Abstract
Aberrant overexpression and/or activation of epidermal growth factor receptor (EGFR) is associated with many types of cancers. EGFR variant III (EGFRvIII) is a common in-frame deletion mutant, which lacks a large part of the extracellular portion (exons 2–7), including components of the ligand-binding domain. Although EGFR has been extensively studied as a molecular imaging target, information about EGFRvIII-targeted molecular imaging is lacking. In this study, the EGFR-specific affibody, therapeutic antibody panitumumab, and ligand EGF were labeled with IRDye 800CW (Ex/Em: 774/789 nm), yielding Aff800, Pan800, and EGF800, respectively. The binding affinities of the labeled agents were compared in cell-based assays using a rat glioma cell line F98 parental (F98-p) lacking EGFR expression, and 2 F98-derived transgenic cell lines expressing EGFR or EGFRvIII (designated as F98-EGFR and F98-vIII, respectively). Results showed that all agents could bind to F98-EGFR, with Pan800 having the highest binding affinity, followed by Aff800 and EGF800. Pan800 and Aff800, but not EGF800, also bound to F98-vIII. In vivo animal imaging demonstrated that compared with F98-p tumors, F98-EGFR tumors generated higher signals with all three agents. However, in the case of F98-vIII, only Pan800 and Aff800 signals were higher. Analysis of tissue lysates showed that a large portion of Pan800 was degraded into small fragments in F98-EGFR and F98-vIII tumors, possibly due to proteolytic digestion after its specific binding and internalization. In conclusion, Pan800 and Aff800 could be used as imaging agents for both wild-type EGFR and EGFRvIII, whereas EGF800 only targets wild-type EGFR.
Introduction
The epidermal growth factor (EGF) receptor (EGFR, HER1, ErbB1) belongs to the ErbB family of type I tyrosine kinases (TKs). It is a transmembrane cell surface glycoprotein composed of a 1186 amino acid-polypeptide that contains an extracellular ligand-binding domain, a hydrophobic transmembrane domain, an intracellular tyrosine kinase domain and a non-catalytic carboxyl terminal tail.Citation1,Citation2 Various ligands for EGFR have been identified, with EGF (a small polypeptide, MW 6045 Da) as the most extensively characterized for its binding and activation of EGFR. Due to the high binding affinity to its natural receptor EGFR, EGF has been labeled with various radionuclidesCitation3,Citation4 and fluorescent dyesCitation5,Citation6 for in vivo animal imaging of EGFR expression. Overexpression and mutation of EGFR have both been identified in a variety of cancers, especially those associated with poor survival and recurrences in patients.Citation7 EGFR variant III (EGFRvIII) is a common in-frame deletion mutant that lacks a large part of the extracellular portion (exons 2–7, residues 6–273), including components of the ligand-binding domain.Citation2,Citation8 As a result, EGFRvIII is unable to bind EGF and other ligands. However, it is constitutively tyrosine-phosphorylated, although at a lower level compared with ligand-activated EGFR, and is able to activate downstream signaling pathways.Citation8 EGFRvIII is particularly associated with glioblastoma and several other cancer types, such as non-small-cell lung cancer, prostate, ovarian and breast cancers.Citation8,Citation9
EGFR-targeted anti-cancer medicines have been developed either by targeting the extracellular ligand-binding domain with neutralizing antibodies, or by inhibiting the tyrosine kinase activity using small molecules.Citation2,Citation10 Cetuximab (a humanized mouse IgG1 antibody) and panitumumab (a fully human IgG2 antibody) have been approved as selected EGFR-targeted cancer treatments. Both cetuximab and panitumumab have been studied for molecular imaging of EGFR expression in xenograft tumors.Citation11-Citation13 It was demonstrated in a malignant mesothelioma xenograft model that 86Y-labeled panitumumab may be a better imaging agent compared with 86Y-labeled cetuximab due to the lower liver uptake of panitumumab.Citation14
Affibody molecules are affinity proteins composed of 58 amino acid residues (MW 6–7 kDa). They are derived from one of the IgG-binding domains of staphylococcal protein A. A dimeric form of EGFR-specific affibody (13.7 kDa) was labeled with the near-infrared (NIR) fluorescent dye IRDye® 800CW and used for imaging A431 xenograft tumors.Citation15 A study using an 111In-labeled EGFR-specific affibody showed that although the dimeric form exhibited superior cellular retention of radioactivity in cell-based assays, the monomeric form performed better in tumor imaging of live animals.Citation16
EGFR has been extensively studied as a molecular imaging target.Citation17 A variety of EGFR-binding molecules (including EGF, antibodies, antibody fragments, affibodies, nanobodies and tyrosine kinase inhibitor derivatives) have been employed as tumor imaging agents.Citation18 In contrast, examples of EGFRvIII-targeted molecular imaging are lacking. In this study, the EGFR-specific affibody (monomeric), panitumumab, and EGF were labeled with IRDye 800CW. The binding affinity and specificity of the labeled agents were compared in cell-based assays using a rat glioma cell line F98 parental (F98-p) devoid of EGFR expression, and 2 F98-derived transgenic cell lines expressing EGFR or EGFRvIII (designated as F98-EGFR and F98-vIII, respectively).Citation19 An in vivo animal imaging study with these agents was also performed in mice bearing F98-p, F98-EGFR, and F98-vIII xenograft tumors.
Results
Cellular binding of IRDye 800CW-labeled agents
The successful incorporation of dye into the imaging agents was monitored by gel electrophoresis (data not shown). The IRDye 800CW-labeled affibody, panitumumab, and EGF were designated as Aff800, Pan800, and EGF800, respectively.
