Abstract
Accumulating evidence suggests that Ginsenoside Rg3 appears to inhibit tumor growth including Lewis lung carcinoma, intestinal adenocarcinomas or B16 melanoma by inhibiting cell proliferation, tumor cell invasion and metastasis. Endothelial progenitor cells (EPCs) appear to play a key role in the growth of early tumors by intervening with the angiogenic switch promoting tumor neovessel formation by producing angiogenic cytokines during tumor progression. This paper reports a novel mechanism of Ginsenoside Rg3, a candidate anticancer bio-molecule, on tumor angiogenesis by inhibiting the multiple bioactivities of EPCs. When Ginsenoside Rg3 was applied to the ex vivo cultured outgrowth ECs, a type of EPCs, it inhibited the cell proliferation, cell migration and tubular formation of EPCs. Importantly, Ginsenoside Rg3 attenuated the phosphorylation cascade of the VEGF dependent p38/ERK signaling in vitro. The xenograft tumor model clearly showed that Ginsenoside Rg3 suppresses tumor growth and tumor angiogenesis by inhibiting the mobilization of EPCs from the bone marrow microenvironment to the peripheral circulation and modulates VEGF-dependent tumor angiogenesis. In conclusion, this study provides a potential therapeutic molecule, Ginsenoside Rg3, as an anticancer drug by inhibiting the EPC bioactivities.
Introduction
Since the uncontrolled growth of solid tumors is closely related to tumor angiogenesis by delivering nutrients and oxygen for the survival of tumor cells, as well as providing a route for metastatic spread, adequate control of tumor angiogenesis has been an attractive target for tumor therapy.Citation1,Citation2 Recently, the accumulating evidence has indicated the importance of bone marrow-derived stem/progenitor cells because these progenitor cells and endothelial cells to the tumor vasculature involves tumor-induced neovascularization.Citation3,Citation4
Angiogenesis promoting cells including endothelial progenitor cells (EPCs, outgrowth ECs), hematopoietic precursor cells, mesenchymal stem cells (MSCs) are mobilized from the bone marrow (BM) via injury-derived mobilization promoting factors, such as vascular endothelial growth factor (VEGF), granulocyte colony-stimulating factor (G-CSF), soluble c-kit ligands (s-cKitL) and/or stromal-derived factor-1 (SDF-1) to accelerate the growth of solid tumors.Citation5,Citation6 Importantly, Lyden D et al. demonstrated that the impaired recruitment of bone-marrow-derived endothelial and hematopoietic precursor cells blocks tumor angiogenesis and growth.Citation7 Similarly, accumulating evidence suggests that EPCs are closely related to tumor angiogenesis by accelerating the growth of early tumors by intervening with the angiogenic switch promoting tumor neovessel during tumor progression.Citation7-Citation11 Therefore, the adequate inhibition of a functional cascade, such as the mobilization step of angiogenesis-promoting progenitor cells, initiating from the BM microenvironment to the peripheral blood, and an incorporation step into ischemic tumor tissue during neovessel formation by specific molecules, is a potent and promising anticancer strategy.Citation12,Citation13 On the other hand, these findings and the role of effective therapeutic targeting bio-molecules by inhibiting tumor angiogenesis promoted by stem/progenitor cells has not been elucidated.
Recently, a wide array of naturally occurring substances derived from edible and medicinal plants have been reported to preserve the anticarcinogenic and antimutagenic properties, partly due to their chemopreventive activities of phytochemicals including anti-inflammatory and antioxidant bioactivities.Citation14,Citation15 Ginseng has been used as folk medicine in Asian countries for thousands of years to treat various diseases owing to its wide range of pharmacological and therapeutical actions, such as biomodulation, anti-hypoxia stress, and anti-aging activities in central nervous system, immune system and cardiovascular system. The effects of ginseng include general “tonic,” anti-fatigue, anti-stress, immunomodulatory and anti-cancer.Citation16,Citation17 Ginsenoside Rg3 is a pure compound extracted from ginseng that inhibits growth and induces the apoptosis of tumor cells. Accumulating evidence suggests that it inhibits tumor growth by inhibiting the invasion and metastasis of various tumors including Lewis lung carcinoma,Citation18,Citation19 intestinal adenocarcinomas, B16 melanoma,Citation20,Citation21 as well as suppressing the proliferation of prostate cancer cells.
During tumor progression, VEGF signaling is an important aspect of the tumor angiogenesis process because it closely related to the accelerated growth of tumors, invasion and metastasis, leading to the outgrowth of pathogenic vessels.Citation22 Similarly, VEGF signaling and monoclonal antibodies and small molecule inhibitors of VEGF or its receptors have been established as a proven approach to inhibit tumor growth.Citation23-Citation25 In an attempt to elucidate the molecular mechanism underlying the possible tumor vessel promoting activity of stem/progenitor cells, many studies have recently demonstrated that VEGF signaling plays a critical role in the upregulation of a range of cellular functions of EPCs, such as cell migration, proliferation, survival and angiogenesis.Citation26 In sites of hypoxic tumor tissue, the recruited EPCs secrete abundant VEGF and promote an abnormal vascular network. This network that forms in tumors is often leaky and hemorrhagic, partly due to the overproduction of VEGF (also known as vascular permeability factor, VPF). In addition to the well-defined effects on blood vessel formation, there is evidence indicating that overproduction of VEGF also enhances tumor inflammation.Citation7,Citation27 As the downstream signaling cascade of VEGF-mediated EPC biology to regulate EPC migration, proliferation, growth and survival, the activation of two major signal pathways of EPCs, the phosphatidylinositol 3-kinase-AKT and Raf-MEK-extracellular signal regulated kinase (ERK) pathways, appears to be involved in the promotion of tumor angiogenesis.Citation28,Citation29 Therefore, in an attempt to block of tumor angiogenesis for the treatment of a variety of clinical cancer patients, the possibility of VEGF targeting molecules would provide a promising cancer therapeutic approach for the effective control of tumor angiogenesis.
Determining the regulation substance of VEGF-induced tumor angiogenesis has been a major focus in natural product screening research. For example, Apigenin, a natural product, inhibits tumor angiogenesis by decreasing HIF-1α and VEGF expression.Citation30 Similarly, Thymoquinone, a phytochemical compound found in the plant Nigella sativa, inhibits tumor angiogenesis and tumor growth by suppressing extracellular signal-regulated kinase signaling pathways.Citation31 Until now, although antiangiogenic molecules dominantly target endothelial cells and exhibit effective control of tumor angiogenesis, the finding of a natural product-derived single compound to control the tumor mass via the marked delay of tumor angiogenesis, particularly through the modulation of stem/progenitor cells bioactivities, are largely unknown.
