Abstract
It is now widely accepted that the development and progression of a tumor toward the malignant phenotype is highly dependent on interactions between tumor cells and the tumor microenvironment. Different components of the tumor microenvironment may have stimulatory or inhibitory effects on tumor progression by regulating the gene expression repertoire in tumor cells and stromal cells.
This review analyzes novel research findings on breast cancer progression, discussing acquisition of the metastatic phenotype in breast disease in relation to different aspects of cross-talk among components of the tumor microenvironment. Knowledge of the interaction of all of these factors would contribute to elucidating the mechanisms that disrupt regulatory/signaling cascades and downstream effects in breast cancer.
Introduction
Breast cancer research has traditionally focused on the tumorigenic transformation of breast epithelial cells due to the influence of accumulated genetic alterations in the cells on cell proliferation and organization, among other processes. However, there are also concurrent structural, cellular, functional, genetic, and epigenetic changes in the surrounding stromaCitation1-Citation3 that alter the effects of normal stroma on the growth, morphogenesis, and plasticity of epithelial cells, contributing to the development of the tumorigenic epithelial phenotype.Citation4 Although cancer development derives from multiple processes in which epithelial cells are targeted and transformed, it is evident that genetic and epigenetic alterations in epithelial cells do not provide the sole explanation of carcinogenesis.
Mina Bissell was a pioneering researcher in breast cancer who highlighted the crucial role of the extracellular matrix (ECM) and nuclear environment in gene expression of normal and malignant tissues.Citation5-Citation7 She developed the term “dynamic reciprocity,” which emphasizes the role of the cell in constantly responding to and modulating the environment, considering tissue-specific histogenic properties to be the result of a dynamic reciprocity between stroma and epithelium.
Other authors have reported that the epithelial phenotype is regulated by a combination of forces from outside and inside the cell acting on the matrix that surround them.Citation8 Hence, tumor cells are exposed to a myriad of altered mechanical forces that dramatically modify their behavior, and these findings directly implicate matrix changes in tumor progression.Citation9
The importance of the microenvironment in the regulation of carcinogenesis is well-documented. Some authors have reported that cancer cells can reverse their malignant properties when placed among normal tissues. Thus, the recombination of mammary carcinoma cells with normal mammary gland stroma demonstrated the ability of the stroma to reprogram neoplastic epithelial cells.Citation10 Bussard and coworkersCitation11 first demonstrated the capacity of human cells to recognize signals generated by the mouse mammary gland microenvironment during gland regeneration in vivo. According to these findings, human embryonal carcinoma-derived progeny interact with mouse mammary cells during mammary gland regeneration and are directed to differentiate into cells that exhibit diverse mammary epithelial cell phenotypes. Using a theory-neutral experimental strategy, Maffini et al.Citation12 separately exposed mammary stroma and normal mammary epithelial cells to a rapidly metabolized chemical carcinogen and to vehicle and then recombined these tissues to determine which combination resulted in mammary carcinomas. The recombination of carcinoma-exposed stroma with vehicle-exposed epithelium resulted in neoplasms. Taken together, these experimental observations evidence the pivotal role of the stroma in mammary gland carcinogenesis, supporting the concept of carcinogenesis and neoplasia as emergent supracellular phenomena that are highly dependent on tissue architecture.
It has been clarified that epigenetic and the tumor microenvironment play a crucial role during tumor development, and a wide range of pathways have been implicated in cancer, including self-sufficiency in growth signals, insensitivity to anti-growth signals, limitless replicative potential, evasion of apoptosis, sustained angiogenesis, tissue invasion, and metastasis.Citation13 Inflammation was recently added to this list; an inflammatory component is present in the microenvironment of most neoplastic tissues, and the connection between inflammation and cancer goes beyond an increased risk for a subset of tumors.Citation14
The aim of this article was to review and integrate recent data on microenvironmental components in tumor progression.
Role of Microenvironment in Tumor Initiation and Progression
The initiation of breast cancer is known to be due to transforming (genetic and epigenetic) events in a single cell.Citation15 There are two prevailing models of breast cancer tumorigenesis and evolution: “the cancer stem cell hypothesis”Citation16 and the stochastic model of carcinogenesis.Citation17
Numerous observations in human patients also support the hypothesis that the host microenvironment plays an important role in breast cancer tumorigenesis. For example, polymorphism in various genes encoding ECM proteins influences the risk of breast cancer metastasis.Citation18 Whole-genome analyses of breast cancer in patients with and without BRCA1/2 mutations have determined the extent of genomic instability in the malignant breast epithelium and in the adjacent tumor stroma. These results suggest that accumulation of genomic instability in the cancer stroma might facilitate a microenvironment that promotes genomic instability in the epithelium and, subsequently, neoplastic transformation.Citation19
Pathologists have long noticed that the tissue microenvironment dramatically changes during tumor formation, and it is widely believed that the tumor-associated stroma is activated by malignant epithelial cells to foster tumor growth by secreting growth factors, increasing angiogenesis or facilitating cell migration, among other mechanisms. These phenomena eventually lead to remote organ metastasis, as in the progression from normal mammary gland to ductal carcinoma in situ (DCIS) and finally to invasive ductal carcinoma (IDC).Citation20 It has also been demonstrated that paracrine interactions between stromal and tumor epithelial cells can promote the proliferation, invasiveness, tumorigenicity, and metastatic potential of immortalized epithelial or cancer cells.Citation21-Citation24
Based on these assumptions, several groups performed comprehensive gene expression and genetic profiling studies to compare among in situ, invasive, and metastatic breast carcinomas, but they failed to identify any tumor stage–specific gene signatures.Citation25 However, they focused mainly on tumor epithelial cells and there was little in-depth study on the potential involvement in tumor progression of other epithelial and myoepithelial cells or of the stroma.Citation26 Other studies have reported somatic genetic alterations, including gene copy number changes, microsatellite instability (MSI) and point mutations in tumor suppressor genes, and oncogenes in breast tumor stroma.Citation27-Citation34
In 2004, FukinoCitation31 compared global genomic alterations between carcinomatous epithelium and tumor stroma from 134 sporadic invasive breast carcinoma samples. The results suggested that clonal selection in malignant epithelial cells is strongly biased toward a smaller subset of loci in comparison to that observed in stromal cells. In 2007, the same group analyzed the relationship between stromal genomic alterations and clinicopathological features of sporadic breast cancer. They concluded that clinical tumor progression, as assessed from clinical-pathological features, may be more influenced by locally acquired changes in the stromal environment than by the carcinoma cell genotype per se.Citation32 Hence, the combination of stromal and epithelial genetic changes produces a greater range of outcome scenarios than can otherwise be explained by carcinoma cell genotype alone ().
Figure 1. Microenvironmental players in breast cancer development. (1) Tumor initiation and progression: genetic changes involved (genetic profile changes, microsatellite instability, tumor suppressor gene and oncogene point mutations, LOH); epithelial to mesenchymal transition (EMT); bystander effects (BEs) are related to soluble factors (TNFα, free radicals -ROS, RNS-); sublethally-irradiated fibroblasts enhance tumor growth; metalloproteinases (MMPs) control the growth-antigrowth balance. (2) Tumor invasion: DCIS to IDC transition is associated with stromal gene expression changes; MMPs are responsible for altering extracellular matrix remodeling; mesenchymal-epithelial interactions (MEIs) are a consequence of the transcriptional response of fibroblasts to Wnt signals; E-cadherin cleavage is produced by MMPs. (3) Angiogenesis: extracellular matrix metalloproteinase inducer (EMMPRIN) appears to modulate VEGF secretion in an MMP-dependent manner; hypoxic conditions stimulate HIF-1α production by TAMs. (4) Immune response: leukocyte recruitment and tumor microenvironment (TME) remodeling is performed by some members of RAS and MYC gene families; Some MMPs modulate the bioactivity of CXCL-1/KC and CXCL-8/IL-8 implicated in chemotactic activity on neutrophils; cytokines control the immune and inflammatory milieu. (5) Metastasis: lymphovascular invasion (LVI) is related to the presence of lymph node metastasis and is a marker of poor prognosis. Mobilization of bone marrow-derived cells induced by primary tumor (PT) generates pre-metastatic niche (PMN); epigenetics regulation; HGF-HDAC-CXCR-4 interactions related to invasive phenotype.
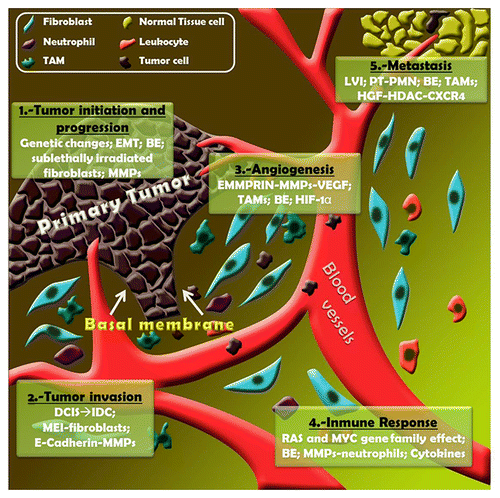
Normal epithelial cells are not affected in the same way by cancer-associated fibroblasts, indicating that abnormalities must be present in both epithelial and stromal cell compartments.Citation35,Citation36 In fact, tumor growth is more enhanced by fibroblasts that are sublethally irradiated before injection than by unirradiated cells, due to the former’s acquisition of an activated phenotype. The tumor-promoting role of irradiated stromal cells has raised concerns about the possible undesirable side-effects of radiation therapy during cancer treatment, suggesting that radiation-induced cancer might not solely result from genetic damage in tumor epithelial cells.Citation37 Thus, Nguyen et al. used an experimental model to demonstrate the effects of ionizing radiation (IR) in the early development of estrogen receptor (ER)-negative breast cancers. They showed how both of these characteristics (early onset and ER-negativity) could be caused by the effects of IR on the stroma, independent of any direct effect of IR on the epithelium. This effect is dependent upon the stromal production of transforming growth factor-β (TGFβ), differentiating between the contributions of stromal and epithelial tissues to the development of mammary gland tumors.Citation38
In 2004, the first systematic profiling of the various stromal cell types by Allinen demonstrated that gene expression alterations in the tumor microenvironment of all types accompany the progression from normal breast tissue to DCIS to IDC, evidencing the participation of all of these cell types in tumorigenesis.Citation39 In 2009, MaCitation40 extended gene expression analysis to the tumor stromal microenvironment, where he observed extensive gene expression alterations, similar to previous findings in the tumor epithelium,Citation41 even at the pre-invasive DCIS stage.
