Abstract
Up to 30% of cancer patients undergoing curative surgery develop local recurrences due to positive margins. Patients typically receive adjuvant chemotherapy, immunotherapy and/or radiation to prevent such relapses. Interestingly, evidence supporting these therapies is traditionally derived in animal models of primary tumors, thus failing to consider surgically induced tumor microenvironment changes that may influence adjuvant therapy efficacy. To address this consideration, we characterized a murine model of local cancer recurrence. This model was reproducible and generated a postoperative inflammatory tumor microenvironment that resembles those observed following human cancer surgery. To further validate this model, antagonists of two pro-inflammatory mediators, TGFβ and COX-2, were tested and found to be effective in decreasing the growth of recurrent tumors. We appreciated that preoperative TGFβ inhibition led to wound dehiscence, while postoperative initiation of COX-2 inhibition resulted in a loss of efficacy. In summary, although not an exact replica of all human cancer surgeries, our proposed local recurrence approach provides a biologically relevant and reliable model useful for preclinical evaluation of novel adjuvant therapies. The use of this model yields results that may be overlooked using traditional preclinical cancer models that fail to incorporate a surgical component.
Introduction
Last year, 1.4 million people developed cancer in the United States, and cancer related mortality accounted for 625,000 deaths (the most common cause of death).Citation1 Surgery remains the most effective therapy for solid tumors, but up to 30% of patients undergoing curative cancer surgery will recur locally.Citation2 It is well established that local recurrences are often the result of residual disease persisting and expanding following incomplete resections (positive margins).Citation2
Positive margins are located in a unique microenvironment influenced by surgical manipulation. Multiple studies reveal that the expression of cyclooxygenase-2 (COX-2), a prostaglandin-producing enzyme, is increased in the surgical wound and residual tumor microenvironment following resection.Citation3 Transforming growth factors (TGF) are also present in surgical wounds and where encourage healing.Citation4 In additional to wound healing properties, these factors have has been suggested to promote survival and growth of recurrent disease from residual tumor cells. In fact, models in which tumors cells are injected near a surgical wound have been found to grow more aggressively than tumor cells in a naïve microenvironment.Citation3
Given the dangers of developing locally recurrent disease, patients are commonly treated with postoperative (or “adjuvant”) protocols incorporating chemotherapy, radiotherapy and/or immunotherapy. Despite obvious limitations, small animal models are typically utilized for high-throughput preclinical studies to examine the value of therapies.Citation5,Citation6 Unfortunately, only a limited number of preclinical studies evaluate adjuvant protocols in animal models that incorporate surgical wounding and postoperative tumor microenvironment.Citation5 Instead, the preclinical evidence supporting adjuvant therapies is based on primary tumor models and then assumed to be applicable to postoperative recurrences. Given the array of changes in the tumor microenvironment following surgery, we postulated that preclinical studies investigating adjuvant therapies should be conducted in recurrence models that incorporate surgical wounding factors and changes in the postoperative microenvironment.
The purpose of this study was to critically evaluate and develop a surgical model of local cancer recurrence that incorporates surgical wounding and recurrent microenvironment variables. Although not a perfect model for all “incomplete” human cancer surgeries, where micrometastatic disease is thought to be left behind, we found that a positive margin approach was reproducible, allowed for growth kinetic analysis and displayed similarities to tumor microenvironment changes associated with human cancer surgery. We thus utilized this model to test the hypothesis that adjuvant immunotherapies targeting two pro-tumor inflammatory mediators, TGFβ and COX-2, can improve postoperative results for cancer surgery. Both approaches were effective, however, several issues (wound dehiscence, timing of adjuvant therapies and synergy with the surgical procedure) were discovered that would have been overlooked in a traditional preclinical tumor models that fail to incorporate surgical variables.
Results
Traditional approaches of local cancer recurrences following surgery are inconsistent
The most frequently used model of postoperative local recurrences involves establishing a flank tumor, completely excising the tumor, and then monitoring for tumor recurrence.Citation7 To evaluate this approach, three different tumor cell lines (TC1, AKR, or AB12) were injected into a subcutaneous location on the murine flank. Once tumors were established and measured 200 mm3, 500 mm3, 800 mm3, or 1,100 mm3, mice underwent complete tumor excision. Surgical sites were monitored for local recurrence daily. Interestingly, after 2 mo of monitoring, fewer than 20% (21 of 120 mice) developed locally recurrent disease (data not shown). Nearly 75% of those tumors that relapsed were observed following the resection of a large (~800 mm3 or ~1,100 mm3) TC1 or AKR tumor. These findings likely reflect the aggressive growth of AKR and TC1 tumors which often abut fascial planes and structures such as ribs, vertebral bodies, and the peritoneum. Despite these challenging operations, diligent surgery did cure nearly all of mice (data not shown). These findings suggest that the traditional approach of complete tumor excision is unreliable in reproducing local recurrences in many tumor lines.
The positive margin resection model reproducibly develops recurrent tumors
Based on these observations, we hypothesized that a more reproducible flank tumor model of local recurrence would incorporate incomplete tumor excision (deliberately leaving a “positive margin”). To evaluate this approach, flanks of mice were again inoculated with TC1, AKR, or AB12 tumors. Once tumors were fully established (approximately 500mm3), 95% of the tumor volume was sharply resected with a scalpel, leaving behind a positive margin (see methods).Citation8 Appropriate residual tumor volumes were determined by measuring tumor dimensions before and after surgery. Of note, we determined that a residual volume of 5% was the smallest quantity of tumor that allowed for sufficient preservation of blood supply and viable tumor allowing for consistent tumor re-growth (data not shown).
Locally recurrent disease was observed in greater than 97% of mice undergoing “positive margin” surgery (data not shown). Recurrences were difficult to recognize within the immediate perioperative period as a result of suturing techniques and normal healing which produced local inflammation. This focal swelling, which often visually and tactilely resembled tumor recurrence, resolved within 72 h; thus recurrent disease could not be appreciated before 4 to 7 d following surgery. In several animals, the wounds were re-opened at 1 and 3 d and inspected for the location of the residual tumor. In all these cases, the residual tumor could not be located by three independent observers. However, within 7 d, tumors recurred and the local growth of recurrent disease was highly consistent and reproducible within given experiments (). In addition, time-to-disease progression (defined as the time when nodules reached 500mm3 in volume) was consistent within respective cell lines ().