Therefore, this study examined whether ginsenoside Rg3 is a therapeutic targeting biomolecule for the suppression of angiogenesis promoting EPCs, eventually inhibiting the tumor vasculature by blocking the VEGF-VEGFR2 intracellular signaling pathways. Indeed, Ginsenoside Rg3 inhibits the VEGF dependent in vitro migratory capacity and cell proliferation of EPCs by attenuating the phosphorylation cascade of VEGF-p38 axis in vitro. Ginsenoside Rg3 also blocks the angiogenesis properties including both the in vitro tubular formation capacity and in vivo blood vessel formation demonstrated by matrigel plug assays. Furthermore, xenograft murine model shows that ginsenoside Rg3 abrogates the mobilization of BM-derived EPCs and inhibits tumor angiogenesis, thereby attenuating Lewis lung carcinoma (LLC) tumor growth and the formation of the tumor vasculature.
Results
Effect of Ginsenoside Rg3 on cell viability and apoptosis in EPCs and LLCs
Since Ginsenoside Rg3 can affect the cell viability of EPCs and LLCs, the viability assay was performed in the condition of treatment of Ginsenoside Rg3 in time- or dose-dependent manner. As shown in Figure S1A–C, there are no significant difference in cell viability and cell number in Ginsenoside Rg3-treated groups at least less than 72 h. An apoptosis assay was performed in serum free media by checking the expression of cleaved caspase3, a typical indicator of apoptosis to further clarify the effect of Ginsenoside Rg3 on cell survival of EPCs and LLCs. As shown in and Figure S2A, we observed a significant increase in the level of cleaved caspase3 after a treatment with Ginsenoside Rg3 in a time- and dose-dependent manner, suggesting that the incubation of Ginsenoside Rg3 drastically promoted programmed cell death signals in CB-derived EPCs and LLCs. Moreover, we clarified these findings using FACS analysis by analyzing apoptotic cells, indicating Annexin V (+)/PI (-) cells. As shown in Figure S2B, Ginsenoside Rg3 led to significantly increase in apoptotic cells and dead cells.
Figure 1. Effect of Ginsenoside Rg3 on the cell viability and apoptosis of EPCs. (A) The chemical structure of Ginsenoside Rg3. (B) Endothelial phenotype of outgrowth ECs, derived from isolated cord blood MNCs was confirmed by FACS analysis, demonstrating the expression of endothelial markers CD31, CD34, KDR, CXCR4, c-Kit, CD133. The graph represents the percentage of outgrowth ECs. (C, D) Cytotoxic effect was determined using EPCs and LLCs in the presence of Ginsenoside Rg3. After cultivation for 24 h under normal culture conditions, the total cell number was analyzed using a WST-1 assay. The cell number was not altered significantly by a treatment with up to 600 ng/mL Ginsenoside Rg3, demonstrating that Ginsenoside Rg3 does not affect the viability of EPCs and LLC. (E, F) Apoptosis effect was determined using EPCs and LLCs in the presence of Ginsenoside Rg3. After cultivation for 24 h under serum free culture conditions, cleaved caspase-3, a typical indicator of apoptosis, was increased after treatment with Ginsenoside Rg3 in a dose dependent manner, as demonstrated by western blot analysis.
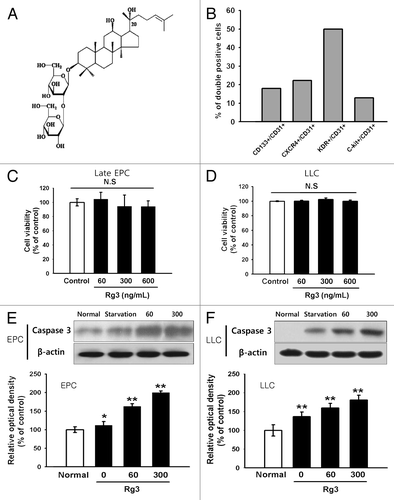
Effect of Ginsenoside Rg3 on VEGFR2 expression and cell proliferation in EPCs
The effects of Ginsenoside Rg3 in VEGFR2 expression was first examined by RT-PCR, real time RT-PCR and western blotting to examine the effect of Ginsenoside Rg3 on the anti-proliferative properties for EPCs ( and ). The expression level of the VEGFR2 mRNA and protein was decreased markedly by Rg3 in a dose dependent manner. In addition, proliferation properties were assessed after stimulating with Ginsenoside Rg3 in the presence of VEGF. Significantly, Ginsenoside Rg3 inhibited VEGF dependent cell proliferation in a dose-dependent manner (). This study focused on the VEGF dependent signaling pathways to examine the effect of Ginsenoside Rg3 on the signal cascade of cell proliferation of EPCs. Western blot analysis was performed using p-ERK1/2, ERK1/2, p-p38, p38, p-JNK and JNK antibodies. β-actin was used as a protein loading control. As shown in , the phosphorylation of VEGF-dependent ERK and p38 signaling were decreased significantly but not in JNK signaling, while there is no significant difference in Ginsenoside Rg3-treated groups without VEGF stimulation (Figure S4A).
Figure 2. Effect of Ginsenoside Rg3 on the VEGFR2 expression in EPCs. (A) mRNA level of VEGFR2 in Rg3-treated outgrowth ECs, demonstrated by RT-PCR analysis. (B) VEGFR2 protein expression in Rg3-treated outgrowth ECs, demonstrated by western blotting analysis.
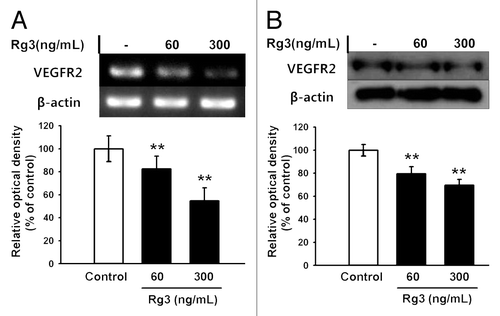
Figure 3. Effect of Ginsenoside Rg3 on the proliferation of EPCs. (A) Effect of Ginsenoside Rg3 on VEGF-induced cell proliferation in outgrowth ECs. Cell proliferation assay was performed at 24 h after treatment with or without Ginsenoside Rg3 in the presence of VEGF. **p < 0.005 (significance between control and VEGF), *p < 0.05 (significance between VEGF and VEGF plus Ginsenoside Rg3). (B) Ginsenoside Rg3 attenuates the phosphorylation of ERK and p38 protein levels but not JNK, as demonstrated by western blotting analysis using the outgrowth ECs. The total ERK, JNK, p38 and β-actin were probed as a protein loading control. All data was obtained from at least three independent experiments. (C) The graph represents the relative optical density of each group via the ERK, p38, JNK control group. The bar graph indicating each percentage was expressed using the untreated groups as 100%. *p < 0.05, **p < 0.005.