Finak recently developed a stroma-derived prognostic predictor that forecasts clinical outcome with greater accuracy in comparison to predictors or signatures derived from whole tissue.Citation42 The results published by this author reflect the heterogeneity of breast cancer, whose outcome is affected by genetic and epigenetic changes in tumor epithelium as well as stroma. Thus, Farmer showed that increased stromal gene expression is predictive of resistance to chemotherapy in the setting of a randomized controlled trial.Citation43
Invasion and Metastasis: Key Events for Tumor Expansion
The most important moment in tumor progression is the DCIS-IDC transition and the metastatic scatter from the primary tumor to remote organ sites. DCIS is distinguished from IDC by the extension of malignant epithelial cells beyond the myoepithelial cell layer into the basement membrane,Citation44 a cell-associated ECM that underlies the epithelia, endothelia, and surrounding peripheral nerve fibers, muscles, and fat cells. However, unlike the epithelial compartment, which demonstrates no or scant gene expression changes at the transition from DCIS to invasive carcinoma, the stromal compartment demonstrates significant gene expression change at this transition point (). More specifically, whereas only three epithelial genes were differentially regulated at the transition from DCIS to invasive cancer, 76 stromal genes were upregulated and 229 stromal genes were downregulated at the same transition point. Genes in the epithelium were predominantly associated with the cell cycle, whereas those in the stroma were associated with ECM components and the matrix metalloproteases (MMPs) responsible for ECM remodeling. The stromal genes also included those related to the cell cycle, indicating that increased proliferation is common to both the tumor epithelium and stroma.Citation45
An emerging area in tumor microenvironment research suggests that invasive cancer cells actively recruit stromal cells and interact with them to create a tumor microenvironment that promotes tumor growth and possibly metastasis (). Metastasis is an extraordinarily complex process. In order to successfully colonize a secondary site, a cancer cell must complete a sequential series of steps, which typically includes: separation from the primary tumor, invasion through surrounding tissues and basement membranes, entry and survival in the circulation, lymphatics, or peritoneal space, and arrest in a distant target organ.Citation46 These are usually, but not always,Citation47 followed by extravasation into the surrounding tissue, survival in the foreign microenvironment, proliferation, and induction of angiogenesis, all the while evading apoptotic death and the immune response.
The locoregional and systemic spread of breast cancer cells to lymph nodes and distant organs takes place after the invasion of tumor cells into lymphatic channels and blood vessels, respectively. Although the lymphatic and blood vessels of the breast can be discerned by using immunohistochemical markers, this is not usually done in practice, and the invasion of lymphatic and blood vessels is collectively reported as “lymphovascular invasion” (LVI). LVI is present in approximately 15% of invasive ductal breast carcinomas, although its frequency differs widely among studies (5–50%). It is unusual to find LVI in lobular carcinomas.Citation48 LVI correlates with the presence of lymph node metastasesCitation49,Citation50 and indicates a poor prognosis.Citation51-Citation53 El-GoharyCitation54 investigated intratumoral and peritumoral lymphatic and blood vessel densities and their correlation with various other prognostic parameters in breast cancer, including LVI and survival data. He found that D2-40-detected LVI and CD31-detected microvessel density are valuable tools for identifying patients with higher risk of metastatic disease and poorer survival prognosis. Results obtained by Ejlertsen confirm that LVI is a marker of poor prognosis in patients with early-stage breast cancer, finding statistically significant evidence for heterogeneity in the association between LVI and overall survival according to risk group.Citation55
Increasing research attention has been paid to the participation in metastasis of the microenvironment, which is now recognized as a potentially important factor in cancer progression. Biomechanical remodeling of the microenvironment by stromal caveolin-1 (Cav1) has been implicated in tumor invasion and metastasis. Cav1 plays an important role in the topographical organization of tissues, and its expression modifies mammary tissue architecture. A recent study used immunostaining for Cav1 in a tissue microarray of 132 primary breast tumors and 35 normal breast tissues in order to score specifically for expression level in fibroblastic stroma, reporting significantly higher Cav1 expression in tumor stroma (p < 0.0001). Cox proportional hazards analysis showed that patients with stromal Cav1 expression had a 2.5-fold higher 10-y mortality risk. In vitro assessments confirmed that Cav1 expression was significantly higher in breast cancer-associated fibroblasts than in normal fibroblasts. These results suggest that stromal Cav1 expression may be a potential indicator of breast carcinoma progression. The same authors also examined the contribution of fibroblastic Cav1 to stroma-dependent tumorigenesis in an in vivo evaluation of Cav1-dependent matrix remodeling at the tumor-stroma interface of early tumors (8 d), showing that tumors grown in Cav1 knockout mice were minimally invasive. They identified Cav1 as an important key of ECM remodeling and highlighted its role in tumorigenesis through the regulation of matrix-dependent cell behavior.Citation56 It has also been argued that the increased contractility of tumor cells and their associated stromal fibroblasts induce tension-dependent matrix remodeling to promote the linear reorientation of collagen fibers surrounding the invasive front of the tumor.Citation8
Microenvironmental cues have been described as playing a major role at secondary and primary tumor sites, and it was recently demonstratedCitation57 that primary tumors induce the mobilization of bone marrow-derived cells to metastatic target organs before the arrival of disseminated tumor cells.Citation58 These cells take residence within the target organ and are thought to establish a pre-metastatic niche, i.e., a set of novel microenvironmental stimuli conducive to tumor cell survival and growth. Thus, tumor cells induce changes in the microenvironment via the cytokine-mediated mobilization of bone marrow cells and are themselves subject to the new conditions established by these cells at the secondary site.Citation58
Epigenetic Control of Tumor Development
Epigenetic changes are alterations in gene expression induced by DNA methylation, histone modifications, and RNA-associated silencing that describe mitotically stable states and changes in gene activity.Citation59
Because epigenetic states are reversible, they can be modified by environmental factors, which may contribute to the development of abnormal phenotypes.Citation59 Epigenetic changes can be alternative mechanisms that also underlie the relative stability of abnormal stromal cell phenotypes. Results published by Lin indicated that tumor stromal fibroblasts support neoplastic progression by manipulating the epigenome of mammary epithelial cells.Citation60
DNA methylation
Global DNA hypomethylation is associated with gene reactivation and chromosomal instability, and up to 50% of breast cancer cases show reduced 5-methylcytosine content in comparison to normal tissue.Citation61
Hypermethylation is one of the best-documented epigenetic events in mammalian cells.Citation62 When CpG islands are hypermethylated, the activity of the regulatory proteins that promote transcription is restrictedCitation63 by the tight packing nucleosomes, and this mechanism is therefore involved in gene repression and chromosomal instabilities.Citation64 Results of hypermethylation include suppression of tumor suppressor genes, chromatin condensation, and suppression of DNA repair genes.
In breast cancer, numerous genes that are unmethylated in non-malignant tissue become aberrantly methylated in the tumor, including the tumor suppressor gene BRCA1.Citation65 The tumor expression of ERs is a very important prognostic marker that predicts the response to endocrine therapy. In a substantial proportion of breast cancers, the loss of ER expression, which carries a poor prognosis, results from the hypermethylation of CpG islands within the ER-α promoter.
The past two decades have seen growing recognition of the importance of the microenvironment in the control of cellular differentiation and tissue polarity.Citation66,Citation67 In this regard, PlachotCitation66 suggested that DNA methylation is a mechanism by which mammary epithelial differentiation is coordinated at both cellular and tissue levels; he found acinar morphogenesis to be accompanied by chromatin remodeling and increased expression of MeCP-2, a mediator of DNA-methylation-induced gene silencing, revealing that the epigenome can be influenced by the microenvironment.
Dumont recently hypothesized that cells in development, differentiation, or disease states are in an “epigenetically plastic state” and can therefore be programmed by the microenvironment to acquire epigenetic changes associated with tumorigenesis.Citation68 In the same line, Hu applied a methylation-specific digital karyotyping technique to epithelial and myoepithelial cells and stromal fibroblasts from normal breast tissue and from in situ and invasive breast carcinomas.Citation69 He found distinct epigenetic alterations in all three cell types during breast tumorigenesis in a tumor stage- and cell type-specific manner, suggesting that epigenetic changes play a role in maintaining the abnormal cellular microenvironment in breast cancer.
