Abstract
Integrin αvβ3 receptor is expressed on several types of cancer cells, including pancreatic cancer cells, and plays an important role in tumor growth and metastasis. The ability to target the integrin αvβ3 receptor on cancer cells increases the efficacy of targeted therapy and reduces the side effects. The aim of this study is to develop a novel arginine-glycine-aspartic acid (RGD) peptide-conjugated albumin nanoparticle to enhance the intracellular uptake of anticancer drug into the pancreatic cancer cells through receptor-mediated endocytosis. In cellular uptake studies, the fluorescent signal of RGD-conjugated BSANPs in BxPC3 cells was higher than that of BSANPs without RGD conjugation as determined by fluorescence spectrophotometer. We also found that BSANPs bound to BxPC3 cells in a time- and concentration-dependent manner. The uptake of RGD-conjugated BSANPs by pancreatic cancer cells was inhibited by an excess amount of free RGD peptide, indicating that the binding and/or uptake were mediated by the αvβ3 receptor. Furthermore, the nanoparticles were found to be located close to the nuclei by using laser scanning confocal microscopy. Besides, no significant in vitro cytotoxicity was observed as measured with MTT assay. Both in vitro and in vivo antitumor efficacy was improved by targeting gemcitabine-loaded nanoparticles to BxPC-3 cells using RGD peptides. Therefore, the RGD-conjugated BSANPs hold great potential as an effective drug delivery system to deliver therapeutic agents to pancreatic cancer.
Introduction
Pancreatic cancer is the fourth most common cause of adult cancer death, accounting for an estimated 43,140 new cases and 36,800 deaths in the USA for 2010.Citation1 Although great progress has been made in diagnosis and targeted therapy, the prognosis of pancreatic cancer still remains poor. Due to the lack of early diagnosis and high incidence of metastatic disease at initial diagnosis, only 15–20% of patients are resectable.Citation2-Citation4 Even so, the 5 y survival rate among those radically operated patients is only about 5–20%.Citation2,Citation5,Citation6 To date, chemotherapy still remains the most important adjuvant therapy in patients with pancreatic malignancies. Currently, gemcitabine has been recommended as the first-line therapy for advanced pancreatic cancer. However, due to a high degree of inherent or acquired chemoresistance, only modest benefit was obtained in patients with advanced disease, even combined with other chemotherapeutic agents.Citation7 In addition, conventional administration of chemotherapeutic agents is often compromised by their systemic toxicity and lack of selectivity. Furthermore, rapid metabolization, poor distribution among cells and the inability of drugs to cross cellular barriersCitation8 all limit the clinical administration of chemotherapeutic agents. Therefore, searching for novel therapeutical strategies is imperative. Recently, a wide range of different types of drug delivery systems have been investigated, including liposomes, polymers, dendrimers, molecular conjugates, etc.Citation8-Citation11 However, the safety issue of these artificial materials remains unknown. Albumin is a safe, nontoxic, biocompatible and biodegradable water-soluble protein present in human plasma and is being widely used clinically, which makes it an ideal candidate for drug delivery vehicle. In addition, tumors are able to trap plasma proteins and utilize their degradation products for proliferation. Bovine serum albumin (BSA) and human serum albumin (HSA) have 80% sequence homology with less than 1% difference in their molecular weights and they have the same isoelectric point. Therefore, BSA was substituted for HSA in our study.Citation12,Citation13
Despite excellent biological properties in using albumin nanoparticles as drug delivery vehicles, further investigations on optimizing their ability to selectively target cancer cells and releasing chemotherapeutical agents in a controlled manner are much needed. To solve the problem of site-specific targeting, some researchers have attempted to enhance tissue specificity of albumin-based drug carriers by coupling them with targeting agents, such as follic acidCitation14 and transferrin.Citation15 Ideally, it would be much better if a targeting agent with therapeutic effect could be bound to albumin physically or covalently. The arginine-glycine-aspartic acid (RGD) sequence was discovered as cell attachment site in fibronectin some 20 y ago. It was found that RGD peptides were able to inhibit tumor metastasis and induce cell apoptosis.Citation16-Citation19 Since then, peptides containing RGD motif have been extensively used in studies of cell adhesion, migration, growth and apoptosis via RGD-integrin interactions.
In our study, we present a new approach to link RGD peptides to albumin nanoparticles with the goal of targeting integrin αvβ3 to enhance its tissue-specific delivery and anticancer activity. Integrin αvβ3 is expressed on many kinds of cancer cells, such as lung cancer,Citation20 ovarian cancer,Citation21 breast cancerCitation22 and melanoma.Citation23 As for pancreatic cancer, integrin αvβ3 is expressed not only on primary tumors, but also on metastatic tumors.Citation24,Citation25
In this study, bovine serum albumin nanoparticles (BSANPs) were prepared by disolvation-crosslinking method and conjugated with RGD peptides via amino groups. In order to trace the nanoparticles, albumin nanoparticles were labeled with fluorescein isothiocyanate (FITC). The cellular uptake of RGD-conjugated nanoparticles was determined by fluorescence spectrophotometery and confocal microscopy in αvβ3 expressing pancreatic cancer cells. Finally, antitumor efficacy studies of the complexes were examined both in vitro and in vivo.
Results
Preparation and characterization of RGD-conjugated BSANP
In this study, we used the well-established disolvation-crosslinking method to prepare BSANPs. BSANPs with smooth surfaces, good dispersion and relatively uniform size distributions were selected. Mean diameters were 130 nm (ranging from 94–166 nm). Zeta potentials were -30.77 mV.
