Abstract
Mammalian or mechanistic target of rapamycin (mTOR) is involved in growth, aging, and age-related diseases including cancer. There is an extensive cross talk between p53 and mTOR. In cell culture, p53 inhibits the mTOR pathway in a cell type-dependent manner. p53-deficient mice develop pro-inflammation and cancer. We have shown that rapamycin delayed cancer and extended lifespan, thus partially substituting for p53. Here we show that a marker of mTOR activity, phosphorylated S6 (p-S6), is increased in the hearts of p53-deficient mice. Furthermore, cardiac p-S6 correlated with body weight. Also, p53−/− mice were slightly hyperinsulinemic with a tendency to elevated IGF-1. Radiation exacerbated the difference between IGF-1 levels in normal and p53−/− mice. Noteworthy, radiation induced Thr-308 Akt phosphorylation in the livers (but not in the hearts) of both p53+/+ and p53−/− mice. Simultaneously, radiation decreased p-S6 in the livers of normal mice, consistent with the negative effect of p53 on mTOR. Our data indicate that the activity of mTOR is increased in some but not all tissues of p53−/− mice, associated with the tendency to increased insulin and IGF-1 levels. Therefore, the absence of p53 may create oncophilic microenvironment, favoring cancer.
Introduction
The p53 tumor suppressor can cause cell cycle arrest, apoptosis, and cellular senescence depending on cellular context.Citation1-Citation5 Although this is sufficient to explain its anti-cancer effect, p53 can suppress cancer independently of these activities.Citation6,Citation7 Noteworthy, p53 suppresses anabolic metabolismCitation5-Citation11 expression of repeats and noncoding RNAs,Citation12 NFκB,Citation13 the mTOR pathway,Citation9,Citation14-Citation20 and conversion from quiescence to senescence.Citation21,Citation22 p53 may promote and suppress senescence.Citation23-Citation31 While arresting cell cycle, p53 itself does not promote cellular senescence. It is growth-promoting pathways such as PI3K/mTOR that convert p53-induced cell cycle arrest into senescence.Citation21,Citation32-Citation35 By inhibiting the mTOR pathway, p53 can suppress conversion from arrest to senescence.Citation21,Citation22,Citation36 Senescent microenvironment favors cancer.Citation37-Citation46 Noteworthy, overexpression of p53 under physiological regulation increases lifespan and decreases cancer incidence.Citation47 Cancer is an age-related disease and its incidence increases exponentially with age. Aging is the most important “risk factor” of cancer and any interventions that slow aging also delay cancer.Citation44,Citation48-Citation52 Rapamycin slows down aging in numerous organisms, including those that do not die from cancer.Citation53-Citation59 As an anti-cancer and anti-aging agent, rapamycin can delay cancer not only directly but also indirectly: by slowing down aging. Mice lacking p53 die from cancer early in life.Citation60-Citation62 Rapamycin extends lifespan and delays cancer in p53+/− and p53−/− mice.Citation63-Citation65 In p53−/− mice both direct (anti-cancer) and indirect (anti-aging) models identically predict that rapamycin will delay cancer and extend life span. There is a hint for indirect effects: rapamycin is more effective when given early in life.Citation63 (In contrast, classic anti-cancer agents such as radiation promote cancer, especially when given early in life.) Do p53−/− mice have metabolic and tissue alterations that may favor both aging and cancer? It was already shown that p53−/− mice are characterized by pro-inflammatory syndromeCitation66,Citation67 and accelerated atherosclerosis.Citation68-Citation70 Here we investigated the insulin/Akt/mTOR pathway in p53−/− mice. Conventionally, we performed measurements after overnight fasting. We also included a second condition: radiation. In cell culture, in order to affect mTOR pathway, p53 should be induced. Therefore, we expected that the difference between normal and p53−/− mice would be exacerbated by radiation. For consistency, all mice were fasted overnight before examination.
Results
AKT/mTOR pathway in p53-knockout mice
First we measured phosphorylated AKT and S6 in the hearts (). We previously found that the heart muscle is suitable to measure these markers.Citation71 Levels of Akt phosphorylation at Thr-308 were not significantly different in p53-knockout mice (). Phospho-S6 (p-S6), the most validated target of the mTOR pathway, was significantly higher in p53-knockout mice (P = 0.0108). Radiation tended to decrease p-S6 in normal mice but not in p53-knockout mice ().
Figure 1. Levels of p-S6 and p-AKT in the hearts of normal (p53+/+) and p53-knockout (p53−/−) mice. (A) Immunoblot analysis of protein lysates from the hearts of 3.5-mo-old normal (p53+/+) and p53-knockout (p53−/−) mice, which were untreated (control) or irradiated with 10 gray (Rad), fasted overnight, and sacrificed. Numbers indicate individual mice. (B and C) Quantitave analysis of the data shown in (A). Quantified intensities of phosphorylated S6 (p-S6) signal (left panel) and signal of AKT phosphorylated at Thr308 (right panel) presented as mean ± SE. P values indicate statistically significant difference in p-S6 levels between p53+/+ vs p53−/− mice.