Fiegl studied DNA methylation profiles in cancer cells and tumor stroma in Her-2/neu positive patients and found aberrant DNA methylation in both the stroma and associated cancer cells, reporting significant differences in HER-2/neu expression and DNA methylation of five genes.Citation70 Two of these genes, which are both involved in estrogen metabolism (progesterone receptor [PGR] and17-β-estradiol metabolizing enzyme [HSD17B4]), were concordantly methylated in the stroma and associated cancer cells. Hence, the question as to which cell type initiated the aberrant methylation appears to be unresolved.
miRNAs
MicroRNAs (miRNAs) are small non-coding RNAs of 18–25 nucleotides in length that function as negative regulators. MiRNAs post-transcriptionally regulate gene expression by either inhibiting mRNA translation or inducing mRNA degradation, and they participate in a wide variety of physiological and pathological cellular processes (e.g., proliferation, differentiation, apoptosis and development)Citation71 by simultaneously controlling the expression levels of hundreds of genes.Citation72,Citation73 Overexpressed miRNAs in breast cancer may function as oncogenes by downregulating tumor-suppressor genes, and miRNA genes are frequently located at fragile sites and in minimal regions of LOH, minimal regions of amplification, and common breakpoint regions.Citation74 The first genome-wide profiling of miRNAs in breast cancerCitation75 identified 29 differentially expressed candidate miRNAs, of which 15 distinguished between breast cancer and normal breast tissue. In a bioinformatic analysis, Dalmay and EdwardsCitation76 proposed potential miRNA target sites on 3′UTR sequences of genes that are involved in metastasis and that underlie the morphological changes associated with malignancy in the microenvironment.
As in the case of protein-coding genes, DNA sequences encoding miRNA were found to be a target of aberrant DNA methylation,Citation77 explaining in part how miRNAs may be upregulated (through DNA hypomethylation) or downregulated (through DNA hypermethylation) in cancer. In this context, authors have identified some miRNAs that are repressed by CpG hypermethylation in cancers in comparison to normal tissue (i.e. miR-9-1 in breast cancer).Citation78 In relation to this finding and observations that the distant tumor microenvironment can affect metastasis, it was recently reportedCitation79 that miRNAs against Tac-1 mRNA may affect quiescence of breast cancer cells in the marrow cavity, promoting bone marrow metastasis.
Furthermore, miRNAs are involved in stromal cell interaction with hematopoietic stem cells (HSCs) and the neural-hematopoietic-immunological system. HSC functioning involves the production of hematopoietic regulators, including cytokines, neuropeptides, and neurotransmitters, to which stromal cells are able to respond in an adaptive manner. MiRNAs have also been proposed to serve as the link between cancer and chronic inflammation, although the precise role of miRNAs in these biological processes remains unclear.Citation80
Histone modifications
The post-translational acetylation and methylation of lysine residues in histone tails is another epigenetic process that can regulate gene expression. Histone acetylation typically results in an “open” chromatin configuration that facilitates the access of transcription factors to DNA and gene transcription, but the reverse scenario can silence tumor suppressor genes in cancer cells.Citation81,Citation82
Changes in the composition of the ECM have been shown to induce a global and complete reorganization of chromatin. Recently, Sandal proposed a link between ECM-chromatin remodeling and changes in cell phenotypeCitation83 via ECM, type I collagen, and epithelial-mesenchymal transition (EMT).
Interactions Between Stromal and Tumor Epithelial Cells
Several mitogens stimulate both wound re-epithelialization and carcinoma growth. SDF-1α is a mitogen for keratinocytes and is expressed in endothelial cells and fibroblasts of normal or wounded skinCitation84 and tumors. Other keratinocyte mitogens have been identified as crucial regulators of the proliferation process, including members of the epidermal growth factor (EGF) family.Citation85 These mitogens are also frequently overexpressed in cancer and have been found to stimulate tumor cell proliferation and invasion.Citation86 In contrast to these positive regulators of epithelial repair, transforming growth factor-β (TGF-β) and its downstream effector SMAD-3 are negative regulators of wound re-epithelialization and also inhibit proliferation of numerous carcinoma cells, at least in the early stages of tumorigenesis.Citation45,Citation87 Therefore, it seems likely that cancer cells reactivate a latent behavioral program that is usually confined to embryonic development and tissue repair but operates in cancer cells in an exaggerated and uncontrolled manner.Citation45
Infiltration of lymphocytes, macrophages, mast cells and neutrophils is a hallmark of inflammatory, defense, and tissue repair reactions that are often present in tumors, and it not only indicates the presence of the disease but also triggers tumor progression and development. Tumor-associated macrophages (TAMs) are a major component of the leukocyte infiltrate, and activated macrophages have been shown to exert both direct and indirect tumoricidal action.Citation88 Studies on their function using different mouse models of breast cancer revealed that macrophages are required to regulate both the angiogenic switch and tumor progression and therefore play a key role in tumor development, invasion, and metastasis.Citation89,Citation90 The tumor-promoting activities of TAM may result from their ability to express numerous tumor-promoting characteristics, such as growth factors for breast tumor cells, angiogenic mediators, ECM-degrading enzymes (MMPs), and inflammatory cytokines. For instance, monocyte-derived cytokines, such as TNF-α, may intensify chemokine expression by tumor or stroma cells besides possessing other promalignant properties. The resulting positive feedback loop may act in conjunction with other promalignant activities of tumor-derived and inflammatory cell-derived factors and with chemokine-induced site-specific metastasis formation.Citation91
Generally, inflammatory cells can secrete cytokines, growth factors, and chemokines, release reactive oxygen intermediates to stimulate proliferation, prevent apoptosis, direct morphogenesis, and induce mutagenic changes that may increase tumor cell DNA damage.Citation92 The overall effect of these activities may be a key factor in breast cancer metastasis and disease progression.
Oxidative stress
There is growing research interest in oxidative stress as an environmental characteristic of all solid tumors. Free radicals generated during tumor growth are known to damage all cellular components, influencing a variety of molecular signaling pathways that regulate tumor growth, apoptosis, angiogenesis, ECM remodeling, and the formation of intercellular communication structures.Citation93,Citation94
During the course of normal cellular metabolism, oxygen is consumed and reactive oxygen species (ROS) are produced, including superoxide, hydrogen peroxide, and hydroxyl radicals. If not effectively dissipated, ROS can accumulate and damage resident proteins, lipids, and DNA. Enzymes involved in redox regulation and DNA repair scavenge ROS and repair the resulting damage in order to preserve a functional cellular environment, but increased ROS accumulation and/or unrepaired DNA damage can lead to the initiation and progression of cancer.
It is also known that persistent inflammation and the generation of reactive oxygen and nitrogen species (RNS) play pivotal roles in tissue injury during disease pathogenesis and in the reaction to toxicant exposures via sustained inflammation, cellular proliferation, cytotoxicity, and induction of a pro-angiogenic environment.Citation95 Curtis recently identified a number of oxidative stress and DNA repair proteins that are needed to prevent the accumulation of ROS and influence the estrogen-responsiveness of MCF-7 breast cancer cells, showing that these proteins may be differentially expressed in normal mammary tissue, DCIS, and IDC.Citation96 Some authors demonstrated that these oxidative stress proteins form an interactive network, acting collectively to regulate oxidative stress and maintain a functional cellular environment.Citation97-Citation100
Formation of new blood vessels
Angiogenesis, the formation of new blood vessels from existing vasculature, is a tightly regulated event that plays a major role in embryonic development, wound healing, and pathologic conditions such as tumor growth and progression. Neovascularization is essential for the development of tumors, because it not only supplies nutrients (e.g., growth factors and oxygen) for the growing tumor but also provides a vascular route for metastasis ().Citation101
The most important signaling molecules for the regulation of the vasculature are the vascular endothelial growth factors (VEGF-A, VEGF-B, VEGF-C, VEGF-D), placenta growth factor (PlGF), vascular endothelial growth factor receptors (VEGFR-1, VEGFR-2, VEGFR-3), and angiopoietin growth factors (Ang-1, Ang-2, Ang-3/-4) and their receptors (Tie-1, Tie-2).Citation102-Citation105 Tyrosine kinase receptors, fibroblast growth factor receptor-1 (FGFR-1), VEGF receptors (VEGFRs), and Tie-2 are the main receptors that promote angiogenesis. Tie-2 and VEGFR-1/-2 are selectively expressed by endothelial cells,Citation106 and FGFs promote angiogenesis via dependent crosstalk between FGF-VEGF signaling axes.Citation107 The FGF signaling axis has been shown to be essential during EMT stages through regulation of the EMT-associated zinc finger transcription factor, Snail, a transcriptional repressor of the epithelial phenotype caretaker, E-cadherin.Citation108
VEGF-induced Src activation has also been implicated in the progression and metastasis of various solid tumors, but an alternative intrinsic mechanism of Src activation may be important in both cancer and stromal cells. MezquitaCitation109 recently characterized a new family of intracellular isoforms of VEGFR-1 that result from an alternative initiation of transcription in intronic sequences of the VEGFR-1 gene in cancer and stromal cells.