For labeling BSANPs with cyclic RGD peptide, BSANP suspension was pre-incubated with the heterobiofunctional cross-linker, MBS. Activated BSANPs were then incubated with cyclic RGD peptide. Transmission electronic microscopic micrographs confirmed the smooth surfaces, good dispersion and moderately uniform size distribution of cyclic RGD peptide-conjugated BSANPs. Both conjugated and unconjugated nanoparticles were then labeled by FITC, a commonly used tool for tracking protein distribution.
Characteristics of GEM-loaded RGD-conjugated BSANPs
RGD-BSANPs were loaded with gemcitabine using adsorption method. The drug encapsulation rate was 92.16% and drug loading efficiency was 12.8%. The in vitro drug-release curves for GEM-RGD-BSANPs are shown in . The drug-release behavior exhibited a biphasic pattern, which was drug burst release at the initial stage and followed by sustained release at a constant rate. Burst release occurred for 30 min after administration. The rates of burst release in 30 min were 53.25 ± 2.23%. Then the release gradually became slower. The slow release times were about 8 h, showing the potential of the nanoparticles as a sustained drug delivery system. The nanoparticles do not aggregate over time. As shown in , after dissolved in PBS of pH 8.5 and kept in 4°C for 4 weeks, the sample dose not aggregate and still appears milky white colloidal solution.
Expression of αvβ3 receptor
To test the specific bioactivity of the conjugated nanoparticles in αvβ3 integrin expressing cells, the αvβ3 level in four pancreatic cancer cell lines was examined by flow cytometry. Among the four cell lines, BxPC-3 cells showed the highest expression of αvβ3 compared with the other three cells (). BxPC-3 cells were therefore chosen in our study.
Figure 3. Flow cytometric analysis of surface-expressed integrin αvβ3 receptor in BxPC-3, SW1990, PANC-1 and CFPAC-1 cells. Histograms depict relative fluorescence intensity (log scale) of control (above) and integrin αvβ3 (below). BxPC-3 cells showed the highest expression of αvβ3 compared with the other three cells [The positive rate of integrin αvβ3 receptor on the four cell lines: (A) 86.94%, (B) 71.50%, (C) 13.58%, (D) 12.51%].
![Figure 3. Flow cytometric analysis of surface-expressed integrin αvβ3 receptor in BxPC-3, SW1990, PANC-1 and CFPAC-1 cells. Histograms depict relative fluorescence intensity (log scale) of control (above) and integrin αvβ3 (below). BxPC-3 cells showed the highest expression of αvβ3 compared with the other three cells [The positive rate of integrin αvβ3 receptor on the four cell lines: (A) 86.94%, (B) 71.50%, (C) 13.58%, (D) 12.51%].](/cms/asset/1d3a35f4-afba-448d-8436-de43087e14ec/kcbt_a_10918692_f0003.gif)
Cell uptake studies
Observation of uptake of nanoparticles to the cell by fluorescence microscopy
The uptake of the RGD-conjugated BSANPs and BSANPs by BxPC-3 cells was visualized using fluorescence microscopy. The fluorescence intensity of the former group was found to be significantly higher than that of control group without peptide conjugation (). The result revealed the dependence of nanoparticle recognition and uptake on the conjugation of RGD peptide.
Figure 4. Fluorescein isothiocyanate (FITC) was labeled onto the nanoparticles to visualize the uptake of nanoparticles. Fluorescent signal was observed directly under the fluorescence microscope with an unaided eye. The signal of RGD-conjugated BSANPs treated cells (B) was higher than that of BSANPs without RGD conjugation (A).
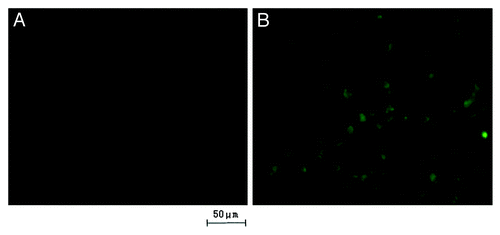
Concentration and time dependence of nanoparticle uptake
In separate experiments, the effects of incubation concentration and time on nanoparticle uptake were studied. BxPC-3 cells were incubated with different concentrations of FITC-labeled RGD-conjugated BSANPs or BSANPs using the protocol mentioned above. With the increase of incubation concentrations of RGD-conjugated BSANPs, the intensity of fluorescence was increased (). Moreover, the intensity of fluorescence of RGD-conjugated BSANPs was significantly higher than that of BSANPs without RGD conjugation at different concentration points (p < 0.05).
Figure 5. Concentration dependence of nanoparticles uptake by BxPC-3 cells. With the increase of incubation concentration of nanoparticles, the intensity of fluorescence enhanced gradually. The nanoparticles were incubated with BxPC-3 cells for 16 h. The intensity of fluorescence of RGD-conjugated BSANPs was significantly higher than that of BSANPs without RGD conjugation at different concentration points (n = 3, *p < 0.05).
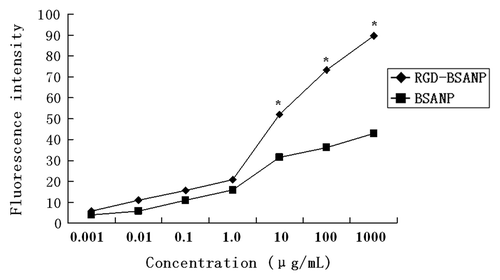
Furthermore, BxPC-3 cells were incubated with 10 μg/ml FITC-labeled nanoparticles for various lengths of time. The intensity of fluorescence of RGD-conjugated BSANPs was increased in a time-dependent manner ().