The F98 rat glioma cell line was derived from CD-Fischer 344 rats. Human EGFR or EGFRvIII was introduced into F98 cells to generate F98-EGFR or F98-vIII transgenic cell lines. The transgene expression levels for both F98-EGFR and F98-vIII were about 1.2 × 105 receptors per cell.Citation19 The binding of Aff800 (50 nM), Pan800 (10 nM), and EGF800 (50 nM) to F98-EGFR was demonstrated in . The fluorescence signals were mainly localized to the cell membrane. However, fluorescent foci (probably representative of internalized agents) were also observed inside the cells. In the case of F98-vIII cells, Aff800, and Pan800 showed similar signal patterns as with F98-EGFR cells (). However, the EGF800 signal from F98-vIII cells was much weaker compared with F98-EGFR cells (see plate assays below for quantitative data), consistent with previous findings that EGFRvIII could not bind the ligand EGF.Citation8 As a comparison, F98-p cells were stained with the same agents. None of these agents produced detectable signal except EGF800, which produced weak signal comparable to F98-vIII (Fig. S1).
Figure 1. Microscopic examination of Aff800 (50 nM), Pan800 (10 nM), and EGF800 (50 nM) binding and uptake by F98-EGFR (A) and F98-vIII (B) cells. DAPI was used to stain the nuclei. Scale bar: 10 μm.
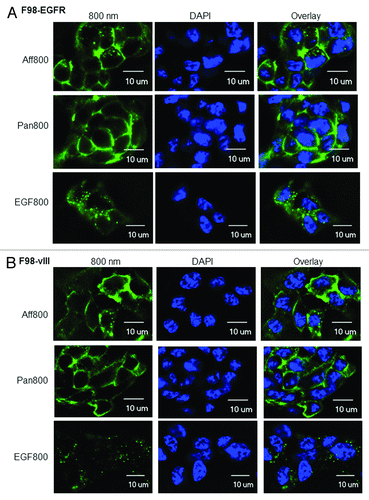
Increasing concentrations (ranging from 0.098 to 100 nM) of imaging agents were incubated with F98-EGFR, F98-vIII, or F98-p cells. As expected, all three agents (Aff800, Pan800, and EGF800) bound to F98-EGFR in a dose-dependent manner. In contrast, there was almost no fluorescence signal after incubating the free dye IRDye 800CW (IR800) with F98-EGFR (). Pan800 showed the highest binding affinity (Kd = 0.28 nM), followed by Aff800 (Kd = 1.25 nM) and EGF800 (Kd = 3.15 nM). Pan800 and Aff800 also bound to F98-vIII cells in a dose-dependent manner (), with Kd values of 0.21 nM and 1.32 nM, respectively. Because EGFRvIII lacks the ligand-binding domain, F98-vIII cells exhibited little signal following treatment with EGF800 at low concentrations (<10 nM) (). The fluorescence signals at higher EGF800 concentrations (>10 nM) were likely due to non-specific binding because F98-p cells (devoid of EGFR) also produced similar signals following equivalent EGF800 treatments (). Little signal was detected when Aff800 and Pan800 were incubated with F98-p (), indicating that their interactions with F98-EGFR and F98-vIII are highly specific.
Figure 2. Concentration-dependent binding and uptake of different probes. Different concentrations of Aff800, Pan800, EGF800 and free IRDye 800CW (IR800) were incubated with F98-EGFR (A), F98-vIII (B), and F98-p (C). The fluorescence signals were determined after removal of unbound probes (n = 3 for each data point).
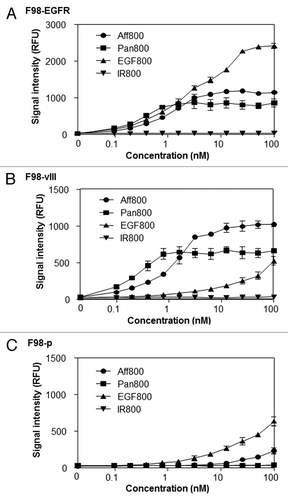
Competition of binding with EGFR-specific agents
Competition analysis was performed by incorporating different concentrations (ranging from 0.49 to 500 nM) of unlabeled agents in the binding studies of F98-EGFR and F98-vIII. The concentrations of labeled agents were 1.1 nM, 0.3 nM, and 1.1 nM for Aff800, Pan800, and EGF800, respectively. In addition to affibody, panitumumab and EGF, an EGFR-specific nanobody 7D12Citation20 was included as a competitor. All competitors were able to block the binding of labeled agents to F98-EGFR. With the increase of competitor concentrations, the binding signal of Aff800, Pan800, and EGF800 decreased (). It was also demonstrated that panitumumab (Pan) exhibited the most prominent competition, followed by other agents in the order of EGF, affibody (Aff), and nanobody 7D12.
Figure 3. Competition of Aff800, Pan800, and EGF800 binding by unlabeled molecules. Aff800 (A and B), Pan800 (C and D), and EGF800 (E and F) were incubated with F98-EGFR and F98-vIII in the presence of different concentrations (ranging from 0.49 to 500 nM) of unlabeled nanobody 7D12, affibody, EGF, or panitumumab. The fluorescence signals were determined after removal of unbound probes (n = 3 for each data point).
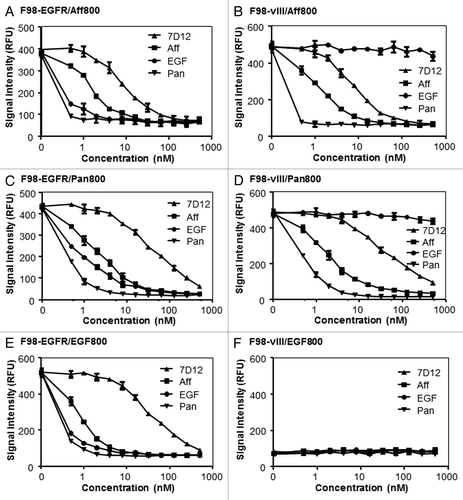
Panitumumab, affibody, and nanobody 7D12 also competed with the binding of Aff800 and Pan800 to F98-vIII in a dose-dependent manner. In contrast, EGF did not block Aff800 and Pan800 binding with F98-vIII as it did with F98-EGFR (). As EGF800 did not bind to F98-vIII cells specifically, no competition effect was observed ().
