Abstract
Acute lymphoblastic leukemia (ALL) is a heterogeneous disorder, and primary drug resistance and relapse are thought to be the main causes for treatment failure in ALL patients. For these refractory or relapsed patients, there is an increasing demand to identify novel therapeutic approaches, which will highly rely on the use of xenotransplantation models in translational research. Given the critical role that the spleen plays in the hematopoiesis and lymphopoiesis in adult mice, intrasplenic inoculation of ALL cells into immunodeficient mice may represent a feasible route for leukemic xenotransplantation. In the present study, engraftments via intrasplenic inoculation in anti-mCD122 mAb conditioned NOD/SCID mice were achieved in 5 out of 11 cases, and the engrafted cells reconstituted a complete leukemic phenotype. The engrafted cells sustained the self-renewal capacity of leukemia-initiating cells as tested by serial xenotransplantation and can be used for evaluation of antileukemic drugs. These data suggest that the combination of intrasplenic inoculation and the targeted depletion of CD122+ cells could provide a novel approach for the xenotransplantation of ALL cells in NOD/SCID mice. Furthermore, this model can be used for stem cell research, long-term analysis of engraftment kinetics and in vivo drug tests.
Introduction
Acute lymphoblastic leukemia (ALL) is the most common form of childhood cancer and approximately 80% of affected children can be cured with high-dose chemotherapy.Citation1 Despite this relative success, ALL remains one of the most common causes of death in children and has a much poorer outcome in adults.Citation2 The majority of adults with ALL will ultimately relapse; in addition, up to 20% will have primary resistant disease.Citation3,Citation4 Therefore, there is an increasing demand to identify novel therapeutic approaches for the treatment of relapsed or refractory ALL patients, and these approaches will rely heavily on the use of xenotransplantation models in translational research.Citation5,Citation6
The nonobese diabetic/severe combined immunodeficiency (NOD/SCID) mouse strain, which lacks functional mature B and T cells, has defects in natural killer cells and has defects in complement and macrophage functions, has been shown to be receptive to engraftment of normal and malignant human hematopoietic cells.Citation7 The NOD/SCID model of ALL retains the phenotypic and genotypic characteristics of the original patient sample and provides an accurate representation of the human disease.Citation8 Over the past decades, accumulating technical strategies have made it possible to xenotransplant primary hematopoietic cells into immunodeficient mice, but the disease penetrance in the human leukemic xenotransplantation model is undoubtedly finite.Citation7,Citation9-Citation11 The challenge of xenotransplantation is likely due to the lack of a supportive microenvironment or to the host’s immune system preventing the transplanted cells from reaching their niche;Citation12 these possibilities should be taken into account when making additional technical refinements to this model.
The spleen in adult mice is not only an extramedullary organ where hematopoietic stem cells (HSCs) can reside,Citation13 but it is also the largest peripheral lymphoid organ that retains the ability to produce lymphocytes. Therefore, we hypothesized that, in addition to bone marrow, the spleen may function as a stem cell niche for leukemia initiating cells (LICs), or leukemic stem cells (LSCs), and facilitate the engraftment of ALL xenografts. In the present study, we report a previously undescribed ALL xenotransplantation model established via an intrasplenic route in unirradiated NOD/SCID mice. Furthermore, we validated the model’s efficiency in stem cell research and preclinical testing of antileukemic drugs by serial transplantation and in vivo drug treatment.
Results
Engraftments of ALL blasts
Engraftments via intrasplenic inoculation were achieved in 5 out of 11 cases, and the engraftment levels ranged from 55.45–91.48%, 70.10–98.33% and 35.05–78.26% in the spleen, bone marrow and peripheral blood, respectively (). In all engrafted cases, there was a persistent post-inoculation increase in human CD45+ cells in the peripheral blood () and a consistent correlation of engraftment levels between the spleen and the peripheral blood (p < 0.01) () as well as between the spleen and the bone marrow (p < 0.01) (). These correlations suggested that overt leukemia was progressively reestablished after intrasplenic inoculation.
