Abstract
Tumor-associated stroma (TAS) is not simply a supporting element for cancer cells, but plays an important role in tumor growth, invasion and metastasis. Changes on the level of stromal constituents, such as loss of Caveolin-1 and increased thymidine phosphorylase (TP) expression, have been associated with tumor aggressiveness. The mutual cooperation between stromal fibroblasts and cancer cells is another distinguishing feature, which has recently emerged. In this investigation, both the loss of Caveolin-1 and the increased TP expression in the prostatic TAS was associated with high Gleason score (p = 0.0002 and 0.003, respectively); the two proteins were acting both independently and synergistically. In addition, TP was significantly associated with high stromal Ki-67 (MIB1) proliferation index (p = 0.03). Analysis of the metabolic interactions between stromal and epithelial elements showed that, while prostatic cancer cells express principally (> 91%) lactate dehydrogenase-5 (LDH-5) (anaerobic metabolism), the tumor-associated fibroblasts/myofibroblasts (TAFs) express largely (67.8%) LDH-1 (aerobic metabolism)—the terms TAFs and TAS are used interchangeably. These two isoenzyme pathways act complementary; the LDH-5 pathway converts pyruvate to lactate, whereas the LDH-1 enzyme system utilizes the secreted metabolite lactate to produce pyruvate, essential for continuous energy supply to tumor cells. Monocarboxylate transporter-1 (MCT-1)—the main facilitator of lactate uptake in tumor cells, was expressed exclusively in prostate cancer cells and related directly to LDH-5 overexpression. These findings support and extend our previous studies on energy recycling between the aerobic stroma and the anaerobic cancer cells within the framework of Warburg effect.
Introduction
The stromal-epithelial interactions are becoming increasingly important in tumor pathology, as they have gradually come to assume a dominant role in tumor growth, invasion and metastasis [reviewed in refs. Citation1 and Citation2]. In rat and mouse prostatic carcinogenesis, for example, testosterone and estradiol together may induce malignant transformation but only in the presence of tumor-associated fibroblasts/myofibroblasts (TAFs).Citation3 (The terms TAFs and tumor-associated stroma/TAS will be used interchangeably). Similarly, co-transfection of both stromal and epithelial cells of the urogenital sinus with ras and myc oncogenes is essential for epithelial prostatic carcinogenesis,Citation4 while loss of the transforming growth factor-β (TGF-β) responsiveness in fibroblasts results in prostatic intraepithelial neoplasia.Citation5 Furthermore, fibroblasts obtained from human prostatic carcinomas, but not normal prostatic fibroblasts, promote malignant transformation of initiated human epithelial cells from benign prostatic hyperplasia.Citation6
Indeed, TAFs exhibit a distinct protein profile compared with stromal cells of the adjacent normal or hyperplastic epithelium. Overexpression of stromal proteins SPARC/osteonectin, thymidine phosphorylase (TP) and collagen types III and IV has been linked with tumor aggressiveness.Citation7-Citation9 It has been also reported, recently, that the loss of Caveolin-1, a principal structural protein of the plasma membrane expressed in normal and hyperplastic fibroblasts,Citation10 is intimately related to malignant growth.Citation11 Others found that Caveolin-1 favors cell elongation and promotes force-dependent contraction, matrix alignment and microenvironment stiffening to facilitate tumor invasion.Citation12
In addition, the metabolic enzyme profile expressed by TAFs (mainly lactate dehydrogenase-B and pyruvate dehydrogenase), while different to that expressed by cancer cells (largely lactate dehydrogenase-A, pyruvate dehydrogenase-kinase and hypoxia inducible factor-1α),Citation13,Citation14 is essentially complementary. Thus, TAFs, by having high LDH-1 levels, convert lactate to pyruvate (aerobic metabolism), which is then taken up by cancer cells to draw energy production after conversion of pyruvate to lactate (anaerobic metabolism) via the LDH-5 pathway.