Targeting EGFR- or EGFRvIII-expressing xenograft tumors with Aff800, Pan800, and EGF800
Aff800, Pan800, and EGF800 were evaluated in vivo for their abilities to target EGFR- and EGFRvIII-expressing tumors. F98-p, F98-EGFR, and F98-vIII xenograft tumors were established on the same mouse at the left hip, left shoulder and right shoulder, respectively. In vivo animal imaging (dorsal view) showed that both Aff800 and Pan800 accumulated preferentially in F98-EGFR and F98-vIII tumors vs. F98-p tumors (). Unlike Aff800, Pan800 produced lower fluorescence signal in the kidney region, an observation that was confirmed by ex vivo imaging of dissected organs (discussed below). EGF800 fluorescence signal was high in the F98-EGFR tumor, but not in F98-vIII and F98-p tumors, thereby consistent with the results of cell-based assays. Similar to Aff800, intense EGF800 signals were observed in the kidney region. It should be noted that the fluorescence signal intensities were adjusted to different scales for different probes due to the dramatic differences in their clearance profiles. At 24 h after administration of equivalent amounts of probes, the whole-body fluorescence signal of Pan800 was about 4.7-fold of that of Aff800, whereas EGF800 signal was about 45% of that of Aff800 (Fig. S2).
Figure 4. Optical imaging of mice bearing F98-p, F98-EGFR, and F98-vIII xenograft tumors. (A) Representative whole mouse images (dorsal view) acquired at 24 h after administration of Aff800 (left), Pan800 (center), and EGF800 (right). The white arrows indicate the tumors: 1, F98-p; 2, F98-EGFR; 3, F98-vIII. The red arrow heads indicate kidneys. Kidneys of Pan800-injected mouse were not marked as they were not visible. Note that the scales for fluorescence signal intensities are adjusted individually for each probe. (B) Ex vivo imaging of tissue distribution of mice injected with Aff800 (upper left), Pan800 (upper right), and EGF800 (lower left). The layout of different tissues is illustrated in the lower right corner.
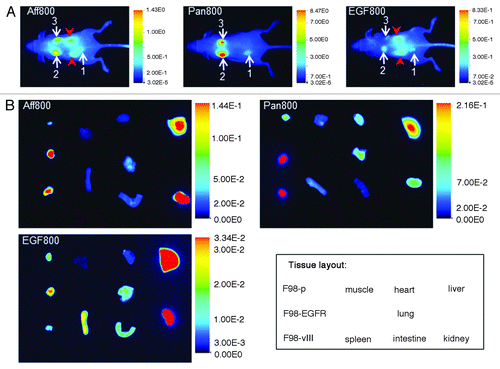
To compare the fluorescence signals in different organs, the organs were dissected and imaged ex vivo (). Consistent with the whole-animal imaging, Aff800 and Pan800 signals were higher in F98-EGFR and F98-vIII tumors than in F98-p tumors. Analysis of tissue sections revealed that the ratios of F98-EGFR tumor signal to F98-p tumor signal for Aff800 and Pan800 were 4.1 ± 0.8 and 3.8 ± 0.6, respectively. For F98-vIII tumors, the ratios (relative to F98-p) were 3.4 ± 0.9 and 4.5 ± 1.1 respectively. In the case of EGF800, F98-EGFR tumor produced higher fluorescence signal than F98-p tumor (F98-EGFR to F98-p ratio was 2.1 ± 0.5), whereas F98-vIII tumor signal was similar to that of F98-p. The fluorescence signals in the livers and kidneys of animals injected with Aff800 or EGF800 were much higher compared with other organs. However, the kidney-associated signals were low in Pan800-injected mice.
Tumor tissue lysate analysis
Tumor tissue lysates were analyzed by gel electrophoresis under non-reducing conditions. Equivalent amounts of lysate proteins were loaded on the gel. As shown in , the major fluorescent bands in tumor lysates had the same size as the control probe (lane 1), suggesting that most Aff800 in tumor tissues was intact. However, higher levels of Aff800 accumulation were noted in F98-EGFR (lane 3) and F98-vIII (lane 4) tumors than in the F98-p tumor (lane 2). Weaker-intensity bands at lower molecular weights were also detected. These bands were found in all tumor tissues, including F98-p, implying that they resulted from non-specific degradation. Interesting results were obtained for Pan800. Although intact probes (MW ~150 kDa) were detected in all tumor tissue lysates, a smaller product (MW ~10 kDa) was detected only in F98-EGFR and F98-vIII tumors, but not in the F98-p tumor. This result most likely indicates that the 10-kDa product resulted from agent internalization and degradation following the specific binding of Pan800 to either EGFR or EGFRvIII. In the case of EGF800, most of the administered probes were degraded to a smaller sized product. F98-EGFR tumor tissue lysate contained higher overall fluorescence signal compared with those from F98-p and F98-vIII.
Figure 5. Analysis of tumor tissue lysates. Tumor tissue samples from mice injected with Aff800 (left), Pan800 (center), and EGF800 (right) were homogenized in RIPA buffer and analyzed on a non-reducing BIS-TRIS gel. F98-p, F98-EGFR, and F98-vIII tumor lysates (lanes 2, 3, and 4) were run on the gel together with the respective pure imaging agent (lane 1) and free dye IRDye 800CW (lane 5). The protein marker was shown on the left side (molecular weights in kDa from top to bottom: 250, 150, 100, 75, 50, 37, 25, 20, 15, 10).