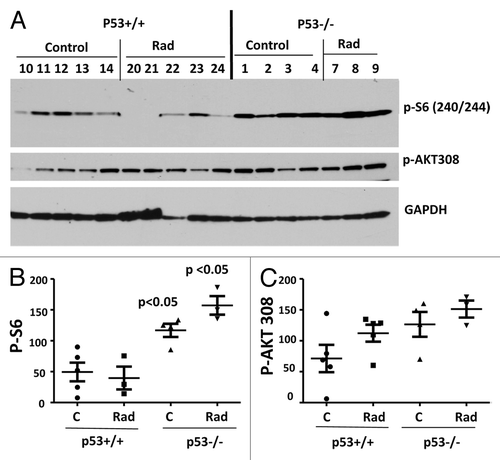
We next measured p-Akt-Thr-308 and p-S6 in the liver. Basal levels of p-Akt (Thr-308) and p-S6 were not changed in the livers of p53-knockout mice (). Radiation dramatically increased hepatic p-AKT (Thr-308) in both types of mice (). However, this was not translated into phosphorylation of S6 in normal mice ( and ). In normal mice, there was a dose-dependent decrease in p-S6 by radiation, which reached statistical significance at 10 Gray (). While activating Akt (Thr-308) in a p53-independent manner (), radiation decreased p-S6 in a p53-dependent manner (). Phosphorylation of Akt at Ser-473, a site phosphorylated by mTORC2, was not significantly affected by radiation in the livers of normal mice ().
Figure 2. Levels of p-S6 and p-AKT in the livers of normal (p53+/+) and p53-knockout (p53−/−) mice. (A) Immunoblot analysis of protein lysates from the livers of 3.5-mo-old normal (p53+/+) and p53-knockout (p53−/−) mice, which were untreated (control) or irradiated for 1 h (Rad), fasted overnight and sacrificed. Note: p53−/− mice in Rad group received 10 gray radiation; p53+/+ Rad group received 5 gray (mice 18 and 19) or 10 gray (mice 23 and 24) radiation. Numbers indicate individual mice. (B and C) Quantitative analysis of data shown in (A). Quantified intensities of phosphorylated S6 (p-S6) signal (left panel) and signal of AKT phosphorylated at Thr308 (right panel) presented as mean ± SE. P < 0.0001 values indicate statistically significant difference between respective control and irradiated groups using two-tailed t test analysis.
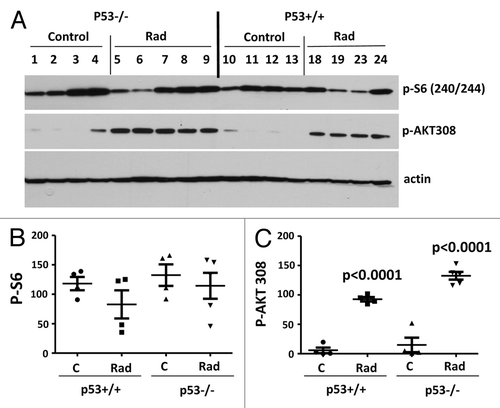
Figure 3. p-S6 and p-AKT (S473) in the livers of normal (p53+/+) mice: control and irradiated. (A) Immunoblot analysis of protein lysates from the livers of 3.5-mo-old normal (p53+/+) mice, which were untreated (control) or irradiated for 1 h with 5 gray (Rad [5]) or 10 gray (Rad [10]), fasted overnight, and sacrificed. (B and C) Quantitative analysis of data shown in (A). Quantified intensities of phosphorylated S6 (p-S6) signal (left panel) and signal of AKT phosphorylated at Ser473 (right panel) presented as mean ± SE. P = 0.0115 value indicates statistically significant difference between control and irradiated with 10 gray groups using two-tailed t test analysis.
![Figure 3. p-S6 and p-AKT (S473) in the livers of normal (p53+/+) mice: control and irradiated. (A) Immunoblot analysis of protein lysates from the livers of 3.5-mo-old normal (p53+/+) mice, which were untreated (control) or irradiated for 1 h with 5 gray (Rad [5]) or 10 gray (Rad [10]), fasted overnight, and sacrificed. (B and C) Quantitative analysis of data shown in (A). Quantified intensities of phosphorylated S6 (p-S6) signal (left panel) and signal of AKT phosphorylated at Ser473 (right panel) presented as mean ± SE. P = 0.0115 value indicates statistically significant difference between control and irradiated with 10 gray groups using two-tailed t test analysis.](/cms/asset/ff6f2353-ae13-459d-aea2-74ccad8c9ece/kcbt_a_10926947_f0003.gif)
Metabolic alterations in p53-knockout mice
Given that mTOR drives cellular mass growth, hypertrophy of p53−/− mice was expected. In agreement, p53−/− mice were heavier than normal (p53+/+) mice (). The difference was not dramatic but still statistically significant (P = 0.0355). Noteworthy, fasting leptin levels showed the opposite tendency (), in contrast to a strong positive correlation between weight and leptin in mice of the same genotype shown previously.Citation71,Citation72 Since leptin is secreted by fat cells and reflects fat content, p53-knockout mice seem to be more hypertrophic rather than fatter. Importantly, there was a positive correlation between body weight and p-S6 levels in the heart tissue (). Fasting levels of glucose did not differ between p53-deficient and normal mice (). Fasting insulin levels were increased in p53−/− mice (). Noteworthy, levels of insulin and IGF-1 were not altered in younger p53−/− mice, when measured without fasting (not shown). This indicates that hyperinsulinemia is detectable in certain conditions, depending on the age and fasting. We next irradiated mice to induce p53. Radiation did not affect glucose levels in both types of mice and increased insulin levels only in normal mice (). Levels of IGF-1, a marker associated with accelerated aging,Citation73-Citation75 tended to be increased in p53−/− mice, reaching a statistical significance after radiation ().
