Abstract
FP3 is an engineered protein which contains the extracellular domain 2 of VEGF receptor 1 (Flt-1) and extracellular domain 3 and 4 of VEGF receptor 2 (Flk-1, KDR) fused to the Fc portion of human immunoglobulin G1. Previous studies demonstrated its antiangiogenic effects in vitro and in vivo, and its antitumor activity in vivo. In this study, patient-derived tumor tissue (PDTT) xenograft models of primary colon carcinoma and lymphatic and hepatic metastases were established for assessment of the antitumor activity of FP3 in combination with capecitabine. Xenografts were treated with FP3, capecitabine, alone or in combination. After tumor growth was confirmed, volume and microvessel density in tumors were evaluated. Levels of VEGF, and PCNA in the tumor were examined by immunohistonchamical staining, level of thymidine phosphorylase (TP) was examined by ELISA, and levels of related cell signaling pathways proteins expression were examined by western blotting. FP3 in combination with capecitabine showed significant antitumor activity in three xenograft models (primary colon carcinoma, lymphatic metastasis, and hepatic metastasis). The microvessel density in tumor tissues treated with FP3 in combination with capecitabine was lower than that of the control. Antitumor activity of FP3 in combination with capecitabine was significantly higher than that of each agent alone in three xenograft models (primary colon carcinoma, lymphatic metastasis, and hepatic metastasis). This study indicated that addition of FP3 to capecitabine significantly improved tumor growth inhibition in the PDTT xenograft models of primary colon carcinoma and lymphatic and hepatic metastases.
Keywords: :
Introduction
Fluoropyrimidine-based regimens remain the backbone of therapy for colorectal cancer (CRC). Capecitabine (N4-pentyloxycarbonyl-5′-deoxy-5-fluorocytidine, Xeloda) is an oral fluoropyrimidine drug widely used and is enzymatically metabolized to 5-FU by thymidine phosphorylase (TP) highly expressed in tumors.Citation1-Citation3 The p.o. administered fluoropyrimidine capecitabine has shown antitumor activity in xenograft models, including CRC.Citation4-Citation6 Randomized controlled trials have shown that in the management of CRC, capecitabine can safely and effectively replace i.v. 5-fluorouracil/leucovorin as monotherapy.Citation7-Citation9
FP3 (also named as KH902 or KH903) is an engineered protein which contains the extracellular domain 2 of VEGF receptor 1 (Flt-1) and extracellular domain 3 and 4 of VEGF receptor 2 (Flk-1, KDR) fused to the Fc portion of human immunoglobulin G1.Citation10,Citation11 Previous studies indicated that FP3 had promise as a local antiangiogenic treatment of human CNV (choroidal neovascularization)-related AMD (age-related macular degeneration).Citation10,Citation12-Citation14 In subsequent studies, it was demonstrated that FP3 has an inhibitory efficacy in VEGF-mediated proliferation and migration of human umbilical vein endothelial cells, and in VEGF-mediated vessel sprouting of rat aortic ring in vitro.Citation11 It was also demonstrated that FP3 has an antitumor effect in non-small cell lung cancer (A549), breast cancer (MCF-7), hepatocellular carcinoma (HepG2), and colorectal cancer (LoVo) cell lines xenograft models,Citation11,Citation15 and patient-derived tumor tissue (PDTT) xenograft models of gastric carcinomaCitation16 and colon carcinoma with lymphatic and hepatic metastasesCitation17 in nude mice.
Combining inhibition of VEGF by bevacizumab and chemotherapy by capecitabine could significantly improve tumor growth inhibition.Citation18-Citation20 However, whether combining inhibition of VEGF by FP3 and chemotherapy by capecitabine could act additive or synergistically is still unknown. In the present study, we evaluated the antitumor activity of FP3 in combination with capecitabine in the PDTT xenograft models of primary colon carcinoma with lymphatic and hepatic metastases.
Results
Antitumor activity of FP3 in combination with capecitabine
We first evaluated the antitumor activity of FP3 in combination with capecitabine in PDTT xenografts of primary colon carcinoma, lymphatic metastasis and hepatic metastasis. FP3 was administered i.v. at 15 mg/kg twice a week for 3 weeks and capecitabine was orally administered at 150 mg/kg daily for 14 d. On Day 22 (21 d after the treatment was initiated), there were statistically significant differences in tumor volume between the control and the groups treated with capecitabine, FP3 and FP3 in combination with capecitabine (p < 0.001, ) in all three xenograft models. No body weight-related toxicity was found in each group (). There were no statistically significant differences in tumor volume between the group treated with FP3 in combination with capecitabine and the groups treated with FP3 and capecitabine alone in xenograft models of primary colon carcinoma (). However, there were statistically significant differences in tumor volume between the group treated with FP3 in combination with capecitabine and the groups treated with FP3 (p < 0.05, ) and capecitabine (p < 0.001, ) alone in xenograft models of colon carcinoma lymphatic metastasis. There were also statistically significant differences in tumor volume between the group treated with FP3 in combination with capecitabine and the groups treated with capecitabine (p < 0.001, ) alone in xenograft models of colon carcinoma hepatic metastasis.