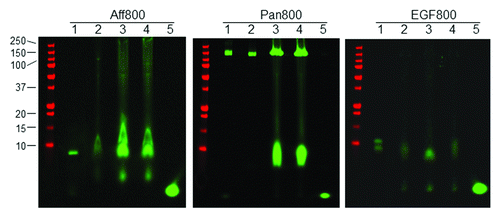
Discussion
Due to its low cost, ease of use, longer time window for image capture, and ability to track multiple probes simultaneously, fluorescence imaging is becoming increasingly popular in biomedical research. Fluorescence imaging at NIR wavelengths (700–900 nm) is particularly suitable for in vivo animal imaging studies because of its reduced light absorption and scattering in tissues, as well as the low tissue autofluorescence in the NIR region.Citation21,Citation22 A variety of targeting molecules labeled with NIR fluorophores have been successfully used to detect different molecular and cellular events for in vivo animal imaging. Examples include the small molecule 2-deoxyglucose (2-DG),Citation23,Citation24 small peptide RGD,Citation25 large peptides annexin V,Citation26 EGF,Citation5,Citation6,Citation27,Citation28 affibody,Citation15,Citation29 and large antibodies.Citation30-Citation32 Quenched probes that only produce fluorescence signals in the presence of tumor-specific proteinases have also been developed.Citation33
Cell surface receptors, such as EGFR, that are overexpressed in cancers can be reliable targets for molecular imaging. In this study, three EGFR-specific probes were compared. After labeling with IRDye 800CW reactive dye, these probes maintained relatively high binding affinities, with Kd values of Aff800 and EGF800 in the low nanomolar range, and the Kd of Pan800 in the sub-nanomolar range. The specificities of these probes were demonstrated by the low fluorescence signals associated with the F98-p cell line (lacking EGFR) in both cell-based assays and animal imaging studies.
Aff800,Citation15 EGF800,Citation6 and Pan800Citation34 have been used to target wild-type EGFR in animal imaging studies. However, none of them were characterized for EGFRvIII targeting. In this study we investigated the abilities of Aff800, Pan800, and EGF800 to target both wild-type EGFR and EGFRvIII mutant. It is noteworthy that the affibody monomer, instead of dimer, was used because a previous study demonstrated that the 111In-labeled monomer produced superior results for animal imaging.Citation16 F98-EGFR and 98-vIII cells expressing EGFR and EGFRvIII respectively,Citation19 as well as the F98-p parental cells were employed. These cell lines comprise an excellent system for comparing EGFR- and EGFRvIII-specific binding because all cell lines are derived from the same parental cell line, rendering observed differences attributable only to EGFR or EGFRvIII transgenes. Results showed that Aff800 and Pan800 bound to both F98-EGFR and F98-vIII, while EGF800 only bound to F98-EGFR. The Kd values of Aff800 and Pan800 for F98-vIII were similar to those for F98-EGFR. Unlabeled EGF competed with the binding of Aff800 and Pan800 to F98-EGFR, but not to F98-vIII. These results indicate that although the deletion of exons 2–7 from the extracellular portion disrupts the binding of EGFR to its natural ligand EGF,Citation8 the binding epitopes for affibody and panitumumab remain intact. It is noteworthy that another therapeutic antibody, cetuximab, also binds to EGFR and EGFRvIII with similar affinities (0.38 nM for EGFR-expressing MDA-MB-231 cells and 0.4 nM for EGFRvIII-expressing 32D-EGFRvIII cells).Citation35 Compared with the abundant information about EGFR-targeted in vivo imaging, EGFRvIII remains an under-explored target. A rare example is the imaging of EGFRvIII-expressing tumor (U87MG.de2–7) with the chimeric antibody ch806, which binds specifically to overexpressed, mutant, or ligand-activated EGFR.Citation36 Recently, another monoclonal antibody 4G1 specifically recognizing EGFRvIII was developed and used for tumor imaging.Citation37 It would be interesting to label these antibodies with IRDye 800CW for comparison with Pan800 for EGFR-vIII imaging in future studies.
The expression levels of receptors on F98 transgenic cells (1.2 × 105 per cell) is lowCitation19 compared with the commonly used A431 cells (2 × 106 per cell).Citation38 The sizes of xenograft tumors in this study were smaller (30–50 mg) compared with other studies (100–300 mg).Citation39,Citation40 Our results demonstrate the robustness of these imaging agents for identification of small tumors with relatively low receptor levels. The capability of a probe to bind to both EGFR and EGFRvIII can be valuable in certain applications, such as imaging of heterogeneous tumors containing both versions of receptors. Aff800 and Pan800 may be effective for that purpose.
The clearance rates of Aff800 and EGF800 were faster than that of Pan800. In situations where imaging must be performed within a short time after probe administration, Aff800 and EGF800 are more desirable. The tissue distribution patterns of these probes were different. Because the sizes of affibody and EGF are well below the renal clearance threshold of proteins (60 kDa), they were likely cleared through the kidneys. As a result, fluorescence signals in kidneys were high. High signals were also observed in the liver for both Aff800 and EGF800, possibly due to the specific binding of Aff800 and EGF800 to murine EGFR expressed in the liver.Citation15 Pan800 was mainly cleared through the liver due to its large size, resulting in a higher signal in the liver compared with the kidney. Its relative low renal accumulation makes Pan800 a better option for imaging of tumors in proximity to the kidneys.
One interesting observation is the degradation of Pan800 in F98-EGFR and F98-vIII tumors, but not in the F98-p tumor. This strongly supports the conclusion that Pan800 binds to F98-EGFR and F98-vIII specifically in vivo. Although antibody fragmentation after binding and internalization has been suggested in earlier studies,Citation41 this study reports the first time that the proteolytic digestion of a fluorophore-labeled antibody after specific binding and internalization can be visualized by gel electrophoresis. This method, with further optimization, could be applied to antibody binding and internalization analysis.