Figure 4. Metabolic profile of 3.5-mo-old normal (p53+/+) and p53−/− mice. (A) Weight (g) of normal (n = 15) and p53-knockout (n = 9) mice. Data present mean ± SE. (B) Fasting leptin levels of control normal (n = 5) and p53-knockout (n = 4) mice presented as mean ± SE. (C) Correlation between weight and intensity of p-S6 signal in the heart of control normal and p53-knockout mice. r, Pearson coefficient. (D) Glucose levels (fasting) in normal and p53-knockout mice: control and irradiated—presented as mean ± SE. (E) Insulin levels (fasting) in normal and p53-knockout mice: control and irradiated—presented as mean ± SE. P = 0.0392 and P = 0.0442 values indicate statistically significant difference between control and combined irradiated groups of p53+/+ mice and control and irradiated groups of p53−/− mice, respectively. (F) IGF1 levels in plasma of normal and p53-knockout mice: control and irradiated—presented as mean ± SE. P = 0.0115 value indicated statistically significant difference between control and combined irradiated groups of p53+/+ mice.
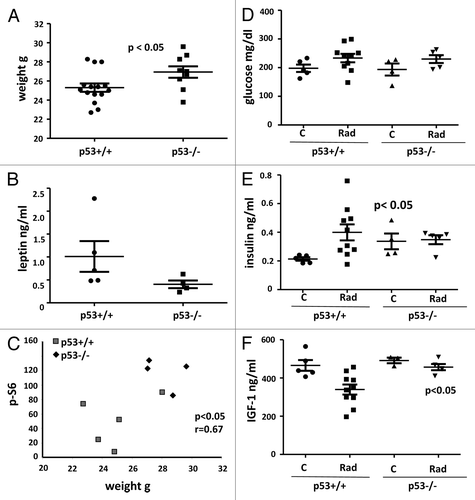
Discussion
We showed that nutrient- and insulin-sensing pathways are slightly dysregulated in p53−/− mice. Our study provides a glimpse into the complexity of p53-dependent regulation of mTOR in the organism. There were tissue-specific (the liver vs. the heart) differences in phosphorylation of S6 and Akt. P-S6 levels were increased in the heart muscle of p53−/− mice. Increased levels of p-S6 predict systemic alterations: increased body growth and insulin levels. In fact, p53−/− mice were slightly heavier than normal mice, consistent with the role of mTOR in growth. Also, activated mTOR can cause insulin resistance by feedback inhibition of insulin signaling.Citation76,Citation77 In fact, basal levels of fasting insulin were increased in p53−/− mice, consistent with resistance to insulin, given that levels of glucose were unchanged. The mTOR/S6K pathway is involved in aging.Citation78 Hyperinsulinemia due to insulin resistance may accelerate aging and cancer.Citation79 In cell culture, in order to inhibit the mTOR pathway, p53 should be induced.Citation21 Therefore, mice were irradiated to imitate the cell culture conditions and to exacerbate p53-dependent trends. Indeed, in irradiated p53−/− mice, levels of IGF-1 were significantly increased compared with irradiated normal mice. Higher levels of IGF-1 are associated with accelerated aging in mice and humans.Citation80-Citation84 The most striking alteration caused by radiation was phosphorylation of Akt at Thr-308 in the livers of both types of mice, thus in a p53-independent manner (). In response to insulin and IGF-1, Thr-308 is normally phosphorylated, which leads to activation of Akt (). In normal mice, radiation induced Thr-308 phosphorylation and decreased p-S6 ().
Metabolic alterations in normal tissues of p53−/− mice can be termed oncophilic. The main cause of cancer in p53−/− mice is the loss of cell cycle control in cells that give rise to tumors. Also, loss of p53 may activate mTOR in tumor cells, given that activation of the PI-3K/mTOR pathway is the most common alteration in cancer.Citation85,Citation86 In addition, our data suggest that there may be also organism-dependent factors of accelerated tumorogenesis. This can explain why calorie restriction, which is known to inhibit mTOR and decelerate organismal aging, delays cancer, and prolongs life span in p53−/− mice.Citation87 Similarly, rapamycin delayed cancer in p53+/− and p53−/− mice.Citation63,Citation64 Noteworthy, the effect of rapamycin was blunted when it was used later in life, consistent with its indirect anti-cancer effect.Citation63 In conclusion, mice lacking p53 is characterized by oncophilic metabolism, an additional factor fostering carcinogenesis.
Materials and Methods
Mice
Animal studies were conducted in accordance with the regulations of the Committee of Animal Care and Use at Roswell Park Cancer Institute. In the study 15 normal (p53+/+) 3.5-mo-old C57B1/6 male and 9 p53-knockout 3.5-mo-old male mice were used. The colony of p53-knockout mice on a C57B1/6 background (obtained from Jackson Laboratories) was maintained by crossing p53+/− females with p53−/− males followed by genotyping of the progeny by PCR as described previously.Citation88 Animals were randomly assigned to control and irradiated groups. Normal (p53+/+) mice were divided into 3 groups: control (untreated) (n = 5), irradiated with 5 gray (n = 5), and irradiated with 10 gray (n = 5). p53−/− mice were divided into 2 groups: control (n = 4) and irradiated with 10 gray (n = 5). All mice were fasted overnight and sacrificed. Fasted blood and organs were collected. Plasma was prepared and used for biochemical analyses.