Figure 1. Response curve of FP3 and capecitabine (Xeloda) in the PDTT xenograft models of primary colon carcinoma (A), lymphatic metastasis (B), and hepatic metastasis (C). Ten mice per group were treated with the corresponding agent according to Materials and Methods. Data shown are means ± SEM. The differences between control tumor volumes, FP3-treated, capecitabine-treated, and FP3 + capecitabine-treated tumor volumes were analyzed by using one-way ANOVA. ***p < 0.001, **p < 0.05. Experiments were repeated at least two times with similar results.
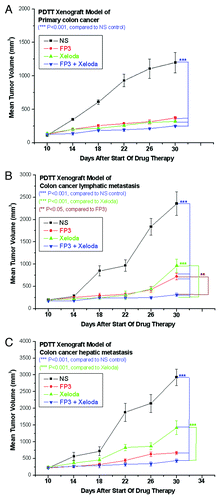
Figure 2. Tolerability to FP3, capecitabine (Xeloda), and FP3 in combination with capecitabine (Xeloda) in the PDTT xenograft models of primary colon carcinoma (A), lymphatic metastasis (B), and hepatic metastasis (C). Ten mice per group were treated with the corresponding agent according to Materials and Methods. Data shown are means ± SEM. The differences between control tumor volumes, FP3-treated, capecitabine-treated, and FP3 + capecitabine-treated tumor volumes were analyzed by using one-way ANOVA. Experiments were repeated at least two times with similar results.
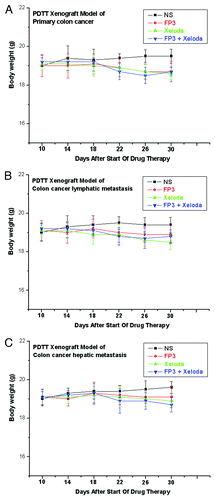
Effect of FP3 in combination with capecitabine on tumor-associated angiogenesis
To evaluate the effects of FP3 on tumor-associated angiogenesis in PDTT xenografts of primary colon carcinoma, lymphatic metastasis and hepatic metastasis, selected tumors were sectioned and immunostained with antibodies to CD31 and α-SMA, so that the vasculature could be visualized (–). This analysis revealed that vasculature was nearly absent in FP3-treated and FP3 in combination with capecitabine-treated xenografts. FP3 and FP3 in combination with capecitabine (treatment for 21 d) almost completely blocked tumor-associated angiogenesis, with the stunted tumors being largely avascular (–). In contrast to the FP3-treated and FP3 in combination with capecitabine-treated tumors, tumors in saline-treated mice and capecitabine-treated mice not only were much larger but also had a very high vascular density (–). These results indicate that FP3 administration reduces xenograft size and concurrently causes decreased microvessel growth, and the antiangiogenic effects were mainly induced by FP3 not by capecitabine.
Figure 3. FP3 and capecitabine (Xeloda) decreased vascular structure in the xenograft model of primary colon carcinoma. Vasculature was examined by angiography with immunostaining for endothelial cells (using anti-CD31 antibody; bar = 100 μm), and pericytes (using anti-α-SMA antibody; bar = 100 μm). Figure 4. FP3 and capecitabine (Xeloda) decreased vascular structure in the xenograft model of colon carcinoma lymphatic metastasis. Vasculature was examined by angiography with immunostaining for endothelial cells (using anti-CD31 antibody; bar = 100 μm), and pericytes (using anti-α-SMA antibody; bar = 100 μm).
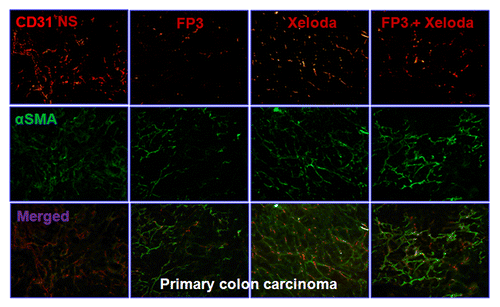
Figure 4. FP3 and capecitabine (Xeloda) decreased vascular structure in the xenograft model of colon carcinoma lymphatic metastasis. Vasculature was examined by angiography with immunostaining for endothelial cells (using anti-CD31 antibody; bar = 100 μm) and pericytes (using anti-α-sMa antibody; bar = 100 μm)
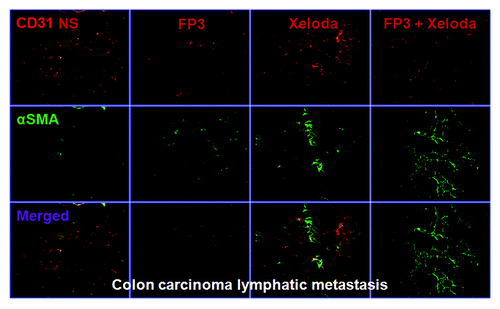
Figure 5. FP3 and capecitabine (Xeloda) decreased vascular structure in the xenograft model of colon carcinoma hepatic metastasis. Vasculature was examined by angiography with immunostaining for endothelial cells (using anti-CD31 antibody; bar = 100 μm), and pericytes (using anti-α-SMA antibody; bar = 100 μm).