In this investigation, we studied the important constituents of prostatic TAS, namely Caveolin-1 and TP, and the isoenzymes LDH-1 and LDH-5 regulating tumor cell and TAFs metabolism in relation to tumor aggressiveness. The role of lactate and pyruvate monocarboxylate transporter-1 (MCT1) in these tissues was also explored. The proliferation index Ki-67 (MIB1) in the prostatic TAS was evaluated.
Results
TP, Caveolin-1 and MIB1 expression in TAF
Strong TP expression was evident from 0–90% (median 10%) per optical field in tumor-associated fibroblasts and myofibroblasts (TAFs). Using the median value as a cut-off point, prostatic cancer cases were grouped into low (< 10%) and high (≥ 10%) reactivity; in fact, 54/96 (56.2%) cases were of high stroma TP reactivity ().
Figure 1. Metabolic interactions between tumor cells and tumor-associated stroma (TAS) in prostatic cancer. (DAB chromogen for (A–E); fast red chromogen for (F). Magnification x200). (A) Loss of Caveolin-1 in prostatic TAS (arrows). (B) Retention of Caveolin-1 expression in prostatic TAS (arrows). (C) Hyperplastic prostatic tissue (Control). Note high Caveolin-1 expression in the non-neoplastic stroma (arrows). (D) High LDH-1 expression in prostatic TAS (arrows). Note absence of tumor cell immunoreactivity. (E) High LDH-5 expression in tumor cells. Note absence of immunoreactivity in TAS (arrows). (F) High TP reactivity in TAS arrows). (G) Membranous MCT1 expression in prostate cancer cells without TAS reactivity.
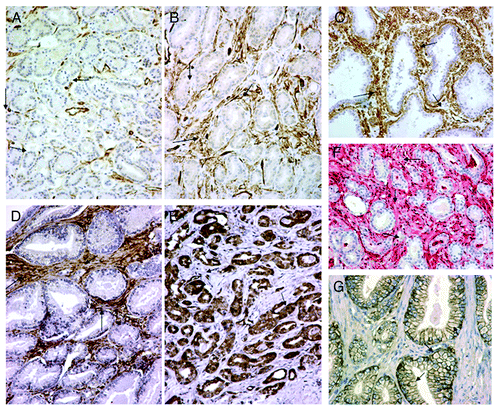
Caveolin-1 was largely downregulated in TAFs (), relative to hyperplastic prostatic stroma (). Strong expression was detected from 0–90% (median 20%) per optical field (). The median value was used as a cut-off point to define categories of low (< 20%) and high (≥ 20%) Caveolin-1 reactivity; thus, 36/96 (37.5%) cases showed considerable reduction or loss of stroma Caveolin-1.
The proliferation index Ki-67 (MIB1) was very low in prostatic TAFs and in most cases (over 70 of the 96) was completely lacking. The recorded nuclear values ranged from 0–10% (median 1%). The 66th upper percentile score (3) was used to separate cases into low (< 3%) and high (≥ 3%) reactivity; 27/96 (28.1%) cases were shown to have high stroma MIB1 proliferation index. MIB1 expression was not observed in fibroblasts of hyperplastic areas.
LDH-1 and LDH-5 expression in TAF
LDH-1 was the dominant feature in TAS (). It was expressed in almost 70% (65/96 cases, i.e., 67.8%) with a range of 15–95% and a median 50%). The remaining cases (31/96, 32.3%) lacked LDH-1 expression. By contrast, LDH-5 was detected in less than a third in TAS (). It was seen in 27/96 of prostate cancer cases, i.e., 28.1%, with a range of 10–60% and a median 20%.
Interestingly, the extent of LDH-1 expression in TAS was directly associated with the expression of LDH-5 in cancer cells (p = 0.03, r = 0.22). No association with Caveolin-1, TP or Gleason score was noted. A direct association was also noted between stromal and cancer cell LDH-5 expression, especially in relation to nuclear expression (p < 0.0001, r = 0.40). There was no association with Gleason score.