Immunoblot analysis
Tissues were homogenized in Bullet blender using stainless steel 0.5 mm diameter beads (Next Advantage, Inc.) and RIPA lysis buffer supplemented with protease and phosphatase inhibitors tablets (Roche Diagnostics). Lysates were cleared by centrifugation at 4 °C at 13 000 rpm. Equal amounts of protein were separated on gradient Criterion gels (BioRad). Primary antibodies used: rabbit anti-phospho S6(Ser 240/244), anti-phospho AKT(Ser473), and phospho AKT(Thr308) (Cell Signaling Biotechnology); monoclonal anti-β-actin-peroxidase (Sigma-Aldrich).
Glucose levels in blood plasma
Glucose levels in blood plasma were measured using Accu-Chek Aviva strips (McKesson).
Insulin concentration in blood plasma
Insulin concentration in blood plasma was measured using Insulin (Mouse) Ultrsensitive ELISA kit (ALPCO Diagnostics) according to manufacturer’s protocol. Data were analyzed using range of insulin standards and four parameter logistic fit.
IGF1 concentration in blood plasma
IGF1 concentration in blood plasma was determined using IGF1 (Mouse/Rat) ELISA kit (ALPCO Diagnostics) according to manufacturer’s protocol. Data were analyzed using range of IGF1 standards and four parameter logistic fit.
Leptin concentration in blood plasma
Leptin concentration in blood plasma was determined using Mouse Leptin ELISA kit (Crystal Chem Inc.) using according to manufacturer’s protocol. Data were analyzed using range of leptin standards and four parameter logistic fit.
Statistical analyses
Statistical analyses were performed using GraphOad Prizm 5.00 for Windows, GraphPad Software, http://www.graphpad.com.
Disclosure of Potential Conflicts of Interest
No potential conflicts of interest were disclosed.
References
- Vogelstein B, Lane DP, Levine AJ. Surfing the p53 network. Nature 2000; 408:307 - 10; http://dx.doi.org/10.1038/35042675; PMID: 11099028
- Rozan LM, El-Deiry WS. p53 downstream target genes and tumor suppression: a classical view in evolution. Cell Death Differ 2007; 14:3 - 9; http://dx.doi.org/10.1038/sj.cdd.4402058; PMID: 17068503
- Gudkov AV, Komarova EA. Dangerous habits of a security guard: the two faces of p53 as a drug target. Hum Mol Genet 2007; 16:Spec No 1 R67 - 72; http://dx.doi.org/10.1093/hmg/ddm052; PMID: 17613549
- Levine AJ, Oren M. The first 30 years of p53: growing ever more complex. Nat Rev Cancer 2009; 9:749 - 58; http://dx.doi.org/10.1038/nrc2723; PMID: 19776744
- Vousden KH, Prives C. Blinded by the Light: The Growing Complexity of p53. Cell 2009; 137:413 - 31; http://dx.doi.org/10.1016/j.cell.2009.04.037; PMID: 19410540
- Li T, Kon N, Jiang L, Tan M, Ludwig T, Zhao Y, Baer R, Gu W. Tumor suppression in the absence of p53-mediated cell-cycle arrest, apoptosis, and senescence. Cell 2012; 149:1269 - 83; http://dx.doi.org/10.1016/j.cell.2012.04.026; PMID: 22682249
- Blagosklonny MV. Tumor suppression by p53 without apoptosis and senescence: conundrum or rapalog-like gerosuppression?. Aging (Albany NY) 2012; 4:450 - 5; PMID: 22869016
- Zhu Y, Prives C. p53 and Metabolism: The GAMT Connection. Mol Cell 2009; 36:351 - 2; http://dx.doi.org/10.1016/j.molcel.2009.10.026; PMID: 19917243
- Feng Z, Levine AJ. The regulation of energy metabolism and the IGF-1/mTOR pathways by the p53 protein. Trends Cell Biol 2010; 20:427 - 34; http://dx.doi.org/10.1016/j.tcb.2010.03.004; PMID: 20399660
- Altilia S, Santoro A, Malagoli D, Lanzarini C, Ballesteros Álvarez JA, Galazzo G, Porter DC, Crocco P, Rose G, Passarino G, et al. TP53 codon 72 polymorphism affects accumulation of mtDNA damage in human cells. Aging (Albany NY) 2012; 4:28 - 39; PMID: 22289634
- Madan E, Gogna R, Bhatt M, Pati U, Kuppusamy P, Mahdi AA. Regulation of glucose metabolism by p53: emerging new roles for the tumor suppressor. Oncotarget 2011; 2:948 - 57; PMID: 22248668
- Leonova KI, Brodsky L, Lipchick B, Pal M, Novototskaya L, Chenchik AA, Sen GC, Komarova EA, Gudkov AV. p53 cooperates with DNA methylation and a suicidal interferon response to maintain epigenetic silencing of repeats and noncoding RNAs. Proc Natl Acad Sci U S A 2013; 110:E89 - 98; http://dx.doi.org/10.1073/pnas.1216922110; PMID: 23236145