Figure 6. Time-dependence of nanoparticles uptake by BxPC-3 cells. The concentration of nanoparticles was 10 μg/mL. The intensity of fluorescence increased as time went on. Cells treated with FITC labeled RGD-conjugated BSANPs showed higher fluorescence intensity than cells treated with BSANPs without RGD conjugation (*p < 0.05).
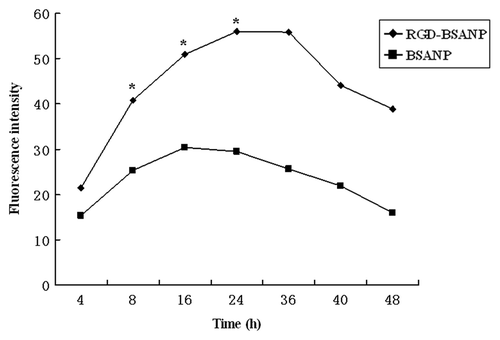
Competition experiments
For the competition experiments, excessive free RGD peptides were added into the cell samples 3 h prior to the incubation with RGD-conjugated BSANPs for another 6 h at room temperature. As shown in , pretreatment of free RGD peptide significantly reduced cellular uptake of RGD-conjugated BSANPs into BxPC-3 cells, suggesting that the cellular uptake of RGD-conjugated BSANPs into BxPC-3 cells is through receptor-mediated endocytosis.
Figure 7. Inhibition of RGD-conjugated BSANPs uptake by free RGD peptides. Excessive free RGD peptides were added into the cell samples 3 h prior to the incubation with RGD-conjugated BSANPs for another 6 h at room temperature. Cellular uptake of RGD-conjugated BSANPs was inhibited by free RGD peptide.
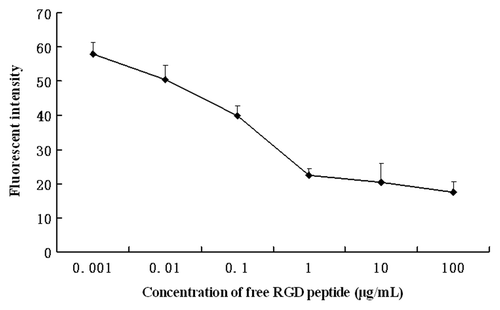
Confocal microscope analysis
The laser scanning confocal microscopy was applied to track the location of nanoparticles. The location of nanoparticles in BxPC-3 cells was shown by green fluorescence using confocal microscopy (). The nuclei were stained in blue. The green fluorescence was observed in close proximity to the nuclei, which confirmed that the nanoparticles were indeed inside the cells and not simply adsorbed to the outer surface.
In vitro cytotoxicity
BxPC-3 cells were exposed to nanoparticles with or without RGD conjugation at 0.01, 0.1, 1, 10 and 100 μg/mL for 24, 48 and 72 h. Cell viability was not significantly decreased as the exposure time and concentration was increased (). No significant difference was found between the RGD-conjugated BSANPs and BSANPs (p > 0.05). RGD-conjugated BSANPs showed negligible cytotoxicity even at high concentrations.
In vitro anticancer activity
The effects of different drugs on the proliferation of BxPC-3 cells were examined by MTT assay at various concentrations for 48 and 72 h respectively. The anticancer activity of RGD-conjugated gemcitabine-loaded nanoparticles (RGD-BSANP-GEM) was compared with the free drug (Gemcitabine, GEM) and drug-loaded nanoparticles (BSANP-GEM). Empty BSANPs and RGD-BSANPs were used as the blank to evaluate any possible cytotoxic effect. All three treatment inhibited the growth of BxPC-3 cells in a dose-dependent manner (). Furthermore, RGD-BSANP-GEM showed a significant improvement in anticancer activity compared with the free drug, the drug-loaded nanoparticles (p < 0.05).
Figure 10. Analysis of cell viability treated with different agents. Compared with other groups, the in vitro antitumor efficacy was improved by targeting gemcitabine-loaded nanoparticles to BxPC-3 cells using RGD peptides,*p < 0.05, vs. BSANP-GEM and GEM group. (A) 48 h, (B) 72 h.
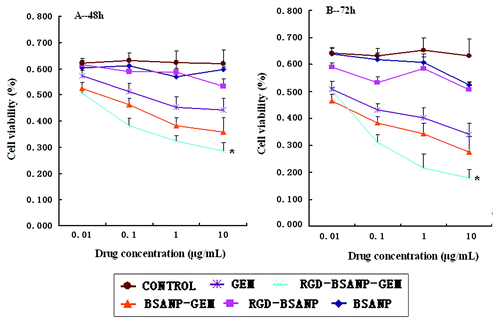
In addition, there was negative correlation between cell viability and αvβ3 integrin expression (). In BxPC-3 cells, RGD-BSANP-GEM showed the highest anticancer activity, indicating the importance of αvβ3 integrin in the targeted therapy of pancreatic cancer.
Cell cycle analysis and apoptosis
As shown in , treatment of the cells with GEM and BSANPs or RGD-BSANPs for 72 h arrested the cells at the G0/G1 phase. The RGD-BSANP-GEM treatment further increased the percentage of cells arrested at the G0/G1 phase, while significantly decreased the population of the cell in G2/M phase. RGD-BSANP-GEM treated cells had the highest apoptotic rate, although no significant differences were found among the six groups (p > 0.05).