LDH-1 and LDH-5 expression in cancer cells
LDH-5 was the dominant feature in the cytoplasm and nuclei of prostatic cancer cells. Over 87/96 cases, i.e., 90.6%, stained positively for LDH-5. The median proportion of cancer cells expressing cytoplasmic LDH-5 was 60% (range 0–100%), while that of the nucleus was 15% (range 0–70%). Lack of LDH-5 expression was noted in only 9/96 (9.4%) cases. In linear regression analysis, a direct association between cytoplasmic LDH-5 expression and high Gleason score was established (p = 0.006, r = 0.26). No analogous association with Caveolin-1, TP or MIB1 expression was observed.
There was a distinct lack of LDH-1 in prostatic cancer cells; in over two-thirds (66/96) of cases LDH-1 was not expressed by prostate cancer cells (range 0–100%, median 0%). LDH-1 reactivity in > 50% was noted in only 10/96 cases (10.5%), with the majority of cancer cells exhibiting simultaneous overexpression of LDH-5.
Association of TP and Caveolin-1 in TAS with Gleason score
shows the association of TP and Caveolin-1 with Gleason score and the other variables analyzed. A high TP expression (≥ 10%) and a loss or considerable reduction of Caveolin-1 (< 20%) in TAS were both associated with high Gleason score (p = 0.0002 and p = 0.003, respectively). In addition, the high TP stromal expression was associated with an increase fibroblast MIB1 proliferation index (p = 0.03).
Table 1. Association of TP and Caveolin-1 with Gleason score and other variables studied
Linear regression analysis of TP and Caveolin-1 vs. Gleason score, using the data as continuous variables, confirmed a significant direct association of TP with Gleason score (p = 0.001, r2 = 0.11), and an inverse association of Caveolin-1 expression with Gleason score (p < 0.0001; r2 = 0.17; ). Unpaired analysis of Gleason score used as a continuous variable showed similar significant associations ().
Figure 2. Association of Gleason score with TP and Caveolin-1. (A) Linear regression analysis. (B) Group analysis. (C) Combined Caveolin-1/TP group analysis. Bars and whiskers show the median value and range as well as the 25th and 75th percentiles.
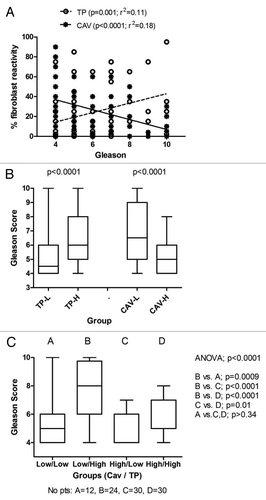
Double stratification analysis (Caveolin-1/TP vs. Gleason), showed that only cases exhibiting simultaneously low Caveolin-1 and high TP expression had a high Gleason score (p < 0.0009), while low Caveolin-1 expression per se did not have any impact. High TP, even in the context of high Caveolin-1 expression showed a weaker, but still significant, association (p = 0.01) with high Gleason score (). The stronger impact of high TP on Gleason score was also confirmed in a bivariate regression analysis to assess the independence of the above association. Stroma TP expression was an independent predictive variable of high Gleason score (p = 0.001, hazard ratio 6.3 (2.1–19.2). Stroma Caveolin-1 expression was also an independent, though weaker, predictor of low Gleason Score (p = 0.01, hazard ratio 0.3 (0.1–0.8).
There was no association of the proliferation index MIB1 in TAFs with Gleason score, T-stage, or patient's age.
Caveolin-1 and TP vs. LDH-1 and LDH-5 in TAS
Tumors with considerable reduction or loss of stromal Caveolin-1 (36/96 cases) tended to express LDH-1 (15/36, 41.6%) rather than LDH-5; in fact, only one case expressed LDH-5, whereas 35/36 (97.2%) did not. The high stromal TP reactivity cases (54/96 cases) was associated closer with high LDH-1 expression (22/54 (40.7%), with only one case (1/54, 2%) expressing high LDH-5 reactivity.
