Abstract
Cancer gene therapy requires tumor-specific delivery and expression of a transgene to maximize antitumor efficacy and minimize side effects. In this study, we developed a new tumor-targeting, homologous recombination-based adenovirus vector system, HRAVS. HRAVS is composed of two adenovirus vectors, Ad.CMV.IR containing reverse sequence (IR) and a CMV promoter and Ad.IR.EGFP comprising the report gene EGFP and IR. For improved viral DNA replication and transgene expression, the E1a gene was added to HRAVS to generate the enhanced HRAVS, EHRAVS, which consists of Ad.CMV.IR and Ad.IR.EGFP/E1a. The optimal vector composition ratio of Ad.CMV.IR to Ad.IR.EGFP or Ad.IR.EGFP/E1a was identified as 30:70 based on EGFP expression efficiency in tumor cells. The transgene expression of HRAVS and EHRAVS was efficiently and specifically activated in tumor cells only and not in normal cells. Moreover, compared with HRAVS, EHRAVS infection led to higher virus yields and transgene expression and higher toxicity to tumor cells, and these results could be related to the involvement of E1a genes. The results in present study suggest the need for in vivo antitumor study using these new dual-Ad vector systems based on the homologous recombination.
Introduction
Adenoviruses based on serotype 5 (Ad5) are widely used as an expression vector in cancer gene therapy due to their potential to transduce a variety of tumors after local or systemic application.Citation1-Citation3 To improve the transduction efficiency, specificity, and safety of Ad vectors, multiple strategies for targeting Ad5 to tumor tissues have been explored. One method is to regulate the expression of E1A and/or E1B using the tumor specific promoters, such as α-fetoprotein promoter for hepatocellular carcinoma,Citation4 tyrosinase enhancer/promoter for melanoma,Citation5 and prostate-specific antigen promoter for prostate cancer.Citation6 However, this approach is usually restricted by cancer types and cancer cell heterogeneity. Moreover, the enhancers and promoters from viral genomes can compromise the activity and specificity of the tumor-specific promoters.Citation7 Other efforts that introduce mutation into E1A and/or E1B genes can improve the specificity of viral propagation in tumor cells, but often lead to decreased efficiency of viral replication.Citation8-Citation10
An alternative strategy for tumor-selective adenoviral replication is to delete the Ad E1A and/or E1B, which are dispensable in tumor cells.Citation11,Citation12 E1A and E1B are the immediate-early viral genes, and the expression of E1A and E1B can act as the main transactivator of other viral promoters, force quiescent cells into the cell cycle and prevent early cell apoptosis for virus reproduction. Ads with the deletion of E1A and E1B genes (AdE1-) cannot replicate in normal cells, but they still can specifically reproduce in a variety of human tumor cells, partially due to the tumor cell-derived proteins that can, to some extent, complement the functions of E1A and E1B.Citation11,Citation12 The inverted repeats (IRs) inserted into the E1 region of AdE1- vectors can efficiently mediate predictable genomic homologous recombination between the IRs during viral DNA replication.Citation13 To implement this principle in a replication-dependent expression system, several vectors have been constructed, such as Ad.IR.E1A and Ad5/35.IR.E1A/TRAIL.Citation14,Citation15 The viral genomes of these vectors contain a promoter, the IR and an exogenous gene in the direction of 3′–5′ in the E1 region. After homologous recombination between the IRs in progeny genomes, the promoter can be connected with the exogenous gene, creating a functional expression cassette. The genomic rearrangement is dependent on viral DNA replication specifically in tumor cells, ensuring the tumor-specificity of the Ad vectors.Citation16 It should be noted that the homologous recombination mentioned above may also occur in vector propagation in HEK-293 cells, leading to exogenously expressed progeny virus. This makes obtaining pure vectors difficult.