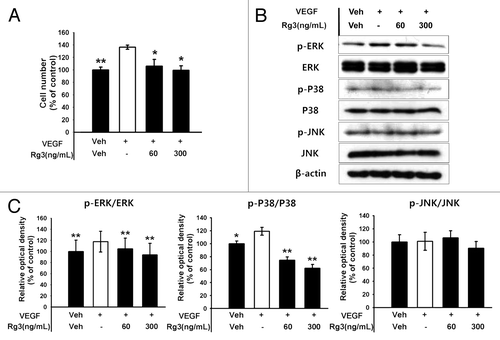
Effect of Ginsenoside Rg3 on cell cycle progression
To further determine if Ginsenoside Rg3 affects the pivotal proteins for cell cycle progression in EPCs, cell cycle signaling molecules involved in the G1 phase were further assessed by western blotting analysis. As shown in and Figure S4B, Ginsenoside Rg3 profoundly inhibited the accumulation of cyclin D1, cyclin E and Cdk2, but not Cdk4, in response to VEGF signals, suggesting that the delayed accumulation of cycline E and Cdk2 in G1 phase progression by a treatment of Ginsenoside Rg3 might affect the level of VEGF dependent EPC proliferation,
Figure 4. Effect of Ginsenoside Rg3 on cell cycle progression in EPCs. (A) The cell cycle progression was analyzed by western blotting analysis using multiple antibodies against pivotal cell cycle molecules. (B) The graph represents the relative optical density of each group via the β-actin control group. The bar graph indicating each percentage was expressed using the untreated groups as 100%. *p < 0.05, **p < 0.005.
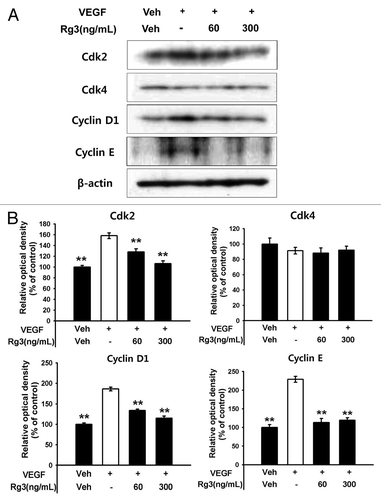
Effect of Ginsenoside Rg3 on VEGF-induced migratory capacity and tubular formation bioactivity of EPCs
As the cell migration properties during tumor angiogenesis is the one of major events underlying tubular formation, a pivotal process of neovessel formation, the effect of Ginsenoside Rg3 on the migratory capacity of EPCs was first examined using a wound healing assay. As predicted, the VEGF-treated EPCs facilitated the repair of the wounded monolayer ( and Fig. S5A). In particular, Ginsenoside Rg3 blocked the VEGF-induced wound healing capability of EPCs in a dose-dependent manner, suggesting that Ginsenoside Rg3 affects the migratory ability of EPCs directly during vascular repair ().
Figure 5. Effect of Ginsenoside Rg3 on the ability of migration of EPCs. (A) Representative optical micrographs showing wound healing by monolayers. Inhibitive effect of Rg3 on VEGF induced outgrowth ECs migration. Ex vivo cultured outgrowth ECs were subjected to wound healing migration assay. Bar, 500 μm. (B) Bar graph represents the number of migrated cells. Fields were chosen randomly from various section levels to ensure the objectivity of sampling. *significance between the control and VEGF, p < 0.05, significance between VEGF and VEGF plus Ginsenoside Rg3, p < 0.05.
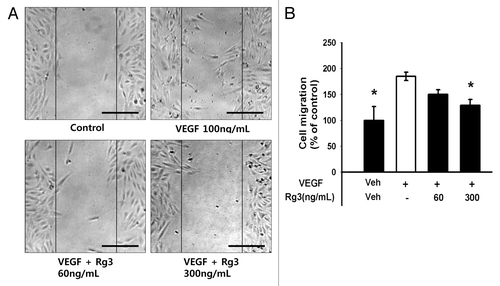
Since Ginsenoside Rg3 can affect the functional tubular formation capability of EPCs, the tube forming assay was next performed after the activation of EPCs with increasing concentrations of Ginsenoside Rg3. After 6 h, significant increase in the capillary-like tubular structures was observed in EPC control group. On the other hand, the Ginsenoside Rg3-treated EPCs group showed a significant disruption of the ability of EPCs to form tubes (), as demonstrated by the random counting of both branches and tube lengths. This suggests that Ginsenoside Rg3-treated EPCs revealed a significant functional defect in tubular formation ().
Figure 6. Effect of Ginsenoside Rg3 on the ability of tube formation of EPCs. (A) Representative tubular network structure in the outgrowth ECs with or without Ginsenoside Rg3. Bar, 500 μm. (B, C) Significant differences between Rg3-treated outgrowth ECs and vehicle-treated outgrowth ECs. The bar graph represents the number of intact loops in the capillary networks, as measured by image-J software. The graph represents the length of the tube in the capillary networks (*p < 0.05, **p < 0.005).
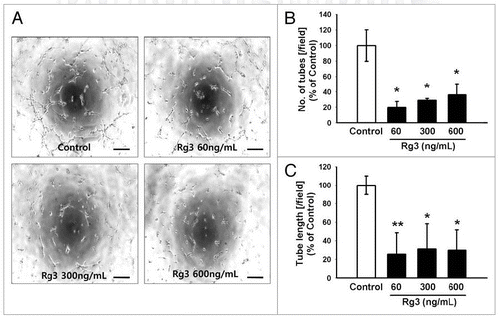
Effect of Ginsenoside Rg3 on tumor angiogenesis and tumor growth
A murine LLC tumor xenograft experiment was performed to clarify whether Ginsenoside Rg3 inhibits EPC-derived microvessels in tumor progression (). At the time of sacrifice, all the mice treated with the vehicle only had a large tumor volume reaching 227 ± 24 mm3. A significant decrease in swelled tumor mass (103 ± 58 mm3) was observed when LLC cells (5 × 104) were injected into a mouse flank together with Ginsenoside Rg3 (). Therefore, the Ginsenoside Rg3 treatment decreased the level of tumor growth by more than 50% compared with the tumor and vehicle injected group.
Figure 7. Effect of Ginsenoside Rg3 on tumor angiogenesis and tumor growth. (A) After s.c. injection with 5 × 104 LLC tumor cells, the mice were administered orally with the DDW vehicle (control) or 600 μg/kg Ginsenoside Rg3 daily for 23 d after the initiation of therapy (n = 5). The mice were sacrificed at day 24 because the mice treated with the vehicle only had large tumors. (B) The vehicle or Ginsenoside Rg3 (600 μg/kg) was administered orally everyday using protocols of oral administration injection. Tumor growth was measured with calipers every 3 or 4 d using the formula V = height ×length × depth (mm3). Tumor depth is defined as the horizontal extent of tumor’s lateral part. Bar, 1 cm. There is significant difference between tumor group and Rg3 treated tumor group at day 24. (C) Representative photomicrographs of CD31 capillaries (5~20 μm in diameter) and arterials (150~500 μm in diameter) in tumor sections of LLC bearing mice. Bar, 500 μm. (D, E) The number of CD31-stained capillary expressing arterial and capillary were counted using image J program. The fields were chosen randomly from various section levels to ensure the objectivity of sampling. *p < 0.05.