Table 1. Cell cycle distribution and apoptotic index of BxPC-3 with different treatments
In vivo antitumor efficacy of GEM-loaded RGD-conjugated BSANPs
The therapeutic effect of the RGD-conjugated BSANPs was further evaluated in a mouse model with pancreatic cancer xenografts. As shown in , after 21 d of treatment, all treatment groups showed tumor inhibitory effect compared with the control group. The RGD-BSANP-GEM group had tumors not only significantly smaller than the control group (the saline group), but also smaller than those treated with either single gemcitabine or gemcitabine-loaded nanoparticles (p < 0.05). This was consistent with in vitro findings, indicating that RGD peptide could improve the antitumor efficacy of gemcitabine.
Figure 12. Changes in the tumor volume and weight after treatment in nude mice bearing xenografts of human pancreatic cancer with saline (control, 1), BSANP alone (2), RGD-BSANP conjugate (3), GEM alone (90 mg/kg, 4), BSANP-GEM (90 mg/kg GEM equivalent, 5) and RGD-BSANP-GEM (90 mg/kg GEM equivalent, 6). The in vivo tumor growth was significantly reduced by treatment with RGD-BSANP-GEM (*p < 0.05).
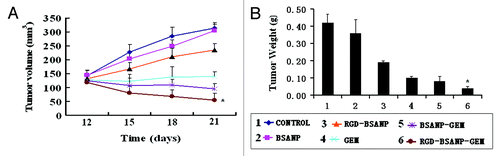
Discussion
Many studies have shown that nanoparticles loaded with chemotherapeutic drugs can be delivered into tumor cells. Nanoparticles passively accumulate in the tumor in a non-targeted way via the Enhanced Permeability and Retention (EPR) effect. Ideally, it would be much better if a targeting agent could be physically conjugated to the surface of nanoparticles, and deliver the vehicle through a target-way, which represents a potential approach to further enhance the antitumor efficacy. The integrins are a family of cell-surface glycoproteins that mediate cell survival, proliferation, and cell migration through explicit non-covalent interactions with endogenous extra cellular matrix (ECM) proteins. They are highly expressed on activated endothelial cells and solid tumor cells but are only minimally present in resting endothelial cells. Among members of the integrin family, integrin αvβ3 has prominent functions in cancer biology, which plays a key role in tumor angiogenesis, apoptosis and metastasis. We developed a novel carrier system based on albumin nanoparticles, which allows for the attachment of RGD peptides for targeting purposes, an anticancer drug, and a fluorescent probe at non-competing binding sites to enable visualization of the cellular uptake.
Unlike the artificial materials used in other studies, albumin is a safe, nontoxic, biocompatible and biodegradable water-soluble protein, which is present in human plasma and has been widely used clinically. Albumin nanoparticles were prepared by the disolvation-crosslinking technique.Citation31 BSANPs with smooth surfaces, good dispersion and relatively uniform size distributions were obtained. The mean diameters of BSANPs were 130 nm. It has been indicated that the size of the nanoparticles plays a crucial role in their interactions with the cells. Nanoparticles of 100–200 nm size acquired the best properties for cellular uptake. Therefore, the size of nanoparticles used in our study is within the optimal range.Citation29
The ability of FITC-labeled BSANPs with or without RGD peptide conjugation to target αvβ3 positive BxPC-3 cells was evaluated by fluorescence microscopy. The results clearly indicated that RGD-conjugated BSANPs selectively accumulate in BxPC-3 cells when compared with BSANPs without RGD conjugation. We also found that RGD-conjugated BSANPs bound to BxPC3 cells in a time- and concentration-dependent manner. It was noted that, the uptake level reached the maximum and then declined after 24 h. The decrease of fluorescencent intensity in nanoparticle uptake could be attributed to the degradation or photobleaching of FITC.Citation29,Citation32 In addition, RGD-containing peptides were found to be able to directly induce cell apoptosis, which could also cause the reduction of fluorescencent intensity.
In addition, the uptake of RGD-conjugated BSANPs by pancreatic cancer cells was blocked by addition of free RGD peptide, indicating that the RGD peptides are mediating the binding of the functionalized BSANPs to the cells. Furthermore, we investigated the intracellular localization of FITC-labeled BSANPs by laser scanning confocal microscopy. The nanoparticles were found to be located close to the nuclei. Therefore, when functionalized nanoparticles loaded with chemotherapeutic agents enter the cells, drugs can be released from the nanoparticles, accumulate inside the cells and ultimately reach the cell nuclei.
Both the in vitro and in vivo antitumor efficacy was improved by targeting gemcitabine-loaded nanoparticles to BxPC-3 cells using RGD peptides. Chemotherapeutic agents work mainly by inhibiting cell proliferation and/or inducing cell apoptosis. As demonstrated by flow cytometry, RGD arrested cells at G0/G1 phase, indicating that one of the mechanisms by which RGD inhibited cell proliferation was via obstruction of cell cycle progression. Gemcitabine arrested cells at S phase by inhibiting DNA synthesis.Citation33 Our results showed that gemcitabine-loaded RGD-conjugated nanoparticles resulted in significantly antitumor effects in BxPC-3 cells, as indicated by MTT and cell apoptosis assays, respectively. Furthermore, the in vitro results were further validated by the decreased tumor volume and weight in vivo. Nowadays, patients with advanced pancreatic cancer have limited response rate to gemcitabine, which is mainly due to resistance to apoptosis induced by chemotherapeutic agents. Therefore, the RGD-conjugated BSANPs represent a novel carrier system to deliver drugs into the pancreatic cancer cells and other cancer cells.