To circumvent this shortcoming, a new homologous recombination-based adenovirus vector system (HRAVS), consisting of Ad.IR.CMV and Ad.IR.EGFP, was constructed in this study. This system achieves transgene expression in progeny viruses only after DNA homologous recombination. Because no genome rearrangement can occur during virus proliferation in HEK-293 cells, this system is more convenient for production and application. E1a, a well-known tumor suppressor gene, is the first viral gene expressed during adenovirus infection.Citation17,Citation18 The multiple functions of E1A include transcriptional activation, induction of cellular DNA synthesis, cell transformation, antitumor activities and sensitization to different categories of antitumor drugs.Citation19-Citation21 Therefore, enhanced HRAVS (EHRAVS) that consists of Ad.IR.CMV and Ad.IR.EGFP/E1a, was developed to improve viral DNA replication, transgene expression and antitumor efficacy. The tumor cell-specific transgene expression and cytotoxicity of HRAVS and EHRAVS were investigated.
Results
Construction of HRAVS and EHRAVS
An oncolytic adenovirus deleted for E1 and E3 was used as vector backbone. In Ad.CMV.IR, the CMV promoter was positioned upstream of the IR flanked by a SV40pA stop signal (), and in Ad.IR.EGFP and Ad.IR.EGFP/E1A, the IR was placed upstream of the EGFP or EGFP-IRES-E1A cassette, respectively (). The recombination system HRAVS was composed of Ad.CMV.IR and Ad.IR.EGFP and EHRAVS was comprised of Ad.CMV.IR and Ad.IR.EGFP/E1A. Replication of the viruses of the recombination system in the tumor cells led to homologous recombination between progeny DNA, forming the Ad.CMV.IR.EGFP () or Ad.CMV.IR.EGFP/E1A expression cassettes ().
Figure 1. (A) Schematic structure of Ad.CMV.IR, Ad.IR.EGFP, and Ad.IR.EGFP/E1a. (B) HRAVS, which is composed of Ad.CMV.IR and Ad.IR.EGFP, specifically replicates in tumor cells. The homologous recombination is activated, leading to the formation of Ad.CMV.IR.EGFP and EGFP expression. (C) EHRAVS, which is composed of Ad.CMV.IR and Ad.IR.EGFP/E1a, specifically replicates in tumor cells. The homologous recombination between the two viruses results in the production of Ad.CMV.IR.EGFP/E1a, which expresses both EGFP and E1a, and the latter can enhance the virus replication in the tumor cells.
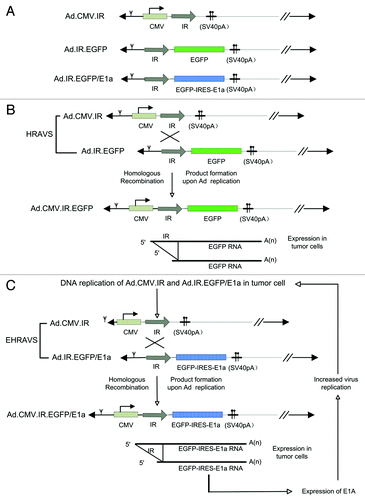
Tumor cell-selective EGFP expression and optimal virus composition ratio of HRAVS and EHRAVS
After homologous recombination, the EGFP expression was under the control of CMV promoter. However, significant gene expression was observed only in tumor cells and not in normal cells (), indicating the specific occurrence of homologous recombination in tumor cells. Thus, tumor-specific transgene expression could be achieved using this homologous recombination-based adenovirus vector system.
Figure 2. EGFP expression in the cells infected with HRAVS at MOI of 100. (A) The tumor and normal cells were infected with HRAVS at 1:1 of Ad.CMV.IR to Ad.IR.EGFP. After 72 h, the EGFP expression was examined through fluorescence assay in the HCS system. (B) The EGFP expression in A549 cells were examined after 72 h treatment with HRAVS at various ratios of Ad.CMV.IR to Ad.IR.EGFP. (C) Time course of the EGFP expression in A549 and MRC-5 cells. The cells were infected with HRAVS at 30:70 of Ad.CMV.IR to Ad.IR.EGFP.