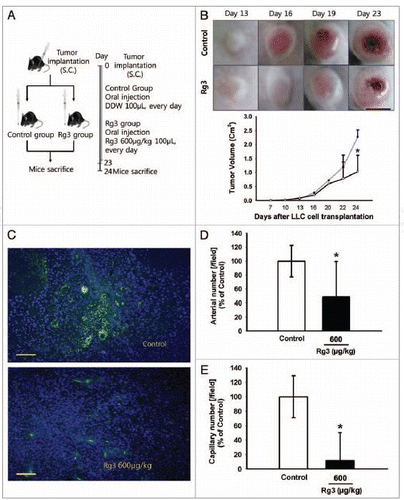
Next, the capillary density of both tumor and vehicle group and tumor and Ginsenoside Rg3 group was examined As shown in , the Rg3 treated group showed much less blood vessel formation at a dose of 600 μg/kg than the vehicle only injected group in the LLC tumor bearing mice. CD31-positive endothelial cells were detected much less of the tumor tissue of the Ginsenoside Rg3 treatment group, as demonstrated by the arterial and capillary density in each group. In particular, the number of arterial and capillary vessels was reduced significantly in the Rg3 treated group ().
Effect of Ginsenoside Rg3 on EPC mobilization step
To examine the effect of Ginsenoside Rg3 on the mobilization kinetics of EPCs, flow cytometry analysis was performed at 5 d after the oral administration of Ginsenoside Rg3 daily in LLC tumor bearing mice (). The sub-fraction of CD34(+)/VEGFR2(+) cells was assessed using isotype IgG negative antibodies in a gated fraction of CD45(-) cells () in PB-derived mononuclear cells. The circulating CD45(-)/CD34(+)/VEGFR2(+) cells represent an increased expression pattern of LLC tumor bearing mice, but there was little expression in the vehicle only group. As shown in , there was significant difference in the number of circulating CD45(-)/CD34(+)/VEGFR2(+) cells between the tumor group and Rg3-treated tumor group, suggesting that Ginsenoside Rg3 might modulate the BM microenvironment and regulate the number or bioactivities of EPCs after induction of LLC tumor in mice.
Figure 8. Effect of Ginsenoside Rg3 on EPC mobilization. (A) After s.c. injection with 5 × 104 LLC, the mice were administered orally with DDW vehicle (control) or 600 μg/kg Ginsenoside Rg3 daily for 4 d after the initiation of therapy. The circulating MNCs was harvested at day 5 by Ficoll-gradient centrifugation. (B) Subsequent gating procedure was used to deplete the CD45 hematopoietic cells. Double positive fraction for detecting CD34+VEGFR2+ cells in the gated CD45- cell population (subsets represented in the upper-right quadrant) was expressed as circulating progenitor cells (EPCs) in murine PB. This figure means negative control and positive control. (C) Statistical difference between Ginsenoside after the oral administration of both Rg3 and vehicle in LLC tumor bearing mice (n = 5). The bar graph represents a marked difference in the EPC frequency at day 5 after daily injection of Rg3 or the vehicle. *p < 0.05.
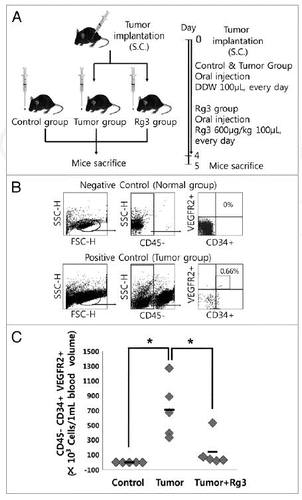
Effect of Ginsenoside Rg3 on VEGF-induced neovessel formation
Ginsenoside Rg3 may control VEGF-induced tumor angiogenesis considering that both recruiting steps into ischemic tissue and BM mobilization steps into PB are generally initiated by VEGF signaling. To test this hypothesis, a matrigel plug assay in mice was performed to examine the inhibitory effect of Ginsenoside Rg3 on neovessel formation. As shown in , dark red in plugs were observed with VEGF, but a significant decrease in the red color was noted in the plugs with Ginsenoside Rg3 and VEGF group, indicating that a treatment with Ginsenoside Rg3 resulted in a decrease in blood vessel formation compared with the VEGF-treated plugs. To further test this hypothesis, the matrigel plug tissue was by staining with CD31, a typical endothelial marker, to visualize the infiltrating and differentiated endothelial cells. As shown in and Figure S5B, the number of CD31-positive endothelial cells was much lower in the plugs with both VEGF and Ginsenoside Rg3 than in the VEGF-only group.
Figure 9.Ginsenoside Rg3 inhibits VEGF-induced angiogenesis in vivo. (A) Experimental protocols of VEGF dependent matrigel plug assay to examine the effect of Ginsenoside Rg3 on neovessel formation. (B) Representative photomicrographs of CD31 stained matrigel sections of mice treated with the vehicle, VEGF (100 ng/mL in matrigel) and various doses of Ginsenoside Rg3 (150 ng/mL and 600 ng/mL) with VEGF (100 ng/mL). Bar, 500 μm. (C) The number of capillaries was counted using the image J program. The fields were chosen randomly from various section levels to ensure the objectivity of sampling. The graph represents the number of CD31 positive capillaries (*p < 0.05). Percent inhibition was expressed using untreated groups as 100% (n = 5).
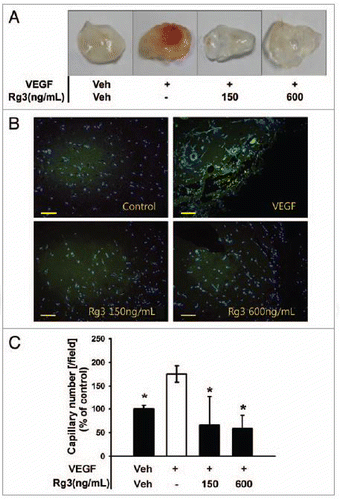
Discussion
The neovessels during tumor progression are formed mainly from the preexisting vessels by the proliferation and migration of adult endothelial cells, which appear to be promoted by recruited BM-derived stem/progenitor cells in hypoxic tumors.Citation32,Citation33 Of particular interest are BM-derived EPCs, because they have been reported to play a key role in the growth of early tumors and metastatic lesions by mediating the angiogenic switch.Citation7-Citation9 EPCs have also been proposed to supply an alternative source of tumor endothelium, and consequently contribute to angiogenesis by directly incorporating them into the nascent vasculature as differentiated endothelial cells.Citation10,Citation11 Therefore, the specific targeting of EPC bioactivities appears to be one of potent targets for antiangiogenic therapy without side effects.Citation7-Citation9,Citation34 The present data showed that the major biologically active component of the natural medicine of Ginseng, Ginsenoside Rg3, attenuates tumor growth and neovessel formation by targeting functional activities of the resident EPCs. Based on these results, it was concluded that Ginsenoside Rg3 effectively inhibits the multiple bioactivities of EPCs including cell proliferation in response to VEGF signaling, migratory capacity including bone marrow mobilization into the peripheral blood, and the promotion of tubular formation, as well as suppressing the effect of tumor angiogenesis and tumor growth, as demonstrated in the in vivo xenograft murine tumor model.