Conclusion
In this study, albumin nanoparticles prepared by the desolvation-crosslinking technique were conjugated with RGD peptide. Cell uptakes studies were performed in BxPC3 cells since they overexpress αvβ3 receptors. It was demonstrated that RGD-conjugated albumin nanoparticles were successfully taken up into BxPC-3 cells in a selective fashion. Both the in vitro and in vivo antitumor efficacy was improved by targeting gemcitabine-loaded nanoparticles to BxPC-3 cells using RGD peptides. Further studies are in progress to define the exact molecular mechanism of this new drug delivery system both in vitro and in vivo.
Materials and Methods
Chemicals
BSA was purchased from Bo’ao Biological Technology Co, Ltd., cyclic RGD peptides with the sequence: cyclo(-Arg-Gly-Asp-D-Tyr-Lys)-Cys, were purchased from GL Biochem Ltd. Fluorescein isothiocyanate (FITC) was from Sigma Chemical Co. RPMI1640 and DMSO were from GIBCO Laboratories, and MTT and trypsin were from Sigma Chemical Co. MBS, m-maleimidobenzoyl-N-hydroxysuccinimide ester, was also purchased from Sigma. DAPI was from Roche. Glutaraldehyde, NaOH, dehydrated alcohol, and double-distilled water were used. All the chemicals were of at least analytical grade.
Cell culture
Four human pancreatic cancer cell lines, BxPC3, SW1990, PANC-1and CFPAC-1, were purchased from the Shanghai Institute of Biochemistry and Cell Biology. The cells were cultured in RPMI 1640 supplemented with 10% fetal bovine serum, 50 U penicillin/mL, and 50 μg streptomycin/mL in a humidified atmosphere with 95% O2 and 5% CO2 at 37°C.
Preparation of bovine serum albumin nanoparticles
The albumin nanoparticles were prepared by the desolvation-crosslinking technique as previously described.Citation26 Briefly, 10 ml aqueous BSA (2.5% w/v) was prepared. The pH was adjusted to 8.0–8.2 with NaOH (1 mol/L). Under constant stirring, dehydrated ethanol (25–40 mL) was subsequently added drop-wise into the mixture at a rate of 1 mL/min until the formation of milky white colloidal solution. After the desolvation process, glutaraldehyde solution was added to the mixture under stirring for 16–20 h to induce crosslinking. Then, the mixture was rotary evaporated (ZX-91 rotary evaporator, Institute of Organic Chemistry, Chinese Academy of Sciences) at 40°C to remove the ethanol. The remaining colloidal solution were separated by centrifugation in a TCL-16C high-speed centrifuge at 14,000 r/min for 20 min (Shanghai Anting Scientific Instrument Factory). After removing the supernatant, the precipitated nanoparticles were resuspended in purified water, yielding a suspension of nanoparticles.
Characterization of nanoparticles
The size and zeta potential of the nanoparticles were detected by NicompTM 380/ZLS electric potential/particle diameter radiometry (Nicomp). Nanospheres were dispersed in isotonic NaCl (1 mg/mL) and examined at 25°C at a lightscattering angle of 90°. The mean particle size was determined in a condition of scattering intensity as intensity weight. The zeta potential was detected in a 10 V electric field. The morphology of nanoparticles was examined by a transmission electronic microscope (Hitachi H-600) after being dried on copper grids at room temperature.
Conjugation of RGD peptide to BSANPs
BSANPs modification with MBS was performed as described by Masahiro Tomita and coworkers.Citation27 BSANPs were first activated by heterobiofunctional cross-linker, MBS (10 mg/ml in DMF) for half an hour at room temperature with gentle agitation. After activation, the suspension was incubated with cyclic RGD peptide at a molar ratio of 10:1 for 3 h at room temperature. In this step, the maleimido group of MBS reacted with the free sulfhydryl group of the Cys residue on the peptide. Then the reaction mixture was dialyzed against PBS (pH 7.2) at 4°C overnight.
FITC labeling to BSANPS and RGD-conjugated BSANPs
The synthesis of FITC-labeled nanoparticles was based on the reaction between the isothiocyanate group of FITC and the primary amino group of nanoparticles.Citation28,Citation29Briefly, FITC was mixed with BSANPs in sodium bicarbonate buffer, pH 9.5, followed by incubation for 20–24 h in darkness at room temperature while stirring. It was then dialyzed against distilled water using a cellulose membrane (molecular weight cutoff 8,000–12,000) for 2 d and lyophilized. The BCA Protein Assay Kit (Pierce) was used to measure the protein concentration according to the manufacturer's instructions. The fluorescein–BSANP conjugates were resuspended in sodium phosphate buffer 20 mM (pH 7.4) at a BSANPs concentration of 7 mg/mL.
Preparation of gemcitabine-loaded RGD-conjugated albumin nanoparticles
GEM-loaded RGD-conjugated BSANPs were prepared by the adsorption method. The precipitated RGD-conjugated nanoparticles were resuspended in purified water. Ten mg/mL aqueous GEM was added under magnetic stirring with pH adjusted to 8.5 with 1 mol/L NaOH. After continued magnetic stirring for 12 h, the nanoparticles were separated by centrifugation in a high-speed centrifuge at 14,000 r/min for 20 min. The precipitated nanoparticles were suspended in purified water and subjected to cryodesiccation, yielding a desiccated powder of nanoparticles. The drug loading efficiency (LE) of nanoparticles and encapsulation efficiency (EE) of the process were calculated as indicated below:
LE =
EE =
In Vitro Drug Release Assay
The drug release profiles from nanoparticles were measured in vitro. One milliliter of 1 mg/mL GEM-NSP was sealed in a dialysis bag and immersed in 49 mL of PBS containing 10% (v/v) fetal bovine serum (FBS) at 37°C with a shaking rate of 100 r/min. A sample (0.5 mL each) was withdrawn from the medium at designated time intervals and the same volume of fresh medium was added. Each sample was mixed with 1.5 mL of acetonitrile, vortexed for 3 min, and centrifuged, and the amount of gemcitabine in the supernatant was determined by HPLC. All measurements were performed in triplicate.