Table 1. Engraftments of patient samples in NOD/SCID mice
Figure 1. Engraftment of acute lymphoblastic leukemia in anti-mCD122 mAb-pretreated NOD/SCID mice. (A) Consecutive monitoring of human engraftment by flow cytometry over time. Peripheral blood samples from mice transplanted with ALL samples were examined at different time points after transplantation. (B) Correlation of bone marrow (BM) and spleen engraftment after intrasplenic injection (p < 0.01). (C) Correlation of peripheral blood (PB) and spleen engraftment after intrasplenic injection (p < 0.01).
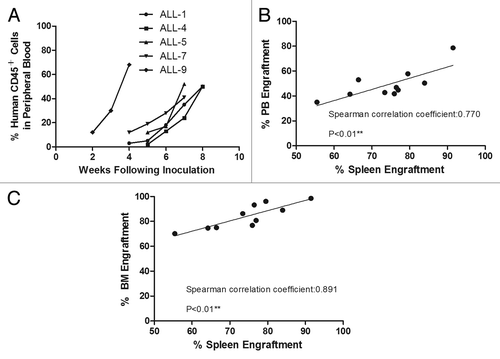
Consistent with the results from Fluorescence-activated cell sorting (FACS, ), both immunohistochemistry (IHC, ) and fluorescent in situ hybridization (FISH, ) revealed an infiltration of leukemia cells in the spleen, liver, lung, kidney and other organs at the endpoint. Furthermore, aberrant fusion genes derived from the patients were detected by RT-PCR in the established xenografts (), which indicated that ALL blasts inoculated in spleen are able to recapitulate the original diseases.
Figure 2. Evaluation of ALL engraftment in NOD/SCID mice at the endpoint. (A–D) Representative results from the FACS analysis of a NOD/SCID mouse transplanted with pro-B-ALL (case ALL-5). (A) The total nucleated cell population was gated on the basis of light scattering properties (FSC/SSC). Cells from the peripheral blood (B), spleen (C) and bone marrow (D) were stained with FITC-conjugated anti-human CD45 to calculate the percentage of human cells (hCD45+ %). (E–H) Representative results from IHC staining of the same mouse. Deparaffinized sections of the spleen (E), liver (F), lung (G) and kidney (J) were stained with anti-human CD45RB antibody (original magnification × 400). (I) FISH analysis of the infiltrated blasts. Cells were detected with a fluorescence-labeled probe and counterstained with DAPI. The probe used was p17H8, which is specific for centromeric repeat sequences on human chromosome 17. Only engrafted human cells will present two green signals, whereas the cells from mouse will not. (J) Nested PCR analysis of MLL/AF4 fusion transcript for the detection of xenotransplantation in the pro-B-ALL model (derived from case ALL-5, carrying MLL/AF4). The internal positive control E2A (690 bp) and amplified product (~450 bp) are shown in lanes 1 and 2, respectively.
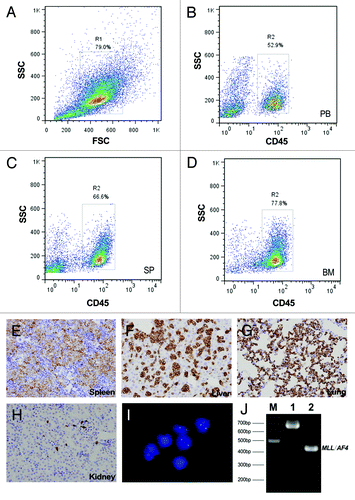
Serial transplantations
To demonstrate that in situ inoculation within the spleen could sustain the self-renewal capacity of LSCs in vivo, 3 passages of serial transplantations were performed in five engrafted cases. Except for case ALL-1 and case ALL-4, in which the engraftments accelerated at the secondary and tertiary passages (p < 0.05), respectively, there were no significant deviations in the disease latency or in the engraftment kinetics during the serial transplantations (). Immunophenotyping for each passage was also determined by flow cytometry. Although in a single engraftment case, ALL-1, a minor population (< 1%) acquired temporary expression of human CD33 on secondary passage that later diminished, expression of human CD2, CD19 and CD33 was stable up to three passages in NOD/SCID mice from all five engrafted cases. In addition, the genetic lesions of xenografts from different passages were also confirmed by RT-PCR as noted above. In brief, with no loss of engrafting capacity, the leukemia cells inoculated within the spleen recapitulated the original disease for up to three passages in serial transplantations.