During tumor progression, VEGF signaling is tightly involved in the onset of the tumor vasculatures and tumor growth, contributing significantly to pathological angiogenesis, eventually leading to accelerated growth rates of tumors, invasion and metastasis.Citation22,Citation35 Importantly, these results suggest that Ginsenoside Rg3 inhibits angiogenesis by suppressing the VEGF-VEGFR2 signaling cascade of EPCs. Ginsenoside Rg3 decreases the expression of VEGFR2 at the RNA and protein levels in EPCs and abrogated the VEGF-dependent multiple signal cascades, underlying the cell proliferation signals, as demonstrated by the in vitro western blotting assay. Similarly, the in vivo matrigel plug assay indicated that Rg3 significantly inhibits VEGF-induced angiogenesis effectively.
In addition to the direct effects for the nascent vasculature of Ginsenoside Rg3 during tumor progression, these results support the hypothesis that Ginsenoside Rg3 may suppress the mobilization of BM-derived EPCs.Citation36 As the precise control of progenitor cells during tumor progression is critical for inhibiting tumor growth and tumor vasculature, a significant decrease in peripheral progenitors by the oral administration of Ginsenoside Rg3 provides a clear explanation of tumor angiogenesis, including a dramatic decrease in tumor capillary growth in solid tumors in the in vivo xenograft murine tumor model.
The data suggests that Ginsenoside Rg3 suppresses the multiple signal cascades including VEGF dependent p38 and ERK signal pathways. The VEGF-dependent ERK signal cascade appears to be involved in cell proliferation, survival and cell migration.Citation29 As for the EPC biology, adequate modulation of the VEGF dependent ERK signals may be a therapeutic target for abrogating tumor angiogenesis. Importantly, these results suggest that Ginsenoside Rg3 attenuates the phosphorylation cascade of VEGF dependent p38 signals, indicating that Ginsenoside Rg3 affects multiple VEGF-mediated downstream signal cascades. This data are clearly supported by an analysis of cell cycle modulators including cyclin E and Cyclin D1, as Rg3 inhibits cell cycle progression by suppressing cyclin E and Cyclin D1. Interestingly, an analysis of the VEGF dependent cell cycle of EPCs showed that Ginsenoside Rg3 inhibits the accumulation of Cdk2 but not Cdk4. Previous studies reported that the Cyclin D/Cdk4/6 axis specifically phosphorylates pRB at a subset of its phosphorylation sites, even though the complete phosphorylation of pRB requires cyclin E-Cdk2 to specifically target the remaining phosphorylation sites.Citation37 Therefore, the present finding that the inhibition of Cdk2 by Ginsenoside Rg3 led to a blockade of the G1 stage in cell cycle progression might provide a strong correlation with the delayed progression of the second step of VRGF dependent pRB phosphorylation in Ginsenoside Rg3 treated EPCs.Citation38,Citation39
Overall, this study showed that Ginsenoside Rg3 inhibited EPC proliferation, migration, tube formation, and effectively abrogated the VEGF dependent neovessel formation in vivo and attenuated EPC mobilization, leading to delayed tumor progression and tumor angiogenesis. These results provide strong evidence that Ginsenoside Rg3 is a potential drug candidate for cancer chemotherapy with low chemotoxic side effects (). The finding of a natural product showing a synergistic effect in both a direct abrogating effect on endothelial cells in tumor vessel and adequate inhibition of vascular promoting cells bioactivities, would provide an promising approach for the inhibition of tumor growth and neovessel formation. In the future, further experiments underlying the further molecular mechanism of Ginsenoside Rg3 in the EPC biology in various tumors will help determine of the roles of EPC bioactivities in controlling tumor angiogenesis, angiogenic switch and tumor metastasis.
Materials and Methods
Drugs
Ginsenoside Rg3 was purchased from BTGin Co., Ltd (Okcheon). A solution of Rg3 was freshly prepared in DMSO (10 mg/ml). shows the chemical structure of Ginsenoside Rg3.
Isolation of human outgrowth ECs and cell culture
HUCB was supplied by the Pusan National University Hospital. Freshly isolated MNCs were cultured in endothelial culture media (EGM-2, Lonza) supplemented with 5% fetal bovine serum (FBS), angiogenic growth factors (10 ng/mL bFGF, 20 ng/mL VEGF, 10 ng/mL IGF and 10 ng/mL EGF) and other factors, such as ascorbic acid, GA-1000. After 4 d of culture, the non-adherent cells were removed, and the tightly attached fraction of cells were re-plated and cultured for a further 3 d, and long time culture was continued using spindle-shaped colonies for 14–21 d by refreshing the EGM-2 media. The endothelial characteristics of the attached spindle-shaped cells were examined after the uptake of Dil-conjugated Ac-LDL(Dil-Ac-LDL)(Biomedical Technologies Inc.) and chemical binding with FITC-conjugated isolectin B4 (Sigma Chemical Co.), a standard marker of endothelial lineage cells. The EPCs were identified as double positive cells by fluorescence microscopy (data not shown). Immunohistochemistry showed that the ex vivo cultured outgrowth EC expresses several endothelial lineage markers, including CD31, KDR/Flk-1, as well as several progenitor surface markers, including CD34, c-kit and CD133, and a pivotal functional marker, CXCR4, a receptor of SDF-1, which is involved in homing the outgrowth EPC in ischemic sites ().
Cytotoxicity test
To determine the cytotoxicity of Ginsenoside Rg3 in ex vivo cultured outgrowth EC, the cells were seeded (1 × 104cells/well) onto gelatin-coated, 96-well culture plates in EGM-2 medium, supplemented with 5% fetal bovine serum (FBS), angiogenic growth factors. Ginsenoside Rg3 was added at a concentration of 60 ng/mL~600 ng/mL for 24, 48, 72 h. The cytotoxicity was evaluated by the addition of 10 μl/well of WST-1(Ez-cytox cell viability assay kit). After 4 h, the optical densities were measured at 540 nm using a microplate reader (TECAN, Sunrise)
Proliferation Assay
Ex vivo cultured outgrowth ECs (1 × 104 cells/well) were plated onto gelatin-coated, 96-well plates. After 24 h, the cells were serum starved in EBM-2 medium supplemented with 0.1% FBS for 24 h. They were then incubated with various Ginsenoside Rg3 concentrations in the presence of 100 ng/mL VEGF for 48 h. The viable cells were analyzed using WST-1 assay (Ez-cytox cell viability assay kit).