- Gurova KV, Hill JE, Guo C, Prokvolit A, Burdelya LG, Samoylova E, Khodyakova AV, Ganapathi R, Ganapathi M, Tararova ND, et al. Small molecules that reactivate p53 in renal cell carcinoma reveal a NF-kappaB-dependent mechanism of p53 suppression in tumors. Proc Natl Acad Sci U S A 2005; 102:17448 - 53; http://dx.doi.org/10.1073/pnas.0508888102; PMID: 16287968
- Matthew EM, Hart LS, Astrinidis A, Navaraj A, Dolloff NG, Dicker DT, Henske EP, El-Deiry WS. The p53 target Plk2 interacts with TSC proteins impacting mTOR signaling, tumor growth and chemosensitivity under hypoxic conditions. Cell Cycle 2009; 8:4168 - 75; http://dx.doi.org/10.4161/cc.8.24.10800; PMID: 20054236
- Horton LE, Bushell M, Barth-Baus D, Tilleray VJ, Clemens MJ, Hensold JO. p53 activation results in rapid dephosphorylation of the eIF4E-binding protein 4E-BP1, inhibition of ribosomal protein S6 kinase and inhibition of translation initiation. Oncogene 2002; 21:5325 - 34; http://dx.doi.org/10.1038/sj.onc.1205662; PMID: 12149653
- Stambolic V, MacPherson D, Sas D, Lin Y, Snow B, Jang Y, Benchimol S, Mak TW. Regulation of PTEN transcription by p53. Mol Cell 2001; 8:317 - 25; http://dx.doi.org/10.1016/S1097-2765(01)00323-9; PMID: 11545734
- Feng Z, Zhang H, Levine AJ, Jin S. The coordinate regulation of the p53 and mTOR pathways in cells. Proc Natl Acad Sci U S A 2005; 102:8204 - 9; http://dx.doi.org/10.1073/pnas.0502857102; PMID: 15928081
- Levine AJ, Feng Z, Mak TW, You H, Jin S. Coordination and communication between the p53 and IGF-1-AKT-TOR signal transduction pathways. Genes Dev 2006; 20:267 - 75; http://dx.doi.org/10.1101/gad.1363206; PMID: 16452501
- Levine AJ, Harris CR, Puzio-Kuter AM. The interfaces between signal transduction pathways: IGF-1/mTor, p53 and the Parkinson Disease pathway. Oncotarget 2012; 3:1301 - 7; PMID: 23211569
- Loayza-Puch F, Drost J, Rooijers K, Lopes R, Elkon R, Agami R. p53 induces transcriptional and translational programs to suppress cell proliferation and growth. Genome Biol 2013; 14:R32; http://dx.doi.org/10.1186/gb-2013-14-4-r32; PMID: 23594524
- Demidenko ZN, Korotchkina LG, Gudkov AV, Blagosklonny MV. Paradoxical suppression of cellular senescence by p53. Proc Natl Acad Sci U S A 2010; 107:9660 - 4; http://dx.doi.org/10.1073/pnas.1002298107; PMID: 20457898
- Korotchkina LG, Leontieva OV, Bukreeva EI, Demidenko ZN, Gudkov AV, Blagosklonny MV. The choice between p53-induced senescence and quiescence is determined in part by the mTOR pathway. Aging (Albany NY) 2010; 2:344 - 52; PMID: 20606252
- Tucci P. Caloric restriction: is mammalian life extension linked to p53?. Aging (Albany NY) 2012; 4:525 - 34; PMID: 22983298
- de Keizer PL, Laberge RM, Campisi J. p53: Pro-aging or pro-longevity?. Aging (Albany NY) 2010; 2:377 - 9; PMID: 20657035
- Coppé JP, Patil CK, Rodier F, Sun Y, Muñoz DP, Goldstein J, Nelson PS, Desprez PY, Campisi J. Senescence-associated secretory phenotypes reveal cell-nonautonomous functions of oncogenic RAS and the p53 tumor suppressor. PLoS Biol 2008; 6:2853 - 68; http://dx.doi.org/10.1371/journal.pbio.0060301; PMID: 19053174
- Lee JH, Bodmer R, Bier E, Karin M. Sestrins at the crossroad between stress and aging. Aging (Albany NY) 2010; 2:369 - 74; PMID: 20606249
- Horikawa I, Fujita K, Harris CC. p53 governs telomere regulation feedback too, via TRF2. Aging (Albany NY) 2011; 3:26 - 32; PMID: 21266744
- Dulic V. Be quiet and you’ll keep young: does mTOR underlie p53 action in protecting against senescence by favoring quiescence?. Aging (Albany NY) 2011; 3:3 - 4; PMID: 21248373
- Galluzzi L, Kepp O, Kroemer G. TP53 and MTOR crosstalk to regulate cellular senescence. Aging (Albany NY) 2010; 2:535 - 7; PMID: 20876940
- McGee MD, Day N, Graham J, Melov S. cep-1/p53-dependent dysplastic pathology of the aging C. elegans gonad. Aging (Albany NY) 2012; 4:256 - 69; PMID: 22562940
- Chao SK, Horwitz SB, McDaid HM. Insights into 4E-BP1 and p53 mediated regulation of accelerated cell senescence. Oncotarget 2011; 2:89 - 98; PMID: 21399233
- Leontieva OV, Blagosklonny MV. DNA damaging agents and p53 do not cause senescence in quiescent cells, while consecutive re-activation of mTOR is associated with conversion to senescence. Aging (Albany NY) 2010; 2:924 - 35; PMID: 21212465