Molecules labeled with the IRDye 800CW NIR fluorescent dye have been widely used for animal imaging studies.Citation18 A toxicity study on IRDye 800CW revealed no observed adverse effects at a dose approximately 10 000 times higher than the projected dose for in vivo imaging. This was the first toxicity study of an NIR dye capable of bioconjugation.Citation42 IRDye 800CW labeled bevacizumab (an anti-vascular endothelial growth factor antibody) has proved to be a highly specific and sensitive probe for detection of tumor lesions in mouse models, and a preclinical toxicity study on this probe is underway to enable clinical trials.Citation43 Aff800 and Pan800 may also have potential for clinical translation, especially for optical image-guided surgery in cancer patients.
Materials and Methods
Chemicals and reagents
The EGFR-specific affibody (monomer)Citation40 was provided by Affibody AB. Panitumumab is a product from Amgen. The EGFR-specific nanobody 7D12 was a kind gift from Dr Paul van Bergen en Henegouwen (Utrecht University). IRDye 800CW fluorescent dyes (Ex/Em: 774/789 nm) and IRDye 800CW-labeled EGF (EGF800) were from LI-COR Biosciences. TO-PRO-3 and DAPI nucleic acid stain reagents were purchased from Invitrogen. Recombinant human EGF was purchased from R&D Systems. TCEP·HCl and RIPA buffer were obtained from Fisher Scientific.
Conjugation of EGFR-specific affibody and panitumumab with IRDye 800CW
Panitumumab was labeled with IRDye 800CW NHS ester using a protein labeling kit (LI-COR Biosciences). In brief, 3 M equivalents of IRDye 800CW NHS ester per protein were used for the labeling reaction. The reaction was conducted at room temperature for 2 h. The unreacted dye was removed using a Zeba Spin Desalting Column (Fisher Scientific).
The affibody molecules used in this study contain a unique C-terminal cysteine residue for labeling with maleimides. TCEP·HCl (5 mM) was used to monomerize the affibodies by reducing the disulfide bond that spontaneously formed between the cysteine residues. The excess TCEP·HCl was removed by passing the reaction mixture through a Zeba Spin Desalting Column before adding IRDye 800CW maleimide. The affibody/IRDye 800CW maleimide reaction mixture was incubated at room temperature for 3 h before high performance liquid chromatography (HPLC) purification. HPLC purification was performed on an Agilent 1100 Series HPLC instrument fitted with a Zorbax 300SB-C18 analytical column (4.6 × 250 mm, 5 µm). The HPLC mobile phase consisted of mixtures of Buffer A (50 mM triethylammonium acetate + 4% acetonitrile, pH 6.0) and Buffer B (50 mM triethylammonium acetate + 80% acetonitrile). HPLC system equilibration was performed at 40 °C using the specified parameters: 20:80 Buffer B/A, flow rate 1.0 mL/min. After loading the labeling reaction mixture onto the system, elution was performed with the following gradient: 20:80 to 100:0 Buffer B/A over 10 min, hold at 100:0 Buffer B/A for 5 min, flow rate 1.0 mL/min. Dye-labeled molecules were detected at λ = 780 nm; the desired product eluted at 10.3 min. Fractions containing the desired product in ≥95% purity (λ = 780 nm) were pooled and concentrated in vacuo. The HPLC-purified product was dissolved in double-distilled water and dialyzed with PBS using a Slide-A-Lyzer cassette (0.5 mL, 3.5 K MWCO) (Fisher Scientific) per the manufacturer’s instructions. After dialyzing for 2 h at 25 °C, the IRDye 800CW-labeled agent was lyophilized to afford a green solid.
The concentrations of IRDye 800CW-labeled panitumumab and affibody molecules, and the labeling efficiency were calculated using absorbances at 280 nm and 778 nm. The dye-to-protein ratios of IRDye 800CW-labeled panitumumab and affibody were 1.5 and 0.9, respectively.
Cell culture
The rat glioma cell line F98 (F98-p) and F98-derived transgenic cell lines F98-EGFR and F98-vIII were obtained from the American Type Culture Collection (ATCC). Cells were maintained in Dulbecco’s modified Eagle’s medium (DMEM) supplemented with 10% fetal bovine serum (FBS) and 1% penicillin-streptomycin (complete DMEM).
Cell binding and uptake assay
F98-p, F98-EGFR, and F98-vIII cells were seeded at approximately 3 × 104 cells per well in 96-well plates and cultured overnight. The cell density was about 80–90% confluent at the time of assay. The imaging agents were diluted in complete DMEM to designated concentrations and incubated with cells in the 37 °C incubator for 2 h. For competition studies, different concentrations of unlabeled agents were incubated with the cells together with labeled imaging agents. After incubation with imaging agents, cells were fixed with 3.7% formaldehyde/PBS and washed with PBS containing 0.1% Tween 20 (PBST). The cells were then incubated with TO-PRO-3 (nuclear stain) for normalization of cell numbers. After three additional washes with PBST, the plate was scanned on an Odyssey Infrared Imaging System (LI-COR Biosciences) for fluorescence signal intensities, which reflect the cellular binding and uptake levels.Citation15 The data were analyzed with GraphPad Prism 5 (GraphPad Software, Inc.). Kd values of different agents were determined based on the cell binding signals and agent concentrations.
Microscopic analysis
Cells were seeded in 6-well plates with coverslips and cultured overnight. The cells were incubated with imaging agents at 37 °C, 5% CO2 for 2 h, rinsed with PBS, fixed with 3.7% formaldehyde/PBS, permeabilized and washed with PBST. DAPI staining agent was used to visualize the nuclei by microscopy. After the final wash, the coverslips were mounted on glass slides with Fluoromount reagent (Sigma). The images were acquired using an Olympus IX81 Inverted microscope system equipped with a halogen bulb (Olympus). NIR filters (EX: 710/75 nm, EM: 810/90 nm; Chroma Technology Corp.) were used for IRDye 800CW detection. The images were deconvolved using the accompanying software.Citation44
In vivo imaging of xenograft tumors
All animals were cared for and maintained under the supervision and guidelines of the University of Nebraska–Lincoln Institutional Animal Care and Use Committee. Mice were maintained on a purified maintenance diet (AIN-93M) from Harlan Teklad. The xenograft tumors were established as previously described with modifications.Citation45 In brief, the SCID hairless mouse, Crl:SHO-PrkdcscidHrhr, obtained from Charles River Laboratories, Inc. at 5 weeks of age, were subcutaneously injected with 106 suspension cells in 0.1 ml serum-free medium. F98, F98-;EGFR, and F98-vIII cells were inoculated on the same mouse at the rear left, front left and front right positions, respectively.