MCT-1 expression
A distinct MCT1 membranous expression was detected in a varying proportion of prostatic cancer cells, ranging from 0–80% (median 20%); . There was complete lack of MCT1 staining in TAS. Correlation analysis revealed a significant association between MCT1 expression and strong cytoplasmic LDH5 reactivity (p = 0.002. r = 0.40).
Multivariate analysis of cancer cells and TAS to Gleason score
Multivariate analysis of all variables studied according to Gleason score (< 7 vs. > 7) showed an independent association with stromal TP (p = 0.001, t-ratio 3.22) and Caveolin-1 expression (p = 0.01, t-ratio 2.45). In multivariate analysis according to capsular invasion, none of the variables analyzed showed any significant association.
Discussion
The tumor microenvironment plays a critical role in the process of tumor initiation and progression. To this end, thymidine phosphorylase (TP) and Caveolin-1 were shown to have an important part. In addition, the isoenzymes lactate dehydrogenase-1 and -5 (LDH1 and LDH5) appears to organize tumor cells and tumor-associated fibroblasts (TAS) into a harmonious cooperative metabolic domain, ensuring the survival of tumor cells.13
Thymidine phosphorylase (TP) is a notable angiogenic factor stimulating new blood vessel formation in many human malignancies, including prostate adenocarcinoma.Citation16,Citation17 The enzyme is primarily expressed by tumor-associated fibroblasts and myofibroblasts (TAFs) and, to a lesser extent, by cancer cells and macrophages.Citation18,Citation19 A high TP expression is frequently associated with high angiogenesis and with adverse prognostic factors in prostate carcinomas, reflecting an aggressive phenotype.Citation8,Citation17 Indeed, in this study, an intense stromal TP reactivity in > 10% of TAFs was significantly associated with high MIB1 proliferation index and with high Gleason score; the latter being one of the most important predictors of poor clinical outcome.
Caveolin-1, a plasma membrane protein residing in specialized lipid rafts called caveolae,Citation10 is consistently expressed in the normal and hyperplastic prostate stroma, but is considerably reduced and often completely lost in TAS - a phenomenon which was recently associated with advanced prostatic cancer and metastatic disease.Citation20 Such a loss or reduction of stromal Caveolin-1 in prostatic cancer, relative to hyperplastic stroma, was convincingly shown in the present investigation. Thus, a reduction of stromal Caveolin-1 in more than 80% of the TAS was related with high Gleason score and, marginally, with capsular invasion. Comparative analysis of low Caveolin-1 levels with high TP expression revealed that the co-existence of both defined a stronger relation with Gleason score. This probably suggests that, although the loss of stromal Caveolin-1 is closely associated with aggressiveness, alternative pathways are equally involved in the matter.
Analysis of LDH isoenzymes showed that cancer cases with loss of Caveolin-1 only exceptionally expressed the anaerobic enzyme LDH-5 in TAS, whereas the same tumors demonstrated in the same compartment upregulation of the aerobic enzyme LDH-1 in nearly 40% of cases. It is, therefore, most unlikely that TAS with loss of Caveolin-1 may sustain an aerobic metabolism, as previously suggested.Citation21 Interestingly, the very same conclusions were reached for the prostatic TAS in the presence of Caveolin-1. Thus, prostate adenocarcinomas, while commonly lacking stromal LDH-5, showed upregulation of LDH-1 in over two thirds of cases, irrespective of the absence or otherwise of Caveolin-1 in the prostatic TAS. Furthermore, the expression of LDH-5 in cancer cells was as high as 90%, whereas the same cell population displayed a rather low LDH-1 incidence. These findings were taken to mean that TAFs utilize lactic acid to produce pyruvate, which can then be taken up by cancer cells to promote lactic acid/energy production via anaerobic metabolism. Continuous acquisition of energy through this recycling mechanism constitutes the Warburg effect. This is further supported by the overexpression of MCT-1, a lactate transporter, in cancer cell membranes (never in TAS), a pattern that was significantly associated with LDH5 overexpression and, hence, lactate production. MCT1 eventually facilitates lactate extrusion in highly glycolytic cancer cells to avoid cell death from intracellular acidosis.