Wound healing assay
Ex vivo cultured outgrowth ECs (1.5 × 105 cells/well) and LLCs were starved with EBM-2 medium or DMEM containing 0.1% FBS for 24 h. For the wound healing assays, the monolayers grown on 24-well plates were wounded with a micropipette tip. The cells were washed to remove the debris, incubated for 8 h in low-serum media containing different Ginsenoside Rg3 concentrations and 100 ng/mL VEGF, and observed by optical microscopy.
Tube formation assay
Ex vivo cultured outgrowth ECs (2 × 104 cells/well) and LLCs (2 × 104 cells/well) were seeded on a 96-well culture plate coated with 55 μl per well of matrigel matrix (BD, 10ml vial) for 30 min at 37°C. The plates were examined for tube formation after 6h incubation. After incubation, the level of tubular formation was determined, after randomly taking a picture per well at ×40 magnification by optical microscopy. The tube length was measured using image-J software (http://rsb.info.nih.gov).
Flow cytometric analysis
To examine the number of EPCs in the Rg3-treated LLC tumor bearing mice, flow cytometry analysis was performed using several monoclonal antibodies specifically against CD45, CD34 and Flk-1. Briefly, the freshly isolated MNCs were incubated with directly labeled allophycocyanin (APC)-conjugated anti-mouse CD45 (BD PharMingen), fluorescein isothiocyanate (FITC)-conjugated anti-mouse CD34 (BD PharMingen) and Phycoerythrin (PE)-conjugated anti-mouse Flk-1 (BD PharMingen) antibodies for 30 min on ice. In addition, to examine the number of apoptotic cells in Ginsenoside Rg3-treated groups, the flow cytometry analysis was performed using Annexin V/PI system (BD PharMingen). The immunofluorescence-labeled cells were fixed with 2% paraformaldehyde and analyzed by FACS flow cytometry (Becton Dickinson) and Cell Quest Software counting 10,000 events per sample.
Reverse transcription (RT)-PCR
The total RNA was isolated from the EPCs using the TRIZOL reagent (TaKaRa) according to the manufacturer’s recommendations. RT-PCR was performed as detailed previously.Citation40 The primer pair sequences for the amplification of human VEGFR2 mRNA were as follows: 5′-GTGACCAACATGGAGTCGTG-3′ and 5′-TGCTTCACAGAAGACCATGC-3′. The samples were first denatured at 94°C for 5 min and amplified for 30 cycles (94°C 30 sec, 50°C 30 sec, 72°C 30 sec) and a final extension at 72°C for 5 min. The PCR products were run on 1% agarose gel.
RT-quantitative PCR
The total RNA was isolated from the EPCs using the TRIZOL reagent (TaKaRa) according to the manufacturer’s recommendations and 1 µg of total RNA was used to synthesize first-strand cDNA by PrimeScript II 1st strand cDNA Synthesis Kit (TaKaRa), in accordance with the manufacturer’s instructions. RT-qPCR was performed using a 7500 real time system (Applied Biosystems) and the Power SYBR Green PCR Master Mix (Applied Biosystems). The PCR reaction volume was 20 µL containing 1 µL of diluted cDNA and 10 µM of each primer. PCR conditions were set as an initial polymerase activation step for 2 min at 95°C, followed by 40 cycles of 15 sec at 94°C for template denaturation, 15 sec at 60°C for annealing and 20 sec at 72°C for extension and fluorescence measurement. In addition, each PCR reaction included a reverse transcription negative control to check for potential genomic DNA contamination. Reagent contamination was also detected by a reaction mix without template. All samples were amplified in triplicates and the mean was used for RT-qPCR analysis. The CT method (Livak and Schmittgen, 2001) was applied to quantify the relative cDNA amount and β-actin level was used as internal control.
The sequence of primers were as follows: forward 5′-CCAGTCAGAGACCCACGTTT-3′, reverse 5′-AGTCTTTGCCATCCTGCTGA-3′ for human VEGFR2; forward 5′- AACACCCCAGCCAT GTACG-3′, reverse 5′-ATGTCACGCACGATTTCCC-3′ for human β-actin.
Western blot analysis
The proteins were separated by SDS-PAGE and transferred to a membrane (MILLIPORE, Immobilon-P). The membranes were blocked in a blocking solution (tris-buffered saline, TBS) containing 0.1% (v/v) Tween-20 and 5% (v/v) non-fat skim milk for 1 h and incubated overnight with anti-VEGFR2(1:1,000), anti-Cleaved Caspase-3(1:1,000), anti-phospho-ERK1/2(1:1,000), anti-ERK1/2(1:1,000), anti-p38(1:1,000), antibodies (Cell Signaling Technology), or anti-Flk-1(1:1,000), anti-p-JNK(1:1,000), anti-JNK(1:1,000), anti-p-p38(1:1,000), anti-Cdk2(1:1,000), anti-Cdk4(1:1,000), anti-Cyclin D1(1:1,000), anti-Cyclin E(1:1,000), anti-β-actin(1:1,000) antibodies (Santa Cruz Biotechnology). The membranes were washed extensively in Tris-buffered saline containing 0.1% (v/v) Tween-20 before incubation for 1 h with the secondary anti-mouse (1:1,000) or anti-rabbit (1:3,000) antibodies conjugated to horseradish peroxidase. After washing with PBS, the protein was visualized using an ECL solution (GE Healthcare) and X-ray film.
Lewis lung carcinoma (LLC) cell culture and transplantation
The LLC was maintained in Dulbeccos modified Eagles medium (DMEM, WelGENE) supplemented with 10% FBS and 1% antibiotics. C57BL/6 mice were inoculated intradermally with 5 × 104 LLC and the tumor size was monitored [Volume = height × length × depth (cm3)].
LLC xenograft mouse model
The 7-week-old male C57BL/6 mice were divided into groups (five mice each group). The LLC cells were injected s.c. (5 × 104 per mouse) into the mice. After the s.c. injection, the mice were orally administrated with or without 600 μg/kg of Ginsenoside Rg3 every day. The tumor sizes were recorded every three days, and the tumor size was determined using Vernier calipers. After 24 d, the mice with s.c. tumors were sacrificed.
Immunofluorescence analysis
The tumor tissue from the mice was removed immediately on day 24 of Rg3 treatment, fixed in 4% paraformaldehyde (in PBS) overnight at 4°C and embedded in paraffin. Serial sections (5 μm) were cut through the entire tumor tissue, mounted on poly-l-lysine microscope slides, and processed for immunohistochemistry after deparaffinizing three times in xylene for 4 min each. After passing through a decreasing concentration of ethanol, the sections were stained with the antibody against CD31 (BD, Nj) at 4°C overnight. Immunostaining was visualized using the appropriate Alexa 488-conjugated secondary antibody (Invitrogen) with a fluorescence microscope.