The αvβ3 receptor expression of pancreatic cancer cells
The αvβ3 receptor expression was determined by flow cytometric analysis.Citation30 Cells were cultured as monolayers in DMEM supplemented with 10% FBS (v/v) and maintained at 37°C in a humidified incubator (95% humidity) containing 5% CO2. Cultured cells were harvested and seeded with a concentration of 106 cells per well on the 24-well plates. Cells were incubated with either 3% bovine serum albumin in phosphate buffered saline only (blank cells and secondary antibody only cells) or with mouse anti-human αvβ3 antibody (Abcam) at 1:100 dilution for 1 h on ice. Cells were washed twice with PBS before all wells except for blanks were treated with a 1:100 dilution of a FITC-labeled goat anti-mouse IgG (Abcam) antibody on ice for 1 h in the dark. Finally, cells were washed again twice with PBS. Flow cytometric analysis was performed immediately using a FACScalibur flow cytometer (Becton Dickinson). All experiments were performed with replicates of three.
Cell uptake studies
BxPC-3 cells were seeded in 96-well plates at a density of 4.3 × 103 cells per well and cultured in the presence of FITC-labeled RGD-conjugated BSANPs or BSANPs in a particle concentration of 10 μg/mL. After 6 h of incubation at room temperature, the cells were washed with PBS three times. The image was visualized via fluorescence microscopy (Olympus X 51).
To confirm the role of selective targeting to tumor cells by αvβ3 receptor, competition experiments were performed. Different concentrations of free RGD peptides were added 3 h prior to the nanoparticle administration (10 μg/mL). After the 6 h incubation period, the cells were washed three times with PBS and analyzed under fluorescence spectrophotometer.
The influence of time and concentration on the cellular uptake was also evaluated. FITC-labeled RGD-conjugated BSANPs or BSANPs (with concentration of 10 μg/mL) were incubated with cells at room temperature for various length of time. The uptake of RGD-conjugated BSANPs or BSANPs with different concentration at room temperature was also detected. The cells were washed three times before analyzing. Each measurement was performed in three wells.
For the confocal microscope analysis, cells were treated with RGD-conjugated BSANPs at a concentration of 100 μg/mL for 3 h, washed with PBS three times and fixed for 30 min using 4% paraformaldehde solution. Cell nuclei were stained with DAPI, followed by rinses in PBS (three times, 2 min each). Cells were then examined using a Zeiss Pascal LSM 5 confocal microscope (Carl Zeiss).
Cell toxicity assay
Cytotoxicity of RGD-conjugated BSANPs was determined by using the MTT assay. In brief, BxPC-3 cells were seeded into 96-well plates at a density of 1.0 × 105 cells per well in RPMI 1640 supplemented with 10% fetal calf serum and allowed to attach overnight. The culture medium was refreshed on the next day, and the cells were exposed to 0.01–100 μg/mL nanoparticle suspension in 20 μl final volume/well for 24, 48 and 72 h separately. After incubation, MTT solution (20 μl of 5 mg/ml stock solution) was added to each well and incubated at 37°C for 4 h. The cell culture medium was aspirated carefully and 150 μl DMSO was added to each well and mixed thoroughly to dissolve the formazan crystals. Optical density (OD) was spectroscopically measured at 490 nm. Untreated cells were taken as the control with 100% viability. Each measurement was performed three times. Results were calculated with respect to the untreated control.
Cell proliferation assay
Similarly, the cells were seeded in 96-well plates (5 × 103 cells/well) and incubated at 37°C, 5% CO2 overnight. The following day, the indicated concentrations of GEM and BSANPs or RGD-BSANPs in PBS were added into each well, and the cells were incubated for additional 48 and 72 h. Cells treated with only fresh medium were used as untreated control. The number of cells alive was determined using an MTT assay. Experiments were repeated thrice.
Apoptosis
After BxPC-3 cells had been exposed to different drugs for 24 h in 6-well plates, the degree of apoptosis was assessed by annexin V binding assay as instructed by the manufacturer (BD Biosciences). After trypsinization, cells were incubated with annexin V-FITC and PI for 10 min at room temperature in the dark and then analyzed by flow cytometry. The percentages of stained cells in each quadrant were quantified using CellQuest software (BD Biosciences).
Cell cycle analysis
After treatment for 24 h, BxPC-3 cells were harvested, ashed with ice-cold PBS and fixed with 70% ethanol at 4°C overnight. The ethanol was removed by centrifugation and approximately 106 cells were re-suspended in PBS containing PI (50 μg/mL, Sigma) for 30 min in the dark before analyzed on flow cytometry (FACScalibur). The percentage of cells at G0/G1, S, or G2/M phase was thereby documented. The experiment was repeated three times.
Animal model and treatment
The tumor models were generated by subcutaneous injection of 3 × 106 BxPC-3 cells in 50 μL PBS into the flank of male BALB/C-nu/nu mice. Mice with xenografts of approximately 5 mm in length (7 d after tumor inoculation) were injected intravenously twice per week four times with 150–200 μL of different formulations of GEM and BSANPs or RGD-BSANPs (GEM 90 mg/Kg). On the 21st day after inoculation, all mice were euthanized by CO2 overdose and the tumor size and weight were assessed. The tumor sizes were measured by a digital caliper and calculated using the formula for a prolated sphere: 0.5 × A × B2, where A is the length of the tumor and B is the width. All animal experiments were performed according to our institutional and NIH guidelines for care and use of research animals.