Table 2. Rates of primary, secondary and tertiary engraftment
In vivo drug treatment
Because conserved disease phenotypes and observable splenomegaly were present in all engrafted cases, xenografts from case ALL-1 were chosen at random for use in in vivo drug treatments. The in vivo responses of the xenografts to vincristine and dexamethasone were designed to mimic chemotherapy on ALL patients. The leukemia cells took more than 4 weeks to reappear in the peripheral blood following the initiation of vincristine treatment, whereas only 2 weeks were required for reappearance in the dexamethasone-treated mice (). The overall survival of the vincristine group was notably longer than the survival of the dexamethasone and saline groups (p < 0.01, ), which indicates that while both vincristine and dexamethasone are able to retard the progression of engraftment and improve the survival rates, the xenografts were more sensitive to vincristine than to dexamethasone. These data were consistent with the clinical response of complete remission that was achieved when this patient was give VDLP regimen as his induction therapy.
Figure 3. In vivo drug treatment. Mice were inoculated with xenografts derived from case ALL-1 and treated with vincristine (V), dexamethasone (D) or saline control (S) as described in Materials and Methods. During the treatment, engraftment was monitored by continuous flow cytometry. Leukemia cells took more than 4 weeks to reappear in the peripheral blood following the initiation of vincristine treatment and took approximately 2 weeks in the dexamethasone-treated group (p < 0.01) (A). The EFS of NOD/SCID mice was quantified as the time from the initiation of treatment to the time that the leukemic population (hCD45+ %) reached 50% in the peripheral blood or to the time that the mice first showed indications of morbidity (> 20% weight loss, lethargy and ruffled fur). As demonstrated in this figure, both vincristine and dexamethasone were able to extend the survival (p < 0.01), although the xenografts were more sensitive to vincristine than to dexamethasone (B).
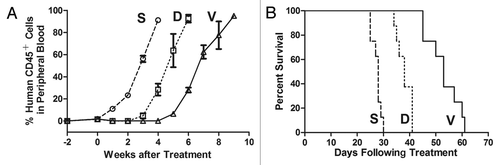
Discussion
Although human leukemic xenotransplantation in immunodeficient mice provides a current “gold-standard” system for the evaluation of LSCsCitation14,Citation15 and serves as an attractive assay for studies of leukemogenesisCitation12 and antileukemic therapy,Citation5 additional technical refinements may lead to higher levels of engraftment, decrease animal mortality, and a phenotype that more closely resembles human leukemia.Citation16 In this study, we present the first data indicating that intrasplenic inoculation of human primary ALL blasts are able to reconstitute a complete leukemic phenotype in anti-mCD122 mAb- (TM-β1) conditioned NOD/SCID mice, sustain the self-renewal capacity of LSCs and may be used for in vivo drug treatments.
Mazurier et al. previously reported that the inoculation of cells directly into the BM cavity of the femur allowed for a robust myeloerythroid graft at 2 weeks after transplantation, which indicated that an intrafemoral assay could provide an effective tool in stem cell research.Citation10 It is well known that stem cells are supported by an anatomical and functional structure referred to as the stem cell niche.Citation17 In the bone marrow, endosteal and perivascular regions have both been proposed to be niche sites, and osteoblasts and vascular endothelial cells are considered the major components in endosteal and perivascular niches, respectively.Citation18-Citation20 Using a NOD/SCID/IL2γg–/– (NOG) mouse model of human AML, Ishikawa et al. showed that LSCs homed to the endosteal niche of bone marrow, where they underwent cell cycle quiescence and obtained graft advantage over normal HSCs. Furthermore, the quiescent endosteal LSCs were responsible for the chemotherapeutic resistance and the subsequent AML relapse.Citation21,Citation22
In addition to the bone marrow, the spleen represents a homing site for stem cells. In rodents, the spleen remains hematopoietically active throughout life,Citation13 and HSCs function similarly in both the bone marrow and the spleen.Citation23 By contrast, the spleen does not support hematopoiesis after birth in humans.Citation13 Given that the spleen is an extramedullary organ supporting HSC in mice and that in situ injection within the spleen, in contrast to intravenous injection, can protect LSCs from transplant rejection prior to their homing, intrasplenic injection could represent a feasible route for leukemic xenotransplantation in immunodeficient mice. In the current study, 5 out of 11 ALL samples were able to engraft successfully via intrasplenic inoculation in serial transplantations and were able to infiltrate different organs progressively with time . Therefore, this model could facilitate the long-term analysis of engraftment kinetics.