Imaging studies began when tumors reached approximately 5 mm in size. Mice were anesthetized with 2% isoflurane during the probe administration and imaging procedures. The imaging agent (0.5 nmol) was diluted in 100 μl PBS, filter sterilized, and injected intravenously (tail vein). After 24 h, the images were acquired with a Pearl Impulse Imager (LI-COR Biosciences). The Ex/Em settings for the 700 nm channel and 800 nm channel were 685/720 nm and 785/820 nm, respectively. The images were analyzed using Pearl Cam software.
Organ and tissue analysis
After whole animal imaging, mice were sacrificed and tissues collected. The excised organs were rinsed in PBS, and imaged using a Pearl Impulse Imager. To analyze the imaging agents in tumor tissues, tissue samples were homogenized in RIPA buffer using an Omni GLH homogenizer (Omni International Inc.). After centrifugation, the supernatants of the tissue samples were run on a BIS-TRIS gel at non-reducing conditions and analyzed by in-gel fluorescence scanning on an Odyssey Infrared Imaging System.Citation44
Additional material
Download Zip (138.8 KB)Acknowledgments
We thank Affibody AB for providing affibody molecules and Dr Paul van Bergen en Henegouwen (Utrecht University) for providing nanobody 7D12. We also thank Drs Amy Schutz-Geschwender, Nisha Padhye, and Teresa Urlacher for critical reading of the manuscript.
Disclosure of Potential Conflicts of Interest
During these studies H.G., J.L.K., L.C., and D.M.O. were employees at LI-COR Biosciences.
Supplemental Materials
Supplemental materials may be found here:
http://www.landesbioscience.com/journals/cbt/article/26719/
References
- Kari C, Chan TO, Rocha de Quadros M, Rodeck U. Targeting the epidermal growth factor receptor in cancer: apoptosis takes center stage. Cancer Res 2003; 63:1 - 5; PMID: 12517767
- Pines G, Köstler WJ, Yarden Y. Oncogenic mutant forms of EGFR: lessons in signal transduction and targets for cancer therapy. FEBS Lett 2010; 584:2699 - 706; http://dx.doi.org/10.1016/j.febslet.2010.04.019; PMID: 20388509
- Reilly RM, Kiarash R, Sandhu J, Lee YW, Cameron RG, Hendler A, Vallis K, Gariépy J. A comparison of EGF and MAb 528 labeled with 111In for imaging human breast cancer. J Nucl Med 2000; 41:903 - 11; PMID: 10809207
- Velikyan I, Sundberg AL, Lindhe O, Höglund AU, Eriksson O, Werner E, Carlsson J, Bergström M, Långström B, Tolmachev V. Preparation and evaluation of (68)Ga-DOTA-hEGF for visualization of EGFR expression in malignant tumors. J Nucl Med 2005; 46:1881 - 8; PMID: 16269603
- Ke S, Wen X, Gurfinkel M, Charnsangavej C, Wallace S, Sevick-Muraca EM, Li C. Near-infrared optical imaging of epidermal growth factor receptor in breast cancer xenografts. Cancer Res 2003; 63:7870 - 5; PMID: 14633715
- Kovar JL, Johnson MA, Volcheck WM, Chen J, Simpson MA. Hyaluronidase expression induces prostate tumor metastasis in an orthotopic mouse model. Am J Pathol 2006; 169:1415 - 26; http://dx.doi.org/10.2353/ajpath.2006.060324; PMID: 17003496
- Yarden Y, Sliwkowski MX. Untangling the ErbB signalling network. Nat Rev Mol Cell Biol 2001; 2:127 - 37; http://dx.doi.org/10.1038/35052073; PMID: 11252954
- Pedersen MW, Meltorn M, Damstrup L, Poulsen HS. The type III epidermal growth factor receptor mutation. Biological significance and potential target for anti-cancer therapy. Ann Oncol 2001; 12:745 - 60; http://dx.doi.org/10.1023/A:1011177318162; PMID: 11484948
- Wikstrand CJ, Reist CJ, Archer GE, Zalutsky MR, Bigner DD. The class III variant of the epidermal growth factor receptor (EGFRvIII): characterization and utilization as an immunotherapeutic target. J Neurovirol 1998; 4:148 - 58; http://dx.doi.org/10.3109/13550289809114515; PMID: 9584952
- Baselga J. Targeting tyrosine kinases in cancer: the second wave. Science 2006; 312:1175 - 8; http://dx.doi.org/10.1126/science.1125951; PMID: 16728632
- Cai W, Chen K, He L, Cao Q, Koong A, Chen X. Quantitative PET of EGFR expression in xenograft-bearing mice using 64Cu-labeled cetuximab, a chimeric anti-EGFR monoclonal antibody. Eur J Nucl Med Mol Imaging 2007; 34:850 - 8; http://dx.doi.org/10.1007/s00259-006-0361-6; PMID: 17262214
- Rosenthal EL, Kulbersh BD, King T, Chaudhuri TR, Zinn KR. Use of fluorescent labeled anti-epidermal growth factor receptor antibody to image head and neck squamous cell carcinoma xenografts. Mol Cancer Ther 2007; 6:1230 - 8; http://dx.doi.org/10.1158/1535-7163.MCT-06-0741; PMID: 17431103