Figure 1. A positive margin model of local recurrence after surgery is consistent and allows for the study of recurrent tumor growth kinetics. (A) Mice were injected with the AB12, AKR, and TC1 cell lines, and 95% of tumor mass was excised. Mean growth in the positive margin model of local recurrence was measured. (B) Mean time to disease progression (defined as a recurrent nodule volume of greater than or equal ~500 mm3) was measured. (C) Representation: Growth of tumors in the positive resection model was divided into 3 major phases: initial tumor growth (Line A), growth of the established tumor (Line B), and growth of the recurrent tumor (Line C). (D) Growth velocity of tumor growth for each phase was compared within TC1, AB12, and AKR cell lines. (E) Comparisons of recurrent growth to early growth: a 268%, 291%, and 180% increase velocity was observed in the TC1, AB12 and AKR cell lines, respectively. When compared with established tumor growth velocities, recurrent tumor growth differed by 3% in TC1, 14% in AB12 and 17% in AKR. *, values were calculated using the following formula: (vr/vi) x 100%; where vr and vi were defined as locally recurrent tumor (Line C) and the growth velocity of the early (Line A), respectively. **, values calculated using formula |(vr-vest)/vest| x 100%; where vr and vest were defined as the growth of the locally recurrent tumor (Line C) and the growth velocity of the established (Line B), respectively.
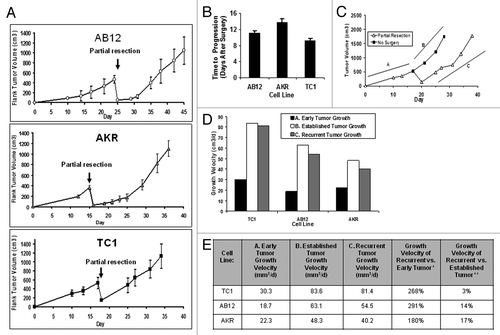
In order to assess the practicality and reproducibility of this approach, we repeated these experiments with lab personnel of varying surgical experience (an undergraduate student, a medical student, two technicians, an attending surgeon). There was some variation in ability; however, all personnel were able to leave behind positive margins after 5 to 7 attempts. After performing approximately 15 to 20 resections, personnel gained sufficient experience that it was not necessary to confirm dimensions of the residual tumor before and after surgery and could be accurately estimated by quick visual inspection (data not shown). Accurate resection of a wide variety of tumor types, including aggressive AKR and TC1 flank tumors which can cavitate and necrose, was achievable.
As a first application of our model, we evaluated the common notion that surgical manipulation accelerates tumor growth.Citation9 Again, mice were injected with tumor cells (TC1, AB12, or AKR) on the flank. Once tumors measured 500 mm3, mice were randomized to partial resection or no surgery. The rate of growth of recurrent tumors was compared with the rate of growth of unresected tumors. Tumors were monitored and the rate of growth was calculated using the slope of the best fit linear approximation. Recurrent tumors from the positive margins had an accelerated rate of growth when compared with the growth of de novo tumors (; line A vs. line C). A 268%, 291%, and 180% increase in growth velocity was observed in the TC1, AB12, and AKR flank tumors, respectively (). However, the rate of tumor growth of a partially resected tumor was similar to the growth rate of an established tumor (defined as the growth of tumors after reaching ~500 mm3) (; line B vs. line C). In all three cell lines tested, the growth velocity of the recurrent nodule varied by less than 17% of the rate of established tumors (). This suggests that resected tumors do not grow faster postoperatively, but continue along the same kinetics as an established tumor.
Together these data demonstrate that the partial resection model can be reproducible and takes into account a number of issues (i.e., anesthesia, perioperative immunosuppression, wound healing, growth kinetics) encountered in human cancer surgery. Furthermore, its consistent pattern among various tumor types allows for analysis by comparison of growth velocities and time to disease progression which may be of utility in evaluating adjuvant treatments.
Positive margin surgery induces an inflammatory state in the postoperative tumor microenvironment
It is well documented that surgery generates a vigorous inflammatory response marked by infiltration of the surgical site by innate-immune system leukocytes (namely neutrophils and macrophages), growth factors and cytokine production (for example, TGFβ, PDGF).Citation3,Citation10-Citation12 This microenvironment is in direct contact with residual tumor that ultimately gives rise to locally recurrent disease. To further validate our positive margin model of local recurrences, we examined the immunologic changes within the surgically-induced tumor microenvironment of positive margins.
Mice bearing established AB12 and TC1 tumors (500 mm3) were randomized to surgery or no intervention. Residual tumor margins were tagged with a stitch to help locate these nodules after 24 h, 48 h and one week. At each of these time points, the wounds were opened and the tumors harvested. Flow cytometry of the tumors remaining in the surgical wound revealed a robust increase of infiltrating leukocytes at 24 h in mice undergoing surgery that only partially returned to baseline by one week (). Leukocytes were primarily CD11b+ with a rapid influx of neutrophils in residual tumors at 24 h in mice undergoing positive margin surgery vs. non-surgical mice; (). By 7 d following surgery, inflammatory responses subsided, however the percentage of neutrophils was still increased ().
Figure 2. The positive margin model of local recurrence generates a surgical microenvironment that resembles a human surgical field. (A) Representative flow tracings of mice bearing AB12 tumors. Leukocytes (CD45+), neutrophils (CD45+, CD11b+, Ly6G+) and macrophages (CD45+, CD11b+, Ly6G-, F4/80+) were quantified. Gating was performed using a viability probe, followed by selecting CD45+ cells. Surgical site (at 24 h, 48 h, and 7 d following positive margin surgery) was compared with an established tumor (no surgery). (B) Immunohistochemical analysis of the microenvironment of recurrent tumors compared with primary tumors demonstrates the difference in infiltrating immune cells particularly neutrophils. Black arrows indicate leukocytes in the left hand column and neutrophils in the right hand column in primary tumors (at the time of surgery) vs. residual tumors (24 h after surgery).
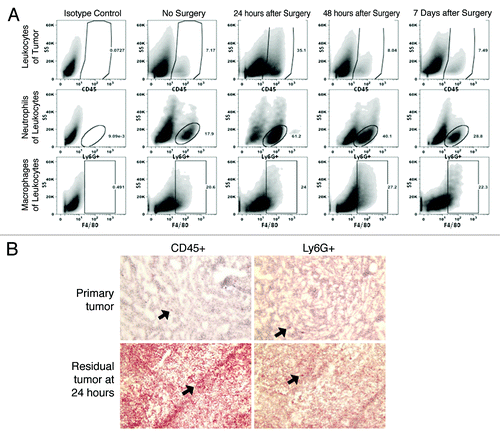
These results suggest that this model generates an inflammatory response that resembles that described in human surgical microenvironments where it is known that rapid infiltration of innate immune cells occurs.Citation3,Citation10-Citation12
Perioperative TGFβ inhibition improves postoperative outcomes, but has dangerous wound healing consequences
Multiple preclinical studies have demonstrated that systemic TGFβ blockade impedes tumor progression in murine models of mesothelioma,Citation13,Citation14 gliomas,Citation15 NSCLCCitation16 and renal cell carcinomas.Citation17 Additional studies have suggested that TGFβ blockade, when combined with tumor vaccination, generates improved anti-tumor immune responses.Citation15,Citation16,Citation18 Our group has shown a positive role for TGFβ inhibition using an ALK5 kinase inhibitor as a surgical adjuvant,Citation14 however, these results were not obtained using models that incorporate changes in the recurrent tumor microenvironment which were induced by surgical manipulation.