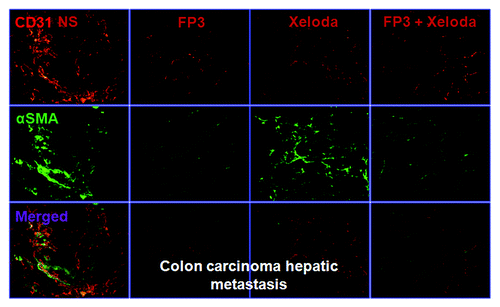
Effect of FP3 in combination with capecitabine on tumor cell proliferation
We next measured cell proliferation in the treated tumors of PDTT xenografts of primary colon carcinoma, lymphatic metastasis and hepatic metastasis. By immunohistochemical staining, we found that VEGF expressions in FP3-treated and FP3 in combination with capecitabine-treated tumors were significantly suppressed ( and ). We also found PCNA expressions in FP3-treated, capecitabine-treated, and FP3 in combination with capecitabine-treated tumors were significantly suppressed ( and ). We also measured the levels of related cell signaling pathways proteins by using western blotting. However, no significant differences were found in each group of each xenograft model, except that the expression level of pERK was upregulated ().
Figure 6. Effects of FP3 and capecitabine (Xeloda) on the expression of VEGF and PCNA in the PDTT xenograft models of primary colon carcinoma. Original magnifications, × 100. Effects of FP3 and capecitabine (Xeloda) on the expression of VEGF and PCNA in the PDTT xenograft models of colon carcinoma lymphatic metastasis. Original magnifications, × 100.
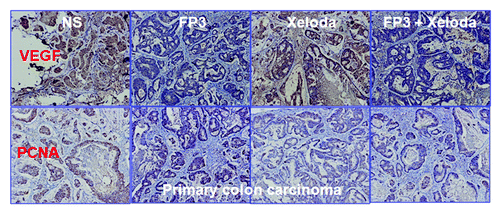
Figure 7. Effects of FP3 and capecitabine (Xeloda) on the expression of VeGF and PCNA in the PDTT xenograft models of colon carcinoma lymphatic metastasis. Original magnifications, x100.
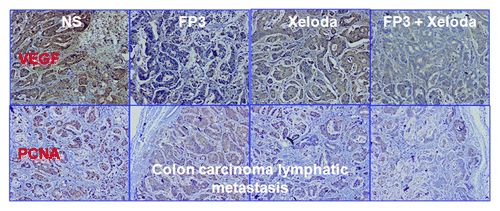
Figure 8. Effects of FP3 and capecitabine (Xeloda) on the expression of VEGF and PCNA in the PDTT xenograft models of colon carcinoma hepatic metastasis. Original magnifications, × 100.
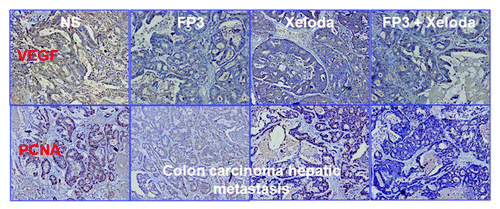
Figure 9. Immunoblotting data of the proteins Akt, pAkt (Ser308 and Ser473), ERK, pERK (Thr202/Tyr204), MAPK, pMAPK (Thr180/Tyr182) and GAPDH (as loading control) showing the effects of FP3 and capecitabine (Xeloda) on the proteins expression in the PDTT xenograft models of primary colon carcinoma and its corresponding lymphatic and hepatic metastases.
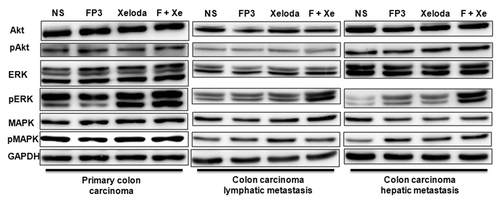
Levels of TP in tumor tissues
We measured the level of TP in tumor tissues in xenograft models. Levels of tumor TP were not changed after i.v. administration of FP3 at 15 mg/kg twice a week for 3 weeks in the PDTT xenograft models of primary colon carcinoma, lymphatic and hepatic metastasis ().