Inflammatory response
Over the past few decades, researchers have demonstrated that inflammation can promote tumorigenesis. Most if not all solid malignancies trigger an intrinsic inflammatory response that builds up a protumorigenic microenvironment.Citation110 It has been reported that certain oncogenes, e.g., RAS and MYC family members, induce a transcriptional program for remodeling the tumor microenvironment via the recruitment of leukocytes, the expression of tumor-promoting chemokines and cytokines, and the induction of an angiogenic switch.Citation111,Citation112
Oxygen and nutrient needs of tumors are usually inadequately met due to a poor blood supply to their core, producing necrotic cell death and the release of proinflammatory mediators such as IL-1.Citation113 Furthermore, cancer therapy can initiate a strong inflammatory response produced by massive necrotic cell death.Citation114
Tumor initiation may be enhanced by inflammation, which increases mutation rates. In an inflammatory milieu, ROS and RNS, widely produced by immune cells, are able to induce DNA damage and genomic instability.Citation14 Increased mutation rates can also be produced by an alteration in mismatch repair enzymes, and ROS can produce their direct oxidative inactivation.Citation115 Inflammation may also promote cancer cell intravasation through the production of mediators that increase vascular permeability.Citation115
The most frequently found immune cells in the tumor microenvironment are TAMs, which promote tumor growth, angiogenesis, invasion and metastasis.Citation115 TAMs are involved in the hypoxia-triggered formation of new blood vessels, producing chemokines in response to hypoxic signals.Citation115 In tumor tissues, they accumulate in hypoxic areas, possibly due to the specific upregulation of chemoattractants by hypoxia. Thus, after their extravasation into tumors, monocytes may migrate into hypoxic areas by following a hypoxically generated chemoattractant gradient. An alternative hypothesis for the entrapment of TAMs is that they are retained at hypoxic sites after recruitment due to the downregulation of chemokine receptors by TAMs or of chemokines by the cancer cells.Citation116
Neutrophils can also act either as pro-tumorigenic or tumoricidal cells, and TGF-β may play a crucial role in this respect.Citation117 Neutrophils are frequently present at sites of breast tumorigenesis and secrete substances that can modify tumor growth and invasiveness, including ROS and proteinases. Novel therapies to inhibit cancer progression could result from the identification of neutrophil-derived substances that promote tumor growth. Alternatively, highly activated and cytotoxic neutrophils could be generated within the tumor microenvironment in order to unleash the tumoricidal potential of the innate host immune response. Natural killer cells are the only immune cells that cannot act as tumor-promoting cells.
The cytokine and chemokine gradient may be more important than the immune cell content for tumorigenesis. Cytokines control the immune and inflammatory milieu to either favor antitumor immunity or enhance tumor progression, and they also have direct effects on cancer cell growth and survival.
Recent studies showed that metastasis is orchestrated by a close collaboration among cancer, inflammatory, and stromal cells. For instance, Snail, which regulates EMT by repressing E-cadherin transcription,Citation118 is stabilized in response to TNF- α signaling.
Expression of matrix metalloproteinases (MMPs)
Degradation of the ECM, allowing tumor cells to invade local tissue, is essential for the development of distant metastases and is primarily influenced by the activity of tumor-secreted proteinases. Four main classes of proteinases have been described: serine proteinases, aspartatic proteinases, cysteine proteinases, and MMPs. Collectively, they are capable of breaking down all ECM components, and their expression is often associated with a poor prognosis. MMPs are a family of structurally and functionally related endopeptidases that play a crucial role in tumor invasion and metastasis through their ability to degrade ECM proteins; with the exception of MMP-11, they are secreted as inactive zymogens and activated outside the cell by other activated MMPs or serine proteases.Citation119,Citation120 Their activation requires proteolytic removal of the propeptide-domain, enabling access to the catalytic site of the MMPs. Their activity is precisely regulated under physiological conditions to prevent tissue disruption, but variable expression levels of several MMPs have been found in breast cancer patients.Citation121 Furthermore, some of the resulting ECM-protein fragments possess new biological functions; for example, the cleavage of laminin 5 or collagen type IV can promote the migration of different cell types.Citation119,Citation120
Type IV collagen is abundant in the basement membranes that separate epithelial cells from the underlying stroma, and increased expression and activity of MMP-2 and -9 in tumors lead to the degradation of basement membranes, an essential step in tumor invasion.Citation121 Köhrmann classified the expression of these human-specific MMPs as higher, lower, or the same in breast cancer tissue vs. normal breast tissue, finding a higher expression in MMP-1, 2, 8–13, 15, 19, 23, 24, 27 and 28, which may therefore be associated with breast cancer development.121 They found that the four breast cancer cell lines studied constitutively express a wide variety of MMPs at mRNA and protein level and may be candidates for in vitro model systems to study the role of MMPs in breast cancer biology.
It is now known that these MMPs not only degrade physical barriers but also participate in tumor growth, apoptosis, angiogenesis, EMT, invasion, metastasis, and inflammation.Citation122 Yu and Stamenkovic first described the role of MMPs in controlling tumor growth, showing that they altered the balance between growth and anti-growth signals in the tumor microenvironment.Citation123 Tumor cells can take advantage of stromal cell-synthesized TGF-β, which can protect them against the immune system. TGF-β activation is promoted by proteolytic activation of MMP-9, which is commonly secreted by inflammatory cells in the microenvironment, and MMP-2 and -14 are known to activate TGF- β1.Citation122,Citation124
A further role of MMPs is in the promotion of angiogenesis, assisting the formation of new blood vessels. For instance, MMP-9 controls the bioavailability of VEGF needed to generate neovascularization in tumors. MMP-9, conveyed by inflammatory cells, enables an angiogenic switch by making sequestered VEGF bioavailable for its VEGFR-2 receptor in pancreatic islet tumors.Citation122,Citation125 It has been observed that overexpression of MMP-3 is able to drive EMT, the initial event in tumor progression, besides inducing chromosome instability.Citation126
Separation of tumor cells from the tumor and their exit through blood vessels, crucial steps in tumor progression and metastasis, are controlled by MMPs. MMP-3, an overexpressed metalloproteinase in several cancers, can break up cells by disrupting E-cadherin intercellular junctions. It has also been observed that MMP-2 and -9 can degrade the collagen net surrounding the tumor, facilitating the exit of these cells toward other tissues.Citation124 Jeon and Lee recently demonstrated that histone deacetylase inhibition restores the expression of reversion-inducing cysteine-rich protein with Kaza-l motif (RECK tumor suppression protein) under hypoxic conditions, inhibiting cancer cell migration and invasion by repressing MMP-2 and MMP-9 activity.Citation127
It has been shown that some proteolytic fragments of MMP-processed ECM have chemotactic properties. Thus, ECM breakdown during airway inflammation generates the fragment N-acetyl Pro-Gly-Pro (PGP), a tripeptide that exerts chemotactic activity via activation of CXC chemokine receptors on neutrophil granulocytes. There is also strong evidence that MMPs are implicated in TNF-α activation, since conversion of pro-TNF-α into the soluble cytokinetic form requires proteolytic cleavage by ADAM-17 or by various MMPs, including MMP-1, 2, 3, 9, 12, 14, 15, and 17.Citation122
In summary, MMPs play a pivotal role in the tissue remodeling associated with various physiological and pathological processes (e.g., morphogenesis, angiogenesis, tissue repair, chronic inflammation, rapid tumor growth, invasion, and metastasis) by degrading the ECM. MMPs may therefore be considered viable drug targets in cancer treatments. MMPs appear to be important in early cancer progression (local invasion and micrometastasis) and may no longer be required once metastases have been established. Cancer cells can induce stromal cells to synthesize MMPs by using ECM metalloproteinase inducer and cytokine stimulatory mechanisms. MMPs also promote cell migration and the release of growth factors sequestered in the ECM. In fact, MMPs have a dual function in tumor angiogenesis: MMP-2 and MT-1-MMP break down basement membrane barriers in the early stage of angiogenesis, while other MMPs are involved in the generation of angiostatin, an angiogenic inhibitor. Despite recent progress in identifying the multiple roles of MMPs in cancer, our understanding of their function is far from complete. Precise knowledge on their regulation may allow the development of novel therapies for patients with invasive cancer.
Changes in the Microenvironment and Clinical Setting
There are two competing views of tumor-stromal interaction, not necessarily mutually exclusive. On the one hand, researchers have proposed that the stromal reaction to tumor could be due to wound response.Citation128,Citation129 On the other hand, researchers have found evidence that the stroma can play a more active role in the induction or promotion of oncogenesis.Citation36,Citation130-Citation133
Over the past few years, authors have shown that interaction between tumor cells and their microenvironment may determine the clinical behavior, prognosis, and response to specific treatment protocols. Finak first addressed how changes in tumor stroma can affect disease outcome,Citation42 while Bergamaschi classified invasive breast tumors according to their expression of ECM components and suggested that the clinical outcome is strongly related to stromal characteristics.Citation134 Other authors reported a major contribution of stromal genes to drug sensitivity.Citation43
Rajski recently observed that patients with early stage breast cancer and high expression of the breast fibroblast-derived IGF-I signature had a significantly higher risk of developing metastasis and a significantly lower overall survival rate than those with low expression of this signature.Citation135 This author proposed IGF-I-induced gene signatures derived from stromal fibroblasts as promising predictors of the response to IGF-I targeted therapies.
Sharma found a correlation between the type of stromal response in DCIS and IDC within the same patient to both the macrophage response and the fibroblast stromal signatures, offering the first characterization of these signatures in DCIS.Citation136 These signatures have significant clinicopathological associations and tend to be conserved as the tumor progresses from DCIS to invasive breast cancer. This author suggested a classification of DCIS based on the expression of groups of functionally related genes involved in the stromal response. They also advocated that stroma-targeted therapies could treat pre-invasive disease. These findings improve the possibility of identifying tumors that will respond to a specific stroma-targeted therapy that might be developed in the tumor microenvironment field.
Finally, given the above-reported evidence on the important role of the microenvironment of the tumor in its development, progression, and response to therapy, there is a need to combine the efforts of researchers to create more informative experimental models for dissecting the roles of microenvironment components. This is essential to understand the consequences of changes in this tissue compartment in relation to breast cancer outcome. This would allow us to develop and test optimal intervention strategies for the treatment of cancer patients and to suggest future directions for the development of novel diagnostic or therapeutic modalities.
Acknowledgments
This study was supported by the Instituto de Salud Carlos III, PI 080728 to M.I.N. S.R. is supported by the Fundación Benéfica San Francisco Javier y Santa Cándida, University of Granada. A grant from the Spanish Ministry of Science and Education to F.A. greatly aided this work. This research was also funded by the San Cecilio University Hospital, Granada. The authors thank Richard Davies for improving the English style of the manuscript. We are also indebted to Juan Jesus Priego Córdoba for his assistance in designing the figure.
