1016/S0016-5085(99)70558-5
- Tajima A, Hess MT, Cabrera BL, Kolodner RD, Carethers JM. The mismatch repair complex hMutS alpha recognizes 5-fluorouracil-modified DNA: implications for chemosensitivity and resistance. Gastroenterology 2004; 127:1678 - 1684; PMID: 15578504; http://dx.doi.org/10.1053/j.gastro.2004.10.001
- Meyers M, Wagner MW, Mazurek A, Schmutte C, Fishel R, Boothman DA. DNA mismatch repair-dependent response to fluoropyrimidine-generated damage. J Biol Chem 2005; 280:5516 - 5526; PMID: 15611052; http://dx.doi.org/10.1074/jbc.M412105200
- Fischer F, Baerenfaller K, Jiricny J. 5-Fluorouracil is efficiently removed from DNA by the base excision and mismatch repair systems. Gastroenterology 2007; 133:1858 - 1868; PMID: 18054558; http://dx.doi.org/10.1053/j.gastro.2007.09.003
- Carethers JM, Smith EJ, Behling CA, Nguyen L, Tajima A, Doctolero RT, et al. Use of 5-fluorouracil and survival in patients with microsatelliteunstable colorectal cancer. Gastroenterology 2004; 126:394 - 401; PMID: 14762775; http://dx.doi.org/10.1053/j.gastro.2003.12.023
- Jover R, Zapater P, Castells A, Llor X, Andreu M, Cubiella J, et al. The efficacy of adjuvant chemotherapy with 5-fluorouracil in colorectal cancer depends on the mismatch repair status. Eur J Cancer 2009; 45:365 - 373; PMID: 18722765; http://dx.doi.org/10.1016/j.ejca.2008.07.016
- Ribic CM, Sargent DJ, Moore MJ, Thibodeau SN, French AJ, Goldberg RM, et al. Tumor microsatellite-instability status as a predictor of benefit from fluorouracil-based adjuvant chemotherapy for colon cancer. N Engl J Med 2003; 349:247 - 257; PMID: 12867608; http://dx.doi.org/10.1056/NEJMoa022289
- Sargent DJ, Marsoni S, Monges G, Thibodeau SN, Labianca R, Hamilton SR, et al. Defective mismatch repair as a predictive marker for lack of efficacy of fluorouracil-based adjuvant therapy in colon cancer. J Clin Oncol 2010; 28:3219 - 3226; PMID: 20498393; http://dx.doi.org/10.1200/JCO.2009.27.1825
- Liu A, Yoshioka K, Salerno V, Hsieh P. The mismatch repair-mediated cell cycle checkpoint response to fluorodeoxyuridine. J Cell Biochem 2008; 105:245 - 254; PMID: 18543256; http://dx.doi.org/10.1002/jcb.21824
- Chung H, Chaudhry J, Lopez CG, Carethers JM. Cyclin E and histone H3 levels are regulated by 5-fluorouracil in a DNA mismatch repair-dependent manner. Cancer Biol Ther 2010; 10:1147 - 1156; PMID: 20930505; http://dx.doi.org/10.4161/cbt.10.11.13447
- Sambrook J, Russell DW. Working with bacteriophage M13 vector. Molecular Cloning: A Laboratory Manual 2001; 3:Cold Spring Harbor, NY Cold Spring Harbor Laboratory Press 1 - 52
- Jiricny J. The multifaceted mismatch-repair system. Nat Rev Mol Cell Biol 2006; 7:335 - 346; PMID: 16612326; http://dx.doi.org/10.1038/nrm1907
- Hewish M, Lord CJ, Martin SA, Cunningham D, Ashworth A. Mismatch repair deficient colorectal cancer in the era of personalized treatment. Nat Rev Clin Oncol 2010; 7:197 - 208; PMID: 20177404; http://dx.doi.org/10.1038/nrclinonc.2010.18
- Zhou B, Dong Q, Ma R, Chen Y, Yang J, Sun LZ, et al. Rapid isolation of highly pure single-stranded DNA from phagemids. Anal Biochem 2009; 389:177 - 179; PMID: 19348781; http://dx.doi.org/10.1016/j.ab.2009.03.044
- Yamane A, Shinmura K, Sunaga N, Saitoh T, Yamaguchi S, Shinmura Y, et al. Suppressive activities of OGG1 and MYH proteins against G:C to T:A mutations caused by 8-hydroxyguanine but not by benzo[a]pyrene diol epoxide in human cells in vivo. Carcinogenesis 2003; 24:1031 - 1037; PMID: 12807753; http://dx.doi.org/10.1093/carcin/bgg056