Table 1. Levels of TP from the PDTT xenografts of primary colon carcinoma, lymphatic metastasis, and hepatic metastasis treated with FP3, capecitabine (Xeloda), and FP3 in combination with capecitabine (Xelod®)
Discussion
In our previous work, we successfully established the PDTT xenograft models of colon carcinoma with lymphatic and hepatic metastases.Citation17 The ideal biological characteristics of such PDTT xenograft models as described previouslyCitation17 made us definitely believe that such PDTT models would help us investigate the antitumor activity of FP3 in primary colon carcinoma and its corresponding lymphatic and hepatic metastases. In our present study, we demonstrated the antitumor activity of FP3 in combination with capecitabine in the PDTT xenograft models of primary colon carcinoma and its corresponding lymphatic and hepatic metastases. The antitumor activity of FP3 in combination with capecitabine was significantly higher than that of capecitabine monotherapy in the PDTT xenograft models of colon carcinoma lymphatic and hepatic metastases. However, the combination use of FP3 and capecitabine fares no better than either agent alone against the primary colon carcinoma. In addition, we investigated the mechanisms of the combination effects of FP3 and capecitabine in the xenograft models.
Capecitabine is enzymatically metabolized to 5-FU as a result of the highly expressed TP in the tumor. Some chemotherapeutic drugs, such as the taxanes, have been reported to increase the levels of TP in tumors in xenograft models and to show significantly more potent antitumor activity in combination with capecitabine than each agent as a monotherapy.Citation21,Citation22 It has been also reported that oxaliplatin treatment increased the level of TP in tumor tissues in xenograft models.Citation23 In the present study, we investigated the levels of TP in tumors after treatment with FP3 to evaluate possible therapeutic effects in combination with capecitabine in xenograft models. However, FP3 induced no significant increase in levels of TP in the three xenograft models used, suggesting that the combination effects are a result of mechanisms other than TP upregulation in tumors treated with FP3.
FP3 is a humanized fusion protein which combines ligand binding elements taken from the extracellular domains of VEGF receptors 1 and 2 with the Fc portion of IgG1. It is aimed to bind to all forms of VEGF-A.Citation17 As in many previous studies,Citation11,Citation17 the present study also demonstrated that FP3 decreased microvessel density in the tumor tissues of the xenograft models. In tumors expressing VEGF, tumor growth would be more dependent on angiogenesis regulated by VEGF. Therefore, we investigated the level of VEGF after treatment with capecitabine to possibly explain the mechanisms of the effects of FP3 and capecitabine in combination. However, no significant decrease in tumor VEGF was demonstrated after treatment with capecitabine, suggesting that the combination effects are a result of mechanisms other than change in the level of VEGF in tumors treated with capecitabine.
The unregulated nature of tumor angiogenesis leads to the production of structurally and functionally abnormal vasculature, characterized by a number of different features, such as increased vessel density, diameter, length and tortuosity, abnormally high interstitial fluid pressure, and increased vascular permeability.Citation24,Citation25 These abnormalities prevent the effective delivery of therapy to the tumor. For example, the entry of large molecules, such as chemotherapeutic agents, into the tumor would be impeded and hypoxia results from inconsistent oxygen supply within the tumor, producing regions that would be resistant to radiotherapy and some cytotoxic agents. The potential vessel normalization effect of FP3 in solid tumors as we are investigating now may help make tumor cells more sensitive to cytotoxic chemotherapy and maximize the effectiveness of the overall cancer treatment strategy. In terms of clinical significance, normalization of tumor vasculature by FP3 may also maximize efficacy of concomitant therapy.
In conclusion, this study has demonstrated that FP3 can increase the efficacy of capecitabine in the PDTT xenograft models of primary colon carcinoma and its corresponding lymphatic and hepatic metastases. Based on these results, the combination use of FP3 and capecitabine merit further clinical investigation in patients with advanced colon carcinoma. We will further investigate the mechanisms of the combination therapies using these PDTT xenograft models.
Materials and Methods
Reagents and drugs
Anti-Akt, anti-ERK, and anti-MAPK, and phosphorylation-specific antibodies against Akt (Ser308 and Ser473), ERK (Thr202/Tyr204), and MAPK (Thr180/Tyr182) were purchased from Cell Signaling Technology Inc. (Cell Signaling). The antibody against PCNA was purchased from Cell Signaling Technology Inc. (Cell Signaling). The antibody against VEGF was purchased from Epitomics Inc. The antibody against platelet endothelial cell adhesion molecule-1 (PECAM-1, CD31) (rat monoclonal, clone MEC 13.3) was purchased from BD PharMingen. The antibody against α-smooth muscle actin (α-SMA, rabbit polyclonal) was purchased from Abcam. Fluorescent (Cy3- or FITC-conjuncted) secondary antibodies (goat anti-rat or anti-rabbit) were purchased from Jackson ImmunoResearch. The antibody against GAPDH was purchased from Santa Cruz Biotechnology, Inc. Horseradish peroxidase-conjugated secondary antibodies were purchased from Santa Cruz Biotechnology, Inc. Chemiluminescent detection system was purchased from Amersham Pharmacia Biotech (Arlington Heights, IL). Capecitabine (Xeloda) was purchased from Roche Laboratories (Roche). FP3 was kindly provided as a gift from Kanghong, Biotechnology Inc.