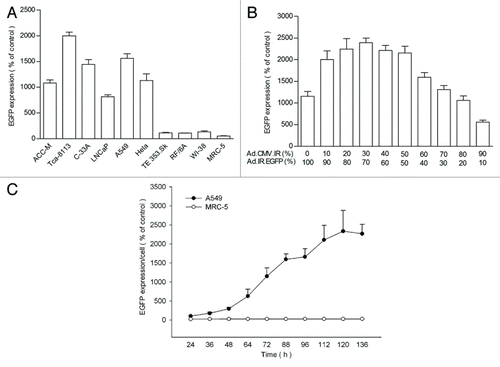
Using A549 as a representative tumor cell, the influence of virus composition ratio of HRAVS on the EGFP expression was explored. The highest EGFP expression was obtained at around 30:70 (Ad.CMV.IR to Ad.IR.EGFP) (). The tumor cells infected with EHRAVS at the same ratio of Ad.CMV.IR to Ad.IR.EGFP/E1a also exhibited the strongest EGFP expression (data not shown). Thus, virus composition ratio of 30:70 was adopted for all other studies. EGFP expression in A549 cells exhibited a time-dependent increase till 120 h after HRAVS infection; in contrast, no obvious EGFP expression was observed in normal MRC-5 cells ().
EGFP expression and replication efficiency of HRAVS and EHRAVS
The EGFP transcripts could be detected in all tested tumor cells. The expression level was tumor-cell dependant, with higher EGFP expression in ACC-M and Tca-8113 cells than in Hep3B and A549 cells, and the quantity of EGFP transcripts increased with the infection time in all tested tumor cells (). Generally, EHRAVS could lead to more EGFP transcripts and protein expression than HRAVS (). No significant EGFP expression was observed in the normal cells treated by HRAVS or EHRAVS ().
Figure 3. EGFP expression and virus yield of the cells treated by HRAVS (30:70 of Ad.CMV.IR to Ad.IR.EGFP) or EHRAVS (30:70 of Ad.CMV.IR to Ad.IR.EGFP/E1a). (A) Real-time PCR analysis of EGFP expression. At indicated time points, total RNA was isolated from the cells infected with HRAVS or EHRAVS. The transcripts of EGFP were quantified using β-actin as an internal control. *P < 0.05, **P < 0.01, and ***P < 0.001 as compared with HRAVS at 48, 74, and 96 h, respectively. (B) Tumor and normal cells were infected with HRAVS or EHRAVS at MOI of 100. After 72 h, EGFP protein expression was examined through fluorescence assay in the HCS system. **P < 0.01 as compared with HRAVS. (C) Cultured cells were infected with HRAVS or EHRAVS at MOI of 30. Cell lysates were harvested at 48 h and titered by plaque assay on HEK-293 cells for virus yield assay. ***P < 0.001 as compared with HRAVS.
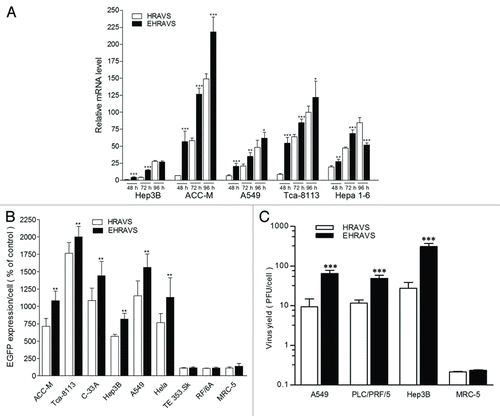
In order to examine whether E1a gene could enhance the ability of adenovirus replication, we evaluated the virus replication efficiency using the plaque assay (). Compared with HRAVS, EHRAVS infection resulted in a significantly increased virus replication by about 2-fold in A549 cells, 0.5-fold in PLC/PRF/5 cells and 10-fold in Hep3B cells, respectively. In contrast, these dual-vector systems could not efficiently replicate in normal cells such as MRC-5.
Effect of HRAVS and EHRAVS on the viability and apoptosis of tumor cells
Tumor and normal cells were treated with HRAVS or EHRAVS at MOI 100 to assess the cytolytic activity. Compared with HRAVS, EHRAVS more effectively killed the tumor cells, but both systems were nontoxic to the normal cells (). Apoptosis analysis in ACC-M and Tca-8113 cells showed that both HRAVS and EHRAVS activated significant apoptosis in tumor cells, and EHRAVS had a stronger apoptosis-inducing capability ().