- Thomas DC, Roberts JD, Kunkel TA. Heteroduplex repair in extracts of human HeLa cells. J Biol Chem 1991; 266:3744 - 3751; PMID: 1995629
- Holmes J Jr, Clark S, Modrich P. Strand-specific mismatch correction in nuclear extracts of human and Drosophila melanogaster cell lines. Proc Natl Acad Sci USA 1990; 87:5837 - 5841; PMID: 2116007; http://dx.doi.org/10.1073/pnas.87.15.5837
- Stoklosa T, Poplawski T, Koptyra M, Nieborowska-Skorska M, Basak G, Slupianek A, et al. BCR/ABL inhibits mismatch repair to protect from apoptosis and induce point mutations. Cancer Res 2008; 68:2576 - 2580; PMID: 18413724; http://dx.doi.org/10.1158/0008-5472.CAN-07-6858
- Lei X, Zhu Y, Tomkinson A, Sun L. Measurement of DNA mismatch repair activity in live cells. Nucleic Acids Res 2004; 32:100; PMID: 15249596; http://dx.doi.org/10.1093/nar/gnh098
- Sunaga N, Kohno T, Shinmura K, Saitoh T, Matsuda T, Saito R, et al. OGG1 protein suppresses G:C→T:A mutation in a shuttle vector containing 8-hydroxyguanine in human cells. Carcinogenesis 2001; 22:1355 - 1362; PMID: 11532855; http://dx.doi.org/10.1093/carcin/22.9.1355
- Modrich P, Lahue R. Mismatch repair in replication fidelity, genetic recombination and cancer biology. Annu Rev Biochem 1996; 65:101 - 133; PMID: 8811176; http://dx.doi.org/10.1146/annurev.bi.65.070196.000533
- Meyers M, Hwang A, Wagner MW, Bruening AJ, Veigl ML, Sedwick WD, et al. A role for DNA mismatch repair in sensing and responding to fluoropyrimidine damage. Oncogene 2003; 22:7376 - 7388; PMID: 14576845; http://dx.doi.org/10.1038/sj.onc.1206941
- Sowers LC, Eritja R, Kaplan B, Goodman MF, Fazakerly GV. Equilibrium between a wobble and ionized base pair formed between fluorouracil and guanine in DNA as studied by proton and fluorine NMR. J Biol Chem 1988; 263:14794 - 14801; PMID: 3170564
- Kremer AB, Mikita T, Beardsley GP. Chemical consequences of incorporation of 5-fluorouracil into DNA as studied by NMR. Biochemistry 1987; 26:391 - 397; PMID: 3828314; http://dx.doi.org/10.1021/bi00376a009
- Santi DV. Perspective on the design and biochemical pharmacology of inhibitors of thymidylate synthetase. J Med Chem 1980; 23:103 - 111; PMID: 6987403; http://dx.doi.org/10.1021/jm00176a001
- Yang G, Scherer SJ, Shell SS, Yang K, Kim M, Lipkin M, et al. Dominant effects of an Msh6 missense mutation on DNA repair and cancer susceptibility. Cancer Cell 2004; 6:139 - 150; PMID: 15324697; http://dx.doi.org/10.1016/j.ccr.2004.06.024
- Lin DP, Wang Y, Scherer SJ, Clark AB, Yang K, Avdievich E, et al. An Msh2 point mutation uncouples DNA mismatch repair and apoptosis. Cancer Res 2004; 64:517 - 522; PMID: 14744764; http://dx.doi.org/10.1158/0008-5472.CAN-03-2957
- Muggia FM, Peters GJ, Landolph JR Jr. XIII International Charles Heidelberger Symposium and 50 Years of Fluoropyrimidines in Cancer Therapy Held on september 6 to 8, 2007 at New York University Cancer Institute, Smilow Conference Center. Mol Cancer Ther 2009; 8:992 - 999; PMID: 19417150; http://dx.doi.org/10.1158/1535-7163.MCT-08-0731
- Igoucheva O, Alexeev V, Yoon K. Differential cellular responses to exogenous DNA in mammalian cells and its effect on oligonucleotide-directed gene modification. Gene Ther 2006; 13:266 - 275; PMID: 16177821; http://dx.doi.org/10.1038/sj.gt.3302643