Table 3. Clinical features for 11 patients with de novo ALL
The ability of some leukemia samples to engraft successfully is not only regulated by stem cell niche but is also limited by the residual elements of the recipient's immune system.Citation12 Induction of tolerance is likely essential for the successful xenotransplantation because of the vigorous immune responses across xenogeneic barriers. Sublethal irradiation of the mice before the adoptive transfer of leukemia cells is a conventional solution to overcome immunologic rejection and enhance the engraftment of stem cells. Nevertheless, it had been reported that irradiation triggers the upregulation of stromal cell-derived factor-1 (SDF-1) in stem cell niche and will thereby reduce the engraftment of ALL blasts in NOD/SCID mice.Citation24 Moreover, due to the epithelial damage of the intestines, irradiation-induced side effects of diarrhea and weight loss lead to increased mortality.Citation6
Pretreatment of NOD/SCID mice with anti-mCD122 mAb (TM-β1), an antibody against the β-chain of IL-2 receptor, is another practical strategy for induction of tolerance. The TM-β1 antibody is directed against both murine NK cells and macrophages but has no targets outside of innate immune cells.Citation25 Studies from McKenzie et al. demonstrated that, compared with all other strategies, leukemic engraftment was highest in anti-mCD122 mAb-conditioned, intrafemorally inoculated NOD/SCID mice at 2 and 6 weeks after transplantation, thereby indicating that targeted depletion of mCD122+ cells rendered the NOD/SCID strain more like the NOD/SCID-β2m–/– strain and lead to enhanced engraftment and reduced mortality.Citation16 Although a comparison of engraftment efficiency with conventional methods was not performed in this paper, no signs of illness or early death were observed prior to the appearance of human CD45+ in the peripheral blood of TM-β1 conditioned mice.
Taken together, the combination of intrasplenic inoculation and the targeted depletion of CD122+ cells provides a novel approach for the xenotransplantation of ALL cells in NOD/SCID mice and can be used for stem cell research, long-term analysis of engraftment kinetics and in vivo drug testing. Future studies are needed to evaluate human leukemic engraftment in various inoculation routes to determine the most effective strategy and assess the potential for the use of this model for the engraftment of human HSCs. Furthermore, this approach has promise as an in vivo assay for revealing the crosstalk between the stem cells and the spleen niche in both physiologic and pathologic conditions.
Materials and methods
Patients and leukemia cells
Mononuclear cells from bone marrow were collected from 11 adult patients with newly diagnosed ALL following a protocol approved by the Institutional Review Board for Human Research at the TongJi Hospital and Medical College at the Huazhong University of Science and Technology. The patients’ diagnoses were made according to the EGIL classification. Patients were selected only on the basis of availability of material for the study. The clinical characteristics of these patients are summarized in . Mononuclear cells were isolated by Ficoll-Hypaque gradient centrifugation (Sigma) and cryopreserved in liquid nitrogen in the presence of 90% fetal bovine serum (FBS, Gibco) and 10% dimethyl sulfoxide (DMSO) until inoculation. As confirmed by morphologic analysis and FACS screening, all the samples were composed of more than 80% leukemic blasts.