Figure 4. Viability and apoptosis of the cells treated with HRAVS (30:70 of Ad.CMV.IR to Ad.IR.EGFP) or EHRAVS (30:70 of Ad.CMV.IR to Ad.IR.EGFP/E1a) (A) Effect of adenovirus infection on cell viability. After 6 h treatment with HRAVS or EHRAVS, CCK-8 solution was added for additional 2 h incubation. Results were determined at the absorbance of 450 nm on a Bio-Rad Model 550 Microplate Reader relative to cell controls as designated 100%. **P < 0.01, *P < 0.05 as compared with HRAVS. (B) Apoptosis assay after adenovirus infection. Cells were infected with HRAVS or EHRAVS at MOI of 100. After 72 h, The apoptosis assay was performed using FITC Annexin V Apoptosis Detection Kit I on a FACSCalibur flow cytometer.
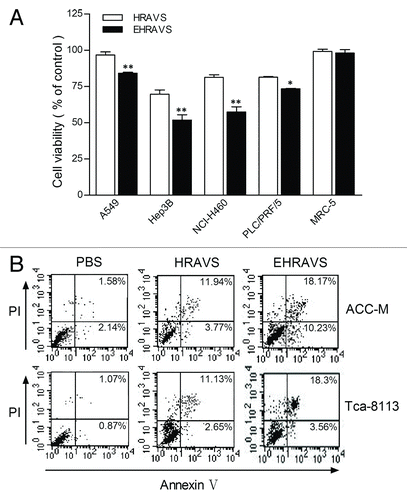
Influence of HRAVS and EHRAVS on the MMP of tumor cells
During apoptosis, engagement of the mitochondrial pathway involves the increased permeability of mitochondrial membrane, which leads to the drop of MMP and the release of apoptosis-induced proteins, such as cytochrome c and Smac/DIABLO. Mitotracker red was used to examine the MMP of Ad-infected cells. EHRAVS, but not HRAVS, induced obvious drop in the MMP of ACC-M and Tca-8113 tumor cells (). This result was consistent with the enhanced toxicity to tumor cells induced by EHRAVS compared with HRAVS ().
Figure 5. The MMP assay of the cells infected with HRAVS (30:70 of Ad.CMV.IR to Ad.IR.EGFP) or EHRAVS (30:70 of Ad.CMV.IR to Ad.IR.EGFP/E1a). The cells were pretreated with PBS, HRAVS or EHRAVS at MOI of 100. After 48 h, the MMP was determined by Mitotracker red staining and fluorescence assay. The red fluorescence can selectively accumulate in mitochondria of ACC-M (A) and Tca-8113 (C) cells and represents as a function of the cell MMP. Quantitation of fluorescence intensity in ACC-M (B) and Tca-8113 (D) cells. **P < 0.01 as compared with PBS group; ##P < 0.01, #P < 0.05 as compared with HRAVS.
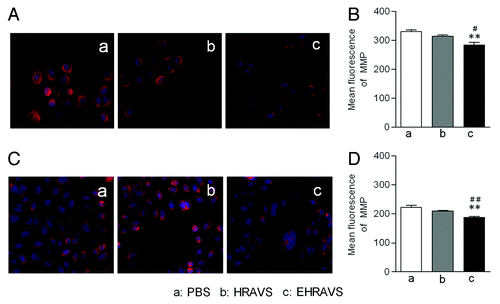
Discussion
In this study, we developed new oncolytic dual-Ad vector systems that utilize the replication-dependent homologous recombination to achieve tumor cell-specific replication and transgene expression. For the similar purpose, the single-vector recombination method has ever been investigated with respect to tumor cell killing and exogenous gene expression.Citation14 But in that system, virus amplification in HEK-293 cell may also induce ubiquitous homologous recombination, making the progeny mixed with not only the expected virus but those with functional gene expression cassette. For large-scale production, obtaining pure adenovirus vector is challenging. Neither of the two vectors in our dual-Ad vector systems has the above-mentioned trouble, and large-scale production might be feasible. Moreover, homologous recombination based on viral DNA replication can only be activated in tumor cells that support the massive virus proliferation. This was proved in the tests showing that EGFP expression can be observed only in tumor cells, and not normal cells, after infection with HRAVS or EHRAVS ( and ). Thus, our strategy achieved tumor-specific exogenous gene expression.
Here, the EGFP gene can be easily replaced by other therapeutic genes for various treatments. In addition, to improve do novo production of virions and Ad-vector spread in tumors, the E1a gene was integrated into HRAVS to generate EHRAVS, which showed accelerated viral replication and spread in tumor cells and created a positive feedback loop for specific tumor cell killing (, –).