Patient and tissue samples
Tumor specimens were obtained at initial surgery from a 40-y-old female colon carcinoma patient with lymphatic and hepatic metastases. Prior written informed consent was obtained from the patient and the study received ethics board approval at First Affiliated Hospital, College of Medicine, Zhejiang University. The patient had not received chemotherapy or radiation therapy before surgery. The histological type was determined according to WHO criteria. The tumor was diagnosed as mucinous adenocarcinoma (T3N2M1).
Establishment of xenografts and treatment protocol
Four- to six-week-old female BALB/c nude mice purchased from Slaccas (Slaccas Laboratory Animal) were housed in a barrier facility and acclimated to 12 h light-dark cycles for at least three days before use. The use of experimental animals adhered to the “Principles of Laboratory Animal Care” (NIH publication #85–23, revised in 1985). All experiments were approved by the Institutional Animal Care and Use Committee of Zhejiang University [approval ID: SYXK(ZHE)2005–0072]. The method to establish the PDTT xenograft models of human colon carcinoma with lymphatic and hepatic metastases were described previously.Citation16,Citation17,Citation26,Citation27
PDTT xenografts of primary colon carcinoma, lymphatic metastasis and hepatic metastasis from the second mouse-to-mouse passage (the third generation, G3) were allowed to grow to a size of 200 mm3, at which time mice were randomized into the following three cohorts. Each cohort included four groups of treatment, with 10 mice in each group. In cohort of xenografts of primary colon carcinoma: (A) control (100 μl saline); (B) FP3, 15 mg/kg, i.v., twice per week; (C) Xeloda, 150 mg/kg in 200 μl [dissolved in sterile 0.9% NaCl solution containing 0.5% (w/v) HPMC], p.o., daily for 14 d; (D) Xeloda, 150 mg/kg in 200 μl, p.o., daily for 14 d + FP3, 15 mg/kg, i.v., twice per week. In cohort of xenografts of colon carcinoma lymphatic metastasis: (A) control (100 μl saline); (B) FP3, 15 mg/kg, i.v., twice per week; (C) Xeloda, 150 mg/kg in 200 μl [dissolved in sterile 0.9% NaCl solution containing 0.5% (w/v) HPMC], p.o., daily for 14 d; (D) Xeloda, 150 mg/kg in 200 μl, p.o., daily for 14 d + FP3, 15 mg/kg, i.v., twice per week. In cohort of xenografts of colon carcinoma hepatic metastasis: (A) control (100 μl saline); (B) FP3, 15 mg/kg, i.v., twice per week; (C) Xeloda, 150 mg/kg in 200 μl [dissolved in sterile 0.9% NaCl solution containing 0.5% (w/v) HPMC], p.o., daily for 14 d; (D) Xeloda, 150 mg/kg in 200 μl, p.o., daily for 14 d + FP3, 15 mg/kg, i.v., twice per week. Mice were treated during 21 d, monitored twice per week for signs of toxicity, and were weighed once a week. Tumor size was evaluated twice a week by caliper measurements using the following formula: tumor volume = (length × widthCitation2) / 2. Relative tumor growth inhibition (TGI) was calculated by relative tumor growth of treated mice divided by relative tumor growth of control mice (T/C). Experiments were terminated on day 30.Citation17
Immunohistochemistry
Selected tumor specimen were fixed in 10% neutral-buffered formalin and embedded in paraffin. Five micromolar sections were cut, dewaxed, rehydrated, and subjected to antigen retrieval. After blocking endogenous peroxidase activity, the sections were incubated with the primary antibodies against VEGF (1:100) and PCNA (1:100) overnight at 4°C. Immunohistochemistry was performed using the streptavidin-biotin peroxidase complex method (Lab Vision). The slides were examined and pictures were taken using an Olympus BX60 (Olympus). Sections known to stain positively were incubated in each batch and negative controls were also prepared by replacing the primary antibody with preimmune sera.Citation17
Fluorescent immunohistochemistry