Yet, Pavlides et al. introduced the term “reverse Warburg effect” to express the view that anaerobic metabolism takes place in the “fibroblastic” tumor compartment, rather than in the epithelial cancer cells themselves.Citation22 They have drawn their conclusion after displaying upregulation of glycolytic enzymes in Caveolin-1(−/−) null bone-marrow-derived stromal cells—a finding which could not be substantiated in our immunohistochemical study. This is in accord with our earlier findings in lung and colon cancer, where tumor cells have increased HIF1α, LDH-5, pyruvate dehydrogenase kinase (PDH-kinase) and GLUT1 expression, while the adjacent stroma principally expressed LDH-1 and PDH in the absence of HIF1α.Citation13,Citation14 Along these lines, Rattigan et al. have recently indicated that fibroblasts of the tumor microenvironment have the capacity to take up lactate expelled from tumor cells and use it as a source of energy.Citation23 Nuclear magnetic resonance (NMR) spectroscopic measurements indicated that lactate is converted to α ketoglutarate by TAFs.
It is concluded that TAS plays an important role in growth and development of prostatic cancer. Loss of Caveolin-1 and overexpression of TP are markers of TAS, closely associated with high Gleason score. Tumor stroma, in contrast to cancer cells, exhibits an aerobic profile of LDH isoenzyme expression with overexpression of LDH-1 and underexpression or lack of LDH-5. These features involve the vast majority of cancer cases, independently of Caveolin-1 or TP expression, highlighting the metabolic co-operation between the aerobic stroma and the anaerobic cancer cells, as Warburg originally suggested (Warburg effect).Citation23 Whether an “inverse Warburg effect” with distinct metabolic stroma/cancer cell interactions exists in a proportion of prostatic tumors or in certain tumor types needs further evaluation.Citation24
Patients and Methods
A series of 96, node negative, prostate cancer specimens obtained from patients who underwent radical prostatectomy at the University General Hospital of Alexandroupolis (Alexandroupolis, Greece) were analyzed. The median age of patients was 67 years (range 44–88) and the median Gleason score was 5 (range 5–10). The tumors were confined to the prostatic gland (stage T1,2) in 77/96 (80.2%) cases, and were invading the prostatic capsule and/or the seminal vesicles (stage T3) in 19/96 (19.8%).
Immunohistochemistry for Caveolin-1, TP and Ki-67
The prostate carcinomas were stained immunohistochemically for the proteins Caveolin-1, TP and MIB1 using the automated Bond-max system (Leica Microsystems). Sections were cut at 3 μm from formalin-fixed paraffin-embedded tissues and dried for 30 min at 80°C. The slides were then covered by Bond Universal Covertiles (Leica Microsystems) and placed into the Bond-max instrument. This was followed by deparaffinization of tissue on the slides with Bond Dewax Solution (Leica Microsystems) at 72° for 15 min; heat-induced antigen retrieval with Bond Epitope Retrieval Solution 2 (Leica Microsystems) for 30 min at 100°C; peroxide block placement on the slides for 5 min at room temperature; and incubation with the primary rabbit polyclonal antibody to Caveolin-1 (ab 18199, Abcam) at dilution 1:700 for 30 min at room temperature. For the detection of TP the tissues were treated with the primary mouse monoclonal antibody P-GF.44.C (University of Oxford, UK)Citation15 at dilution 1:100 for 30 min. For the detection of Ki-67, the MIB1 primary antibody (DAKO) was used at dilution 1:75 for 20 min. The subsequent steps of the procedure were performed at room temperature and included: incubation with Post Primary reagent (Leica Microsystems) for 10 min if it was for Caveolin-1 or 20 min for TP; Bond Polymer (Leica Microsystems) placement on the slides for 10 min for Caveolin-1 or 20 min for TP; and development of color reaction, i.e., 3,3′-diaminobenzidine tetrahydrochloride (DAB) for Caveolin-1 (10 min); fast red chromogen for TP (8 and 4 min, sequentially). The sections were then counterstained with hematoxylin for 5 min, dehydrated and mounted.