We evaluated the efficacy of adjuvant TGFβ inhibition using an anti-TGFβ antibody (1D11; similar to the antibody being used by Genzyme® in human clinical trials) using the positive margin model of local recurrence. Mice were injected with flank AB12 tumors and partial resection surgery was performed 17 d following tumor inoculation (a time when tumors measured 500 mm3). Animals (n = 21) were randomized to receive: 1) the control antibody (13C4) one week prior to surgery and continued for two weeks following surgery (“Control”), 2) the TGFβ blocking antibody (1D11) one week prior to surgery and continued for two weeks following resection (“perioperative 1D11”), or 3) the TGFβ blocking antibody (1D11) beginning at postoperative day four and continued for three weeks (“postoperative 1D11”).
Similar to our results with the adjuvant administration of the ALK5 kinase inhibitor,Citation14postoperative TGF-β inhibition significantly reduced the post-operative tumor volume in mice compared with controls ( vs. ). When TGFβ inhibition was begun before surgery (perioperative protocol) (), we even greater efficacy, with virtually no growth of the post-operative tumors (). Administration of perioperative TGFβ inhibition was associated with decreased tumor growth rate (16.5 vs. 47.1 mm3/d; p = 0.02; ) and increased progression free survival (18.8 vs. 11.1 d; p < 0.001, ) when compared with mice receiving controls.
Figure 3. Evaluation of adjuvant systemic TGFβ blockade on local recurrence in mice bearing AB12 flank tumors. Mice bearing established AB12 tumors were randomized to 3 groups (A, B, and C) (A) Mice (n = 7) were randomized to the control antibody 13C4 and underwent positive margin surgical resection on Day 17. (B) A second group of mice (n = 7) was randomized to a “postoperative 1D11” protocol” consisting of 1D11 administration beginning on postoperative day four and also continued for a total of three weeks. (C) A third group of mice (n = 7) was randomized to “perioperative 1D11” with the 1D11 injections beginning one week prior to surgery and continued for two weeks following surgery. (D) Bar graph summarizing recurrent tumor sizes on Day 34 (Postoperative Day 16). (E) Bar graph summarizing locally recurrent tumor growth kinetics among mice receiving control treatment vs. postoperative 1D11. (F) Bar graph describing progression free survival of mice randomized to control treatment vs. postoperative 1D11. *Signifies a relationship associated with a p < 0.05; **Signifies a relationship that is associated with a p < 0.01.
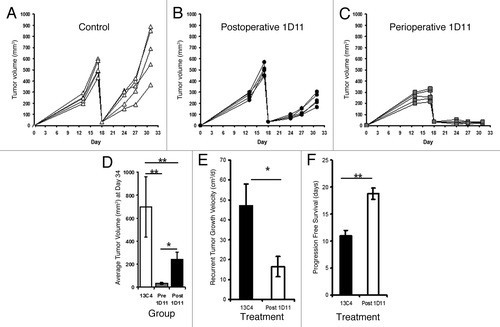
Although encouraging, 60% mice randomized to perioperative TGFβ inhibition experienced significant wound dehiscence, while no wound problems were observed in mice receiving control antibody or postoperative protocols; p = 0.02 (). Open wounds were re-closed using sterile 4–0 sutures under anesthesia and all mice survived.
Figure 4. Representative wound dehiscence observed in mice receiving “perioperative 1D11”; image taken prior to and after open wound closure. The silk sutures were intact and the tissues appeared grossly similar between treated and control mice. However, mice that had received TGFβ had clear wound pathology.
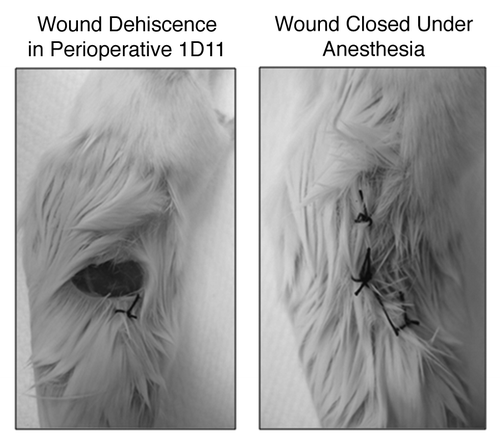
Mechanistic studies revealed that TGFβ inhibition was associated with increased percentages of CD8 T-cells within the recurrent tumor (). Using an in vivo tumor neutralization (Winn) assay, we also found that splenic CD8 T-cells obtained from mice receiving adjuvant TGFβ inhibition were more active in preventing the growth of AB12 cells as compared with controls (p = 0.02) ().
Figure 5. Adjuvant TGFβ blockade increases intratumoral CD8 T-cell trafficking and CD8 T-cell tumor neutralization capability. (A) Representative FACS tracing for intratumoral CD8 T-cells at postoperative day 10 (after two doses of antibody were given). (B) Winn assay results revealing that adjuvant 1D11 increases in vivo CD8 T-cell functional activity. (n = 5 per group). *Signifies a relationship associated with a p < 0.05; **Signifies a relationship that is associated with a p < 0.01.
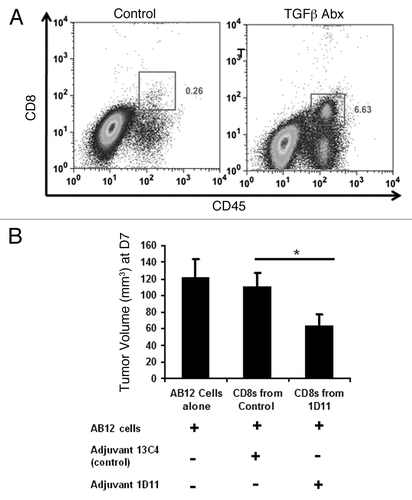
Together these experiments confirm the theoretical benefit of adjuvant anti-TGFβ immunotherapy in preventing local relapses in our surgical model. Further, these experiments highlight important surgical considerations such approaches on wound healing that would have otherwise been missed in non-surgical or suboptimal surgical models. Finally, these findings also validate the importance of accurate surgical models of local recurrence.