- Nayak TK, Garmestani K, Milenic DE, Brechbiel MW. PET and MRI of metastatic peritoneal and pulmonary colorectal cancer in mice with human epidermal growth factor receptor 1-targeted 89Zr-labeled panitumumab. J Nucl Med 2012; 53:113 - 20; http://dx.doi.org/10.2967/jnumed.111.094169; PMID: 22213822
- Nayak TK, Garmestani K, Milenic DE, Baidoo KE, Brechbiel MW. HER1-targeted 86Y-panitumumab possesses superior targeting characteristics than 86Y-cetuximab for PET imaging of human malignant mesothelioma tumors xenografts. PLoS One 2011; 6:e18198; http://dx.doi.org/10.1371/journal.pone.0018198; PMID: 21464917
- Gong H, Kovar J, Little G, Chen H, Olive DM. In vivo imaging of xenograft tumors using an epidermal growth factor receptor-specific affibody molecule labeled with a near-infrared fluorophore. Neoplasia 2010; 12:139 - 49; PMID: 20126472
- Tolmachev V, Friedman M, Sandström M, Eriksson TL, Rosik D, Hodik M, Ståhl S, Frejd FY, Orlova A. Affibody molecules for epidermal growth factor receptor targeting in vivo: aspects of dimerization and labeling chemistry. J Nucl Med 2009; 50:274 - 83; http://dx.doi.org/10.2967/jnumed.108.055525; PMID: 19164241
- Cai W, Niu G, Chen X. Multimodality imaging of the HER-kinase axis in cancer. Eur J Nucl Med Mol Imaging 2008; 35:186 - 208; http://dx.doi.org/10.1007/s00259-007-0560-9; PMID: 17846765
- Gong H, Sampath L, Kovar JL, Olive DM. Targeting EGFR and HER2 for Molecular Imaging of Cancer. In: Schaller B, ed. Molecular Imaging: InTech; 2012:351-374.
- Yang W, Barth RF, Wu G, Ciesielski MJ, Fenstermaker RA, Moffat BA, Ross BD, Wikstrand CJ. Development of a syngeneic rat brain tumor model expressing EGFRvIII and its use for molecular targeting studies with monoclonal antibody L8A4. Clin Cancer Res 2005; 11:341 - 50; PMID: 15671565
- Oliveira S, van Dongen GA, Stigter-van Walsum M, Roovers RC, Stam JC, Mali W, van Diest PJ, van Bergen en Henegouwen PM. Rapid visualization of human tumor xenografts through optical imaging with a near-infrared fluorescent anti-epidermal growth factor receptor nanobody. Mol Imaging 2012; 11:33 - 46; PMID: 22418026
- Massoud TF, Gambhir SS. Molecular imaging in living subjects: seeing fundamental biological processes in a new light. Genes Dev 2003; 17:545 - 80; http://dx.doi.org/10.1101/gad.1047403; PMID: 12629038
- Weissleder R. A clearer vision for in vivo imaging. Nat Biotechnol 2001; 19:316 - 7; http://dx.doi.org/10.1038/86684; PMID: 11283581
- Kovar JL, Volcheck W, Sevick-Muraca E, Simpson MA, Olive DM. Characterization and performance of a near-infrared 2-deoxyglucose optical imaging agent for mouse cancer models. Anal Biochem 2009; 384:254 - 62; http://dx.doi.org/10.1016/j.ab.2008.09.050; PMID: 18938129
- Cheng Z, Levi J, Xiong Z, Gheysens O, Keren S, Chen X, Gambhir SS. Near-infrared fluorescent deoxyglucose analogue for tumor optical imaging in cell culture and living mice. Bioconjug Chem 2006; 17:662 - 9; http://dx.doi.org/10.1021/bc050345c; PMID: 16704203
- Chen X, Conti PS, Moats RA. In vivo near-infrared fluorescence imaging of integrin alphavbeta3 in brain tumor xenografts. Cancer Res 2004; 64:8009 - 14; http://dx.doi.org/10.1158/0008-5472.CAN-04-1956; PMID: 15520209
- Ntziachristos V, Schellenberger EA, Ripoll J, Yessayan D, Graves E, Bogdanov A Jr., Josephson L, Weissleder R. Visualization of antitumor treatment by means of fluorescence molecular tomography with an annexin V-Cy5.5 conjugate. Proc Natl Acad Sci U S A 2004; 101:12294 - 9; http://dx.doi.org/10.1073/pnas.0401137101; PMID: 15304657
- Nitin N, Rosbach KJ, El-Naggar A, Williams M, Gillenwater A, Richards-Kortum RR. Optical molecular imaging of epidermal growth factor receptor expression to improve detection of oral neoplasia. Neoplasia 2009; 11:542 - 51; PMID: 19484143
- Adams KE, Ke S, Kwon S, Liang F, Fan Z, Lu Y, Hirschi K, Mawad ME, Barry MA, Sevick-Muraca EM. Comparison of visible and near-infrared wavelength-excitable fluorescent dyes for molecular imaging of cancer. J Biomed Opt 2007; 12:024017; http://dx.doi.org/10.1117/1.2717137; PMID: 17477732
- Miao Z, Ren G, Liu H, Jiang L, Cheng Z. Cy5.5-labeled Affibody molecule for near-infrared fluorescent optical imaging of epidermal growth factor receptor positive tumors. J Biomed Opt 2010; 15:036007; http://dx.doi.org/10.1117/1.3432738; PMID: 20615009
- Barrett T, Koyama Y, Hama Y, Ravizzini G, Shin IS, Jang BS, Paik CH, Urano Y, Choyke PL, Kobayashi H. In vivo diagnosis of epidermal growth factor receptor expression using molecular imaging with a cocktail of optically labeled monoclonal antibodies. Clin Cancer Res 2007; 13:6639 - 48; http://dx.doi.org/10.1158/1078-0432.CCR-07-1119; PMID: 17982120