Controls
Known positive tissues for Caveolin-1, TP and MIB1 were used as controls. Normal immunoglobulin-G was substituted for the primary antibody as negative control. Staining with omission of the primary antibody was also performed as negative control.
Immunohistochemistry for LDH-1, LDH-5 and MCT-1
Sections, 3 μm thick, were deparaffinized and placed in antigen retrieval target solution pH 9.0 (DAKO), followed by microwaving (3 × 5 min). Non-specific binding was blocked in normal rabbit serum at dilution 1:20 for 30 min. (DAKO, X0902). No rinsing was performed. The primary polycloncal antibody to LDH-1 isoenzyme (ab 9001, Abcam) was applied at dilution 1:200 overnight at 4o C. For the detection of isoenzyme LDH-5 the sheep polycloncal ab 9002 (AbcamUK) was applied at the same dilution overnight. Following washing with PBS, endogenous peroxidase was quenched with (Peroxidase Block, code: K0679, DAKO) for 10 min. It followed washing with PBS. Sections were then incubated with a secondary antibody (Biotinylated Secondary rabbit-antisheep; DAKO, P0163) at 1:100 for 30min, and washed in PBS. Streptavidin-HRP (K0679, DAKO) was applied for 30 min, and sections were again washed in PBS. The color was developed by 15min. incubation with DAB solution and sections were counterstained weakly with hematoxylin.
The ab799 goat polyclonal antibody (Abcam) was used to assess MCT-1 expression at dilution 1:50 and overnight incubation. A similar HRP immunostaining technique was applied using the LSAB+System-HRP kit (DAKO).
Controls
Known positive tissues for LDH-1, LDH-5 and MCT-1 were used as controls. Negative controls were as in the case of Caveolin-1, TP and MIB1.
Expression patterns and assessment of Caveolin-1, TP, Ki-67(MIB1), MCT-1, LDH-1 and LDH-5 in prostatic TAS
The expression of Caveolin-1, TP, MIB1, MCT-1 and the isoenzymes LDH-1 and LDH-5 was recorded in TAS. In these cells (tumor-associated fibroblasts and myofibroblasts), the expression of TP, LDH-1 and LDH-5 was mixed nuclear and cytoplasmic, whereas that of Caveolin-1 was purely cytoplasmic, and the expression of MIB1 was exclusively nuclear. MCT-1 expressed a distinct membranous stain. The percentage of TAFs expressing the proteins under investigation was recorded in all optical fields of a section at ×200 magnification, and the median value was used to define groups of high and low reactivity.
Expression patterns and assessment of MCT1, LDH-1 and LDH-5 in prostatic cancer cells
The pattern of LDH-1 and LDH-5 expression in prostatic cancer cells was mixed nuclear and cytoplasmic. The proportion of tumor cells expressing cytoplasmic and/or nuclear reactivity to isoenzymes LDH was recorded, after examining the entire tumor area at ×200 magnification and the median value was taken to define groups of high and low LDH reactivity. The same procedure was applied for the MCT-1 membranous expression.
Statistical analysis
Statistical analysis was performed using the GraphPad Prism 5.0 package and the Instat packages (GraphPad Software Inc.). All scoring were handled as a categorical and as continuous variable. The Fisher’s exact t-test or the unpaired two-tailed t-test was used to compare categorical variables as appropriate. Linear regression analysis was employed to assess correlations between continuous variables. A p-value of < 0.05 was used for significance.
Disclosure of Potential Conflicts of Interest
There are no conflicts of interest to declare for any of the authors listed in the paper.