Adjuvant COX-2 inhibition improves postoperative outcomes, but timing is critically related to efficacy
COX-2 inhibition has been a well-documented therapy in treating a wide array of tumors including colon, breast, esophageal and lung cancers.Citation19-Citation22 Again, these studies have been derived in animal models of primary tumors or limited surgical models and assumed to be relevant to treating tumor relapses after surgery. Like TGF-β, COX-2 play a major component in wound healing COX-2 and also serves as pro-tumor effectors within the tumor microenvironment.Citation3
With this in mind, we sought to determine the efficacy of COX-2 inhibition preventing local disease recurrence following surgical resection. To evaluate this, we again utilized the AB12 mesothelioma model. Once tumors were fully established, mice were randomized to receive Celecoxib chow or control chow. Three days following randomization, mice underwent positive margin surgery and recurrent disease was monitored. We observed nearly a 50% reduction in tumor volume by postoperative day 14 in mice randomized to COX-2 inhibition ( and C). Mice receiving the drug also were observed to have decreased growth velocity of recurrent tumors (27.3 vs. 86.7 mm3/d; p = 0.007; ) and improved progression free survival (15.2 vs. 7.8 d; p = 0.03; ). In contrast to TGFβ blockade, however, we observed no wound healing deficits (data not shown). Interestingly, if COX-2 inhibition was begun after the immediate postoperative period (i.e., one week after surgery), the therapeutic efficacy of this approach was completely lost (data not shown). This was repeated several times with similar findings suggesting that the timing of initiating COX-2 inhibition is a critical consideration. To further reproduce the efficacy of COX-2 inhibition in preventing local disease relapses, we repeated the aforementioned experiment using the murine esophageal cancer line, AKR. Again, we appreciated decreases in postoperative tumor burdens when initiating COX-2 inhibition prior to surgical resection (Fig. S1).
Figure 6. Adjuvant COX-2 decreases local recurrences via CD8 dependent mechanisms. Mice bearing AB12 Tumors were randomized to control chow (A), n = 5) or Celecoxib chow (B), n = 5) and surgery was performed. Recurrent growth was recorded. (C) Bar graph demonstrating flank tumor volumes at postoperative day 14. (D) Bar graph summarizing locally recurrent tumor growth kinetics among mice receiving control treatment vs. COX-2 chow. (E) Bar graph describing progression free survival of mice randomized to control treatment vs. Celecoxib chow. (F) Depletion of CD8 T-cells at the time of COX-2 chow administration eliminates anti-tumor effects suggesting a role of CD8 T-cells in tumor response. *Signifies a relationship associated with a p < 0.05; **Signifies a relationship that is associated with a p < 0.01.
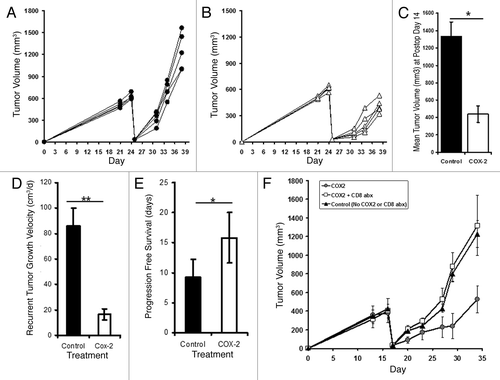
To determine if CD8 T-cell function was augmented after COX-2 inhibition, we repeated the previous experiments using the AB12 model, but depleted CD8 T-cells using anti-murine CD8 antibodies. We found that the effects of celecoxib chow were negated in the presence of CD8 T-cell depletion (). These findings suggest COX-2 activity impairs (either directly or indirectly) CD8 T-cell anti-tumor capabilities, and at least partial restoration of CD8 T-cell function can be achieved using selective COX-2 inhibitors.
Our data revealed a strong effect of adjuvant COX-2 inhibition in inhibiting locally recurrent disease. Unlike our result with TGFβ inhibition, no adverse effects on wound healing were observed when immunotherapy was administered prior to surgical resection. It is important, however, to note the dramatic differential efficacy with relation to the time of drug administration and the surgical resection.
Discussion
Local cancer recurrences following surgical resection of solid organ tumors affect nearly 250,000 patients each year.Citation2 This cohort of patients does exceptionally poorly with dismal long-term outcomes.Citation2 Despite enormous ramifications, there is a paucity of studies that examine therapies in the setting of post-surgical recurrences. In this study, we optimized a model of local recurrence (the “positive margin” model), and then used this model to evaluate the effect of two clinically relevant immunotherapies that influence the tumor microenvironment: systemic TGFβ blockade and COX-2 inhibition. The surgical setting had important effects on both approaches.
In the past, our group and others have studied postoperative local recurrences by incorporating “complete” flank tumor excision followed by monitoring for recurrent growth.Citation7,Citation14,Citation23 We have found that with good technique, flank tumors of most tumor cell lines can be fully excised, and locally recurrent disease develops idiosyncratically over several weeks. Clearly, such models confound therapeutic trials and leave data acquisition inefficient.
Key characteristics of an ideal local recurrence surgical model include: (1) the ability to perform surgery on primary tumors without undue stress to the animal, (2) a high degree of technical feasibility (allowing high-throughput), (3) cost effectiveness, (4) reproducibility and (5) most important, similarity to humans cancer recurrence. The optimal animal model should also utilize an immunocompetent host given the complex inflammatory and immune responses that occur during the perioperative period such as the presence of an endogenous anti-tumor immune response (concomitant immunity),Citation24 perioperative immune suppression,Citation25 and inflammatory consequences associated with wounds and wound healing.Citation10
These requirements make it difficult to use cancer models generated by recent advances in tumor modeling. Transgenic mice that develop orthotopic tumors have proven effective for studying oncologic concepts including carcinogenesis, tumor microenvironment, growth kinetics, and immune system changes. However, with the exception of breast and skin cancers, orthotopic tumors (for example lung, pancreas, liver, brain, colon, or the prostate tumors) are not conducive to surgical resection in mice. Additionally, these models often require prolonged periods for tumor development, resulting in low-throughput models that are ineffective for preclinical evaluations. Another advance in animal cancer modeling has been transfection or genetic manipulation of tumor models with marker genes (i.e., luciferase and green fluorescent protein) which may allow for monitoring recurrent lesions by imaging.Citation26,Citation27Unfortunately, introduction of foreign proteins tends to increase the immunogenicity of the model, thus altering (in some circumstances completely inhibiting) tumor growth in wild type mice with intact immune systems. As a consequence, the vast majority of these imaging studies are performed with transfected cells xenografted into immunodeficient mice.Citation27
Given these hurdles, we sought to develop a model of local cancer recurrence that would reproduce some (but not all) of the factors seen in human surgery. It is important consider that such tumor models deviate from human disease with respect to their tumor locations, variable genetic make-ups, and aggressive growth kinetics; however, cancer models in flank tumor locations allow for humane surgical resection and subsequent post-operative tumor observation. As demonstrated in our data, we began by performing an R0 resection (complete tumor resection), but were able to achieve local control easily, with cure rates approaching nearly 80% time. Although this technique best recapitulates what occurs in the human scenario, it was unfortunately unreliable in generating locally recurrent disease. Given this limitation, we deviated from the traditional human approach of “complete resection” and implemented a partial resection approach, by excising 95% of the tumor while leaving behind a small positive margin. This model is very similar to the clinical “debulking” scenario that might be used in ovarian cancer, brain cancer, and mesothelioma (an R2 resection), but also approximate the positive margin scenario occurring in human surgery. Although these drawbacks are present, this model likely does provide a more stringent model for preclinical adjuvant therapy evaluation given the relatively large amount of residual tumor.