Intrasplenic inoculation of human leukemia cells
All animal experiments described in this study was performed in accordance with and approved by the Institutional Review Board for Animal Welfare at the TongJi Hospital and Medical College at the Huazhong University of Science and Technology. Female NOD/SCID mice, aged 3–4 weeks, were bred and maintained in a specific pathogen-free environment and supplied with sterile food and water. On the day before and the day of transplantation, mice were pretreated once each day intraperitoneally with 200 μg of anti-mouse CD122 monoclonal antibody (TM-β1, Rat IgG2b, Bio X cell). On the day of transplantation, leukemia cells were rapidly thawed, and 5–10 × 106 cells were suspended in 50 μl ice-cold PBS for each mouse. Mice were anesthetized with ketamine (60 mg/kg) and xylazine (9 mg/kg), and a subcostal incision into the skin was made followed by incisions of the abdominal wall and the peritoneum. The spleen was carefully exposed, and leukemia cells were injected slowly into the spleen using an insulin syringe with a 27-gauge needle (Fig. S1). After injection, the spleen was repositioned in the abdominal cavity, and the peritoneum, abdominal wall and skin were sutured separately.
Evaluation of engraftments in NOD/SCID mice
After inoculation, mice were returned to their cages and examined daily for general well-being. Engraftment was monitored by weekly blood collections and FACS analysis for human CD45+ cells. At the first indication of morbidity (> 20% weight loss, lethargy and ruffled fur), or no more than 16 weeks post-inoculation, mice were sacrificed by cervical dislocation. To ascertain the leukemic engraftment levels at this endpoint, the proportions of human CD45+ cells (hCD45+ %) in bone marrow, peripheral blood, and spleen were quantified by flow cytometry. Engraftment of human cells was also evaluated by IHC and FISH. To confirm that our mouse model recapitulated the original disease, immunophenotype and genetic characteristics were inspected by flow cytometry and RT-PCR, respectively. The procedures mentioned above were performed as previously described.Citation26-Citation28
Serial transplantations
To demonstrate that in situ inoculation within the spleen could sustain the self-renewal capacity of LSC in vivo, serial transplantations were performed. Engrafted cells were purified from the minced spleens of established primary models by Ficoll-Hypaque gradient centrifugation and cryopreserved in FBS containing 10% DMSO. Prior to inoculation, cells were thawed and processed exactly as described for primary cells. For a comparison of serial transplantations, equal numbers (5–10 × 106) of thawed cells were inoculated into the mice in each case.
In vivo drug treatments
Inoculation and engraftment monitoring were performed as described above. When the proportion of hCD45+ cells in the peripheral blood reached 1%, mice were randomized to receive vincristine (1 mg/kg every 7 d for 4 weeks), dexamethasone (20 mg/kg Monday–Friday for 4 weeks) or the equivalent volume of saline intraperitoneally. The percent of hCD45+ cells in the peripheral blood was monitored weekly during the course of treatment. At the first indication of morbidity (> 20% weight loss, lethargy and ruffled fur), the mice were killed by cervical dislocation.
Statistical analysis
For statistical analyses in serial transplantations and in drug treatments, the event-free survival (EFS) was calculated from the initiation of treatment. Data for each sample were reported as the mean ± SD. The significance of the difference between the groups was determined using the matched paired t-tests.
Abbreviations: | ||
ALL | = | acute lymphoblastic leukemia |
NOD/SCID | = | nonobese diabetic/severe combined immunodeficiency |
HSC | = | hematopoietic stem cell |
LIC | = | leukemia initiating cell |
LSC | = | leukemic stem cell |
FACS | = | fluorescence-activated cell sorting |
IHC | = | immunohistochemistry |
FISH | = | fluorescent in situ hybridization |
NOG | = | NOD/SCID/IL2γg–/– |
SDF-1 | = | stromal cell-derived factor-1 |
FBS | = | fetal bovine serum |
EFS | = | event-free survival |
Additional material
Download Zip (124.5 KB)Disclosure of Potential Conflicts of Interest
No potential conflicts of interest were disclosed.
Acknowledgments
This study was supported by grants from the Major Program of the National Natural Science Foundation of China (81090414), the National Science Fund for Distinguished Young Scholars (81025011) and the Major State Basic Research Development Program (2009CB521806).