In vivo matrigel plug assay
A matrigel plug assay was performed as described previously.Citation41 Briefly, GFR-matrigel (0.6 ml) containing 20U of heparin and 300ng of mouse VEGF with or without Rg3 (150 ng/mL and 600 ng/mL) were injected subcutaneously into the 7-week-old male C57BL/6 mice (5 mice for each group). After 10 d, the plugs were removed. For immunohistochemistry, the plugs were fixed in 4% PFA and embedded with paraffin. The sections were stained with the anti-CD31 antibody to visualize the infiltrating and differentiated endothelial cells. The number of blood vessels was counted using an image J program. The fields were chosen randomly from various section levels to ensure the objectivity of sampling.
Statistical analysis
A statistical comparison of the two groups was performed using a Students t-test. These results were also analyzed statistically using the Statview 5.0 software package (Abacus Concepts, Inc.). A Scheffés test was performed for multiple comparisons after ANOVA between each group. All data, which was obtained from at least three independent experiments, is expressed as the means ± standard deviations. *p < 0.05, **p < 0.005 were considered significant.
Additional material
Download Zip (902.5 KB)Acknowledgments
This study was supported by National Research Foundation grant funded by the Korea government (2010-0020260) and a grant of the Korean Health Technology R&D Project, Ministry of Health and Welfare, Republic of Korea. (A100322).
Disclosure of Potential Conflicts of Interest
No potential conflicts of interest were disclosed.
References
- Cooney MM, van Heeckeren W, Bhakta S, Ortiz J, Remick SC. Drug insight: vascular disrupting agents and angiogenesis--novel approaches for drug delivery. Nat Clin Pract Oncol 2006; 3:682 - 92; http://dx.doi.org/10.1038/ncponc0663; PMID: 17139319
- Bergers G, Benjamin LE. Tumorigenesis and the angiogenic switch. Nat Rev Cancer 2003; 3:401 - 10; http://dx.doi.org/10.1038/nrc1093; PMID: 12778130
- Skowasch D, Jabs A, Lüderitz B, Bauriedel G. Bone marrow-derived cells and vascular growth. Circ Res 2004; 94:e71; http://dx.doi.org/10.1161/01.RES.0000126418.31311.F8; PMID: 15117831
- Du R, Lu KV, Petritsch C, Liu P, Ganss R, Passegué E, et al. HIF1alpha induces the recruitment of bone marrow-derived vascular modulatory cells to regulate tumor angiogenesis and invasion. Cancer Cell 2008; 13:206 - 20; http://dx.doi.org/10.1016/j.ccr.2008.01.034; PMID: 18328425
- Zheng H, Fu G, Dai T, Huang H. Migration of endothelial progenitor cells mediated by stromal cell-derived factor-1alpha/CXCR4 via PI3K/Akt/eNOS signal transduction pathway. J Cardiovasc Pharmacol 2007; 50:274 - 80; http://dx.doi.org/10.1097/FJC.0b013e318093ec8f; PMID: 17878755
- Shojaei F, Wu X, Qu X, Kowanetz M, Yu L, Tan M, et al. G-CSF-initiated myeloid cell mobilization and angiogenesis mediate tumor refractoriness to anti-VEGF therapy in mouse models. Proc Natl Acad Sci U S A 2009; 106:6742 - 7; http://dx.doi.org/10.1073/pnas.0902280106; PMID: 19346489
- Lyden D, Hattori K, Dias S, Costa C, Blaikie P, Butros L, et al. Impaired recruitment of bone-marrow-derived endothelial and hematopoietic precursor cells blocks tumor angiogenesis and growth. Nat Med 2001; 7:1194 - 201; http://dx.doi.org/10.1038/nm1101-1194; PMID: 11689883
- Nolan DJ, Ciarrocchi A, Mellick AS, Jaggi JS, Bambino K, Gupta S, et al. Bone marrow-derived endothelial progenitor cells are a major determinant of nascent tumor neovascularization. Genes Dev 2007; 21:1546 - 58; http://dx.doi.org/10.1101/gad.436307; PMID: 17575055
- Gao DC, Nolan DJ, Mellick AS, Bambino K, McDonnell K, Mittal V. Endothelial progenitor cells control the angiogenic switch in mouse lung metastasis. Science 2008; 319:195 - 8; http://dx.doi.org/10.1126/science.1150224; PMID: 18187653
- Kopp HG, Ramos CA, Rafii S. Contribution of endothelial progenitors and proangiogenic hematopoietic cells to vascularization of tumor and ischemic tissue. Curr Opin Hematol 2006; 13:175 - 81; http://dx.doi.org/10.1097/01.moh.0000219664.26528.da; PMID: 16567962
- Asahara T, Takahashi T, Masuda H, Kalka C, Chen DH, Iwaguro H, et al. VEGF contributes to postnatal neovascularization by mobilizing bone marrow-derived endothelial progenitor cells. EMBO J 1999; 18:3964 - 72; http://dx.doi.org/10.1093/emboj/18.14.3964; PMID: 10406801
- Gasparini G, Longo R, Toi M, Ferrara N. Angiogenic inhibitors: a new therapeutic strategy in oncology. Nat Clin Pract Oncol 2005; 2:562 - 77; http://dx.doi.org/10.1038/ncponc0342; PMID: 16270097
- Kerr DJ. Targeting angiogenesis in cancer: clinical development of bevacizumab. Nat Clin Pract Oncol 2004; 1:39 - 43; http://dx.doi.org/10.1038/ncponc0026; PMID: 16264798
- Perabo FG, Von Löw EC, Ellinger J, von Rücker A, Müller SC, Bastian PJ. Soy isoflavone genistein in prevention and treatment of prostate cancer. Prostate Cancer Prostatic Dis 2008; 11:6 - 12; http://dx.doi.org/10.1038/sj.pcan.4501000; PMID: 17923857
- Wong VK, Cheung SS, Li T, Jiang ZH, Wang JR, Dong H, et al. Asian ginseng extract inhibits in vitro and in vivo growth of mouse lewis lung carcinoma via modulation of ERK-p53 and NF-κB signaling. J Cell Biochem 2010; 111:899 - 910; http://dx.doi.org/10.1002/jcb.22778; PMID: 20665661
- Surh YJ, Na HK, Lee JY, Keum YS. Molecular mechanisms underlying anti-tumor promoting activities of heat-processed Panax ginseng C.A. Meyer. J Korean Med Sci 2001; 16:Suppl S38 - 41; PMID: 11748375
- Liu CX, Xiao PG. Recent advances on ginseng research in China. J Ethnopharmacol 1992; 36:27 - 38; http://dx.doi.org/10.1016/0378-8741(92)90057-X; PMID: 1501490