Understanding these limitations, we still believe this approach was quite valuable. This resection model was technically feasible, while also being superiorly reproducible. Further all lab personnel were able to master the technique within several attempts. In total, the procedure required less than 10 min per mouse, and the growth curves were consistent among surgeons independent of surgical experience. This approach allowed for further analysis of postoperative tumor growth kinetics, time to disease progression and measurements of the postoperative tumor bed microenvironment. Most importantly, we were able to demonstrate important consequences of manipulating the postoperative tumor microenvironment by systemic inhibition of TGFβ or COX-2.
In addition to feasibility, this approach produces a biologically relevant model of local cancer recurrences and a surgically induced tumor microenvironment. With this consideration in mind, we sought to modulate two inflammatory forces commonly found in the recurrent tumor microenvironment that promote cancer recurrences. The first, TGFβ, is a cytokine with multiple known pro- and anti-tumor immunological effects.Citation28,Citation29 We have over a decade of experience with both anti-TGFβ therapyCitation14,Citation16,Citation30,Citation31and COX-2 inhibitionCitation22,Citation32,Citation33in mouse models. Furthermore, our group has recently begun a clinical trial using an anti-TGFβ monoclonal antibody GC-1008 (Genzyme) in patients with malignant mesothelioma and a trial combining COX-2 inhibition with type I interferon immune-gene therapy approaches. Unfortunately, like many early phase trials, drug evaluation has been implemented in patients with advanced, refractory disease. However, results of previous studies suggest that maximum immunotherapy efficacy is obtained when administered in the setting of minimal disease.Citation34 To this extent we have become interested in pursuing immunotherapy following surgery, which may create “minimal disease” state.
Given that platelets account for a major deposition of TGFβ into the wound at the time of surgery,Citation35 we predicted that beginning TGFβ blockade before would provide maximum benefits. As hypothesized, when 1D11 was administered before surgery, we observed much more dramatic inhibition of growth than when the antibody was begun after surgery. However, our model allowed us to observe a major complication associated with preoperative dosing—dramatic wound breakdown. These findings are consistent with the known role for TGFβ in wound healing,Citation35,Citation36 but were missed in our previous studies where TGFβ was not blocked in the presence of an active wound.Citation14 The use of the positive margin model was instrumental in confirming the theoretical hypothesis involving TGFβ inhibition’s impact on wounding, and will help in developing protocols that maximize benefits while minimizing complications.
The second adjuvant interrogated was a systemic inhibitor of cyclooxygenase-2 (COX-2). Cyclooxygenases catalyzes the rate-limiting step in the formation of prostaglandins which have known immunosuppressive roles.Citation37,Citation38 Two forms exist: the constitutive COX-1 and the inducible COX-2. Compared with COX-1, COX-2 leads to increased formation of PGE2 from arachidonic acid which appears to contribute to the cancer phenotype.Citation37,Citation38COX-2 inhibition presented a second therapeutic dilemma. Whereas we and others have clearly shown that blockade of COX-2 can inhibit primary tumor growth,Citation22,Citation33 COX-2 is strongly upregulated in the wound microenvironment after surgery where it presumably plays a positive role in wound healing and tumor recurrence.Citation3 Using our local recurrence model, we were able to experimentally test the effect of perioperative administration of the COX-2 inhibitor celecoxib. Like TGFβ blockade, perioperative celecoxib was effective in slowing tumor recurrence. However, in this case, no adverse wound healing effects were observed, suggesting that this approach has potential merit for testing in clinical trials involving surgery. When we treated recurrent tumors postoperatively, the efficacy of COX-2 inhibition was essentially lost. This highlights a particular issue as most surgeons are reluctant to utilize a COX-2 inhibitor perioperatively due bleeding concerns.
In summary, adjuvant therapies aimed at limiting postoperative local recurrences have unfortunately been inadequately evaluated in the past, primarily due to suboptimal models. Instead of testing adjuvant therapies in preclinical models that incorporate at least some of the complex effects of surgery and the positive margin microenvironment, data has been extrapolated from non-surgical models. It is possible that the lack of appropriate surgical models can explain the marginal efficacy of many adjuvant therapies.Citation39 This report demonstrates that the positive margin model is an efficient and biologically relevant preclinical tool useful for studying systemic and local therapies addressing post-operative cancer recurrences. We appreciated strong adjuvant effects of both COX-2 inhibitors and TGFβ blockade. More importantly, however, this model provided important information involving wounding issues and adjuvant timing which would have been overlooked in previous cancer models.
Materials and Methods
Animals
Female C57Bl/6 and BALB/c, (6–8 weeks old) mice were purchased from Charles River Laboratories, Inc. (Wilmington, MA). All mice were maintained in a pathogen-free animal facility for one week prior to experimentation. The Animal Care and Use Committees of the University of Pennsylvania approved all animal study protocols described in this publication, and experiments were conducted in compliance with the Guide for the Care and Use of Laboratory Animals.