Selected mice with similar tumor size were anesthetized with ketamine (87 mg/kg) plus xylazine (13 mg/kg) injected intramuscularly. The chest was opened rapidly, and the vasculature was perfused for 3 min at a pressure of 120 mmHg with fixative [4% paraformaldehyde in 0.1 mol/L phosphate-buffered saline (PBS), pH 7.4] from an 18-gauge cannula inserted into the aorta via an incision in the left ventricle. Blood and fixative exited through an opening in the right atrium. After the perfusion, the implanted tumor was removed and placed into fixative for 2 h at 4°C. Specimens were then rinsed several times with PBS, infiltrated overnight with 30% sucrose, embedded in OCT medium and frozen for cryostat sectioning.Citation17 Cryostat sections 8–10 μm in thickness were brought to room temperature, air-dried overnight, then fixed in acetone for 10 min. Slides were allowed to air dry for 30 min and were washed three times for 5 min each in PBS. Samples were then incubated in 5% BSA in PBS for 30 min at room temperature to block nonspecific antibody binding. Next, the sections were incubated with two primary antibodies (CD31, 1:100; and α-SMA, 1:200) overnight at room temperature in humidified chambers diluted in PBS. After several rinses with PBS, specimens were incubated for 1 h at room temperature with fluorescent (Cy3- or FITC-conjuncted) secondary antibodies (goat anti-rat or goat anti-rabbit) diluted (1:200) in PBS. Specimens were rinsed again with PBS, and mounted in Vectashield (Vector Laboratories).Citation17 Tissue sections were examined and digitally photographed using a Zeiss Axiophot fluorescence microscope (Carl Zeiss) equipped with single, dual, and triple fluorescence filters and a low-light, externally cooled, three-chip charge-coupled device (CCD) camera (480 × 640 pixel RGB-color images, CoolCam; SciMeasure Analytical Systems) and saved as TIFF files.Citation17
TP levels measurement
Tumor tissues were homogenized in 10 mM Tris-buffer (pH 7.4) containing 15 mM NaCl, 1.5 mM MgCl2 and 50 μM potassium phosphate buffer using a glass homogenizer. The homogenizer was then centrifuged at 10,000 rpm for 15 min at 4°C and the supernatants were stored at -80°C until use. The protein concentration of the supernatants was determined using a DC protein assay kit (Bio-Rad). The level of TP was measured by ELISA with monoclonal antibodies specific to human TP, as previously described by Nishida et al.Citation28 One unit corresponds to the amount of TP enzyme activity, which phosphorylates 5′-DFUR to 5-FU at rate of 1 μg 5-FU per hour (recombinant human TP).
Western blotting
Protein expression profiles were analyzed by western blotting as previously described.Citation17 Briefly, lysates for immunoblotting were prepared by adding lysis buffer [50 mM TRIS-HCl (pH 7.4), 1% Nonidet P-40, 0.5% sodium deoxycholate, 150 mM NaCl, 0.02% sodium azide, and 0.1% SDS] containing protease and phosphatase inhibitors (Sigma) to the tumor tissue homogenized in fluid nitrogen. After centrifugation at 15,000 rpm at 4°C for 10 min, the supernatants were collected, and the protein concentration was determined using Bio-Rad Protein Assay Kit (Bio-Rad). Protein extracts of tumor lysates (30 μg) were added to a loading buffer [10 mmol/L TRIS-HCl (pH 6.8), 1% SDS, 25% glycerol, 0.1 mmol/L mercaptoethanol, and 0.03% bromophenol blue], boiled, and separated on 8% to 12% (w/v) polyacrylamide gels in the presence of SDS. Molecular weights of the immunoreactive proteins were estimated based on the relative migration with colored molecular weight protein markers (Amersham Pharmacia Biotech). After electrophoresis, the protein blots were electro-transferred to PVDF membranes (Millipore). Then, the membranes were blocked at room temperature with 5% nonfat milk in TBS [10 mmol/L TRIS-HCl (pH 7.5), 0.5 mol/L NaCl, and 0.05% (v/v) Tween 20] buffer for 1 h. The primary antibodies were diluted at 1:1,000 and the membranes were incubated with primary antibodies overnight at 4°C. The antibodies tested were anti-Akt, anti-ERK, anti-MAPK and phosphorylation-specific antibodies against Akt (Ser308 and Ser473), ERK (Thr202/Tyr204) and MAPK (Thr180/Tyr182). The next day, the membranes were washed and incubated for 1 h at room temperature with rabbit immunoglobulin G-horseradish peroxidase-conjugated secondary antibodies (Santa Cruz Biotechnology), at a final dilution of 1:5,000. After washing thrice with TBS, antibody binding was visualized using enhanced chemiluminescence detection system (SuperSignal West Pico) as described by the manufacturer and autoradiography. To show equal protein loading, the blots were stripped and reprobed for GAPDH. This experiment was repeated three times with similar results.
Statistical analysis
Drug sensitivity data are presented as mean ± SEM and analyzed by SPSS 16.0 software. Difference among mean of the groups is determined with one-way ANOVA. Comparison is considered to be statistically significant if p < 0.05.