Supplemental Material
Supplemental material may be found here:
References
- Pui CH, Evans WE. Treatment of acute lymphoblastic leukemia. N Engl J Med 2006; 354:166 - 78; http://dx.doi.org/10.1056/NEJMra052603; PMID: 16407512
- Chatenoud L, Bertuccio P, Bosetti C, Levi F, Negri E, La Vecchia C. Childhood cancer mortality in America, Asia, and Oceania, 1970 through 2007. Cancer 2010; 116:5063 - 74; http://dx.doi.org/10.1002/cncr.25406; PMID: 20629033
- Ribera JM. Advances in acute lymphoblastic leukemia in adults. Curr Opin Oncol 2011; 23:692 - 9; http://dx.doi.org/10.1097/CCO.0b013e32834bb854; PMID: 21892082
- Gökbuget N, Hoelzer D. Treatment of adult acute lymphoblastic leukemia. Semin Hematol 2009; 46:64 - 75; http://dx.doi.org/10.1053/j.seminhematol.2008.09.003; PMID: 19100369
- Liem NL, Papa RA, Milross CG, Schmid MA, Tajbakhsh M, Choi S, et al. Characterization of childhood acute lymphoblastic leukemia xenograft models for the preclinical evaluation of new therapies. Blood 2004; 103:3905 - 14; http://dx.doi.org/10.1182/blood-2003-08-2911; PMID: 14764536
- Lock RB, Liem NL, Papa RA. Preclinical testing of antileukemic drugs using an in vivo model of systemic disease. Methods Mol Med 2005; 111:323 - 34; PMID: 15911988
- Shultz LD, Ishikawa F, Greiner DL. Humanized mice in translational biomedical research. Nat Rev Immunol 2007; 7:118 - 30; http://dx.doi.org/10.1038/nri2017; PMID: 17259968
- Cox CV, Evely RS, Oakhill A, Pamphilon DH, Goulden NJ, Blair A. Characterization of acute lymphoblastic leukemia progenitor cells. Blood 2004; 104:2919 - 25; http://dx.doi.org/10.1182/blood-2004-03-0901; PMID: 15242869
- McCormack E, Bruserud O, Gjertsen BT. Animal models of acute myelogenous leukaemia - development, application and future perspectives. Leukemia 2005; 19:687 - 706; http://dx.doi.org/10.1038/sj.leu.2403670; PMID: 15759039
- Mazurier F, Doedens M, Gan OI, Dick JE. Rapid myeloerythroid repopulation after intrafemoral transplantation of NOD-SCID mice reveals a new class of human stem cells. Nat Med 2003; 9:959 - 63; http://dx.doi.org/10.1038/nm886; PMID: 12796774
- Cheung AM, Fung TK, Fan AK, Wan TS, Chow HC, Leung JC, et al. Successful engraftment by leukemia initiating cells in adult acute lymphoblastic leukemia after direct intrahepatic injection into unconditioned newborn NOD/SCID mice. Exp Hematol 2010; 38:3 - 10; http://dx.doi.org/10.1016/j.exphem.2009.10.007; PMID: 19837128
- Kennedy JA, Barabé F. Investigating human leukemogenesis: from cell lines to in vivo models of human leukemia. Leukemia 2008; 22:2029 - 40; http://dx.doi.org/10.1038/leu.2008.206; PMID: 18685615
- Purton LE, Scadden DT. The hematopoietic stem cell niche (November 15, 2008), StemBook, ed. The Stem Cell Research Community, StemBook, doi/10.3824/stembook. 1.28.1, http://www.stembook.org.