Cell lines
The murine malignant mesothelioma cell line, AB12, was derived from an asbestos-induced tumor and has been previously described in detail.Citation40 The murine esophageal carcinoma cell line, AKR, was derived from mouse esophageal squamous epithelia with cyclin D1 overexpression via Epstein-Barr virus ED-L2 promoter in p53 deficient genetic backgrounds.Citation41 The murine lung cancer cell line, TC1, was derived from mouse lung epithelial cells immortalized with HPV-16 E6 and E7 and transformed with the c-Ha-ras oncogene.Citation42 Cell lines were regularly tested and maintained negative for Mycoplasma spp
Animal models
Mice were injected subcutaneously on the flank with 5x105 AKR tumor cells (C57Bl/6 mice), 1 × 106 AB12 cells (BALB/c mice), or 1.2 × 106 TC1 cells (C57Bl/6 mice) unless otherwise noted. Tumor cells for subcutaneous injections were suspended in 100 μL PBS. Tumor volume was calculated using the Equation (3.14 × long-axis × short-axisCitation2)/6.
Surgery
Mice with flank tumors were anesthetized using ketamine (80 mg/kg) and xyalazine (10 mg/kg) then shaved. A 1cm incision was made adjacent to the tumor. Full resection was achieved by excising 100% of the tumor (including vascular supply) using standard blunt dissection. Partial resections were completed in a similar manner; however, 5% of the tumor burden is left in situ with preservation of blood supply. Sterile silk 3–0 sutures were used to close wounds. Buprenorphine (0.1 mg/kg) was administered immediately following surgery and 6 h after as postoperative analgesia.
Transforming growth factor β (TGFβ) inhibitor, 1D11
The anti-murine TGFβ mAb, 1D11, neutralizes the three isoforms of TGFβ43, while the murine IgG1 mAb against Shigella toxin, 13C4, served as an isotype control. Antibodies were generously provided by Dr. Scott Lonning at Genzyme Oncology. Antibodies (1D11 and 13C4) were suspended in 0.2 ml of pH buffered soluation and administered intraperitoneally at a dose of 25 mg/kg. Once begun, antibodies were given three times per week for three weeks.Citation15,Citation18
COX-2 inhibition
Specific COX inhibition was achieved using the COX-2 inhibitor Celecoxib purchased from our hospital pharmacy. This was incorporated into mouse chow by Test Diet at a concentration of 0.1%. Mice were fed this chow for 2 weeks.
Immunohistochemical studies
Mice were euthanized at which time tumors were harvested and frozen in Tissue-Tek OCT compound (Sakura Finetek USA, Inc.) to be stored at -80°C. Five-micrometer sections were cut. Monoclonal antibodies against CD45 (anti-CD45) and Ly6G (anti-Ly6G) were obtained from BD Biosciences and immunohistochemical staining was performed according to established protocols. Tumor cell infiltrate was quantified by counting the number of positively staining cells in four high-powered ( × 400) fields. Five slides for each specimen were analyzed.
Flow cytometric analysis of tumors
Tumors were harvested and minced into fine pieces in digestion buffer containing 0.1 mg/mL DNase I and 2.0 mg/mL collagenase type IV (Sigma). Samples were incubated at 37°C for 30 min, filtered through a 70-μm filter, and washed twice with PBS. Fc receptors were blocked with anti-mouse CD16/CD32 antibodies (BD Biosciences PharMingen). Cells were washed, then stained for 30 min at 4°C with appropriate antibodies for flow cytometry: CD45-FITC (leukocytes), CD8-APC (CD8 T-cells), Ly6G-PE (neutrophils), CD11b-PerCP (myeloid cells) or F4/80-APC (mature macrophages) (BD Biosciences PharMingen). Flow cytometry was completed using a Becton Dickinson FACS Calibur flow cytometer, and analyzed using FlowJo software.
In vivo tumor neutralization (Winn) assay
Splenocytes were isolated from spleens (3 mice/group) that were harvested 10 d following surgical resection. CD8 T-cells were separated from the total splenocyte pool using MACS isolation system (CD8a [Ly-2] mouse MicroBeads; Miltenyi Biotec). Purity (> 95%) of separation was confirmed by fluorescence-activated cell sorter analysis. Fresh AB12 cells were mixed with CD8 T-cells in a ratio of 3 CD8 T-cells to 1 AB12 tumor cell. The resulting mixture (1.5x106 CD8 T-cells: 0.5 × 106 AB12 tumor cells) was injected subcutaneously into the flanks of 5 naïve BALB/c mice. A control group of 5 mice with AB12 cells alone (0.5 × 106 cells) were injected in an analogous fashion. Tumor size was assessed over the next 7 d.
In vivo depletion of CD8 T-cells
To deplete CD8 T-cells during treatment with COX-2 in our AB12 models, mice received i.p. injections of 200 µg of monoclonal antibodies purified from the anti-CD8 hybridoma 53–6.7 (obtained from the American Type Culture Collection). Antibodies were begun at the time of beginning COX-2 chow. Antibodies were suspended in 200 µL of saline and were given twice per week for 3 weeks.
Statistical analysis
Differences between two groups were compared by Student t-test. Three groups or more were analyzed with one-way analysis of variance (ANOVA) for multiple comparisons with appropriate post hoc testing. The Fisher exact test was used for categorical data. Results are expressed as the mean and the standard error of the mean, unless otherwise noted. “Time to disease progression” was defined as the time from surgery until the recurring tumor reached a volume of 500 mm3. Best-fit linear regressions for models were generated using time from surgery as the independent variable with mean tumor volume or surface area as dependent variables. A p < 0.05 was considered a statistically significant result.
Abbreviations: | ||
COX | = | cyclooxygenase |
PDGF | = | platelet derived growth factor |
TGFβ | = | transforming growth factor beta |
Additional material
Download Zip (498.1 KB)Disclosure of Potential Conflicts of Interest
No potential conflicts of interest were disclosed.
Acknowledgments
JDP was supported by an American Medical Association Seed Grant and the National Institutes of Health (TL1RR024133). LAA was supported from the Lavin Family Supporting Foundation. SS was supported by the National Institutes of Health (K12CA076931) and Society of Surgical Oncology (Clinical Investigator Award).