Statistical analysis
Results were expressed as means of three separate experiments, and were compared by analysis of variance (ANOVA). Differences were considered to be significant at a level of p value < 0.05.
Acknowledgments
This research was supported in part by the Shanghai Economic Commission (07ZH-028) and the National Science Foundation of China (30901760, 30701007 and 30901435).
Disclosure of Potential Conflicts of Interest
No potential conflicts of interest were disclosed.
References
- Jemal A, Siegel R, Xu J, Ward E. Cancer statistics. CA Cancer J Clin 2008; 58:71 - 96; http://dx.doi.org/10.3322/CA.2007.0010; PMID: 18287387
- Li D, Xie KP, Wolff R, Abbruzzese JL. Pancreatic cancer. Lancet 2004; 363:1049 - 57; http://dx.doi.org/10.1016/S0140-6736(04)15841-8; PMID: 15051286
- Linder S, Bostrom L, Nilsson B. Pancreatic cancer in Sweden 1980–2000: a population-based study of hospitalized patients concerning time trends in curative surgery and other interventional therapies. J Gastrointest Surg 2006; 10:672 - 8; http://dx.doi.org/10.1016/j.gassur.2005.10.005; PMID: 16713540
- Cress RD, Yin D, Clarke L, Bold R, Holly EA. Survival among patients with adenocarcinoma of the pancreas: a population-based study (United States). Cancer Causes Control 2006; 17:403 - 9; http://dx.doi.org/10.1007/s10552-005-0539-4; PMID: 16596292
- Smeenk HG, Tran TC, Erdmann J, van Eijck CH, Jeekel J. Survival after surgical management of pancreatic adenocarcinoma: does curative and radical surgery truly exist?. Langenbecks Arch Surg 2005; 390:94 - 103; http://dx.doi.org/10.1007/s00423-004-0476-9; PMID: 15578211
- Schäfer M, Mullhaupt B, Clavien PA. Evidence-based pancreatic head resection for pancreatic cancer and chronic pancreatitis. Ann Surg 2002; 236:137 - 48; http://dx.doi.org/10.1097/00000658-200208000-00001; PMID: 12170018
- Bergman AM, Pinedo HM, Peters GJ. Determinants of resistance to 2’,2’-difluorodeoxycytidine (gemcitabine). Drug Resist Updat 2002; 5:19 - 33; http://dx.doi.org/10.1016/S1368-7646(02)00002-X; PMID: 12127861
- Heister E, Neves V, Tilmaciu C, Lipert K, Beltran VS, Coley HM, et al. Triple functionalisation of single-walled carbon nanotubes with doxorubicin, a monoclonal antibody, and a fluorescent marker for targeted cancer therapy. Carbon 2009; 47:2152 - 60; http://dx.doi.org/10.1016/j.carbon.2009.03.057
- Reddy LH, Couvreur P. Novel approaches to deliver gemcitabine to cancers. Curr Pharm Des 2008; 14:1124 - 37; http://dx.doi.org/10.2174/138161208784246216; PMID: 18473859
- Yang J, Park SB, Yoon HG, Huh YM, Haam S. Preparation of poly epsilon-caprolactone nanoparticles containing magnetite for magnetic drug carrier. Int J Pharm 2006; 324:185 - 90; http://dx.doi.org/10.1016/j.ijpharm.2006.06.029; PMID: 16872766
- Celano M, Calvagno MG, Bulotta S, Paolino D, Arturi F, Rotiroti D, et al. Cytotoxic effects of gemcitabine-loaded liposomes in human anaplastic thyroid carcinoma cells. BMC Cancer 2004; 4:63; http://dx.doi.org/10.1186/1471-2407-4-63; PMID: 15363094
- Kurrat R, Prenosil JE, Ramsden JJ. Kinetics of human and bovine serum albumin adsorption at silica-titania surfaces. J Colloid Interface Sci 1997; 185:1 - 8; http://dx.doi.org/10.1006/jcis.1996.4528; PMID: 9056288
- Gelamo EL, Tabak M. Spectroscopic studies on the interaction of bovine (BSA) and human (HSA) serum albumins with ionic surfactants. Spectrochim Acta A Mol Biomol Spectrosc 2000; 56A:2255 - 71; http://dx.doi.org/10.1016/S1386-1425(00)00313-9; PMID: 11058071
- Zhang L, Hou SX, Mao SJ, Wei DP, Song XJ, Lu Y. Uptake of folate-conjugated albumin nanoparticles to the SKOV3 cells. Int J Pharm 2004; 287:155 - 62; http://dx.doi.org/10.1016/j.ijpharm.2004.08.015; PMID: 15541922
- Ulbrich K, Hekmataraa T, Herberta E, Kreuter J. Transferrin- and transferrin-receptor-antibody-modified nanoparticles enable drug delivery across the blood–brain barrier (BBB). Eur J Pharm Biopharm 2009; 71:251 - 6; http://dx.doi.org/10.1016/j.ejpb.2008.08.021; PMID: 18805484
- Buckley CD, Pilling D, Henriquez NV, Parsonage G, Threlfall K, Scheel-Toellner D, et al. RGD-peptides induce apoptosis by direct caspase-3 activation. Nature 1999; 397:534 - 9; http://dx.doi.org/10.1038/17409; PMID: 10028971