Acknowledgments
We are grateful to Professor K.C. Gatter, University of Oxford, Oxford, U.K for providing us with the monoclonal antibody thymidine phosphorylase. We also thank Mrs Giannoula Kirmizi and Mrs Kyriaki Devetzi for excellent technical assistance.
References
- Sivridis E, Giatromanolaki A, Koukourakis MI. “Stromatogenesis” and tumor progression. Int J Surg Pathol 2004; 12:1 - 9; http://dx.doi.org/10.1177/106689690401200101; PMID: 14765266
- Giatromanolaki A, Sivridis E, Koukourakis MI. The Pathology of tumor stromatogenesis. Cancer Biol Ther 2007; 6:6; PMID: 17534144
- Cunha GR, Hayward SW, Wang YZ, Ricke WA. Role of the stromal microenvironment in carcinogenesis of the prostate. Int J Cancer 2003; 107:1 - 10; http://dx.doi.org/10.1002/ijc.11335; PMID: 12925950
- Thompson TC, Timme TL, Kadmon D, Park SH, Egawa S, Yoshida K. Genetic predisposition and mesenchymal-epithelial interactions in ras+myc-induced carcinogenesis in reconstituted mouse prostate. Mol Carcinog 1993; 7:165 - 79; http://dx.doi.org/10.1002/mc.2940070307; PMID: 8489712
- Bhowmick NA, Chytil A, Plieth D, Gorska AE, Dumont N, Shappell S, et al. TGF-beta signaling in fibroblasts modulates the oncogenic potential of adjacent epithelia. Science 2004; 303:848 - 51; http://dx.doi.org/10.1126/science.1090922; PMID: 14764882
- Olumi AF, Grossfeld GD, Hayward SW, Carroll PR, Tlsty TD, Cunha GR. Carcinoma-associated fibroblasts direct tumor progression of initiated human prostatic epithelium. Cancer Res 1999; 59:5002 - 11; PMID: 10519415
- Koukourakis MI, Giatromanolaki A, Brekken RA, Sivridis E, Gatter KC, Harris AL, et al. Enhanced expression of SPARC/osteonectin in the tumor-associated stroma of non-small cell lung cancer is correlated with markers of hypoxia/acidity and with poor prognosis of patients. Cancer Res 2003; 63:5376 - 80; PMID: 14500371
- Sivridis E, Giatromanolaki A, Anastasiadis P, Georgiou L, Gatter KC, Harris AL, et al, Tumour and Angiogenesis Research Group. Angiogenic co-operation of VEGF and stromal cell TP in endometrial carcinomas. J Pathol 2002; 196:416 - 22; http://dx.doi.org/10.1002/path.1060; PMID: 11920737
- Lagace R, Grimaud JA, Shurch W, et al. Myofibroblastic stromal reaction in carcinomas of the breast and variations of collagenous matrix and structural glycoproteins. Virchows Arch 1985; 408:49 - 59; http://dx.doi.org/10.1007/BF00739962
- Parton RG, Hanzal-Bayer M, Hancock JF. Biogenesis of caveolae: a structural model for caveolin-induced domain formation. J Cell Sci 2006; 119:787 - 96; http://dx.doi.org/10.1242/jcs.02853; PMID: 16495479
- Witkiewicz AK, Dasgupta A, Sotgia F, Mercier I, Pestell RG, Sabel M, et al. An absence of stromal caveolin-1 expression predicts early tumor recurrence and poor clinical outcome in human breast cancers. Am J Pathol 2009; 174:2023 - 34; http://dx.doi.org/10.2353/ajpath.2009.080873; PMID: 19411448
- Goetz JG, Minguet S, Navarro-Lérida I, Lazcano JJ, Samaniego R, Calvo E, et al. Biomechanical remodeling of the microenvironment by stromal caveolin-1 favors tumor invasion and metastasis. Cell 2011; 146:148 - 63; http://dx.doi.org/10.1016/j.cell.2011.05.040; PMID: 21729786