Abbreviations: | ||
AMD | = | age-related macular degeneration |
CNV | = | choroidal neovascularization |
CRC | = | colorectal cancer |
PDTT | = | patient-derived tumor tissue |
TP | = | thymidine phosphorylase |
VEGF | = | vascular endothelial growth factor |
Acknowledgments
This work was supported by the State Key Basic Research and Development Program of China (973 Program, grant no. 2009CB521704), National High-tech Research and Development Program of China (863 Program, grant no. 2006AA02A245), National Natural Science Foundation of China (grant no. 81000894), Zhejiang Provincial Science and Technology Projects (grants no. 2009C13021, 2011C23087), Science Research Fund of Shaoxing (grants no. 2011D10013) and Science Research Fund of Zhuji (grants no. 2011CC7874).
Disclosure of Potential Conflicts of Interest
The authors have declared that no competing interests exist.
Notes
† These authors contributed equally to this work.
References
- Ishikawa T, Utoh M, Sawada N, Nishida M, Fukase Y, Sekiguchi F, et al. Tumor selective delivery of 5-fluorouracil by capecitabine, a new oral fluoropyrimidine carbamate, in human cancer xenografts. Biochem Pharmacol 1998; 55:1091 - 7; http://dx.doi.org/10.1016/S0006-2952(97)00682-5; PMID: 9605432
- Miwa M, Ura M, Nishida M, Sawada N, Ishikawa T, Mori K, et al. Design of a novel oral fluoropyrimidine carbamate, capecitabine, which generates 5-fluorouracil selectively in tumours by enzymes concentrated in human liver and cancer tissue. Eur J Cancer 1998; 34:1274 - 81; http://dx.doi.org/10.1016/S0959-8049(98)00058-6; PMID: 9849491
- Schüller J, Cassidy J, Dumont E, Roos B, Durston S, Banken L, et al. Preferential activation of capecitabine in tumor following oral administration to colorectal cancer patients. Cancer Chemother Pharmacol 2000; 45:291 - 7; http://dx.doi.org/10.1007/s002800050043; PMID: 10755317
- Ishikawa T, Sekiguchi F, Fukase Y, Sawada N, Ishitsuka H. Positive correlation between the efficacy of capecitabine and doxifluridine and the ratio of thymidine phosphorylase to dihydropyrimidine dehydrogenase activities in tumors in human cancer xenografts. Cancer Res 1998; 58:685 - 90; PMID: 9485021
- Ishitsuka H. Capecitabine: preclinical pharmacology studies. Invest New Drugs 2000; 18:343 - 54; http://dx.doi.org/10.1023/A:1006497231579; PMID: 11081570
- Ninomiya I, Terada I, Yoshizumi T, Takino T, Nagai N, Morita A, et al. Anti-metastatic effect of capecitabine on human colon cancer xenografts in nude mouse rectum. Int J Cancer 2004; 112:135 - 42; http://dx.doi.org/10.1002/ijc.20360; PMID: 15305385
- Van Cutsem E, Twelves C, Cassidy J, Allman D, Bajetta E, Boyer M, et al, Xeloda Colorectal Cancer Study Group. Oral capecitabine compared with intravenous fluorouracil plus leucovorin in patients with metastatic colorectal cancer: results of a large phase III study. J Clin Oncol 2001; 19:4097 - 106; PMID: 11689577
- Hoff PM, Ansari R, Batist G, Cox J, Kocha W, Kuperminc M, et al. Comparison of oral capecitabine versus intravenous fluorouracil plus leucovorin as first-line treatment in 605 patients with metastatic colorectal cancer: results of a randomized phase III study. J Clin Oncol 2001; 19:2282 - 92; PMID: 11304782
- Twelves C, Wong A, Nowacki MP, Abt M, Burris H 3rd, Carrato A, et al. Capecitabine as adjuvant treatment for stage III colon cancer. N Engl J Med 2005; 352:2696 - 704; http://dx.doi.org/10.1056/NEJMoa043116; PMID: 15987918
- Teng LS, Jin KT, He KF, Wang HH, Cao J, Yu DC. Advances in combination of antiangiogenic agents targeting VEGF-binding and conventional chemotherapy and radiation for cancer treatment. J Chin Med Assoc 2010; 73:281 - 8; http://dx.doi.org/10.1016/S1726-4901(10)70062-9; PMID: 20603084
- Jin K, He K, Teng F, Li G, Wang H, Han N, et al. FP3: a novel VEGF blocker with antiangiogenic effects in vitro and antitumour effects in vivo. Clin Transl Oncol 2011; 13:878 - 84; http://dx.doi.org/10.1007/s12094-011-0749-z; PMID: 22126731
- Zhang M, Zhang J, Yan M, Li H, Yang C, Yu D. Recombinant anti-vascular endothelial growth factor fusion protein efficiently suppresses choridal neovasularization in monkeys. Mol Vis 2008; 14:37 - 49; PMID: 18246030