Compared with other Ad vectors and single-vector recombination method, the systems presented here have the following features and advantages: First, they are based on the first generation Ad vectors deleted for E1 and E3, which have been widely investigated with respect to the bio-safety, molecular virology and host interaction.Citation22,Citation23 Second, the specific tumor cell killing does not rely on E1 mutations that can compromise the production yield, viral replication and spread.Citation24,Citation25 Third, in contrast to the tumor-specific promoters, the systems utilize a more universal strong CMV promoter to achieve high transgene expression. Moreover, the transgene expression occurs in the late of Ad life cycle. This is particularly important for pro-apoptosis genes, as apoptosis induced early after Ad infection might abort viral replication and impede the formation of infectious virions; apoptosis activated in late in the infection cycle could assist the release of Ad progeny.
Materials and Methods
Cell lines and culture conditions
Tumor cell lines used in this study were A549 (ATCC CCL-185), C-33A (ATCC HTB-31), Hela (ATCC CCL-2), Hep3B (ATCC HB-8064), Hepa 1-6 (ATCC CRL-1830), LNCaP (ATCC CRL-1740), NCI-H460 (ATCC HTB-177), PLC/PRF/5 (ATCC CRL-8024), ACC-M and Tca-8113 (gifts from Prof Wan-Tao Chen, Department of Oral and Maxillofacial Surgery, Ninth People's Hospital, Shanghai Jiao Tong University School of Medicine). Normal cell lines were TE 353.Sk (ATCC CRL-7761), MRC-5 (ATCC CCL-171), WI-38 (ATCC CCL-75), and RF/6A (ATCC CRL-1780). All cells were maintained in Dulbecco’s modified Eagle’s medium (DMEM) supplemented with 10% heat-inactivated fetal bovine serum (FBS), 2 mM l-glutamine, 100 U penicillin/ml, and 100 μg streptomycin/ml in a humidified atmosphere of 5% CO2 at 37 °C.
Adenovirus construction and propagation
A pShuttle-Adeasy system was used to create the adenovirus vectors. The IR fragment was obtained by PCR amplification from pGEM.IR. constructed in our lab, and the PCR primers were 5′-CTAGCAGCTG AGCTAGGACT C-3′ and 5′-GGGAAGCTTG AATTCTTTGC CAA-3′. The PCR fragment was inserted into the SalI and HindIII site of pShuttle-CMV and pShuttle, generating pShuttle.CMV.IR and pShuttle IR, respectively. A pShuttle.IR.EGFP vector was created by removing the EGFP gene from pEGFP-N1 (Clontech) with HindIII and XbalI, and inserting it into the same site of pShuttle.IR. An IRES-E1a fragment was removed from a pIRES-E1a-blunt constructed in our lab by PmlI and NotI digestion,Citation26 and the EGFP fragment was removed from pEGFP-N1 by digestion with XhoI and HpaI. The two fragments were cloned into the XhoI and NotI site of pShuttle IR, forming pShuttle.IR.EGFP/E1a. Then pShuttle plasmids were linearized with PmeI and recombined with pAdEasy-1 in BJ5183 cells. Correct recombinants were selected and re-transformed into DH5α. Purified recombinant plasmids were linearized with PacI and transfected into HEK-293 cells to generate adenoviruses. Recombinant viruses were propagated in HEK-293 cells, purified and plaque-titered as described elsewhere.Citation26 The corresponding viruses were named Ad.CMV.IR, Ad.IR.EGFP, and Ad.IR.EGFP/E1a, respectively. HRAVS is composed of Ad.CMV.IR and Ad.IR.EGFP and EHRAVS consists of Ad.CMV.IR and Ad.IR.EGFP/E1a. The E1 genes (nucleotides 342–3534) and the E3 genes (nucleotides 28 138–30 818) were deleted in both vectors. Real-time PCR analysis was performed to assess the contamination of E1+ replication-competent adenovirus as described elsewhere.Citation27 Virus preparations with fewer than one E1+ viral genome in 106 genomes were used in this study.