- Koukourakis MI, Giatromanolaki A, Harris AL, Sivridis E. Comparison of metabolic pathways between cancer cells and stromal cells in colorectal carcinomas: a metabolic survival role for tumor-associated stroma. Cancer Res 2006; 66:632 - 7; http://dx.doi.org/10.1158/0008-5472.CAN-05-3260; PMID: 16423989
- Koukourakis MI, Giatromanolaki A, Bougioukas G, Sivridis E. Lung cancer: a comparative study of metabolism related protein expression in cancer cells and tumor associated stroma. Cancer Biol Ther 2007; 6:1476 - 9; http://dx.doi.org/10.4161/cbt.6.9.4635; PMID: 17881895
- Griffiths L, Stratford IJ. Platelet-derived endothelial cell growth factor thymidine phosphorylase in tumour growth and response to therapy. Br J Cancer 1997; 76:689 - 93; http://dx.doi.org/10.1038/bjc.1997.447; PMID: 9310231
- Sivridis E, Giatromanolaki A, Papadopoulos I, Gatter KC, Harris AL, Koukourakis MI. Thymidine phosphorylase expression in normal, hyperplastic and neoplastic prostates: correlation with tumour associated macrophages, infiltrating lymphocytes, and angiogenesis. Br J Cancer 2002; 86:1465 - 71; http://dx.doi.org/10.1038/sj.bjc.6600281; PMID: 11986782
- Okada K, Yokoyama K, Okihara K, Ukimura O, Kojima M, Miki T, et al. Immunohistochemical localization of platelet-derived endothelial cell growth factor expression and its relation to angiogenesis in prostate. Urology 2001; 57:376 - 81; http://dx.doi.org/10.1016/S0090-4295(00)00907-9; PMID: 11182368
- Sivridis E, Giatromanolaki A, Koukourakis MI, Bicknell R, Harris AL, Gatter KC. Thymidine phosphorylase expression in endometrial carcinomas. Clin Exp Metastasis 1999; 17:445 - 50; http://dx.doi.org/10.1023/A:1006603709248; PMID: 10651312
- Koukourakis MI, Giatromanolaki A, Kakolyris S, O’Byrne KJ, Apostolikas N, Skarlatos J, et al. Different patterns of stromal and cancer cell thymidine phosphorylase reactivity in non-small-cell lung cancer: impact on tumour neoangiogenesis and survival. Br J Cancer 1998; 77:1696 - 703; http://dx.doi.org/10.1038/bjc.1998.280; PMID: 9635852
- Di Vizio D, Morello M, Sotgia F, Pestell RG, Freeman MR, Lisanti MP. An absence of stromal caveolin-1 is associated with advanced prostate cancer, metastatic disease and epithelial Akt activation. Cell Cycle 2009; 8:2420 - 4; http://dx.doi.org/10.4161/cc.8.15.9116; PMID: 19556867
- Pavlides S, Whitaker-Menezes D, Castello-Cros R, Flomenberg N, Witkiewicz AK, Frank PG, et al. The reverse Warburg effect: aerobic glycolysis in cancer associated fibroblasts and the tumor stroma. Cell Cycle 2009; 8:3984 - 4001; http://dx.doi.org/10.4161/cc.8.23.10238; PMID: 19923890
- Rattigan YI, Patel BB, Ackerstaff E, Sukenick G, Koutcher JA, Glod JW, et al. Lactate is a mediator of metabolic cooperation between stromal carcinoma associated fibroblasts and glycolytic tumor cells in the tumor microenvironment. Exp Cell Res 2012; 318:326 - 35; http://dx.doi.org/10.1016/j.yexcr.2011.11.014; PMID: 22178238
- Warburg O. The Metabolism of Tumors. London, United Kingdom: Constable & Co. Ltd 1930.
- Harris AL. Metabolic compartments in tumor tissue: implications for therapy. Cell Cycle 2012; 11:13 - 4; http://dx.doi.org/10.4161/cc.11.1.18729; PMID: 22193036