References
- Patocs A, Zhang L, Xu Y, Weber F, Caldes T, Mutter GL, et al. Breast-cancer stromal cells with TP53 mutations and nodal metastases. N Engl J Med 2007; 357:2543 - 51; http://dx.doi.org/10.1056/NEJMoa071825; PMID: 18094375
- Hu M, Yao J, Cai L, Bachman KE, van den Brûle F, Velculescu V, et al. Distinct epigenetic changes in the stromal cells of breast cancers. Nat Genet 2005; 37:899 - 905; http://dx.doi.org/10.1038/ng1596; PMID: 16007089
- Fiegl H, Millinger S, Goebel G, Müller-Holzner E, Marth C, Laird PW, et al. Breast cancer DNA methylation profiles in cancer cells and tumor stroma: association with HER-2/neu status in primary breast cancer. Cancer Res 2006; 66:29 - 33; http://dx.doi.org/10.1158/0008-5472.CAN-05-2508; PMID: 16397211
- Shekhar MP, Pauley R, Heppner G. Host microenvironment in breast cancer development: Extracellular matrix-stromal cell contribution to neoplastic phenotype of epithelial cells in the breast. Breast Cancer Res 2003; 5:130 - 5; http://dx.doi.org/10.1186/bcr580; PMID: 12793893
- Bissell MJ, Hall HG, Parry G. How does the extracellular matrix direct gene expression?. J Theor Biol 1982; 99:31 - 68; http://dx.doi.org/10.1016/0022-5193(82)90388-5; PMID: 6892044
- Bissell MJ, Ram TG. Regulation of functional cytodifferentiation and histogenesis in mammary epithelial cells: role of the extracellular matrix. Environ Health Perspect 1989; 80:61 - 70; http://dx.doi.org/10.1289/ehp.898061; PMID: 2647485
- Bissell MJ, Barcellos-Hoff MH. The influence of extracellularmatrix on gene expression: is structure the message?. J Cell Sci Suppl 1987; 8:327 - 43; PMID: 3332665
- Gehler S, Baldassarre M, Lad Y, Leight JL, Wozniak MA, Riching KM, et al. Filamin A–b1 integrin complex tunes epithelial cell response to matrix tension. Mol Biol Cell 2009; 20:3224 - 38; http://dx.doi.org/10.1091/mbc.E08-12-1186; PMID: 19458194
- Dvorak HF, Weaver VM, Tlsty T, Bergers G. Tumor microenvironment and progression. J Surg Oncol 2011; 103:468 - 74; http://dx.doi.org/10.1002/jso.21709; PMID: 21480238
- Maffini MV, Calabro JM, Soto AM, Sonnenschein C. Stromal regulation of neoplastic development: age-dependent normalization of neoplastic mammary cells by mammary stroma. Am J Pathol 2005; 167:1405 - 10; http://dx.doi.org/10.1016/S0002-9440(10)61227-8; PMID: 16251424
- Bussard KM, Boulanger CA, Booth BW, Bruno RD, Smith GH. Reprogramminghuman cancer cells in the mouse mammary gland. Cancer Res 2010; 70:6336 - 43; http://dx.doi.org/10.1158/0008-5472.CAN-10-0591; PMID: 20647316
- Maffini MV, Soto AM, Calabro JM, Ucci AA, Sonnenschein C. The stroma as a crucial target in ratmammary gland carcinogenesis. J Cell Sci 2004; 117:1495 - 502; http://dx.doi.org/10.1242/jcs.01000; PMID: 14996910
- Hanahan D, Weinberg RA. The hallmarks of cancer. Cell 2000; 100:57 - 70; http://dx.doi.org/10.1016/S0092-8674(00)81683-9; PMID: 10647931
- Colotta F, Allavena P, Sica A, Garlanda C, Mantovani A. Cancer-related inflammation, the seventh hallmark of cancer: links to genetic instability. Carcinogenesis 2009; 30:1073 - 81; http://dx.doi.org/10.1093/carcin/bgp127; PMID: 19468060
- Polyak K. Breast cancer: origins and evolution. J Clin Invest 2007; 117:3155 - 63; http://dx.doi.org/10.1172/JCI33295; PMID: 17975657
- Wicha MS, Liu S, Dontu G. Cancer stem cells: an old idea–a paradigm shift. Cancer Res 2006; 66:1883 - 90; http://dx.doi.org/10.1158/0008-5472.CAN-05-3153; PMID: 16488983
- Sgroi DC. Preinvasive breast cancer. Annu Rev Pathol 2010; 5:193 - 221; http://dx.doi.org/10.1146/annurev.pathol.4.110807.092306; PMID: 19824828
- Hunter KW. Host genetics and tumour metastasis. Br J Cancer 2004; 90:752 - 5; http://dx.doi.org/10.1038/sj.bjc.6601590; PMID: 14970848
- Weber F, Shen L, Fukino K, Patocs A, Mutter G, Caldés T, et al. Total-genome analysis of BRCA1/2-related invasive carcinomas of the breast identifies tumor stroma as potential landscaper for neoplastic initiation. Am J Hum Genet 2006; 78:961 - 72; http://dx.doi.org/10.1086/504090; PMID: 16685647
- Allred DC, Mohsin SK, Fuqua SA. Histological and biological evolution of human premalignant breast disease. Endocr Relat Cancer 2001; 8:47 - 61; http://dx.doi.org/10.1677/erc.0.0080047; PMID: 11350726
- Orimo A, Weinberg RA. Stromal fibroblasts in cancer: a novel tumor-promoting cell type. Cell Cycle 2006; 5:1597 - 601; http://dx.doi.org/10.4161/cc.5.15.3112; PMID: 16880743
- Polyak K, Haviv I, Campbell IG. Co-evolution of tumor cells and their microenvironment. Trends Genet 2009; 25:30 - 8; http://dx.doi.org/10.1016/j.tig.2008.10.012; PMID: 19054589
- Hu M, Polyak K. Microenvironmental regulation of cancer development. Curr Opin Genet Dev 2008; 18:27 - 34; http://dx.doi.org/10.1016/j.gde.2007.12.006; PMID: 18282701
- Nelson CM, Bissell MJ. Of extracellular matrix, scaffolds, and signaling: tissue architecture regulates development, homeostasis, and cancer. Annu Rev Cell Dev Biol 2006; 22:287 - 309; http://dx.doi.org/10.1146/annurev.cellbio.22.010305.104315; PMID: 16824016
- Chin K, de Solorzano CO, Knowles D, Jones A, Chou W, Rodriguez EG, et al. In situ analyses of genome instability in breast cancer. Nat Genet 2004; 36:984 - 8; http://dx.doi.org/10.1038/ng1409; PMID: 15300252
- Maruyama R, Choudhury S, Kowalczyk A, Bessarabova M, Beresford-Smith B, Conway T, et al. Epigenetic regulation of cell type-specific expression patterns in the human mammary epithelium. PLoS Genet 2011; 7:e1001369; http://dx.doi.org/10.1371/journal.pgen.1001369; PMID: 21533021
- Kurose K, Gilley K, Matsumoto S, Watson PH, Zhou XP, Eng C. Frequent somatic mutations in PTEN and TP53 are mutually exclusive in the stroma of breast carcinomas. Nat Genet 2002; 32:355 - 7; http://dx.doi.org/10.1038/ng1013; PMID: 12379854
- Kurose K, Hoshaw-Woodard S, Adeyinka A, Lemeshow S, Watson PH. Genetic model of multi-step breast carcinogenesis involving the epithelium and stroma: clues to tumour-microenvironment interactions. Hum Mol Genet 2001; 10:1907 - 13; http://dx.doi.org/10.1093/hmg/10.18.1907; PMID: 11555627
- Moinfar F, Man YG, Arnould L, Bratthauer GL, Ratschek M, Tavassoli FA. Concurrent and independent genetic alterations in the stromal and epithelial cells of mammary carcinoma: implications for tumorigenesis. Cancer Res 2000; 60:2562 - 6; PMID: 10811140
- Qiu W, Hu M, Sridhar A, Opeskin K, Fox S, Shipitsin M, et al. No evidence of clonal somatic genetic alterations in cancer-associated fibroblasts from human breast and ovarian carcinomas. Nat Genet 2008; 40:650 - 5; http://dx.doi.org/10.1038/ng.117; PMID: 18408720
- Fukino K, Shen L, Matsumoto S, Morrison CD, Mutter GL, Eng C. Combined Total Genome Loss of Heterozygosity Scan of Breast Cancer Stroma and Epithelium Reveals Multiplicity of Stromal Targets. Cancer Res 2004; 64:7231 - 6; http://dx.doi.org/10.1158/0008-5472.CAN-04-2866; PMID: 15492239
- Fukino K, Shen L, Patocs A, Mutter GL, Eng C. Genomic instability within tumor stroma and clinicopathological characteristics of sporadic primary invasive breast carcinoma. JAMA 2007; 297:2103 - 11; http://dx.doi.org/10.1001/jama.297.19.2103; PMID: 17507346
- Weber F, Fukino K, Sawada T, Williams N, Sweet K, Brena RM, et al. Variability in organ-specific EGFR mutational spectra in tumour epithelium and stroma may be the biological basis for differential responses to tyrosine kinase inhibitors. Br J Cancer 2005; 92:1922 - 6; http://dx.doi.org/10.1038/sj.bjc.6602557; PMID: 15841079
- Weber F, Shen L, Fukino K, Patocs A, Mutter GL, Caldes T, et al. Total-genome analysis of BRCA1/2-related invasive carcinomas of the breast identifies tumor stroma as potential landscaper for neoplastic initiation. Am J Hum Genet 2006; 78:961 - 72; http://dx.doi.org/10.1086/504090; PMID: 16685647