EGFP expression assay
Cells were seeded at 5 × 103 cell/well in 96-well plates. After 24 h, the cells were infected with HRAVS or EHRAVS. At predetermined time points, the cells were stained with Hoechst 33324 (1:1000, Sigma-Aldrich) for 30 min at 37 °C, and then the plates were scanned on an ArrayScan High Content Screening (HCS) system (Cellomics) for the quantitative assay of EGFP fluorescence.
RNA isolation and real-time PCR assay
Total RNA was extracted from cultured cells infected with HRAVS or EHRAVS at predetermined time points with a RNA isolation kit (Sangon). RNase-free DNaseI (Takara Biotechnology) was used to digest the genomic DNA in the total RNA preparation. Total RNA (1 µg) was used for cDNA synthesis according to the protocol of Takara RNA PCR kit (Takara Bio). The resulting cDNA was used as the template in the real-time PCR to measure the mRNA level of interest. The expression of EGFP was quantified with an iCycler (Bio-Rad) and SYBR green (Takara Bio) was used according to the manufacturer’s protocol. The primers used for real-time PCR are listed in . The expression value was normalized to β-actin. Relative gene expression was determined by assigning the control a relative value of 1.0, with all other values expressed relative to the control.
Table 1. Primers used in real time PCR analysis
Virus yields
Cells were seeded at 2 × 105 cells/well in 6-well plates. After 24 h, the cells were infected with HRAVS or EHRAVS at MOI 10 PFU/cell for 3 h in serum-free medium. After removing the unabsorbed virus, the cell monolayer was washed with PBS and incubated in complete medium for an additional 72 h. The cells were then scraped into the cultured medium and lysed by three freeze-thaw cycles. Three independent infections of each virus-cell combination were titered in duplicate on HEK-293 cells.
Cell viability assay
Cell viability was determined using Cell Counting Assay Kit-8 (Dojindo Laboratories). Cells (5 × 103 cells/well) were seeded in 96-well plates and incubated for 24 h. Cells were infected with viruses for 6 h and 10 μl of CCK-8 solution was then added to each well for another 2 h incubation. The absorbance values were measured at 450 nm (A450) using a Bio-Rad Model 550 Microplate Reader. Cell viability was determined using the following equation: cell viability (%) = (A450 of adenovirus test well/A450 of PBS treated control) × 100%.
Apoptosis assay
Cell apoptosis was determined by annexin V-FITC staining and analyzed by flow cytometry. Cells in 6-well plates were infected with HRAVS or EHRAVS at MOI of 100. After 48 h, both attached and floating cells were processed using an FITC Annexin V Apoptosis Detection Kit I (Becton Dickinson Medical Devices) according to the manufacturer's instructions. Cell apoptosis was quantified on a FACSCalibur flow cytometer (BD). Cells in early apoptosis were FITC-Annexin V positive and PI negative; dead cells or cells in late apoptosis were positive for FITC-Annexin V and PI.
Measurement of mitochondrial membrane potential
ACC-M or Tca8113 cells were seeded on 96-well culture plates and infected with HRAVS or EHRAVS at MOI of 100. After 30 h, live cells were stained with Mitotracker red and Hoechst solution (Cellomics) for 30 min at 37 °C. Then the cells were fixed with 3.7% formaldehyde in PBS-Tween 20 (PBST), washed with PBST and scanned on an ArrayScan HCS system. The red fluorescence selectively accumulated in mitochondria and was used to represent mitochondrial membrane potential (MMP).
Statistical analysis
Statistical analysis was performed using GraphPad Prism 5.0 software. Differences between groups were examined using the Student t test or ANOVA with Bonferroni multiple comparison tests. Differences were considered significant if P value was less than 0.05.
Conclusion
In summary, we developed new dual-Ad vector systems (HRAVS and EHRAVS) for successful tumor cell-specific, replication-activated transgene expression and tumor cell killing. The results in present study consolidate the necessity of in vivo antitumor study using these dual-Ad vector systems.
Acknowledgments
This work was supported by National Basic Research Program of China (2010CB529806), National Natural Science Foundation of China (30873179 and 81272569), Shanghai Rising-Star Program (09QA1403500), Shanghai Pujiang Program (12PJD023), Shanghai Municipal Science and Technology Commission (11ZR1419900), and Innovation Program of Shanghai Municipal Education Commission (11YZ53).