References
- Jemal A, Bray F, Center MM, Ferlay J, Ward E, Forman D. Global cancer statistics. CA Cancer J Clin 2011; 61:69 - 90; http://dx.doi.org/10.3322/caac.20107; PMID: 21296855
- Predina JD, Judy B, Aliperti LA, Fridlender ZG, Blouin A, Kapoor V, et al. Neoadjuvant in situ gene-mediated cytotoxic immunotherapy improves postoperative outcomes in novel syngeneic esophageal carcinoma models. Cancer Gene Ther 2011; 18:871 - 83; http://dx.doi.org/10.1038/cgt.2011.56; PMID: 21869822
- Roh JL, Sung MW, Park SW, Heo DS, Lee DW, Kim KH. Celecoxib can prevent tumor growth and distant metastasis in postoperative setting. Cancer Res 2004; 64:3230 - 5; http://dx.doi.org/10.1158/0008-5472.CAN-03-3050; PMID: 15126364
- Schultz GS, White M, Mitchell R, Brown G, Lynch J, Twardzik DR, et al. Epithelial wound healing enhanced by transforming growth factor-alpha and vaccinia growth factor. Science 1987; 235:350 - 2; http://dx.doi.org/10.1126/science.3492044; PMID: 3492044
- Schreiber K, Rowley DA, Riethmüller G, Schreiber H. Cancer immunotherapy and preclinical studies: why we are not wasting our time with animal experiments. Hematol Oncol Clin North Am 2006; 20:567 - 84; http://dx.doi.org/10.1016/j.hoc.2006.03.001; PMID: 16762725
- Smith MJ, Culhane AC, Killeen S, Kelly MA, Wang JH, Cotter TG, et al. Mechanisms driving local breast cancer recurrence in a model of breast-conserving surgery. Ann Surg Oncol 2008; 15:2954 - 64; http://dx.doi.org/10.1245/s10434-008-0037-5; PMID: 18622646
- Grinshtein N, Bridle B, Wan Y, Bramson JL. Neoadjuvant vaccination provides superior protection against tumor relapse following surgery compared with adjuvant vaccination. Cancer Res 2009; 69:3979 - 85; http://dx.doi.org/10.1158/0008-5472.CAN-08-3385; PMID: 19383917
- Broomfield S, Currie A, van der Most RG, Brown M, van Bruggen I, Robinson BW, et al. Partial, but not complete, tumor-debulking surgery promotes protective antitumor memory when combined with chemotherapy and adjuvant immunotherapy. Cancer Res 2005; 65:7580 - 4; PMID: 16140921
- DeLisser HM, Keirns CC, Clinton EA, Margolis ML. “The air got to it:” exploring a belief about surgery for lung cancer. J Natl Med Assoc 2009; 101:765 - 71; PMID: 19715038
- Stuelten CH, Barbul A, Busch JI, Sutton E, Katz R, Sato M, et al. Acute wounds accelerate tumorigenesis by a T cell-dependent mechanism. Cancer Res 2008; 68:7278 - 82; http://dx.doi.org/10.1158/0008-5472.CAN-08-1842; PMID: 18794114
- Rofstad EK, Mathiesen B, Henriksen K, Kindem K, Galappathi K. The tumor bed effect: increased metastatic dissemination from hypoxia-induced up-regulation of metastasis-promoting gene products. Cancer Res 2005; 65:2387 - 96; http://dx.doi.org/10.1158/0008-5472.CAN-04-3039; PMID: 15781654
- Alsousou J, Thompson M, Hulley P, Noble A, Willett K. The biology of platelet-rich plasma and its application in trauma and orthopaedic surgery: a review of the literature. J Bone Joint Surg Br 2009; 91:987 - 96; http://dx.doi.org/10.1302/0301-620X.91B8.22546; PMID: 19651823
- Suzuki E, Kapoor V, Cheung HK, Ling LE, DeLong PA, Kaiser LR, et al. Soluble type II transforming growth factor-beta receptor inhibits established murine malignant mesothelioma tumor growth by augmenting host antitumor immunity. Clin Cancer Res 2004; 10:5907 - 18; http://dx.doi.org/10.1158/1078-0432.CCR-03-0611; PMID: 15355924
- Suzuki E, Kim S, Cheung HK, Corbley MJ, Zhang X, Sun L, et al. A novel small-molecule inhibitor of transforming growth factor beta type I receptor kinase (SM16) inhibits murine mesothelioma tumor growth in vivo and prevents tumor recurrence after surgical resection. Cancer Res 2007; 67:2351 - 9; http://dx.doi.org/10.1158/0008-5472.CAN-06-2389; PMID: 17332368
- Ueda R, Fujita M, Zhu X, Sasaki K, Kastenhuber ER, Kohanbash G, et al. Systemic inhibition of transforming growth factor-beta in glioma-bearing mice improves the therapeutic efficacy of glioma-associated antigen peptide vaccines. Clin Cancer Res 2009; 15:6551 - 9; http://dx.doi.org/10.1158/1078-0432.CCR-09-1067; PMID: 19861464
- Kim S, Buchlis G, Fridlender ZG, Sun J, Kapoor V, Cheng G, et al. Systemic blockade of transforming growth factor-beta signaling augments the efficacy of immunogene therapy. Cancer Res 2008; 68:10247 - 56; http://dx.doi.org/10.1158/0008-5472.CAN-08-1494; PMID: 19074893
- Lee GT, Hong JH, Kwak C, Woo J, Liu V, Lee C, et al. Effect of dominant negative transforming growth factor-beta receptor type II on cytotoxic activity of RAW 264.7, a murine macrophage cell line. Cancer Res 2007; 67:6717 - 24; http://dx.doi.org/10.1158/0008-5472.CAN-06-4263; PMID: 17638882
- Terabe M, Ambrosino E, Takaku S, O’Konek JJ, Venzon D, Lonning S, et al. Synergistic enhancement of CD8+ T cell-mediated tumor vaccine efficacy by an anti-transforming growth factor-beta monoclonal antibody. Clin Cancer Res 2009; 15:6560 - 9; http://dx.doi.org/10.1158/1078-0432.CCR-09-1066; PMID: 19861451
- Fontana A, Galli L, Fioravanti A, Orlandi P, Galli C, Landi L, et al. Clinical and pharmacodynamic evaluation of metronomic cyclophosphamide, celecoxib, and dexamethasone in advanced hormone-refractory prostate cancer. Clin Cancer Res 2009; 15:4954 - 62; http://dx.doi.org/10.1158/1078-0432.CCR-08-3317; PMID: 19622584
- Hahn T, Alvarez I, Kobie JJ, Ramanathapuram L, Dial S, Fulton A, et al. Short-term dietary administration of celecoxib enhances the efficacy of tumor lysate-pulsed dendritic cell vaccines in treating murine breast cancer. Int J Cancer 2006; 118:2220 - 31; http://dx.doi.org/10.1002/ijc.21616; PMID: 16331615