- Zhang M, Yu D, Yang C, Xia Q, Li W, Liu B, et al. The pharmacology study of a new recombinant human VEGF receptor-fc fusion protein on experimental choroidal neovascularization. Pharm Res 2009; 26:204 - 10; http://dx.doi.org/10.1007/s11095-008-9718-9; PMID: 18854954
- Zhang M, Zhang J, Yan M, Luo D, Zhu W, Kaiser PK, et al, KH902 Phase 1 Study Group. A phase 1 study of KH902, a vascular endothelial growth factor receptor decoy, for exudative age-related macular degeneration. Ophthalmology 2011; 118:672 - 8; http://dx.doi.org/10.1016/j.ophtha.2010.08.008; PMID: 21146224
- Yu DC, Lee JS, Yoo JY, Shin H, Deng H, Wei Y, et al. Soluble vascular endothelial growth factor decoy receptor FP3 exerts potent antiangiogenic effects. [Epub ahead of print] Mol Ther 2012; 20:938 - 47; http://dx.doi.org/10.1038/mt.2011.285; PMID: 22273580
- Jin K, He K, Han N, Li G, Wang H, Xu Z, et al. Establishment of a PDTT xenograft model of gastric carcinoma and its application in personalized therapeutic regimen selection. Hepatogastroenterology 2011; 58:1814 - 22; http://dx.doi.org/10.5754/hge11136; PMID: 21940303
- Jin K, Li G, Cui B, Zhang J, Lan H, Han N, et al. Assessment of a novel VEGF targeted agent using patient-derived tumor tissue xenograft models of colon carcinoma with lymphatic and hepatic metastases. PLoS One 2011; 6:e28384; http://dx.doi.org/10.1371/journal.pone.0028384; PMID: 22164281
- Kolinsky K, Zhang YE, Dugan U, Heimbrook D, Packman K, Higgins B. Novel regimens of capecitabine alone and combined with irinotecan and bevacizumab in colorectal cancer xenografts. Anticancer Res 2009; 29:91 - 8; PMID: 19331137
- Yanagisawa M, Fujimoto-Ouchi K, Yorozu K, Yamashita Y, Mori K. Antitumor activity of bevacizumab in combination with capecitabine and oxaliplatin in human colorectal cancer xenograft models. Oncol Rep 2009; 22:241 - 7; PMID: 19578762
- Saltz LB, Clarke S, Díaz-Rubio E, Scheithauer W, Figer A, Wong R, et al. Bevacizumab in combination with oxaliplatin-based chemotherapy as first-line therapy in metastatic colorectal cancer: a randomized phase III study. J Clin Oncol 2008; 26:2013 - 9; http://dx.doi.org/10.1200/JCO.2007.14.9930; PMID: 18421054
- Sawada N, Ishikawa T, Fukase Y, Nishida M, Yoshikubo T, Ishitsuka H. Induction of thymidine phosphorylase activity and enhancement of capecitabine efficacy by taxol/taxotere in human cancer xenografts. Clin Cancer Res 1998; 4:1013 - 9; PMID: 9563897
- Endo M, Shinbori N, Fukase Y, Sawada N, Ishikawa T, Ishitsuka H, et al. Induction of thymidine phosphorylase expression and enhancement of efficacy of capecitabine or 5′-deoxy-5-fluorouridine by cyclophosphamide in mammary tumor models. Int J Cancer 1999; 83:127 - 34; http://dx.doi.org/10.1002/(SICI)1097-0215(19990924)83:1<127::AID-IJC22>3.0.CO;2-6; PMID: 10449619
- Sawada N, Kondoh K, Mori K. Enhancement of capecitabine efficacy by oxaliplatin in human colorectal and gastric cancer xenografts. Oncol Rep 2007; 18:775 - 8; PMID: 17786335
- Jain RK. Normalizing tumor vasculature with anti-angiogenic therapy: a new paradigm for combination therapy. Nat Med 2001; 7:987 - 9; http://dx.doi.org/10.1038/nm0901-987; PMID: 11533692
- Jain RK. Normalization of tumor vasculature: an emerging concept in antiangiogenic therapy. Science 2005; 307:58 - 62; http://dx.doi.org/10.1126/science.1104819; PMID: 15637262
- Jin K, Teng L, Shen Y, He K, Xu Z, Li G. Patient-derived human tumour tissue xenografts in immunodeficient mice: a systematic review. Clin Transl Oncol 2010; 12:473 - 80; http://dx.doi.org/10.1007/s12094-010-0540-6; PMID: 20615824
- Jin KT, He KF, Li GL, Teng LS. Personalized cancer therapy using a patient-derived tumor tissue xenograft model: a translational field worthy of exploring further?. Pers Med 2010; 7:597 - 606; http://dx.doi.org/10.2217/pme.10.48
- Nishida M, Hino A, Mori K, Matsumoto T, Yoshikubo T, Ishitsuka H. Preparation of anti-human thymidine phosphorylase monoclonal antibodies useful for detecting the enzyme levels in tumor tissues. Biol Pharm Bull 1996; 19:1407 - 11; http://dx.doi.org/10.1248/bpb.19.1407; PMID: 8951154