Disclosure of Potential Conflicts of Interest
No potential conflict of interest was disclosed.
References
- Alemany R. Designing adenoviral vectors for tumor-specific targeting. Methods Mol Biol 2009; 542:57 - 74; http://dx.doi.org/10.1007/978-1-59745-561-9_2; PMID: 19565895
- Oum YH, Carrico IS. Altering adenoviral tropism via click modification with ErbB specific ligands. Bioconjug Chem 2012; 23:1370 - 6; http://dx.doi.org/10.1021/bc200477z; PMID: 22681483
- Banerjee PS, Ostapchuk P, Hearing P, Carrico IS. Unnatural amino acid incorporation onto adenoviral (Ad) coat proteins facilitates chemoselective modification and retargeting of Ad type 5 vectors. J Virol 2011; 85:7546 - 54; http://dx.doi.org/10.1128/JVI.00118-11; PMID: 21613404
- Zhang KJ, Zhang J, Wu YM, Qian J, Liu XJ, Yan LC, et al. Complete eradication of hepatomas using an oncolytic adenovirus containing AFP promoter controlling E1A and an E1B deletion to drive IL-24 expression. Cancer Gene Ther 2012; 19:619 - 29; http://dx.doi.org/10.1038/cgt.2012.40; PMID: 22790965
- Fontecedro AC, Lutschg V, Eichhoff O, Dummer R, Greber UF, Hemmi S. Analysis of adenovirus trans-complementation-mediated gene expression controlled by melanoma-specific TETP promoter in vitro. Virol J 2010; 7:175; http://dx.doi.org/10.1186/1743-422X-7-175; PMID: 20670430
- Pouliot F, Karanikolas BD, Johnson M, Sato M, Priceman SJ, Stout D, et al. In vivo imaging of intraprostatic-specific gene transcription by PET. J Nucl Med 2011; 52:784 - 91; http://dx.doi.org/10.2967/jnumed.110.084582; PMID: 21498525
- Subramanian T, Vijayalingam S, Chinnadurai G. Genetic identification of adenovirus type 5 genes that influence viral spread. J Virol 2006; 80:2000 - 12; http://dx.doi.org/10.1128/JVI.80.4.2000-2012.2006; PMID: 16439556
- Stone D. Novel viral vector systems for gene therapy. Viruses 2010; 2:1002 - 7; http://dx.doi.org/10.3390/v2041002; PMID: 21994667
- Cattaneo R, Miest T, Shashkova EV, Barry MA. Reprogrammed viruses as cancer therapeutics: targeted, armed and shielded. Nat Rev Microbiol 2008; 6:529 - 40; http://dx.doi.org/10.1038/nrmicro1927; PMID: 18552863
- Zheng X, Rao XM, Gomez-Gutierrez JG, Hao H, McMasters KM, Zhou HS. Adenovirus E1B55K region is required to enhance cyclin E expression for efficient viral DNA replication. J Virol 2008; 82:3415 - 27; http://dx.doi.org/10.1128/JVI.01708-07; PMID: 18234796
- Kühnel F, Gürlevik E, Wirth TC, Strüver N, Malek NP, Müller-Schilling M, et al. Targeting of p53-transcriptional dysfunction by conditionally replicating adenovirus is not limited by p53-homologues. Mol Ther 2010; 18:936 - 46; http://dx.doi.org/10.1038/mt.2009.298; PMID: 20040911
- Yun CO, Kim E, Koo T, Kim H, Lee YS, Kim JH. ADP-overexpressing adenovirus elicits enhanced cytopathic effect by induction of apoptosis. Cancer Gene Ther 2005; 12:61 - 71; http://dx.doi.org/10.1038/sj.cgt.7700769; PMID: 15375379
- Liu Y, Tuve S, Persson J, Beyer I, Yumul R, Li ZY, et al. Adenovirus-mediated intratumoral expression of immunostimulatory proteins in combination with systemic Treg inactivation induces tumor-destructive immune responses in mouse models. Cancer Gene Ther 2011; 18:407 - 18; http://dx.doi.org/10.1038/cgt.2011.8; PMID: 21394107