- Sampath L, Kwon S, Ke S, Wang W, Schiff R, Mawad ME, Sevick-Muraca EM. Dual-labeled trastuzumab-based imaging agent for the detection of human epidermal growth factor receptor 2 overexpression in breast cancer. J Nucl Med 2007; 48:1501 - 10; http://dx.doi.org/10.2967/jnumed.107.042234; PMID: 17785729
- Urano Y, Asanuma D, Hama Y, Koyama Y, Barrett T, Kamiya M, Nagano T, Watanabe T, Hasegawa A, Choyke PL, et al. Selective molecular imaging of viable cancer cells with pH-activatable fluorescence probes. Nat Med 2009; 15:104 - 9; http://dx.doi.org/10.1038/nm.1854; PMID: 19029979
- Zhu L, Xie J, Swierczewska M, Zhang F, Lin X, Fang X, Niu G, Lee S, Chen X. Dual-functional, receptor-targeted fluorogenic probe for in vivo imaging of extracellular protease expressions. Bioconjug Chem 2011; 22:1001 - 5; http://dx.doi.org/10.1021/bc200005w; PMID: 21574650
- Heath CH, Deep NL, Sweeny L, Zinn KR, Rosenthal EL. Use of panitumumab-IRDye800 to image microscopic head and neck cancer in an orthotopic surgical model. Ann Surg Oncol 2012; 19:3879 - 87; http://dx.doi.org/10.1245/s10434-012-2435-y; PMID: 22669455
- Patel D, Lahiji A, Patel S, Franklin M, Jimenez X, Hicklin DJ, Kang X. Monoclonal antibody cetuximab binds to and down-regulates constitutively activated epidermal growth factor receptor vIII on the cell surface. Anticancer Res 2007; 27:5A 3355 - 66; PMID: 17970081
- Lee FT, O’Keefe GJ, Gan HK, Mountain AJ, Jones GR, Saunder TH, Sagona J, Rigopoulos A, Smyth FE, Johns TG, et al. Immuno-PET quantitation of de2-7 epidermal growth factor receptor expression in glioma using 124I-IMP-R4-labeled antibody ch806. J Nucl Med 2010; 51:967 - 72; http://dx.doi.org/10.2967/jnumed.109.068395; PMID: 20484439
- Xujie L, Liu Z, Jia B, Shen L, Wang F. In vivo imaging of EGFRvIII-positive tumors using 125I-labeled a novel monoclonal antibody 4G1. J Nucl Med Meeting Abstracts 2013; 54:1151
- Perera RM, Zoncu R, Johns TG, Pypaert M, Lee FT, Mellman I, Old LJ, Toomre DK, Scott AM. Internalization, intracellular trafficking, and biodistribution of monoclonal antibody 806: a novel anti-epidermal growth factor receptor antibody. Neoplasia 2007; 9:1099 - 110; http://dx.doi.org/10.1593/neo.07721; PMID: 18084617
- Lee SB, Hassan M, Fisher R, Chertov O, Chernomordik V, Kramer-Marek G, Gandjbakhche A, Capala J. Affibody molecules for in vivo characterization of HER2-positive tumors by near-infrared imaging. Clin Cancer Res 2008; 14:3840 - 9; http://dx.doi.org/10.1158/1078-0432.CCR-07-4076; PMID: 18559604
- Tolmachev V, Rosik D, Wållberg H, Sjöberg A, Sandström M, Hansson M, Wennborg A, Orlova A. Imaging of EGFR expression in murine xenografts using site-specifically labelled anti-EGFR 111In-DOTA-Z EGFR:2377 Affibody molecule: aspect of the injected tracer amount. Eur J Nucl Med Mol Imaging 2010; 37:613 - 22; http://dx.doi.org/10.1007/s00259-009-1283-x; PMID: 19838701
- Ogawa M, Kosaka N, Choyke PL, Kobayashi H. In vivo molecular imaging of cancer with a quenching near-infrared fluorescent probe using conjugates of monoclonal antibodies and indocyanine green. Cancer Res 2009; 69:1268 - 72; http://dx.doi.org/10.1158/0008-5472.CAN-08-3116; PMID: 19176373
- Marshall MV, Draney D, Sevick-Muraca EM, Olive DM. Single-dose intravenous toxicity study of IRDye 800CW in Sprague-Dawley rats. Mol Imaging Biol 2010; 12:583 - 94; http://dx.doi.org/10.1007/s11307-010-0317-x; PMID: 20376568
- Terwisscha van Scheltinga AG, van Dam GM, Nagengast WB, Ntziachristos V, Hollema H, Herek JL, Schröder CP, Kosterink JG, Lub-de Hoog MN, de Vries EG. Intraoperative near-infrared fluorescence tumor imaging with vascular endothelial growth factor and human epidermal growth factor receptor 2 targeting antibodies. J Nucl Med 2011; 52:1778 - 85; http://dx.doi.org/10.2967/jnumed.111.092833; PMID: 21990576
- Gong H, Kovar JL, Baker B, Zhang A, Cheung L, Draney DR, Corrêa IR Jr., Xu MQ, Olive DM. Near-infrared fluorescence imaging of mammalian cells and xenograft tumors with SNAP-tag. PLoS One 2012; 7:e34003; http://dx.doi.org/10.1371/journal.pone.0034003; PMID: 22479502
- Gong H, Jarzynka MJ, Cole TJ, Lee JH, Wada T, Zhang B, Gao J, Song WC, DeFranco DB, Cheng SY, et al. Glucocorticoids antagonize estrogens by glucocorticoid receptor-mediated activation of estrogen sulfotransferase. Cancer Res 2008; 68:7386 - 93; http://dx.doi.org/10.1158/0008-5472.CAN-08-1545; PMID: 18794126