- Leontieva OV, Natarajan V, Demidenko ZN, Burdelya LG, Gudkov AV, Blagosklonny MV. Hypoxia suppresses conversion from proliferative arrest to cellular senescence. Proc Natl Acad Sci U S A 2012; 109:13314 - 8; http://dx.doi.org/10.1073/pnas.1205690109; PMID: 22847439
- Blagosklonny MV. Cell cycle arrest is not yet senescence, which is not just cell cycle arrest: terminology for TOR-driven aging. Aging (Albany NY) 2012; 4:159 - 65; PMID: 22394614
- Kolesnichenko M, Hong L, Liao R, Vogt PK, Sun P. Attenuation of TORC1 signaling delays replicative and oncogenic RAS-induced senescence. Cell Cycle 2012; 11:2391 - 401; http://dx.doi.org/10.4161/cc.20683; PMID: 22627671
- Leontieva OV, Lenzo F, Demidenko ZN, Blagosklonny MV. Hyper-mitogenic drive coexists with mitotic incompetence in senescent cells. Cell Cycle 2012; 11:4642 - 9; http://dx.doi.org/10.4161/cc.22937; PMID: 23187803
- Krtolica A, Campisi J. Cancer and aging: a model for the cancer promoting effects of the aging stroma. Int J Biochem Cell Biol 2002; 34:1401 - 14; http://dx.doi.org/10.1016/S1357-2725(02)00053-5; PMID: 12200035
- Parrinello S, Coppe JP, Krtolica A, Campisi J. Stromal-epithelial interactions in aging and cancer: senescent fibroblasts alter epithelial cell differentiation. J Cell Sci 2005; 118:485 - 96; http://dx.doi.org/10.1242/jcs.01635; PMID: 15657080
- Martens JW, Sieuwerts AM, Bolt-deVries J, Bosma PT, Swiggers SJ, Klijn JG, Foekens JA. Aging of stromal-derived human breast fibroblasts might contribute to breast cancer progression. Thromb Haemost 2003; 89:393 - 404; PMID: 12574821
- Mikheev AM, Stoll EA, Ramakrishna R, Mikheeva SA, Horner PJ, Rostomily RC. Geropotency: increased malignant potential of aging neural progenitors. Aging (Albany NY) 2012; 4:854 - 5; PMID: 23257545
- Capparelli C, Guido C, Whitaker-Menezes D, Bonuccelli G, Balliet R, Pestell TG, Goldberg AF, Pestell RG, Howell A, Sneddon S, et al. Autophagy and senescence in cancer-associated fibroblasts metabolically supports tumor growth and metastasis via glycolysis and ketone production. Cell Cycle 2012; 11:2285 - 302; http://dx.doi.org/10.4161/cc.20718; PMID: 22684298
- Mercier I, Camacho J, Titchen K, Gonzales DM, Quann K, Bryant KG, Molchansky A, Milliman JN, Whitaker-Menezes D, Sotgia F, et al. Caveolin-1 and accelerated host aging in the breast tumor microenvironment: chemoprevention with rapamycin, an mTOR inhibitor and anti-aging drug. Am J Pathol 2012; 181:278 - 93; http://dx.doi.org/10.1016/j.ajpath.2012.03.017; PMID: 22698676
- Prabhu VV, Warfel NA, El-Deiry WS. CTGF-mediated autophagy-senescence transition in tumor stroma promotes anabolic tumor growth and metastasis. Cell Cycle 2012; 11:2592 - 3; http://dx.doi.org/10.4161/cc.21240; PMID: 22751431
- Lewis DA, Travers JB, Machado C, Somani AK, Spandau DF. Reversing the aging stromal phenotype prevents carcinoma initiation. Aging (Albany NY) 2011; 3:407 - 16; PMID: 21515933
- Greaves M. Leukemogenesis and ageing: 'fit for transformation'?. Aging (Albany, NY Online) 2011; 3:79 - 80
- Henry CJ, Marusyk A, DeGregori J. Aging-associated changes in hematopoiesis and leukemogenesis: what’s the connection?. Aging (Albany NY) 2011; 3:643 - 56; PMID: 21765201
- Matheu A, Maraver A, Klatt P, Flores I, Garcia-Cao I, Borras C, Flores JM, Viña J, Blasco MA, Serrano M. Delayed ageing through damage protection by the Arf/p53 pathway. Nature 2007; 448:375 - 9; http://dx.doi.org/10.1038/nature05949; PMID: 17637672
- Blagosklonny MV. Prevention of cancer by inhibiting aging. Cancer Biol Ther 2008; 7:1520 - 4; http://dx.doi.org/10.4161/cbt.7.10.6663; PMID: 18769112
- Blagosklonny MV. Molecular damage in cancer: an argument for mTOR-driven aging. Aging (Albany NY) 2011; 3:1130 - 41; PMID: 22246147
- Anisimov VN, Berstein LM, Popovich IG, Zabezhinski MA, Egormin PA, Piskunova TS, Semenchenko AV, Tyndyk ML, Yurova MN, Kovalenko IG, et al. If started early in life, metformin treatment increases life span and postpones tumors in female SHR mice. Aging (Albany NY) 2011; 3:148 - 57; PMID: 21386129
- Blagosklonny MV. Rapalogs in cancer prevention: anti-aging or anticancer?. Cancer Biol Ther 2012; 13:1349 - 54; http://dx.doi.org/10.4161/cbt.22859; PMID: 23151465
- Berstein LM. Metformin in obesity, cancer and aging: addressing controversies. Aging (Albany NY) 2012; 4:320 - 9; PMID: 22589237