- Zhang QY, Kang XM, Zhao WH. Antiangiogenic effect of low-dose cyclophosphamide combined with ginsenoside Rg3 on Lewis lung carcinoma. Biochem Biophys Res Commun 2006; 342:824 - 8; http://dx.doi.org/10.1016/j.bbrc.2006.02.044; PMID: 16499874
- Yi C, Huang XB, Hou M. [Experimental study on effect of chemotherapy combined ginsengnoside Rg3 in treating pulmonary carcinoma]. Zhongguo Zhong Xi Yi Jie He Za Zhi 2005; 25:58 - 9; PMID: 15719754
- Iishi H, Tatsuta M, Baba M, Uehara H, Nakaizumi A, Shinkai K, et al. Inhibition by ginsenoside Rg3 of bombesin-enhanced peritoneal metastasis of intestinal adenocarcinomas induced by azoxymethane in Wistar rats. Clin Exp Metastasis 1997; 15:603 - 11; http://dx.doi.org/10.1023/A:1018491314066; PMID: 9344044
- Liu JW, Chen JX, Yu LH, Tian YX, Cui XY, Yan Q, et al. [Inhibitory effect of ginsenoside-Rg3 on lung metastasis of mouse melanoma transfected with ribonuclease inhibitor]. Zhonghua Zhong Liu Za Zhi 2004; 26:722 - 5; PMID: 15733388
- Nagy JA, Chang SH, Dvorak AM, Dvorak HF. Why are tumour blood vessels abnormal and why is it important to know?. Br J Cancer 2009; 100:865 - 9; http://dx.doi.org/10.1038/sj.bjc.6604929; PMID: 19240721
- Ferrara N.. Vascular endothelial growth factor and the regulation of angiogenesis. Recent Prog Horm Res 2000; 55:15 - 35
- Ivy SP, Wick JY, Kaufman BM. An overview of small-molecule inhibitors of VEGFR signaling. Nat Rev Clin Oncol 2009; 6:569 - 79; http://dx.doi.org/10.1038/nrclinonc.2009.130; PMID: 19736552
- Grothey A, Galanis E. Targeting angiogenesis: progress with anti-VEGF treatment with large molecules. Nat Rev Clin Oncol 2009; 6:507 - 18; http://dx.doi.org/10.1038/nrclinonc.2009.110; PMID: 19636328
- Iwaguro H, Yamaguchi J, Kalka C, Murasawa S, Masuda H, Hayashi S, et al. Endothelial progenitor cell vascular endothelial growth factor gene transfer for vascular regeneration. Circulation 2002; 105:732 - 8; http://dx.doi.org/10.1161/hc0602.103673; PMID: 11839630
- Shaked Y, Ciarrocchi A, Franco M, Lee CR, Man S, Cheung AM, et al. Therapy-induced acute recruitment of circulating endothelial progenitor cells to tumors. Science 2006; 313:1785 - 7; http://dx.doi.org/10.1126/science.1127592; PMID: 16990548
- Somanath PR, Razorenova OV, Chen JH, Byzova TV. Akt1 in endothelial cell and angiogenesis. Cell Cycle 2006; 5:512 - 8; http://dx.doi.org/10.4161/cc.5.5.2538; PMID: 16552185
- Murphy DA, Makonnen S, Lassoued W, Feldman MD, Carter C, Lee WMF. Inhibition of tumor endothelial ERK activation, angiogenesis, and tumor growth by sorafenib (BAY43-9006). Am J Pathol 2006; 169:1875 - 85; http://dx.doi.org/10.2353/ajpath.2006.050711; PMID: 17071608
- Fang J, Zhou Q, Liu LZ, Xia C, Hu X, Shi X, et al. Apigenin inhibits tumor angiogenesis through decreasing HIF-1alpha and VEGF expression. Carcinogenesis 2007; 28:858 - 64; http://dx.doi.org/10.1093/carcin/bgl205; PMID: 17071632
- Yi T, Cho SG, Yi Z, Pang X, Rodriguez M, Wang Y, et al. Thymoquinone inhibits tumor angiogenesis and tumor growth through suppressing AKT and extracellular signal-regulated kinase signaling pathways. Mol Cancer Ther 2008; 7:1789 - 96; http://dx.doi.org/10.1158/1535-7163.MCT-08-0124; PMID: 18644991
- Dvorak HF. Angiogenesis: update 2005. J Thromb Haemost 2005; 3:1835 - 42; http://dx.doi.org/10.1111/j.1538-7836.2005.01361.x; PMID: 16102050
- Asahara T, Masuda H, Takahashi T, Kalka C, Pastore C, Silver M, et al. Bone marrow origin of endothelial progenitor cells responsible for postnatal vasculogenesis in physiological and pathological neovascularization (vol 85, pg 221, 1999). Circ Res 1999; 85:477
- Oh HK, Ha JM, O E, Lee BH, Lee SK, Shim BS, et al. Tumor angiogenesis promoted by ex vivo differentiated endothelial progenitor cells is effectively inhibited by an angiogenesis inhibitor, TK1-2. Cancer Res 2007; 67:4851 - 9; http://dx.doi.org/10.1158/0008-5472.CAN-06-2979; PMID: 17510415
- Kerbel RS. Antiangiogenic therapy: a universal chemosensitization strategy for cancer?. Science 2006; 312:1171 - 5; http://dx.doi.org/10.1126/science.1125950; PMID: 16728631
- Nishimura H, Asahara T. Bone marrow-derived endothelial progenitor cells for neovascular formation. EXS 2005; 21:147 - 54; http://dx.doi.org/10.1007/3-7643-7311-3_10; PMID: 15617476
- Ohtsubo M, Theodoras AM, Schumacher J, Roberts JM, Pagano M. Human cyclin E, a nuclear protein essential for the G1-to-S phase transition. Mol Cell Biol 1995; 15:2612 - 24; PMID: 7739542
- Ezhevsky SA, Nagahara H, Vocero-Akbani AM, Gius DR, Wei MC, Dowdy SF. Hypo-phosphorylation of the retinoblastoma protein (pRb) by cyclin D:Cdk4/6 complexes results in active pRb. Proc Natl Acad Sci U S A 1997; 94:10699 - 704; http://dx.doi.org/10.1073/pnas.94.20.10699; PMID: 9380698
- Lundberg AS, Weinberg RA. Functional inactivation of the retinoblastoma protein requires sequential modification by at least two distinct cyclin-cdk complexes. Mol Cell Biol 1998; 18:753 - 61; PMID: 9447971
- Guo J, Schally AV, Zarandi M, Varga J, Leung PC. Antiproliferative effect of growth hormone-releasing hormone (GHRH) antagonist on ovarian cancer cells through the EGFR-Akt pathway. Reprod Biol Endocrinol 2010; 8:54; http://dx.doi.org/10.1186/1477-7827-8-54; PMID: 20509930
- Yue PY, Wong DY, Ha WY, Fung MC, Mak NK, Yeung HW, et al. Elucidation of the mechanisms underlying the angiogenic effects of ginsenoside Rg(1) in vivo and in vitro. Angiogenesis 2005; 8:205 - 16; http://dx.doi.org/10.1007/s10456-005-9000-2; PMID: 16328162