- Anuradha CD, Kanno S, Hirano S. RGD peptide-induced apoptosis in human leukemia HL-60 cells requires caspase-3 activation. Cell Biol Toxicol 2000; 16:275 - 83; http://dx.doi.org/10.1023/A:1026758429238; PMID: 11201051
- Madeja Z, Sroka J. Contact guidance of Walker carcinosarcoma cells by the underlying normal fibroblasts is inhibited by RGD-containing synthetic peptides. Folia Histochem Cytobiol 2002; 40:251 - 60; PMID: 12219835
- Kang IC, Kim DS, Jang Y, Chung KH. Suppressive mechanism of salmosin, a novel disintegrin in B16 melanoma cell metastasis. Biochem Biophys Res Commun 2000; 275:169 - 73; http://dx.doi.org/10.1006/bbrc.2000.3130; PMID: 10944460
- Cui R, Takahashi F, Ohashi R, Gu T, Yoshioka M, Nishio K, et al. Abrogation of the interaction between osteopontin and αvβ3 integrin reduces tumor growth of human lung cancer cells in mice. Lung Cancer 2007; 57:302 - 10; http://dx.doi.org/10.1016/j.lungcan.2007.03.019; PMID: 17482311
- Cannistra SA, Ottensmeier C, Niloff J, Orta B, DiCarlo J. Expression and Function of β1 and αvβ3 Integrins in Ovarian Cancer. Gynecol Oncol 1995; 58:216 - 25; http://dx.doi.org/10.1006/gyno.1995.1214; PMID: 7542622
- Borrirukwanit K, Lafleur MA, Mercuri FA, Blick T, Price JT, Fridman R, et al. The type I collagen induction of MT1-MMP-mediated MMP-2 activation is repressed by αVβ3 integrin in human breast cancer cells. Matrix Biol 2007; 26:291 - 305; http://dx.doi.org/10.1016/j.matbio.2006.10.014; PMID: 17175151
- Tzukert K, Shimony N, Krasny L, Urieli-Shoval S, Gorodetsky R, Avrahami I, et al. Human melanoma cells expressing the αvβ3 integrin are partially protected from necrotic cell death induced by dynamic matrix detachment. Cancer Lett 2010; 290:174 - 81; http://dx.doi.org/10.1016/j.canlet.2009.09.007; PMID: 19804941
- Holloway SE, Beck AW, Girard L, Jaber MR, Barnett CC Jr., Brekken RA, et al. Increased expression of Cyr61 (CCN1) identified in peritoneal metastases from human pancreatic cancer. J Am Coll Surg 2005; 200:371 - 7; http://dx.doi.org/10.1016/j.jamcollsurg.2004.10.005; PMID: 15737847
- Hosotani R, Kawaguchi M, Masui T, Koshiba T, Ida J, Fujimoto K, et al. Expression of integrin αvβ3 in pancreatic carcinoma: relation to mmp-2 activation and lymph node metastasis. Pancreas 2002; 25:30 - 5; http://dx.doi.org/10.1097/00006676-200208000-00021
- Li JM, Chen W, Wang H, Jin C, Yu XJ, Lu WY, et al. Preparation of albumin nanospheres loaded with gemcitabine and their cytotoxicity against BXPC-3 cells in vitro. Acta Pharmacol Sin 2009; 30:1337 - 43; http://dx.doi.org/10.1038/aps.2009.125; PMID: 19730429
- Tomita M, Sugi H, Ozawa K, Tsong TY, Yoshimura T. Targeting antigen-specific receptors on B lymphocytes to generate high yields of specific monoclonal antibodies directed against biologically active lower antigenic peptides within presenilin 1. J Immunol Methods 2001; 251:31 - 43; http://dx.doi.org/10.1016/S0022-1759(01)00299-X; PMID: 11292479
- Du SL, Pan H, Lu WY, Wang J, Wu J, Wang JY. Cyclic RGD peptide-labeled liposomes for targeting drug therapy of hepatic fibrosis in rats. J Pharmacol Exp Ther 2007; 322:560 - 8; http://dx.doi.org/10.1124/jpet.107.122481; PMID: 17510318
- Zhang J, Chen XG, Peng WB, Liu CS. Uptake of oleoyl-chitosan nanoparticles by A549 cells. Nanomedicine 2008; 4:208 - 14; http://dx.doi.org/10.1016/j.nano.2008.03.006; PMID: 18508414
- Merkel OM, Germershaus O, Wada CK, Tarcha PJ, Merdan T, Kissel T. Integrin αvβ3 targeted gene delivery using rgd peptidomimetic conjugates with copolymers of pegylated poly (ethylene imine). Bioconjug Chem 2009; 20:1270 - 80; http://dx.doi.org/10.1021/bc9001695; PMID: 19476331
- Weber C, Coester C, Kreuter J, Langer K. Desolvation process and surface characterisation of protein nanoparticles. Int J Pharm 2000; 194:91 - 102; http://dx.doi.org/10.1016/S0378-5173(99)00370-1; PMID: 10601688
- Son SJ, Bai X, Lee SB. Inorganic hollow nanoparticles and nanotubes in nanomedicine Part 2: Imaging, diagnostic, and therapeutic applications. Drug Discov Today 2007; 12:657 - 63; http://dx.doi.org/10.1016/j.drudis.2007.06.012; PMID: 17706548
- Morgan MA, Meirovitz A, Davis MA, Kollar LE, Hassan MC, Lawrence TS. Radiotherapy combined with gemcitabine and oxaliplatinin pancreatic cancer cells. Transl Oncol 2008; 1:36 - 43; PMID: 18607506