- Stipp D. A new path to longevity. Sci Am 2012; 306:32 - 9; http://dx.doi.org/10.1038/scientificamerican0112-32; PMID: 22279832
- Kapahi P, Chen D, Rogers AN, Katewa SD, Li PW, Thomas EL, Kockel L. With TOR, less is more: a key role for the conserved nutrient-sensing TOR pathway in aging. Cell Metab 2010; 11:453 - 65; http://dx.doi.org/10.1016/j.cmet.2010.05.001; PMID: 20519118
- Johnson SC, Rabinovitch PS, Kaeberlein M. mTOR is a key modulator of ageing and age-related disease. Nature 2013; 493:338 - 45; http://dx.doi.org/10.1038/nature11861; PMID: 23325216
- Kaeberlein M. Longevity and aging. F1000Prime Rep 2013; 5:5; http://dx.doi.org/10.12703/P5-5; PMID: 23513177
- Bjedov I, Toivonen JM, Kerr F, Slack C, Jacobson J, Foley A, Partridge L. Mechanisms of life span extension by rapamycin in the fruit fly Drosophila melanogaster. Cell Metab 2010; 11:35 - 46; http://dx.doi.org/10.1016/j.cmet.2009.11.010; PMID: 20074526
- Flynn JM, O’Leary MN, Zambataro CA, Academia EC, Presley MP, Garrett BJ, Zykovich A, Mooney SD, Strong R, Rosen CJ, et al. Late-life rapamycin treatment reverses age-related heart dysfunction. Aging Cell 2013; 12:851 - 62; http://dx.doi.org/10.1111/acel.12109; PMID: 23734717
- Blagosklonny MV. How to save Medicare: the anti-aging remedy. Aging (Albany NY) 2012; 4:547 - 52; PMID: 22915707
- Donehower LA, Harvey M, Slagle BL, McArthur MJ, Montgomery CA Jr., Butel JS, Bradley A. Mice deficient for p53 are developmentally normal but susceptible to spontaneous tumours. Nature 1992; 356:215 - 21; http://dx.doi.org/10.1038/356215a0; PMID: 1552940
- Donehower LA, Harvey M, Vogel H, McArthur MJ, Montgomery CA Jr., Park SH, Thompson T, Ford RJ, Bradley A. Effects of genetic background on tumorigenesis in p53-deficient mice. Mol Carcinog 1995; 14:16 - 22; http://dx.doi.org/10.1002/mc.2940140105; PMID: 7546219
- Jacks T, Remington L, Williams BO, Schmitt EM, Halachmi S, Bronson RT, Weinberg RA. Tumor spectrum analysis in p53-mutant mice. Curr Biol 1994; 4:1 - 7; http://dx.doi.org/10.1016/S0960-9822(00)00002-6; PMID: 7922305
- Komarova EA, Antoch MP, Novototskaya LR, Chernova OB, Paszkiewicz G, Leontieva OV, Blagosklonny MV, Gudkov AV. Rapamycin extends lifespan and delays tumorigenesis in heterozygous p53+/- mice. Aging (Albany NY) 2012; 4:709 - 14; PMID: 23123616
- Comas M, Toshkov I, Kuropatwinski KK, Chernova OB, Polinsky A, Blagosklonny MV, Gudkov AV, Antoch MP. New nanoformulation of rapamycin Rapatar extends lifespan in homozygous p53-/- mice by delaying carcinogenesis. Aging (Albany NY) 2012; 4:715 - 22; PMID: 23117593
- Donehower LA. Rapamycin as longevity enhancer and cancer preventative agent in the context of p53 deficiency. Aging (Albany NY) 2012; 4:660 - 1; PMID: 23128359
- Komarova EA, Krivokrysenko V, Wang K, Neznanov N, Chernov MV, Komarov PG, Brennan ML, Golovkina TV, Rokhlin OW, Kuprash DV, et al. p53 is a suppressor of inflammatory response in mice. FASEB J 2005; 19:1030 - 2; PMID: 15811878
- Gudkov AV, Gurova KV, Komarova EA. Inflammation and p53: A Tale of Two Stresses. Genes Cancer 2011; 2:503 - 16; http://dx.doi.org/10.1177/1947601911409747; PMID: 21779518
- Guevara NV, Kim HS, Antonova EI, Chan L. The absence of p53 accelerates atherosclerosis by increasing cell proliferation in vivo. Nat Med 1999; 5:335 - 9; http://dx.doi.org/10.1038/6585; PMID: 10086392
- van Vlijmen BJ, Gerritsen G, Franken AL, Boesten LS, Kockx MM, Gijbels MJ, Vierboom MP, van Eck M, van De Water B, van Berkel TJ, et al. Macrophage p53 deficiency leads to enhanced atherosclerosis in APOE*3-Leiden transgenic mice. Circ Res 2001; 88:780 - 6; http://dx.doi.org/10.1161/hh0801.089261; PMID: 11325869
- Mercer J, Bennett M. The role of p53 in atherosclerosis. Cell Cycle 2006; 5:1907 - 9; http://dx.doi.org/10.4161/cc.5.17.3166; PMID: 16929177
- Leontieva OV, Paszkiewicz GM, Blagosklonny MV. Mechanistic or mammalian target of rapamycin (mTOR) may determine robustness in young male mice at the cost of accelerated aging. Aging (Albany NY) 2012; 4:899 - 916; PMID: 23443503
- Leontieva OV, Paszkiewicz G, Demidenko ZN, Blagosklonny MV. Resveratrol potentiates rapamycin to prevent hyperinsulinemia and obesity in male mice on high fat diet. Cell Death Dis 2013; 4:e472; http://dx.doi.org/10.1038/cddis.2012.202; PMID: 23348586