- Bernt K, Liang M, Ye X, Ni S, Li ZY, Ye SL, et al. A new type of adenovirus vector that utilizes homologous recombination to achieve tumor-specific replication. J Virol 2002; 76:10994 - 1002; http://dx.doi.org/10.1128/JVI.76.21.10994-11002.2002; PMID: 12368342
- Bernt KM, Ni S, Tieu AT, Lieber A. Assessment of a combined, adenovirus-mediated oncolytic and immunostimulatory tumor therapy. Cancer Res 2005; 65:4343 - 52; http://dx.doi.org/10.1158/0008-5472.CAN-04-3527; PMID: 15899826
- Glockzin G, Mantwill K, Jurchott K, Bernshausen A, Ladhoff A, Royer HD, et al. Characterization of the recombinant adenovirus vector AdYB-1: implications for oncolytic vector development. J Virol 2006; 80:3904 - 11; http://dx.doi.org/10.1128/JVI.80.8.3904-3911.2006; PMID: 16571807
- Loewenstein PM, Wu SY, Chiang CM, Green M. The adenovirus E1A N-terminal repression domain represses transcription from a chromatin template in vitro. Virology 2012; 428:70 - 5; http://dx.doi.org/10.1016/j.virol.2012.03.021; PMID: 22521914
- Su JL, Cheng X, Yamaguchi H, Chang YW, Hou CF, Lee DF, et al. FOXO3a-dependent mechanism of E1A-induced chemosensitization. Cancer Res 2011; 71:6878 - 87; http://dx.doi.org/10.1158/0008-5472.CAN-11-0295; PMID: 21911455
- Loewenstein PM, Green M. Expression of the adenovirus early gene 1A transcription-repression domain alone downregulates HER2 and results in the death of human breast cancer cells upregulated for the HER2 proto-oncogene. Genes Cancer 2011; 2:737 - 44; http://dx.doi.org/10.1177/1947601911426570; PMID: 22207899
- Chakraborty AA, Tansey WP. Adenoviral E1A function through Myc. Cancer Res 2009; 69:6 - 9; http://dx.doi.org/10.1158/0008-5472.CAN-08-3026; PMID: 19117980
- Gomez-Gutierrez JG, Rao XM, Zhou HS, McMasters KM. Enhanced cancer cell killing by truncated E2F-1 used in combination with oncolytic adenovirus. Virology 2012; 433:538 - 47; http://dx.doi.org/10.1016/j.virol.2012.09.003; PMID: 23021422
- Halldén G, Portella G. Oncolytic virotherapy with modified adenoviruses and novel therapeutic targets. Expert Opin Ther Targets 2012; 16:945 - 58; http://dx.doi.org/10.1517/14728222.2012.712962; PMID: 22880939
- Fukazawa T, Matsuoka J, Yamatsuji T, Maeda Y, Durbin ML, Naomoto Y. Adenovirus-mediated cancer gene therapy and virotherapy (Review). Int J Mol Med 2010; 25:3 - 10; PMID: 19956895
- Hedjran F, Shantanu K, Tony R. Deletion analysis of Ad5 E1a transcriptional control region: impact on tumor-selective expression of E1a and E1b. Cancer Gene Ther 2011; 18:717 - 23; http://dx.doi.org/10.1038/cgt.2011.41; PMID: 21818136
- Ohtani S, Kagawa S, Tango Y, Umeoka T, Tokunaga N, Tsunemitsu Y, et al. Quantitative analysis of p53-targeted gene expression and visualization of p53 transcriptional activity following intratumoral administration of adenoviral p53 in vivo. Mol Cancer Ther 2004; 3:93 - 100; PMID: 14749479
- Ye X, Lu Q, Zhao Y, Ren Z, Ren XW, Qiu QH, et al. Conditionally replicative adenovirus vector carrying TRAIL gene for enhanced oncolysis of human hepatocellular carcinoma. Int J Mol Med 2005; 16:1179 - 84; PMID: 16273304
- Lu Q, Yu DH, Fang C, Liu F, Ye X, Zhao Y, et al. Influence of E3 region on conditionally replicative adenovirus mediated cytotoxicity in hepatocellular carcinoma cells. Cancer Biol Ther 2009; 8:1125 - 32; http://dx.doi.org/10.4161/cbt.8.12.8445; PMID: 19448394