- Guenechea G, Gan OI, Dorrell C, Dick JE. Distinct classes of human stem cells that differ in proliferative and self-renewal potential. Nat Immunol 2001; 2:75 - 82; http://dx.doi.org/10.1038/83199; PMID: 11135582
- Castor A, Nilsson L, Astrand-Grundström I, Buitenhuis M, Ramirez C, Anderson K, et al. Distinct patterns of hematopoietic stem cell involvement in acute lymphoblastic leukemia. Nat Med 2005; 11:630 - 7; http://dx.doi.org/10.1038/nm1253; PMID: 15908956
- McKenzie JL, Gan OI, Doedens M, Dick JE. Human short-term repopulating stem cells are efficiently detected following intrafemoral transplantation into NOD/SCID recipients depleted of CD122+ cells. Blood 2005; 106:1259 - 61; http://dx.doi.org/10.1182/blood-2005-03-1081; PMID: 15878972
- Jones DL, Wagers AJ. No place like home: anatomy and function of the stem cell niche. Nat Rev Mol Cell Biol 2008; 9:11 - 21; http://dx.doi.org/10.1038/nrm2319; PMID: 18097443
- Calvi LM, Adams GB, Weibrecht KW, Weber JM, Olson DP, Knight MC, et al. Osteoblastic cells regulate the haematopoietic stem cell niche. Nature 2003; 425:841 - 6; http://dx.doi.org/10.1038/nature02040; PMID: 14574413
- Zhang J, Niu C, Ye L, Huang H, He X, Tong WG, et al. Identification of the haematopoietic stem cell niche and control of the niche size. Nature 2003; 425:836 - 41; http://dx.doi.org/10.1038/nature02041; PMID: 14574412
- Kiel MJ, Yilmaz OH, Iwashita T, Yilmaz OH, Terhorst C, Morrison SJ. SLAM family receptors distinguish hematopoietic stem and progenitor cells and reveal endothelial niches for stem cells. Cell 2005; 121:1109 - 21; http://dx.doi.org/10.1016/j.cell.2005.05.026; PMID: 15989959
- Ishikawa F, Yoshida S, Saito Y, Hijikata A, Kitamura H, Tanaka S, et al. Chemotherapy-resistant human AML stem cells home to and engraft within the bone-marrow endosteal region. Nat Biotechnol 2007; 25:1315 - 21; http://dx.doi.org/10.1038/nbt1350; PMID: 17952057
- Saito Y, Uchida N, Tanaka S, Suzuki N, Tomizawa-Murasawa M, Sone A, et al. Induction of cell cycle entry eliminates human leukemia stem cells in a mouse model of AML. Nat Biotechnol 2010; 28:275 - 80; PMID: 20160717
- Morita Y, Iseki A, Okamura S, Suzuki S, Nakauchi H, Ema H. Functional characterization of hematopoietic stem cells in the spleen. Exp Hematol 2011; 39:351 - 9, e3; http://dx.doi.org/10.1016/j.exphem.2010.12.008; PMID: 21185906
- Spiegel A, Kollet O, Peled A, Abel L, Nagler A, Bielorai B, et al. Unique SDF-1-induced activation of human precursor-B ALL cells as a result of altered CXCR4 expression and signaling. Blood 2004; 103:2900 - 7; http://dx.doi.org/10.1182/blood-2003-06-1891; PMID: 15070661
- Shultz LD, Banuelos SJ, Leif J, Appel MC, Cunningham M, Ballen K, et al. Regulation of human short-term repopulating cell (STRC) engraftment in NOD/SCID mice by host CD122+ cells. Exp Hematol 2003; 31:551 - 8; http://dx.doi.org/10.1016/S0301-472X(03)00076-6; PMID: 12829032
- Pallisgaard N, Hokland P, Riishøj DC, Pedersen B, Jørgensen P. Multiplex reverse transcription-polymerase chain reaction for simultaneous screening of 29 translocations and chromosomal aberrations in acute leukemia. Blood 1998; 92:574 - 88; PMID: 9657758
- Wu J, Huang L, Huang M, Liu W, Zheng M, Cao Y, et al. Dominant contribution of malignant endothelial cells to endotheliopoiesis in chronic myeloid leukemia. Exp Hematol 2009; 37:87 - 91; http://dx.doi.org/10.1016/j.exphem.2008.08.009; PMID: 18951692
- Wang N, Xiao F, Liu JP, Wang D, Geng Z, Wang J, et al. SLAM family predicting the initiation potential of human acute lymphoblastic leukemia in NOD/SCID mice. Chin Med J (Engl) 2011; 124:3074 - 9; PMID: 22040559