- Howe LR, Subbaramaiah K, Patel J, Masferrer JL, Deora A, Hudis C, et al. Celecoxib, a selective cyclooxygenase 2 inhibitor, protects against human epidermal growth factor receptor 2 (HER-2)/neu-induced breast cancer. Cancer Res 2002; 62:5405 - 7; PMID: 12359744
- Haas AR, Sun J, Vachani A, Wallace AF, Silverberg M, Kapoor V, et al. Cycloxygenase-2 inhibition augments the efficacy of a cancer vaccine. Clin Cancer Res 2006; 12:214 - 22; http://dx.doi.org/10.1158/1078-0432.CCR-05-1178; PMID: 16397045
- Kruklitis RJ, Singhal S, Delong P, Kapoor V, Sterman DH, Kaiser LR, et al. Immuno-gene therapy with interferon-beta before surgical debulking delays recurrence and improves survival in a murine model of malignant mesothelioma. J Thorac Cardiovasc Surg 2004; 127:123 - 30; http://dx.doi.org/10.1016/j.jtcvs.2003.08.034; PMID: 14752422
- Bursuker I, North RJ. Generation and decay of the immune response to a progressive fibrosarcoma. II. Failure to demonstrate postexcision immunity after the onset of T cell-mediated suppression of immunity. J Exp Med 1984; 159:1312 - 21; http://dx.doi.org/10.1084/jem.159.5.1312; PMID: 6232336
- Shakhar G, Ben-Eliyahu S. Potential prophylactic measures against postoperative immunosuppression: could they reduce recurrence rates in oncological patients?. Ann Surg Oncol 2003; 10:972 - 92; http://dx.doi.org/10.1245/ASO.2003.02.007; PMID: 14527919
- Hoffman RM. The multiple uses of fluorescent proteins to visualize cancer in vivo. Nat Rev Cancer 2005; 5:796 - 806; http://dx.doi.org/10.1038/nrc1717; PMID: 16195751
- Kishimoto H, Zhao M, Hayashi K, Urata Y, Tanaka N, Fujiwara T, et al. In vivo internal tumor illumination by telomerase-dependent adenoviral GFP for precise surgical navigation. Proc Natl Acad Sci U S A 2009; 106:14514 - 7; http://dx.doi.org/10.1073/pnas.0906388106; PMID: 19706537
- Teicher BA. Transforming growth factor-beta and the immune response to malignant disease. Clin Cancer Res 2007; 13:6247 - 51; http://dx.doi.org/10.1158/1078-0432.CCR-07-1654; PMID: 17975134
- Yang L, Pang Y, Moses HL. TGF-beta and immune cells: an important regulatory axis in the tumor microenvironment and progression. Trends Immunol 2010; 31:220 - 7; http://dx.doi.org/10.1016/j.it.2010.04.002; PMID: 20538542
- Wallace A, Kapoor V, Sun J, Mrass P, Weninger W, Heitjan DF, et al. Transforming growth factor-beta receptor blockade augments the effectiveness of adoptive T-cell therapy of established solid cancers. Clin Cancer Res 2008; 14:3966 - 74; http://dx.doi.org/10.1158/1078-0432.CCR-08-0356; PMID: 18559619
- Fridlender ZG, Sun J, Kim S, Kapoor V, Cheng G, Ling L, et al. Polarization of tumor-associated neutrophil phenotype by TGF-beta: “N1” versus “N2” TAN. Cancer Cell 2009; 16:183 - 94; http://dx.doi.org/10.1016/j.ccr.2009.06.017; PMID: 19732719
- DeLong P, Tanaka T, Kruklitis R, Henry AC, Kapoor V, Kaiser LR, et al. Use of cyclooxygenase-2 inhibition to enhance the efficacy of immunotherapy. Cancer Res 2003; 63:7845 - 52; PMID: 14633712
- Tanaka T, Delong PA, Amin K, Henry A, Kruklitis R, Kapoor V, et al. Treatment of lung cancer using clinically relevant oral doses of the cyclooxygenase-2 inhibitor rofecoxib: potential value as adjuvant therapy after surgery. Ann Surg 2005; 241:168 - 78; PMID: 15622005
- Kim SK, Wu X, Ragupathi G, Gathuru J, Koide F, Cheung NK, et al. Impact of minimal tumor burden on antibody response to vaccination. Cancer Immunol Immunother 2011; 60:621 - 7; http://dx.doi.org/10.1007/s00262-011-0975-9; PMID: 21267719
- Wang XJ, Han G, Owens P, Siddiqui Y, Li AG. Role of TGF beta-mediated inflammation in cutaneous wound healing. J Investig Dermatol Symp Proc 2006; 11:112 - 7; http://dx.doi.org/10.1038/sj.jidsymp.5650004; PMID: 17069018
- Martinez-Ferrer M, Afshar-Sherif AR, Uwamariya C, de Crombrugghe B, Davidson JM, Bhowmick NA. Dermal transforming growth factor-beta responsiveness mediates wound contraction and epithelial closure. Am J Pathol 2010; 176:98 - 107; http://dx.doi.org/10.2353/ajpath.2010.090283; PMID: 19959810
- Taketo MM. Cyclooxygenase-2 inhibitors in tumorigenesis (part I). J Natl Cancer Inst 1998; 90:1529 - 36; http://dx.doi.org/10.1093/jnci/90.20.1529; PMID: 9790545
- Taketo MM. Cyclooxygenase-2 inhibitors in tumorigenesis (Part II). J Natl Cancer Inst 1998; 90:1609 - 20; http://dx.doi.org/10.1093/jnci/90.21.1609; PMID: 9811310
- Lacchetti C, Ioannidis J, Guyatt G. Surprising results of randomized trials. Most Major Basic Science and Preclinical Promises for Effective Interventions Disapppoint in Clinical Trials. In: Guyatt G RD, Meade MO, Cook DJ, ed. Users’ Guides to the Medical Literature: A Manual for Evidence-Based Clinical Practice. New York: McGraw-Hill, 2008.
- Davis MR, Manning LS, Whitaker D, Garlepp MJ, Robinson BW. Establishment of a murine model of malignant mesothelioma. Int J Cancer 1992; 52:881 - 6; http://dx.doi.org/10.1002/ijc.2910520609; PMID: 1459729
- Opitz OG, Harada H, Suliman Y, Rhoades B, Sharpless NE, Kent R, et al. A mouse model of human oral-esophageal cancer. J Clin Invest 2002; 110:761 - 9; PMID: 12235107
- Lin KY, Guarnieri FG, Staveley-O’Carroll KF, Levitsky HI, August JT, Pardoll DM, et al. Treatment of established tumors with a novel vaccine that enhances major histocompatibility class II presentation of tumor antigen. Cancer Res 1996; 56:21 - 6; PMID: 8548765
- Dasch JR, Pace DR, Waegell W, Inenaga D, Ellingsworth L. Monoclonal antibodies recognizing transforming growth factor-beta. Bioactivity neutralization and transforming growth factor beta 2 affinity purification. J Immunol 1989; 142:1536 - 41; PMID: 2537357