- Hu M, Yao J, Carroll DK, Weremowicz S, Chen H, Carrasco D, et al. Regulation of in situ to invasive breast carcinoma transition. Cancer Cell 2008; 13:394 - 406; http://dx.doi.org/10.1016/j.ccr.2008.03.007; PMID: 18455123
- Cunha GR, Hayward SW, Wang YZ. Role of stroma in carcinogenesis of the prostate. Differentiation 2002; 70:473 - 85; http://dx.doi.org/10.1046/j.1432-0436.2002.700902.x; PMID: 12492490
- Barcellos-Hoff MH. The potential influence of radiation-induced microenvironments in neoplastic progression. J Mammary Gland Biol Neoplasia 1998; 3:165 - 75; http://dx.doi.org/10.1023/A:1018794806635; PMID: 10819525
- Nguyen DH, Oketch-Rabah HA, Illa-Bochaca I, Geyer FC, Reis-Filho JS, Mao JH, et al. Radiation acts on the microenvironment to affect breast carcinogenesis by distinct mechanisms that decrease cancer latency and affect tumor type. Cancer Cell 2011; 19:640 - 51; http://dx.doi.org/10.1016/j.ccr.2011.03.011; PMID: 21575864
- Allinen M, Beroukhim R, Cai L, Brennan C, Lahti-Domenici J, Huang H, et al. Molecular characterization of the tumor microenvironment in breast cancer. Cancer Cell 2004; 6:17 - 32; http://dx.doi.org/10.1016/j.ccr.2004.06.010; PMID: 15261139
- Ma XJ, Dahiya S, Richardson E, Erlander M, Sgroi D. Gene expression profiling of the tumor microenvironment during breast cancer progression. Breast Cancer Res 2009; 11:R7; http://dx.doi.org/10.1186/bcr2222; PMID: 19187537
- Ma XJ, Salunga R, Tuggle JT, Gaudet J, Enright EE, McQuary P, et al. Gene expression profiles of human breast cancer progression. Proc Natl Acad Sci USA 2003; 100:5974 - 9; http://dx.doi.org/10.1073/pnas.0931261100; PMID: 12714683
- Finak G, Bertos N, Pepin F, Sadekova S, Souleimanova M, Zhao H, et al. Stromal gene expression predicts clinical outcome in breast cancer. Nat Med 2008; 14:518 - 27; http://dx.doi.org/10.1038/nm1764; PMID: 18438415
- Farmer P, Bonnefoi H, Anderle P, Cameron D, Wirapati P, Becette V, et al. A stroma-related gene signature predicts resistance to neoadjuvant chemotherapy in breast cancer. Nat Med 2009; 15:68 - 74; http://dx.doi.org/10.1038/nm.1908; PMID: 19122658
- Lerwill MF. Current practical applications of diagnostic immunohistochemistry in breast pathology. Am J Surg Pathol 2004; 28:1076 - 91; http://dx.doi.org/10.1097/01.pas.0000126780.10029.f0; PMID: 15252316
- Schäfer M, Werner S. Cancer as an overhealing wound: an old hypothesis revisited. Nat Rev Mol Cell Biol 2008; 9:628 - 38; http://dx.doi.org/10.1038/nrm2455; PMID: 18628784
- Hunter KW, Crawford N, Alsarraj J. Mechanisms of metastasis. Breast Cancer Res 2008; 10:S2; http://dx.doi.org/10.1186/bcr1988; PMID: 19091006
- Al-Mehdi AB, Tozawa K, Fisher AB, Shientag L, Lee A, Muschel RJ. Intravascular origin of metastasis from the proliferation of endothelium-attached tumor cells: a new model for metastasis. Nat Med 2000; 6:100 - 2; http://dx.doi.org/10.1038/71429; PMID: 10613833
- Hoda SA, Hoda RS, Merlin S, Shamonki J, Rivera M. Issues relating to lymphovascular invasion in breast carcinoma. Adv Anat Pathol 2006; 13:308 - 15; http://dx.doi.org/10.1097/01.pap.0000213048.69564.26; PMID: 17075296
- Gajdos C, Tartter PI, Bleiweiss IJ. Lymphatic invasion, tumor size, and age are independent predictors of axillary lymph node metastases in women with T1 breast cancers. Ann Surg 1999; 230:692 - 6; http://dx.doi.org/10.1097/00000658-199911000-00012; PMID: 10561094
- Weiser MR, Montgomery LL, Tan LK, Susnik B, Leung DY, Borgen PI, et al. Lymphovascular invasion enhances the prediction of non-sentinel node metastases in breast cancer patients with positive sentinel nodes. Ann Surg Oncol 2001; 8:145 - 9; http://dx.doi.org/10.1007/s10434-001-0145-y; PMID: 11258779
- Schoppmann SF, Bayer G, Aumayr K, Taucher S, Geleff S, Rudas M, et al. Prognostic value of lymphangiogenesis and lymphovascular invasion in invasive breast cancer. Ann Surg 2004; 240:306 - 12; http://dx.doi.org/10.1097/01.sla.0000133355.48672.22; PMID: 15273556
- Woo CS, Silberman H, Nakamura SK, Ye W, Sposto R, Colburn W, et al. Lymph node status combined with lymphovascular invasion creates a more powerful tool for predicting outcome in patients with invasive breast cancer. Am J Surg 2002; 184:337 - 40; http://dx.doi.org/10.1016/S0002-9610(02)00950-9; PMID: 12383896
- Fisher ER, Anderson S, Tan-Chiu E, Fisher B, Eaton L, Wolmark N. Fifteen-year prognostic discriminants for invasive breast carcinoma: National Surgical Adjuvant Breast and Bowel Project Protocol-06. Cancer 2001; 91:1679 - 87; http://dx.doi.org/10.1002/1097-0142(20010415)91:8+<1679::AID-CNCR1183>3.3.CO;2-#; PMID: 11309768
- El-Gohary YM, Metwally G, Saad RS, Robinson MJ, Mesko T, Poppiti RJ. Prognostic significance of intratumoral and peritumoral lymphatic density and blood vessel density in invasive breast carcinomas. Am J Clin Pathol 2008; 129:578 - 86; http://dx.doi.org/10.1309/2HGNJ1GU57JMBJAQ; PMID: 18343785
- Ejlertsen B, Jensen MB, Rank F, Rasmussen BB, Christiansen P, Kroman N, et al. Population-based study of peritumoral lymphovascular invasion and outcome among patients with operable breast cancer. J Natl Cancer Inst 2009; 101:729 - 35; http://dx.doi.org/10.1093/jnci/djp090; PMID: 19436035
- Goetz JG, Minguet S, Navarro-Lérida I, Lazcano JJ, Samaniego R, Calvo E, et al. Biomechanical Remodeling of the Microenvironment by Stromal Caveolin-1Favors Tumor Invasion and metastasis. Cell 2011; 146:148 - 63; http://dx.doi.org/10.1016/j.cell.2011.05.040; PMID: 21729786
- Kaplan RN, Riba RD, Zacharoulis S, Bramley AH, Vincent L, Costa C, et al. VEGFR1-positive haematopoietic bone marrow progenitors initiate the pre-metastatic niche. Nature 2005; 438:820 - 7; http://dx.doi.org/10.1038/nature04186; PMID: 16341007
- Schedin P, Elias A. Multistep tumorigenesis and the microenvironment. Breast Cancer Res 2004; 6:93 - 101; http://dx.doi.org/10.1186/bcr772; PMID: 14979914
- Jaenisch R, Bird A. Epigenetic regulation of gene expression: how the genome integrates intrinsic and environmental signals. Nat Genet 2003; 33:Suppl 245 - 54; http://dx.doi.org/10.1038/ng1089; PMID: 12610534
- Lin HJL, Zuo T, Lin CH, Kuo CT, Liyanarachchi S, Sun S, et al. Breast cancer-associated fibroblasts confer AKT1-mediated epigenetic silencing of Cystatin M in epithelial cells. Cancer Res 2008; 68:10257 - 66; http://dx.doi.org/10.1158/0008-5472.CAN-08-0288; PMID: 19074894
- Soares J, Pinto AE, Cunha CV, André S, Barão I, Sousa JM, et al. Global DNA hypomethylation in breast carcinoma: correlation with prognostic factors and tumor progression. Cancer 1999; 85:112 - 8; http://dx.doi.org/10.1002/(SICI)1097-0142(19990101)85:1<112::AID-CNCR16>3.3.CO;2-K; PMID: 9921982
- Giacinti L, Claudio PP, Lopez M, Giordano A. Epigenetic information and estrogen receptor alpha expression in breast cancer. Oncologist 2006; 11:1 - 8; http://dx.doi.org/10.1634/theoncologist.11-1-1; PMID: 16401708
- Baylin SB. DNA methylation and gene silencing in cancer. Nat Clin Pract Oncol 2005; 2:Suppl 1 S4 - 11; http://dx.doi.org/10.1038/ncponc0354; PMID: 16341240
- Jones PA, Baylin SB. The fundamental role of epigenetic events in cancer. Nat Rev Genet 2002; 3:415 - 28; PMID: 12042769
- Esteller M, Silva JM, Dominguez G, Bonilla F, Matias-Guiu X, Lerma E, et al. Promoter hypermethylation and BRCA1 inactivation in sporadic breast and ovarian tumors. J Natl Cancer Inst 2000; 92:564 - 9; http://dx.doi.org/10.1093/jnci/92.7.564; PMID: 10749912