- van Heemst D, Beekman M, Mooijaart SP, Heijmans BT, Brandt BW, Zwaan BJ, Slagboom PE, Westendorp RG. Reduced insulin/IGF-1 signalling and human longevity. Aging Cell 2005; 4:79 - 85; http://dx.doi.org/10.1111/j.1474-9728.2005.00148.x; PMID: 15771611
- Yuan R, Tsaih SW, Petkova SB, Marin de Evsikova C, Xing S, Marion MA, Bogue MA, Mills KD, Peters LL, Bult CJ, et al. Aging in inbred strains of mice: study design and interim report on median lifespans and circulating IGF1 levels. Aging Cell 2009; 8:277 - 87; http://dx.doi.org/10.1111/j.1474-9726.2009.00478.x; PMID: 19627267
- Vitale G, Brugts MP, Ogliari G, Castaldi D, Fatti LM, Varewijck AJ, Lamberts SW, Monti D, Bucci L, Cevenini E, et al. Low circulating IGF-I bioactivity is associated with human longevity: findings in centenarians’ offspring. Aging (Albany NY) 2012; 4:580 - 9; PMID: 22983440
- Um SH, Frigerio F, Watanabe M, Picard F, Joaquin M, Sticker M, Fumagalli S, Allegrini PR, Kozma SC, Auwerx J, et al. Absence of S6K1 protects against age- and diet-induced obesity while enhancing insulin sensitivity. Nature 2004; 431:200 - 5; http://dx.doi.org/10.1038/nature02866; PMID: 15306821
- Shah OJ, Wang Z, Hunter T. Inappropriate activation of the TSC/Rheb/mTOR/S6K cassette induces IRS1/2 depletion, insulin resistance, and cell survival deficiencies. Curr Biol 2004; 14:1650 - 6; http://dx.doi.org/10.1016/j.cub.2004.08.026; PMID: 15380067
- Selman C, Tullet JM, Wieser D, Irvine E, Lingard SJ, Choudhury AI, Claret M, Al-Qassab H, Carmignac D, Ramadani F, et al. Ribosomal protein S6 kinase 1 signaling regulates mammalian life span. Science 2009; 326:140 - 4; http://dx.doi.org/10.1126/science.1177221; PMID: 19797661
- Masternak MM, Panici JA, Bonkowski MS, Hughes LF, Bartke A. Insulin sensitivity as a key mediator of growth hormone actions on longevity. J Gerontol A Biol Sci Med Sci 2009; 64:516 - 21; http://dx.doi.org/10.1093/gerona/glp024; PMID: 19304940
- Suh Y, Atzmon G, Cho MO, Hwang D, Liu B, Leahy DJ, Barzilai N, Cohen P. Functionally significant insulin-like growth factor I receptor mutations in centenarians. Proc Natl Acad Sci U S A 2008; 105:3438 - 42; http://dx.doi.org/10.1073/pnas.0705467105; PMID: 18316725
- Tatar M, Bartke A, Antebi A. The endocrine regulation of aging by insulin-like signals. Science 2003; 299:1346 - 51; http://dx.doi.org/10.1126/science.1081447; PMID: 12610294
- Bartke A. Long-lived Klotho mice: new insights into the roles of IGF-1 and insulin in aging. Trends Endocrinol Metab 2006; 17:33 - 5; http://dx.doi.org/10.1016/j.tem.2006.01.002; PMID: 16458526
- Guevara-Aguirre J, Balasubramanian P, Guevara-Aguirre M, Wei M, Madia F, Cheng CW, Hwang D, Martin-Montalvo A, Saavedra J, Ingles S, et al. Growth hormone receptor deficiency is associated with a major reduction in pro-aging signaling, cancer, and diabetes in humans. Sci Transl Med 2011; 3:70ra13; http://dx.doi.org/10.1126/scitranslmed.3001845; PMID: 21325617
- Bartke A. Healthy aging: is smaller better? - a mini-review. Gerontology 2012; 58:337 - 43; http://dx.doi.org/10.1159/000335166; PMID: 22261798
- Steelman LS, Chappell WH, Abrams SL, Kempf RC, Long J, Laidler P, Mijatovic S, Maksimovic-Ivanic D, Stivala F, Mazzarino MC, et al. Roles of the Raf/MEK/ERK and PI3K/PTEN/Akt/mTOR pathways in controlling growth and sensitivity to therapy-implications for cancer and aging. Aging (Albany NY) 2011; 3:192 - 222; PMID: 21422497
- Janku F, Wheler JJ, Naing A, Stepanek VM, Falchook GS, Fu S, Garrido-Laguna I, Tsimberidou AM, Piha-Paul SA, Moulder SL, et al. PIK3CA mutations in advanced cancers: characteristics and outcomes. Oncotarget 2012; 3:1566 - 75; PMID: 23248156
- Hursting SD, Perkins SN, Phang JM. Calorie restriction delays spontaneous tumorigenesis in p53-knockout transgenic mice. Proc Natl Acad Sci U S A 1994; 91:7036 - 40; http://dx.doi.org/10.1073/pnas.91.15.7036; PMID: 8041741
- Leonova KI, Shneyder J, Antoch MP, Toshkov IA, Novototskaya LR, Komarov PG, Komarova EA, Gudkov AV. A small molecule inhibitor of p53 stimulates amplification of hematopoietic stem cells but does not promote tumor development in mice. Cell Cycle 2010; 9:1434 - 43; http://dx.doi.org/10.4161/cc.9.7.11508; PMID: 20404530