- Plachot C, Lelièvre SA. DNA methylation control of tissue polarity and cellular differentiation in the mammary epithelium. Exp Cell Res 2004; 298:122 - 32; http://dx.doi.org/10.1016/j.yexcr.2004.04.024; PMID: 15242767
- Bissell MJ, Radisky D. Putting tumours in context. Nat Rev Cancer 2001; 1:46 - 54; http://dx.doi.org/10.1038/35094059; PMID: 11900251
- Dumont N, Wilson MB, Crawford YG, Reynolds PA, Sigaroudinia M, Tlsty TD. Sustained induction of epithelial to mesenchymal transition activates DNA methylation of genes silenced in basal-like breast cancers. Proc Natl Acad Sci USA 2008; 105:14867 - 72; http://dx.doi.org/10.1073/pnas.0807146105; PMID: 18806226
- Hu M, Yao J, Cai L, Bachman KE, van den Brule F, Velculescu V, Polyak K, et al. Distinct epigenetic changes in the stromal cells of breast cancers. Nat Genet 2005; 37:899 - 905; http://dx.doi.org/10.1038/ng1596; PMID: 16007089
- Fiegl H, Millinger S, Goebel G, Muller-Holzner E, Marth C, Laird PW, et al. Breast Cancer DNA Methylation Profiles in Cancer Cells and Tumor Stroma: Association with HER-2/neu Status in Primary Breast Cancer. Cancer Res 2006; 66:29 - 33; http://dx.doi.org/10.1158/0008-5472.CAN-05-2508; PMID: 16397211
- Ruan K, Fang X, Ouyang G. MicroRNAs: Novel regulators in the hallmarks of human cancer. Cancer Lett 2009; 285:116 - 26; http://dx.doi.org/10.1016/j.canlet.2009.04.031; PMID: 19464788
- Bartel DP. MicroRNAs: genomics, biogenesis, mechanism, and function. Cell 2004; 116:281 - 97; http://dx.doi.org/10.1016/S0092-8674(04)00045-5; PMID: 14744438
- He L, Hannon GJ. MicroRNAs: small RNAs with a big role in gene regulation. Nat Rev Genet 2004; 5:522 - 31; http://dx.doi.org/10.1038/nrg1379; PMID: 15211354
- Calin GA, Sevignani C, Dumitru CD, Hyslop T, Noch E, Yendamuri S, et al. Human microRNA genes are frequently located at fragile sites and genomic regions involved in cancers. Proc Natl Acad Sci USA 2004; 101:2999 - 3004; http://dx.doi.org/10.1073/pnas.0307323101; PMID: 14973191
- Iorio MV, Ferracin M, Liu CG, Veronese A, Spizzo R, Sabbioni S, et al. MicroRNA gene expression deregulation in human breast cancer. Cancer Res 2005; 65:7065 - 70; http://dx.doi.org/10.1158/0008-5472.CAN-05-1783; PMID: 16103053
- Dalmay T, Edwards DR. MicroRNAs and the hallmarks of cancer. Oncogene 2006; 25:6170 - 5; http://dx.doi.org/10.1038/sj.onc.1209911; PMID: 17028596
- Lujambio A, Ropero S, Ballestar E, Fraga MF, Cerrato C, Setién F, et al. Genetic unmasking of an epigenetically silenced microRNA in human cancer cells. Cancer Res 2007; 67:1424 - 9; http://dx.doi.org/10.1158/0008-5472.CAN-06-4218; PMID: 17308079
- Lehmann U, Hasemeier B, Christgen M, Müller M, Römermann D, Länger F, et al. Epigenetic inactivation of microRNA gene hsa-mir-9-1 in human breast cancer. J Pathol 2008; 214:17 - 24; http://dx.doi.org/10.1002/path.2251; PMID: 17948228
- Corcoran KE, Rameshwar P. Nuclear factor-kappaB accounts for the repressor effects of high stromal cell-derived factor-1alpha levels on Tac1 expression in nontumorigenic breast cells. Mol Cancer Res 2007; 5:373 - 81; http://dx.doi.org/10.1158/1541-7786.MCR-06-0396; PMID: 17409218
- Patel SA, Heinrich AC, Reddy BY, Srinivas B, Heidaran N, Rameshwar P. Breast cancer biology: the multifaceted roles of mesenchymal stem cells. J Oncol 2008; 425895; PMID: 19277104
- Hitchler MJ, Oberley LW, Domann FE. Epigenetic silencing of SOD2 by histone modifications in human breast cancer cells. Free Radic Biol Med 2008; 45:1573 - 80; http://dx.doi.org/10.1016/j.freeradbiomed.2008.09.005; PMID: 18845242
- Strahl BD, Allis CD. The language of covalent histone modifications. Nature 2000; 403:41 - 5; http://dx.doi.org/10.1038/47412; PMID: 10638745
- Sandal T, Valyi-Nagy K, Spencer VA, Folberg R, Bissell MJ, Maniotis AJ. Epigenetic reversion of breast carcinoma phenotype is accompanied by changes in DNA sequestration as measured by AluI restriction enzyme. Am J Pathol 2007; 170:1739 - 49; http://dx.doi.org/10.2353/ajpath.2007.060922; PMID: 17456778
- Florin L, Maas-Szabowski N, Werner S, Szabowski A, Angel P. Increased keratinocyte proliferation by JUN-dependent expression of PTN and SDF-1 in fibroblasts. J Cell Sci 2005; 118:1981 - 9; http://dx.doi.org/10.1242/jcs.02303; PMID: 15840658
- Werner S, Grose R. Regulation of wound healing by growth factors and cytokines. Physiol Rev 2003; 83:835 - 70; PMID: 12843410
- Sibilia M, Kroismayr R, Lichtenberger BM, Natarajan A, Hecking M, Holcmann M. The epidermal growth factor receptor: from development to tumorigenesis. Differentiation 2007; 75:770 - 87; http://dx.doi.org/10.1111/j.1432-0436.2007.00238.x; PMID: 17999740
- Wakefield LM, Roberts AB. TGF-beta signaling: positive and negative effects on tumorigenesis. Curr Opin Genet Dev 2002; 12:22 - 9; http://dx.doi.org/10.1016/S0959-437X(01)00259-3; PMID: 11790550
- Yu JL, Rak J. Host microenvironment in breast cancer development: Inflammatory and immune cells in tumour angiogenesis and arteriogenesis. Breast Cancer Res 2003; 5:83 - 8; http://dx.doi.org/10.1186/bcr573; PMID: 12631386
- Lin EY, Pollard JW. Tumor-associated macrophages press the angiogenic switch in breast cancer. Cancer Res 2007; 67:5064 - 6; http://dx.doi.org/10.1158/0008-5472.CAN-07-0912; PMID: 17545580
- Lewis CE, Hughes R. Inflammation and breast cancer. Microenvironmental factors regulating macrophage function in breast tumours: hypoxia and angiopoietin-2. Breast Cancer Res 2007; 9:209; http://dx.doi.org/10.1186/bcr1679; PMID: 17601353
- Ben-Baruch A. Inflammatory cells, cytokines and chemokines in breast cancer progression: reciprocal tumor-microenvironment interactions. . Breast Cancer Res 2003; 5:31 - 36; http://dx.doi.org/10.1186/bcr554; PMID: 12559043
- Coussens LM, Werb Z. Inflammation and cancer. Nature 2002; 420:860 - 7; http://dx.doi.org/10.1038/nature01322; PMID: 12490959
- Klaunig JE, Kamendulis LM. The role of oxidative stress in carcinogenesis. Annu Rev Pharmacol Toxicol 2004; 44:239 - 67; http://dx.doi.org/10.1146/annurev.pharmtox.44.101802.121851; PMID: 14744246
- Valko M, Rhodes CJ, Moncol J, Izakovic M, Mazur M. Free radicals, metals and antioxidants in oxidative stress-induced cancer. Chem Biol Interact 2006; 160:1 - 40; http://dx.doi.org/10.1016/j.cbi.2005.12.009; PMID: 16430879
- Roberts RA, Laskin DL, Smith CV, Robertson FM, Allen EMG, Doorn JA, et al. Nitrative and oxidative stress in toxicology and disease. Toxicol Sci 2009; 112:4 - 16; http://dx.doi.org/10.1093/toxsci/kfp179; PMID: 19656995
- Curtis CD, Thorngren DL, Nardulli AM. Immunohistochemical analysis of oxidative stress and DNA repair proteins in normal mammary and breast cancer tissue. BMC Cancer 2010; 10:9; http://dx.doi.org/10.1186/1471-2407-10-9; PMID: 20064251
- Schultz-Norton JR, McDonald WH, Yates JR, Nardulli AM. Protein disulfide isomerase serves as a molecular chaperone to maintain estrogen receptor alpha structure and function. Mol Endocrinol 2006; 20:1982 - 95; http://dx.doi.org/10.1210/me.2006-0006; PMID: 16690750
- Rao AK, Ziegler YS, McLeod IX, Yates JR, Nardulli AM. Effects of Cu/Zn superoxide dismutase on estrogen responsiveness and oxidative stress in human breast cancer cells. Mol Endocrinol 2008; 22:1113 - 24; http://dx.doi.org/10.1210/me.2007-0381; PMID: 18258688
- Rao AK, Ziegler YS, McLeod IX, Yates JR, Nardulli AM. Thioredoxin and thioredoxin reductase influence estrogen receptor alpha-mediated gene expression in human breast cancer cells. J Mol Endocrinol 2009; 43:251 - 61; http://dx.doi.org/10.1677/JME-09-0053; PMID: 19620238
- Curtis CD, Thorngren DL, Ziegler YS, Sarkeshik A, Yates JR, Nardulli AM. Apurinic/apyrimidinic endonuclease 1 alters estrogen receptor activity and estrogen-responsive gene expression. Mol Endocrinol 2009; 23:1346 - 59; http://dx.doi.org/10.1210/me.2009-0093; PMID: 19460860
- Hanahan D, Folkman J. Patterns and emerging mechanisms of the angiogenic switch during tumorigenesis. Cell 1996; 86:353 - 64; http://dx.doi.org/10.1016/S0092-8674(00)80108-7; PMID: 8756718
- Shibuya M. Differential roles of vascular endothelial growth factor receptor-1 and receptor-2 in angiogenesis. J Biochem Mol Biol 2006; 39:469 - 78; http://dx.doi.org/10.5483/BMBRep.2006.39.5.469; PMID: 17002866
- Augustin HG, Koh GY, Thurston G, Alitalo K. Control of vascular morphogenesis and homeostasis through the angiopoietin-Tie system. Nat Rev Mol Cell Biol 2009; 10:165 - 77; http://dx.doi.org/10.1038/nrm2639; PMID: 19234476
- Lohela M, Bry M, Tammela T, Alitalo K. VEGFs and receptors involved in angiogenesis versus lymphangiogenesis. Curr Opin Cell Biol 2009; 21:154 - 65; http://dx.doi.org/10.1016/j.ceb.2008.12.012; PMID: 19230644
- Saharinen P, Bry M, Alitalo K. How do angiopoietins Tie in with vascular endothelial growth factors?. Curr Opin Hematol 2010; 17:198 - 205; PMID: 20375888
- Bergers G, Benjamin LE. Tumorigenesis and the angiogenic switch. Nat Rev Cancer 2003; 3:401 - 10; http://dx.doi.org/10.1038/nrc1093; PMID: 12778130
- Acevedo VD, Ittmann M, Spencer DM. Paths of FGFR-driven tumorigenesis. Cell Cycle 2009; 8:580 - 8; http://dx.doi.org/10.4161/cc.8.4.7657; PMID: 19182515
- Ciruna B, Rossant J. FGF Signaling Regulates Mesoderm Cell Fate Specification and Morphogenetic Movement at the Primitive Streak. Dev Cell 2001; 1:37 - 49; http://dx.doi.org/10.1016/S1534-5807(01)00017-X; PMID: 11703922
- Mezquita B, Mezquita J, Pau M, Mezquita C. A novel intracellular isoform of VEGFR-1 activates Src and promotes cell invasion in MDA-MB-231 breast cancer cells. J Cell Biochem 2010; 110:732 - 42; http://dx.doi.org/10.1002/jcb.22584; PMID: 20512933
- Mantovani A, Allavena P, Sica A, Balkwill F. Cancer-related inflammation. Nature 2008; 454:436 - 44; http://dx.doi.org/10.1038/nature07205; PMID: 18650914
- Soucek L, Lawlor ER, Soto D, Shchors K, Swigart LB, Evan GI. Mast cells are required for angiogenesis and macroscopic expansion of Myc-induced pancreatic islet tumors. Nat Med 2007; 13:1211 - 8; http://dx.doi.org/10.1038/nm1649; PMID: 17906636
- Sparmann A, Bar-Sagi D. Ras-induced interleukin-8 expression plays a critical role in tumor growth and angiogenesis. Cancer Cell 2004; 6:447 - 58; http://dx.doi.org/10.1016/j.ccr.2004.09.028; PMID: 15542429
- Vakkila J, Lotze MT. Inflammation and necrosis promote tumour growth. Nat Rev Immunol 2004; 4:641 - 8; http://dx.doi.org/10.1038/nri1415; PMID: 15286730
- Zong WX, Thompson CB. Necrotic death as a cell fate. Genes Dev 2006; 20:1 - 15; http://dx.doi.org/10.1101/gad.1376506; PMID: 16391229
- Grivennikov SI, Greten FR, Karin M. Immunity, inflammation, and cancer. Cell 2010; 140:883 - 99; http://dx.doi.org/10.1016/j.cell.2010.01.025; PMID: 20303878
- Murdoch C, Giannoudis A, Lewis CE. Mechanisms regulating the recruitment of macrophages into hypoxic areas of tumors and other ischemic tissues. Blood 2004; 104:2224 - 34; http://dx.doi.org/10.1182/blood-2004-03-1109; PMID: 15231578
- Fridlender ZG, Sun J, Kim S, Kapoor V, Cheng G, Ling L, et al. Polarization of tumor-associated neutrophil phenotype by TGF-beta: “N1” versus “N2” TAN. Cancer Cell 2009; 16:183 - 94; http://dx.doi.org/10.1016/j.ccr.2009.06.017; PMID: 19732719
- Moreno-Bueno G, Portillo F, Cano A. Transcriptional regulation of cell polarity in EMT and cancer. Oncogene 2008; 27:6958 - 69; http://dx.doi.org/10.1038/onc.2008.346; PMID: 19029937
- Page-McCaw A, Ewald AJ, Werb Z. Matrix metalloproteinases and the regulation of tissue remodelling. Nat Rev Mol Cell Biol 2007; 8:221 - 33; http://dx.doi.org/10.1038/nrm2125; PMID: 17318226
- Nagase H, Visse R, Murphy G. Structure and function of matrix metalloproteinases and TIMPs. Cardiovasc Res 2006; 69:562 - 73; http://dx.doi.org/10.1016/j.cardiores.2005.12.002; PMID: 16405877
- Köhrmann A, Kammerer U, Kapp M, Dietl J, Anacker J. Expression of matrix metalloproteinases (MMPs) in primary human breast cancer and breast cancer cell lines: New findings and review of the literature. BMC Cancer 2009; 9:188; http://dx.doi.org/10.1186/1471-2407-9-188; PMID: 19531263
- Kessenbrock K, Plaks V, Werb Z. Matrix metalloproteinases: regulators of the tumor microenvironment. Cell 2010; 141:52 - 67; http://dx.doi.org/10.1016/j.cell.2010.03.015; PMID: 20371345
- Yu Q, Stamenkovic I. Cell surface-localized matrix metalloproteinase-9 proteolytically activates TGF-beta and promotes tumor invasion and angiogenesis. Genes Dev 2000; 14:163 - 76; PMID: 10652271
- Li JR, Li MQ, Bao JT, Li JZ. Correlation between expression of metastasis-associated gene 1 and matrix metalloproteinase 9 and invasion and metastasis of breast cancer. Zhonghua Yi Xue Za Zhi 2008; 88:2278 - 80; PMID: 19087679
- Bergers G, Brekken R, McMahon G, Vu TH, Itoh T, Tamaki K, et al. Matrix metalloproteinase-9 triggers the angiogenic switch during carcinogenesis. Nat Cell Biol 2000; 2:737 - 44; http://dx.doi.org/10.1038/35036374; PMID: 11025665
- Radisky DC, Levy DD, Littlepage LE, Liu H, Nelson CM, Fata JE, et al. Rac1b and reactive oxygen species mediate MMP-3-induced EMT and genomic instability. Nature 2005; 436:123 - 7; http://dx.doi.org/10.1038/nature03688; PMID: 16001073
- Jeon HW, Lee YM. Inhibition of histone deacetylase attenuates hypoxia-induced migration and invasion of cancer cells via the restoration of RECK expression. Mol Cancer Ther 2010; 9:1361 - 70; http://dx.doi.org/10.1158/1535-7163.MCT-09-0717; PMID: 20442303
- Chang HY, Sneddon JB, Alizadeh AA, Sood R, West RB, Montgomery K, et al. Gene expression signature of fibroblast serum response predicts human cancer progression: similarities between tumors and wounds. PLoS Biol 2004; 2:E7; http://dx.doi.org/10.1371/journal.pbio.0020007; PMID: 14737219
- Dvorak HF. Tumors: wounds that do not heal. Similarities between tumor stroma generation and wound healing. N Engl J Med 1986; 315:1650 - 9; PMID: 3537791
- Olumi AF, Grossfeld GD, Hayward SW, Carroll PR, Tlsty TD, Cunha GR. Carcinoma-associated fibroblasts direct tumor progression of initiated human prostatic epithelium. Cancer Res 1999; 59:5002 - 11; PMID: 10519415
- Bhowmick NA, Moses HL. Tumor-stroma interactions. Curr Opin Genet Dev 2005; 15:97 - 101; http://dx.doi.org/10.1016/j.gde.2004.12.003; PMID: 15661539
- Karnoub AE, Dash AB, Vo AP, Sullivan A, Brooks MW, Bell GW, et al. Mesenchymal stem cells within tumour stroma promote breast cancer metastasis. Nature 2007; 449:557 - 63; http://dx.doi.org/10.1038/nature06188; PMID: 17914389
- Tlsty TD, Coussens LM. Tumor stroma and regulation of cancer development. Annu Rev Pathol 2006; 1:119 - 50; http://dx.doi.org/10.1146/annurev.pathol.1.110304.100224; PMID: 18039110
- Bergamaschi A, Tagliabue E, Sørlie T, Naume B, Triulzi T, Orlandi R, et al. Extracellular matrix signature identifies breast cancer subgroups with different clinical outcome. J Pathol 2008; 214:357 - 67; http://dx.doi.org/10.1002/path.2278; PMID: 18044827
- Rajski M, Zanetti-Dällenbach R, Vogel B, Herrmann R, Rochlitz C, Buess M. IGF-I induced genes in stromal fibroblasts predict the clinical outcome of breast and lung cancer patients. BMC Med 2010; 8:1; http://dx.doi.org/10.1186/1741-7015-8-1; PMID: 20051100
- Sharma M, Beck AH, Webster JA, Espinosa I, Montgomery K, Varma S, et al. Analysis of stromal signatures in the tumor microenvironment of ductal carcinoma in situ. Breast Cancer Res Treat 2010; 123:397 - 404; http://dx.doi.org/10.1007/s10549-009-0654-0; PMID: 19949854